Toxicokinetics of Ag (nano)materials in the soil model Enchytraeus crypticus (Oligochaeta) – impact of aging and concentration†
Received
10th April 2021
, Accepted 6th July 2021
First published on 6th August 2021
Abstract
Silver (Ag) nanomaterials (NMs) are used in many products, eventually reaching the environment at some life stage and as they can be harmful their impact should be assessed. Although research has focused on Ag NM toxicity, less focus has been on toxicokinetics. The aim of this study was to assess the kinetics of Ag nanomaterial (Ag NM300K) and AgNO3 in the soil invertebrate Enchytraeus crypticus. Tests followed OECD guideline 317, with 14 days uptake followed by 14 days elimination in LUFA 2.2 soil. Two sub-lethal concentrations were selected based on enchytraeid sensitivity in a reproduction test (6 and 60 mg Ag per kg for Ag NM300K, and 5 and 45 mg Ag per kg for AgNO3), and spiked soil aged for 3 and 14 days after spiking. Total and 0.01 M CaCl2 extractable soil concentrations were evaluated at day 0, 1 and 14 for all the exposures. Overall, enchytraeids showed increasing Ag uptake with time, followed by a decrease when transferred to clean soil. For the lowest exposure concentrations, the difference in Ag uptake rate constants between 3 and 14 days aging was larger (10-fold) for AgNO3 than for NM300K (uptake rates being highest for soil aged for 3 days), which was in line with the higher CaCl2-extractable Ag concentrations in AgNO3 spiked soil. At the higher exposure concentrations, for AgNO3 the difference in Ag uptake rate constants between 3 and 14 days aged soils was 2-fold, with the bioaccumulation factor (BAF) being highest at 3 days aging. For Ag NM300K, the uptake rate constant was low with virtually no elimination, suggesting that body Ag concentrations may keep on increasing with time leading to a higher risk of longer-term exposure compared to the Ag ions. These findings show the importance of understanding the toxicokinetics of ionic and nano forms of silver and other elements, and the key role of aging in determining NM bioavailability.
Environmental significance
The wide use of silver (Ag) (due to its properties and applications) leads to an increasing release in the environment and potential adverse effects on soil organisms. Changes in silver salt and nanoparticle bioavailability and toxicity (in time) are related with processes of aggregation, agglomeration, dissolution, surface modification, metal speciation and solid phase binding of dissolved ions. For this reason, understanding the toxicokinetics of Ag in soil organisms is key to assess its risk to the environment, because it covers a series of processes that translate the external into internal concentration and respective effects. Our findings confirm that toxicokinetics differ between Ag salt and nano forms and also with soil aging.
|
Introduction
Silver (Ag) has a wide range of applications, mostly due to its antimicrobial activity properties. It is applied in a variety of products, also in the nano form, e.g. daily care products, clothing, sports gear, food packaging, hospital supplies, refrigerators, water disinfectants, air filters or bone cement.1–5 The total production of silver nanomaterials (NMs) in the European Union (EU) was estimated to be 50 tons in 2014.6 Their wide use leads to an increasing release in the environment.5,7,8 Soil is a major sink, where sewage sludge application is one of the largest sources of entry.6,9 As a result, soil organisms are likely exposed to increasing levels of Ag NMs, raising concerns regarding their possible adverse effects.10–13 Silver is a non-essential trace metal and elevated concentrations of Ag NMs are known to cause adverse effects on various soil organisms, e.g. Enchytraeus crypticus,14Eisenia fetida,15Eisenia andrei, Folsomia candida,16Porcellionides pruinosus8 and Lumbricus rubellus.17
Standard ecotoxicity tests as developed to assess the hazards to soil organisms, e.g., the enchytraeid,18 collembolan19 and earthworm reproduction tests,20 involve the exposure to soil (freshly) spiked at a range of concentrations, where endpoints like survival or reproduction are assessed after a certain fixed time of exposure. Chemicals interact with the soil leading to a change in their speciation and bioavailability with time, thus affecting their toxicity.12,21 Dissolution kinetics of NMs translate into complex dynamics of partially dissolved particles, free/complexed ions, adsorption of released ions on the NM surface, etc.22 The importance of considering aging, besides freshly spiked soil, when performing ecotoxicological testing is well known and often highlighted for a variety of chemicals, both organic compounds23,24 and metals.13,22,25–27
Bioavailability of NMs can be determined by measuring uptake in the organism, and to understand internal toxicity elimination kinetics can also be studied.8,28 Toxicokinetics contains a series of processes that translate the external concentration to the internal, including absorption, distribution, metabolism, and excretion (ADME).29 Bioaccumulation studies with AgNO3 and Ag NMs have been performed using E. crypticus,30,31F. candida,32E. andrei,26L. rubellus,33P. pruinosus8 and T. molitor,34 although with different NMs and not including aging.
Hence, the aim of this study was to investigate the toxicokinetics of Ag NMs (and AgNO3 as a reference to account for Ag+ ions released), comparing two aging periods, 3 and 14 days after soil spiking, and 2 exposure concentrations. Although concentration should not influence kinetics, two sub-lethal concentrations were included to further verify the main patterns of Ag uptake in enchytraeids exposed to NMs. Further, the sub-lethal range can be broad and the level of changes induced in the animals, even at sub-lethal exposures, can trigger differences in the process of bioaccumulation. We used the ecologically relevant standard soil model E. crypticus,35,36 which has a widespread distribution in different ecosystems, occurring in large numbers.36 They are representative of the saprophagous mesofauna of the litter layer and play a crucial role in organic matter decomposition and bioturbation, improving the small-scale water and air management of soil, especially when population density is high.37 Although kinetics is not studied, effects with Ag NMs are studied in E. crypticus at various levels, from organism14,38,39 to subcellular, e.g. transcriptomics,40 comet assay,41 so there is considerable level of detail obtained with these particular Ag NM300K. We hypothesized that Ag uptake kinetics would be faster for AgNO3 than for Ag NMs, would not depend on exposure concentration and would be affected by aging.
Materials and methods
Test organism
Enchytraeus crypticus (Enchytraeidae, Oligochaeta) was cultured in agar, consisting of Bacti-Agar medium (Oxoid, Agar No. 1) and a sterilized mixture of 4 different salt solutions at final concentrations of 2 mM CaCl2·2H2O, 1 mM MgSO4, 0.08 mM KCl, and 0.75 mM NaHCO3. Cultures were maintained at 20 ± 1 °C with a 16
:
8 h light
:
dark photoperiod and fed ground autoclaved oats twice a week. Adult animals with well-developed clitellum were selected and used.
Test materials and characterization
Silver nitrate (AgNO3, purity >99%, Sigma-Aldrich) and the reference silver nanomaterial (NM300K), obtained from the European Commission Joint Research Centre (JRC), were used. Ag NM300K is fully characterized.42 In short, Ag NM300K are spherical, consisting of a colloidal dispersion with a nominal silver content of 10.2% w/w, dispersed in 4% w/w of polyoxyethylene glycerol trioleate and polyoxyethylene20 sorbitan monolaurate (Tween 20). Approximately >99% of the particles have a nominal size of 15 nm. Transmission electron microscopy (TEM) showed a size of 17 ± 8 nm and smaller nanoparticles of ca. 5 nm were also present. Further characterization has been done, including in soil media, and is cited throughout the paper.
Test soil and spiking procedures
The natural standard LUFA 2.2 soil (Speyer) was used and had the following main characteristics: pH (0.01 M CaCl2) = 5.6, organic carbon = 1.73%, cation exchange capacity = 9.8 cmolc kg−1, maximum water holding capacity (WHCmax) = 45.8%, and grain size distribution of 8.3% clay (<0.002 mm), 14.9% silt (0.002–0.05 mm), and 76.8% sand (0.05–2.0 mm). The soil was dried at 80 °C for 48 hours before use.
Test concentrations were 5 and 45 mg Ag per kg soil dry weight (DW) for AgNO3 and 6 and 60 mg Ag per kg soil DW for Ag NM300K. These concentrations were selected based on the results of reproduction and full life cycle tests with E. crypticus exposed to freshly spiked soil Bicho et al.,14 being sub-lethal and representing a higher and lower effect, approx. corresponding to the EC10 and EC50.
Spiking was done by mixing aqueous solutions of both materials with the pre-moistened soil. For AgNO3 an aqueous stock solution was prepared, serially diluted and added to soil batches, which were then mixed to obtain a homogeneous distribution and split into replicates. The same procedure was applied for the lowest concentration of Ag NM300K (6 mg Ag per kg). For the higher concentration of Ag NM300K, spiking was done replicate by replicate. Soil moisture content was adjusted to 50% of the WHCmax. Prior to test start, soils were aged for 3 and 14 days at 20 ± 1 °C with a 16
:
8 h light
:
dark photoperiod (same conditions as used in the test).
Experimental procedure
In total, 8 bioaccumulation tests were performed, implementing a full factorial design: 2 test materials (Ag NM300K and AgNO3), 2 concentrations (EC10, EC50) and 2 aging periods (3 and 14 days). The aging behaviour of NMs can differ from that of the ionic metal forms and hence it is important to explore its impacts. The OECD guideline 317 (ref. 43) was followed. In short, 10 adult enchytraeids with well-developed clitellum and of similar size were introduced into each test vessel containing 20 g of moist soil (water control, NM300K dispersant control, spiked Ag materials) and fed with 15 mg of ground oats. After 14 days in the spiked soil (uptake phase), the surviving animals were transferred to clean soil for another 14 days (elimination phase). Animals were sampled at 7 plus 6 time points (uptake: 0, 1, 2, 4, 7, 10 and 14 days; elimination: 15, 16, 18, 21, 24, and 28 days). The tests ran at 20 ± 1 °C in a 16
:
8 h light
:
dark photoperiod. During the test, food (15 mg) and water content (based on weight loss) were replenished weekly. Five replicates were used per treatment/sampling day. At each sampling day, the animals were transferred from soil to ISO water44 for 10 hours to purge their guts from soil particles. After this, the animals were blotted dry on filter paper; five animals were introduced into cryotubes individually (i.e. 1 organism per tube in 5 cryotubes). All animals were frozen at −20 °C until further analysis. Five replicate soil samples were collected at each sampling day (10 for the control), dried at 40 °C for 48 hours and stored at −20 °C for further analysis.
Chemical analysis
Animals were freeze-dried for 24 h, weighted individually and digested with 300 μL of a mixture of HNO3 (65%; Mallbaker Ultrex Ultra-Pure) and HClO4 (70%; Mallbaker Ultrex Ultra-Pure) (7
:
1 v/v) in a block heater (TCS Metallblock Thermostat) using a heating ramp ranging from 85 to 180 °C. After all acid was evaporated, the residue left was dissolved in 300 μl of 1 M HCl and Ag concentrations in the digests were measured by graphite furnace atomic absorption spectrometry (AAS; PinAAcle 900Z, Perkin Elmer, Germany). For quality control of the analysis, the certified reference material DOLT 4 was included in the analysis. Mean (±SD; n = 3) silver concentrations measured in DOLT 4 were 79.8 ± 9.4% of the certified values. Limit of detection (LOD) for Ag was 0.003 mg kg−1.
To determine total Ag concentrations in the test soil, 130 mg samples from days 0, 1 and 14 (3 replicates each) were digested using 2 ml of a destruction mixture of HNO3 (65%, Sigma-Aldrich) and HCl (37%, Sigma-Aldrich) (1
:
4 v/v), in Teflon containers that were closed tightly and heated at 140 °C for 7 hours. Samples from days 15 and 28 of the elimination phase were also analyzed. After cooling, 8 ml of deionized water was added, and the metal concentrations measured by Flame AAS (AAnalyst 100; Perkin Elmer; Germany). Certified reference material ISE sample 989 was included in the analysis; mean (±SD; n = 2) silver concentrations measured in the reference material were 92.4 ± 7.94% of the certified values, respectively. LOD for Ag analysis in soil samples was 0.003 mg Ag per kg dry soil.
For the measurement of the available Ag concentrations, 25 ml of 0.01 M CaCl2 solution and 5 g dry soil were shaken for 2 hours at 200 rpm, using samples from days 0, 1 and 14 (3 replicates each). After sedimentation overnight, pH was measured, and the supernatant was filtered over 0.45 μm nylon syringe filters. The Ag concentration in the 0.01 M CaCl2 extract was measured by Flame AAS (AAnalyst 100; Perkin Elmer; Germany). LOD for Ag analysis in CaCl2 extracts was 0.003 mg Ag per kg dry soil.
Data analysis
Uptake and elimination kinetics of Ag was modelled using a one-compartment model, assuming constant exposure concentrations,45 fitting data to eqn (1A) and (1B) simultaneously:
Uptake phase:
|  | (1A) |
Elimination phase:
|  | (1B) |
where
Ct is the Ag concentration in the enchytraeids after
t days of exposure (mg kg
−1 dry body),
C0 is the background concentration in the enchytraeids (mg kg
−1 dry body),
Ku is the uptake rate constant (kg soil per kg animal per day),
Ke is the elimination rate constant (day
−1),
Kgrowth the growth rate estimated using an exponential growth model (day
−1),
Cexp the exposure concentration (mg kg
−1 soil DW),
t the exposure time (days) and
tc the time when the animals were transferred to clean soil.
The uptake and elimination rate constants were calculated using both the total and available (CaCl2-extractable) concentrations as the exposure concentration (Cexp).
Because extractable concentrations showed a decline in the soil spiked in some of the exposures (see below), the decrease rate was calculated as Kdeg (per day):
| 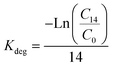 | (2) |
where
C0 and
C14 are the 0.01 M CaCl
2-extractable Ag concentrations at days 0 and 14 (mg kg
−1 dry soil). To correct for this decrease, the decline rate of the available concentration in soil (
Kdeg) was inserted in the kinetics equations to yield:
Uptake phase:
|  | (3A) |
Elimination phase:
|  | (3B) |
The bioaccumulation factor (BAF) (kg soil per kg organism), the ratio between the concentration in the organisms (mg kg
−1 body DW) and the concentration in the soil (mg kg
−1 soil DW) at steady state, was calculated as the ratio of the uptake (
Ku) and the elimination rate constants (
Ke):
|  | (4) |
The half-life for Ag elimination from the enchytraeids was calculated as:
|  | (5) |
One-way analysis of variance (ANOVA) followed by Holm–Sidak method comparison
post hoc test (
p ≤ 0.05) was used to assess significance of the differences between total and CaCl
2 extractable concentrations measured at days 0, 1 and 14.
46
Results
Material characterization
The measured total Ag concentrations in the test soil were 75–90% of the nominal concentration, except for 60 mg Ag per kg soil of Ag NM300K after 3 days aging where it was 60% (Fig. 1, Table S1†). Ag concentrations in the control soil as well as in the soil from the elimination phase (days 15 and 28) generally were below the detection limit.
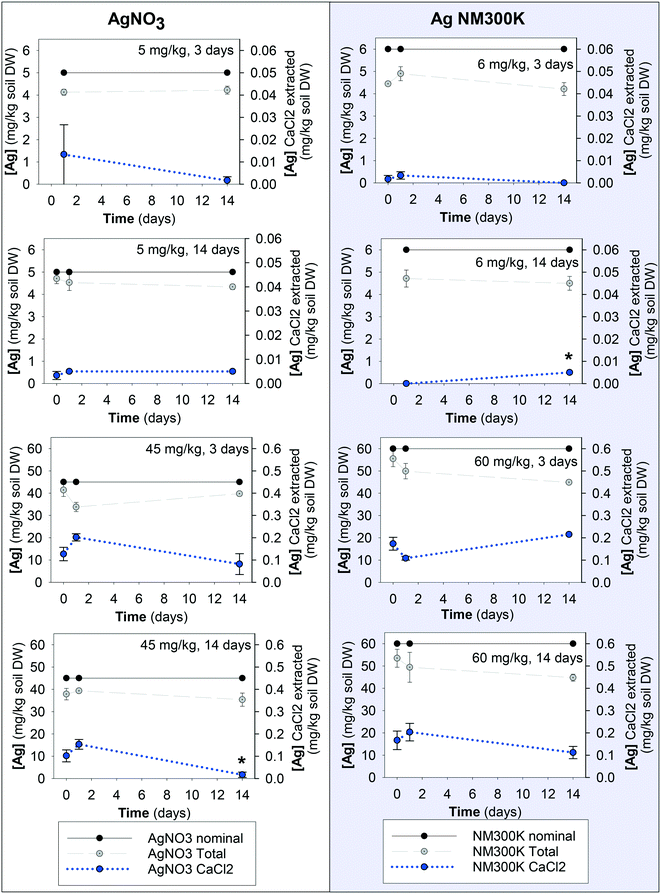 |
| Fig. 1 Nominal, measured total and 0.01 M CaCl2-extractable silver concentrations in LUFA 2.2 soil spiked with AgNO3 and Ag NM 300 K. Tested concentration shown in mg kg−1 soil DW and 3 and 14 days indicate aging periods of 3 and 14 days, respectively. Values are expressed as average ± standard error. *Significant difference between days according to Holm–Sidak post hoc test (p < 0.05). | |
The CaCl2-extractable Ag concentration in the spiked soils showed an increase with increasing total soil concentration (R2 = 0.93). For AgNO3, the CaCl2-extractable concentrations decreased with aging time, while for Ag NM300K there was a significant increase for the lowest exposure concentration after 14 days of aging of the soil. At 60 mg Ag NM300K per kg soil DW exposure, the CaCl2-extractable concentrations decreased with aging time (Fig. 1).
For AgNO3 at 5 mg Ag per kg soil DW aged for 3 days, Kdeg was 0.015 day−1, for the 14 days there was no change. For AgNO3 at 45 mg Ag per kg soil DW, aged for 3 and 14 days, the Kdeg values were 0.049 and 0.15 day−1, respectively. For Ag NM300K at 60 mg Ag per kg soil DW aged for 14 days, Kdeg was 0.036 day−1, with no change for the remaining.
Toxicokinetics
The average survival of the enchytraeids at the end of the 28 day test period is given in Table 1. The validity criteria (≥80% survival) was achieved for nearly all treatments; major exception was the exposure to 60 mg Ag NM300K aged for 3 days where high mortality was seen.
Table 1 Survival and growth rate (Kgrowth) (AV ± SE) of Enchytraeus crypticus during the 28 day period of uptake/elimination kinetics exposure to AgNO3 and Ag NM300K in LUFA 2.2 soil. Kgrowth is based on dry weight increases, and was calculated for the whole 28 days of exposure, using an exponential growth model
Test material |
Aging (days) |
Concentration (mg Ag per kg soil) |
Survival (%) |
K
growth (day−1) |
AgNO3 |
3 |
0 |
84 ± 5.1 |
0.0420 ± 0.018 |
3 |
5 |
84 ± 4.0 |
0.0914 ± 0.009 |
3 |
45 |
60 ± 3.2 |
0.0773 ± 0.007 |
14 |
0 |
78 ± 3.7 |
0.0645 ± 0.015 |
14 |
5 |
82 ± 6.3 |
0.0765 ± 0.006 |
14 |
45 |
68 ± 5.8 |
0.0846 ± 0.007 |
Ag NM300K |
3 |
0 |
82 ± 5.8 |
0.0345 ± 0.018 |
3 |
Dispersant |
74 ± 13.6 |
0.0823 ± 0.017 |
3 |
6 |
73 ± 8.8 |
0.0802 ± 0.009 |
3 |
60 |
20 ± 20 |
0.0888 ± 0.016 |
14 |
0 |
78 ± 3.7 |
0.0413 ± 0.014 |
14 |
Dispersant |
80 ± 5.8 |
— |
14 |
6 |
88 ± 4.9 |
0.0783 ± 0.007 |
14 |
60 |
88 ± 7.3 |
0.0621 ± 0.011 |
The enchytraeids gained weight during the 28 day exposure period. The differences in organism weights were large enough to justify inclusion of the growth rate in the kinetics equations, to account for the potential growth dilution effect on the body silver concentrations (Table 1).
The uptake and elimination kinetics of Ag (Fig. 2) is shown for all exposures except the 60 mg kg−1 soil DW of Ag NM300K aged for 3 days where high mortality occurred and too few organisms were available to adequately model Ag uptake kinetics.
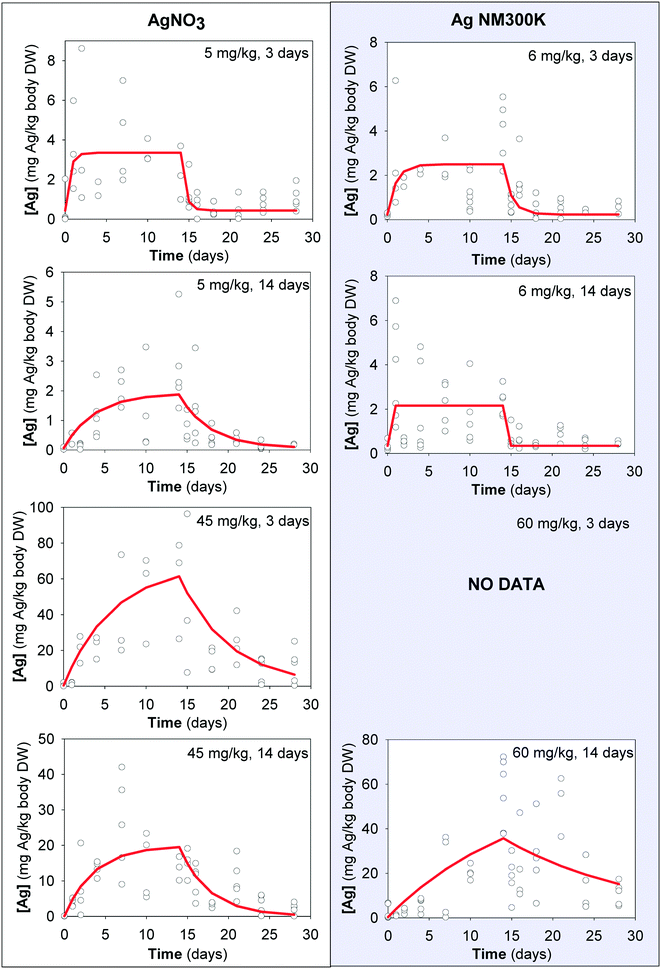 |
| Fig. 2 Uptake and elimination kinetics of Ag in Enchytraeus crypticus exposed to AgNO3 (left) and Ag NM300K (right) in LUFA 2.2 soil. Tested concentrations in mg kg−1 soil DW and 3 and 14 indicate aging periods of 3 and 14 days, respectively. Red lines represent the first-order one-compartment model (eqn (1)) fitted to the data, based on total soil concentrations and corrected for E. crypticus growth rate during the 28 day test period. Each dot is a measured replicate. | |
The kinetic parameters for Ag uptake in E. crypticus, calculated applying the one-compartment model to the data, are shown in Table 2. For AgNO3 [5 mg Ag per kg soil DW], uptake rate constant was approximately 10 fold higher in soil aged for 3 days compared with 14 days (Ku 1.31 vs. 0.11 kg soil per kg animal per day); the same was observed for AgNO3 [45 mg Ag per kg soil DW] (Ku 0.29 vs. 0.15 kg soil per kg animal per day) although with only 2-fold difference. For AgNO3 [5 mg Ag per kg soil DW], the elimination rate constant was similarly 10 fold higher for 3-day aged soil (Ke 1.8 vs. 0.2 day−1). Internal Ag concentration was about 10 fold higher for the exposure to 45 mg Ag per kg soil DW compared to 5 mg Ag per kg soil DW. The BAF was similar for all treatments except for AgNO3 [45 mg Ag per kg soil DW, 3 days aged], being up to 3.3 (vs. 0.73).
Table 2 Toxicokinetic model parameters for the uptake and elimination of silver in Enchytraeus crypticus exposed AgNO3 and Ag NM300K in LUFA 2.2 natural soil aged for 3 or 14 days. Cexp: exposure concentration in spiked soil, expressed as total or 0.01 M CaCl2-extractable concentration, C0: background concentration in the animals, Ku: uptake rate constant, Kdeg: decrease rate of CaCl2-extractable concentrations, Ke: elimination rate constant, BAF: bioaccumulation factor, DT50: time needed to eliminate 50% of the metal. Values were calculated relating internal Ag concentrations in the enchytraeids to total and 0.01 M CaCl2-extractable concentrations in the soil
Aging (days) |
Concentration (mg Ag per kg soil) |
Base |
C
exp (mg kg−1 soil) |
C
0 (mg kg−1 body DW) |
K
u (kg soil per kg animal per day) |
K
u with Kdeg (kg soil per kg animal per day) |
K
e (per day) |
K
e with Kdeg (per day) |
BAF without/with Kdeg |
DT50 without/with Kdeg (days) |
AgNO3 |
3 |
5 |
Total |
4.17 |
0.44 |
1.31 (−0.07–2.7) |
— |
1.8 (−0.3–3.8) |
— |
0.73
|
0.39/2.2 |
CaCl2 |
0.02 |
243 (−12.7–499) |
123 (60.3–186) |
0.31 (0.08–0.5) |
136/394 |
14 |
5 |
Total |
4.52 |
0.06 |
0.11 (0.05–0.17) |
— |
0.2 (0.04–0.34) |
— |
0.58
|
3.66 |
CaCl2 |
0.004 |
111 (50.7–172) |
— |
— |
587 |
3 |
45 |
Total |
38.3 |
0.44 |
0.29 (0.17–0.42) |
— |
0.0887 (0.007–0.17) |
— |
3.3
|
7.81/13.9 |
CaCl2 |
0.14 |
82.0 (46.2–118) |
92.6 (53.5–132) |
0.05 (−0.01–0.01) |
924/1866 |
14 |
45 |
Total |
37.5 |
0.06 |
0.15 (0.09–0.21) |
|
0.19 (0.08–0.31) |
— |
0.76
|
3.63/16.3 |
CaCl2 |
0.09 |
60.4 (35.9–84.9) |
76.3 (53.7–98.7) |
0.04 (−0.01–0.09) |
316/1787 |
Ag NM300K |
3 |
6 |
Total |
4.52 |
0.23 |
9.74 (−) |
— |
20.5 (−) |
— |
0.47
|
0.034 |
CaCl2 |
0.002 |
26 273 (−) |
— |
20.4 |
— |
1285 |
14 |
6 |
Total |
4.61 |
0.35 |
6.66 (−) |
— |
16.8 (−) |
— |
0.40
|
0.041 |
CaCl2 |
0.003 |
11 318 (−) |
— |
15.5 (−) |
— |
729 |
0.045 |
14 |
60 |
Total |
49.2 |
0.35 |
0.08 (0.05–0.11) |
— |
0 |
— |
— |
— |
CaCl2 |
0.16 |
23.5 (14.3–30.8) |
29.9 (16.7–43.2) |
0 |
— |
— |
For Ag NM300K [6 mg Ag per kg soil DW] uptake rate constant was slightly higher in 3 day aged compared to 14 day aged soil (Ku 9.7 vs. 6.6 kg soil per kg animal per day), with similar elimination rate constants (Ke 20.5 vs. 16.8 per day), showing similar BAF values (0.47 vs. 0.4). For NM300K [60 mg Ag per kg soil DW], no comparison is possible between soil aging periods given the low survival in 3 days aged soil. For NM300K [60 mg Ag per kg soil DW, 14 days aged], a very low uptake rate constant was found (Ku 0.08 kg soil per kg animal per day) and the elimination rate constant was close to zero. The observed decrease of the Ag concentration in the animals during the elimination phase can in part be attributed to animal growth dilution rather than to elimination of the Ag from the body.
Relating Ag toxicokinetics to CaCl2-extractable Ag concentrations in the test soil generally reduced the difference in Ku and BAF values between treatments. Only exception was the lowest Ag NM300K concentration which gave an extremely high Ku value, but did give a similar BAF value compared to the AgNO3 treatments.
Discussion
Effect of aging on Ag bioavailability
Despite the similar concentration range tested for AgNO3 and Ag NM300K, the CaCl2-extractable concentrations increased between days 0 and 14 for Ag NM300K but decreased for AgNO3. Diez-Ortiz et al.25 also reported decreasing extractable Ag concentrations from AgNO3 with time in soil incubated for up to 50 weeks, possibly because Ag+ binds stronger to soil. Increasing extractable concentrations upon aging of NMs have also been reported previously,17,25 suggesting that the increase in dissolution of Ag ions from Ag NMs may cause the NMs to serve as a continuous source of Ag+ in media within a longer time period.
Nevertheless, the differences in CaCl2-extractable Ag concentrations between AgNO3 and Ag NM300K spiked soils do not fully explain the observed toxicity of Ag NM300K at 60 mg Ag per kg soil DW [3 days aged], where survival was significantly lower. This result was not expected based on Bicho et al.14 who reported an EC50 for effects of Ag NM300K on the reproduction of E. crypticus of 161 mg Ag per kg in soil also aged for 3 days. The soil measurements refer to Ag concentrations and no assessment could be done regarding the particle size for NM300K, hence it is unknown if the measured Ag was present as nanoparticles or ions, or how much of each fraction was present. There is evidence that Ag NM300K is present as both nanoparticulate and ionic Ag from an equivalent soil exposure.47 Given the observed effects, which differ from AgNO3, it must be considered that previous observations showed higher toxicity of NMs at a lower concentration window, with a non-monotonic dose-related response pattern. This was e.g. shown for Ag at approximately 20 mg Ag NM300K per kg in E. crypticus,14 or at 15 mg Ag NM per kg for L. rubellus,17 and for Ni at approximately 100 mg Ni NPs per kg in E. crypticus.48 Such non-monotonic dose–response relationships have been further confirmed, e.g. for NM300K, where Rodrigues et al.38 studied the effects of 10, 20, 30 and 40 mg L−1 in an aqueous exposure to E. crypticus, showing 40 > 20 > 30 > 10 mg L−1 toxicity for time to hatch, a precursor for observed effects on reproduction (juvenile numbers). Hence, there seems to be an “optimum” lower concentration for maximum dispersion/toxicity. The observed effect of Ag NM300K at 60 mg Ag per kg soil could be the result of the fact that in this study a higher mass of soil was used, 20 g soil instead of 10 g per replicate used by Bicho et al. (2016).14 As a consequence, the biomass/soil ratio was different in both tests, which perhaps may have affected speciation or dissolution of the NMs or its interaction with the enchytraeids. Although this may seem not very likely, it is the only experimental difference between the studies (current and ref. 14), and it is clear that the highest toxicity occurs in this narrow range.
Toxicokinetics
For AgNO3, overall results showed a faster uptake and elimination of Ag in the soil aged for 3 days compared to 14 days, which seems to be in line with the lower CaCl2-extractable concentrations, i.e. a stronger binding of the Ag to binding sites in the soil. Whereas the exposure to 45 mg Ag per kg soil of AgNO3 showed a 2-fold decrease in Ku values between 3 and 14 days aged soil, the exposure to 5 mg showed a 10-fold decrease. This could be due to a depletion (over time) of the available Ag at the lower exposure concentrations. Comparing the 5 and 45 mg Ag per kg soil, at 3 days aged soil there was a higher uptake rate constant for the lower concentration compared to the higher (1.31 and 0.29 kg soil per kg animal per day, respectively) and at 14 days aged soil the uptake rate constants were similar (0.11 and 0.15 kg soil per kg animal per day, respectively). As seen, at day 14 the uptake rate for the 5 mg kg−1 exposure decreased to the uptake rate level of 45 mg kg−1 exposure. This is in line with that the depletion and aging effects are more rapid at lower exposures, as also seen in the CaCl2 concentration i.e. a relatively stronger decrease in the lower exposure. The uptake rate constant was highest for the lowest concentration (i.e. for 3 days aging), which is opposite what was observed in a study with L. rubellus exposed to 20 and 100 mg kg−1 of AgNO3 for 7 days,33 where the highest available concentrations and uptake rate constants were measured in the soils spiked with the highest concentration of AgNO3. The same trend, as for the L. rubellus, was shown for F. candida when exposed for 28 days in a soil spiked with AgNO3: higher spiked concentrations showed higher uptake rate constant.17,32 The BAF was highest for exposure to AgNO3 [45 mg Ag per kg soil DW, 3 days aged] (BAF = 3.3), hence this particular combination of higher Ag concentration and less soil aging induced higher risk.
Through the process of aging, the bioavailability and toxicity of Ag in the ionic form are reduced.17,25 The current knowledge for NMs is that equilibrium is not reached,49 and as a result a continuous change can be expected over time. We hypothesize that if it would be possible to fully analyze the exposure media, we would probably find a complex matrix with NPs in different states of aggregation and agglomeration, in different states of dissolution, free and complexed Ag ions and Ag ions adsorbed on the nanoparticle surfaces.22
For Ag NM300K [6 mg Ag per kg soil DW], no major differences occurred between 3 and 14 days soil aging, but again, uptake rate constant was higher at 3 days aging. The difference in uptake rate constants for AgNO3 between 3 and 14 days aging was in line with the higher CaCl2-extractable Ag concentrations measured in AgNO3 spiked soil. Nevertheless, the BAF (0.4) was relatively low and similar for both AgNO3 [5 mg Ag per kg soil DW] and NM300K [6 mg Ag per kg soil DW], suggesting a similar ability to deal with Ag accumulation by the enchytraeids.
For Ag NM300K [60 mg Ag per kg soil DW], obviously no comparison between 3 and 14 days aging is possible, but the Ag NM300K [60 mg Ag per kg soil DW, 14 days aged] showed a distinct kinetics compared to 6 mg Ag per kg, with very low uptake rate constant (Ku 0.08 kg soil per kg animal per day) and a very low (almost zero) elimination rate constant. A study where E. fetida was exposed to Ag NPs25 reported a higher accumulation of Ag from NPs in the exposure to the lowest concentrations (45 and 112 mg Ag per kg), which is in agreement with our findings.
One possible explanation for the differences between the ionic and nano Ag forms in regard to Ag toxicokinetics patterns could be related to the agglomeration/aggregation state of the NMs at higher concentrations. Hence, for the nanoform such an increased agglomeration/aggregation at higher concentration would lead to a lower bioavailability and therefore a lower uptake by the organisms.50 This would not be the case for the salt. Further, there is likely also a less homogeneous distribution of the NMs in soil as we did observe a relatively large variation of Ag content in the organism's tissue between replicates. So the scenario could be that for Ag NM300K some animals encounter higher exposure and hence high uptake while others do not, leading to greater differences between replicates and possibly also explaining the increased mortality. As further observed, a slower elimination occurred from Ag NM300K [60 mg Ag per kg soil DW, 14 days aged]. This could mean that once taken up, Ag NM300K are more strongly sequestered inside the animals and hence are more difficult to excrete. Apparently, the way the Ag (among other) from the NMs is retained by the animals differs from that for the ionic Ag form,14,40,48,51 which suggests that also some nanoparticulate Ag was taken up by the animals exposed to the higher NM concentration.
Diez-Ortiz et al.25 highlighted the need to allow soil aging/equilibration for long enough for a proper assessment of the risk of Ag NMs and in which case the observed effects for salt and Ag NMs become similar: the uptake rate constants for Ag in E. fetida exposed to the same soil concentration of 15 mg Ag per kg for Ag NMs and AgNO3 were similar after 56 days aging (Ku 0.061 and 0.055 kg soil per kg animal per day, respectively).27 Nevertheless, even though Ke was close to zero at 60 mg kg−1 for AgNM300K aged for 14 days, the elimination curve did show a decrease of the Ag concentration in the animals, which could be explained by growth dilution. The shorter soil aging time used in our study (14 days) compared to 56 day period used in Baccaro et al.27 can help to explain the differences in the results. In any case, the results seem to point to the need to pay particularly attention to the impact of aging of Ag NMs, indicating a higher risk of longer term exposure compared to the Ag ions. The overall recommendation for aging around 2–3 weeks before testing substances could probably be increased for testing of NMs, although this will be case specific and a comparison between aging periods is still of interest at this stage.
Overall, the uptake rate constants of silver in the enchytraeids were higher for the Ag NM300K exposure than for AgNO3. Contrary to our results, Diez-Ortiz et al.33 observed higher Ag uptake in earthworms for ionic Ag than for NMs. A summary of other toxicokinetics studies performed with different forms of silver is shown in Table S2,† including different soil organisms: E. crypticus;30,31E. andrei;26E. fetida,27L. rubellus,33F. candida32 and T. molitor,34 in reference and field soils, and with varying exposure periods. For instance, E. crypticus exposed for 10 days in a quartz sand media presented higher uptake and elimination rate constants both for the ionic and nano Ag forms compared to our results.31 This can be explained by the fact that, in the inert sand matrix used, sorption of Ag was expected to be very low or absent, leading to a much higher bioavailability of both Ag forms. The different results observed in the literature are obtained using different test designs, materials and species, making them very hard to compare.
Changes in silver salt and nanoparticle bioavailability and toxicity are related with processes of particle aggregation, agglomeration and dissolution, surface modification, metal speciation and solid phase binding of dissolved ions.25 All of the mentioned factors will have an effect on the accumulation, and consequently on the toxicity of silver to the organisms. For this reason, understanding of the toxicokinetics of silver, ionic and NMs, in soil organisms is a better way to assess their risk to the environment.
Conclusion
The toxicokinetics of AgNO3 and Ag NM300K in the soil invertebrate Enchytraeus crypticus was adequately described by a one-compartment model at both tested aging periods and exposure concentrations. For the low exposure concentrations (AgNO3 [5 mg Ag per kg soil DW] and Ag NM300K [6 mg Ag per kg soil DW]), the difference in Ag uptake rate constants between 3 and 14 days aging was far higher (10-fold) for AgNO3 than for Ag NM300K, with BAF values 0.73 and 0.47 respectively. This was in line with the higher CaCl2-extractable Ag concentrations measured in AgNO3 spiked soil. For the higher exposure concentrations, the effect of aging was very small (2-fold) for the uptake rate constants of AgNO3 [45 mg Ag per kg soil DW], but he BAF was highest at 3 days aging (3.3). For Ag NM300K [60 mg Ag per kg soil DW], the Ag uptake rate constant was quite low with virtually no elimination. This could mean that upon Ag NM exposure body Ag concentrations keep increasing for longer time leading to a higher risk of longer-term exposure compared to the Ag ions. It is clear that change (as observed here) may continue beyond 14 days and will change in other soil conditions. These findings show the importance of understanding the toxicokinetics of ionic and nano forms of silver and other elements, and the key role of aging in determining NM bioavailability.
Author contributions
FCFS: formal Analysis, investigation, methodology, writing – original draft, PST: investigation, CAMVG, JJSF, MJBA: conceptualization, data curation, formal Analysis, funding acquisition, resources, supervision, writing – original draft. All authors: writing – review & editing.
Conflicts of interest
There are no conflicts of interest to declare.
Acknowledgements
This study was supported by the European Commission Project H2020-NMBP-2017 BIORIMA (GA No. 760928) and H2020-NMBP-13-2018 NANORIGO (GA No. 814530). Thanks are due to FCT/MCTES for the financial support to CESAM (UIDP/50017/2020 + UIDB/50017/2020) through national funds and FCT via a PhD grant to F. Santos (SFRH/BD/118294/2016). The authors would like to thank Rudo Verweij for his technical support in the chemical analysis.
References
- N. Hadrup and H. R. Lam, Oral toxicity of silver ions, silver nanoparticles and colloidal silver – A review, Regul. Toxicol. Pharmacol., 2014, 68(1), 1–7, DOI:10.1016/j.yrtph.2013.11.002.
- N. R. Panyala, E. M. Peña-Méndez and J. Havel, Silver or silver nanoparticles: a hazardous threat to the environment and human health?, J. Appl. Biomed., 2008, 6(3), 117–129, DOI:10.32725/jab.2008.015.html.
- F. Ribeiro, C. A. M. Van Gestel, M. D. Pavlaki, S. Azevedo, A. M. V. M. Soares and S. Loureiro, Bioaccumulation of silver in Daphnia magna : Waterborne and dietary exposure to nanoparticles and dissolved silver, Sci. Total Environ., 2017, 574, 1633–1639 CrossRef CAS PubMed , Available from: https://linkinghub.elsevier.com/retrieve/pii/S0048969716319076.
- J. Fabrega, S. N. Luoma, C. R. Tyler, T. S. Galloway and J. R. Lead, Silver nanoparticles: Behaviour and effects in the aquatic environment, Environ. Int., 2011, 37(2), 517–531, DOI:10.1016/j.envint.2010.10.012.
- R. Behra, L. Sigg, M. J. D. Clift, F. Herzog, M. Minghetti and B. Johnston,
et al. Bioavailability of silver nanoparticles and ions: from a chemical and biochemical perspective, J. R. Soc., Interface, 2013, 10(87), 20130396 CrossRef PubMed , Available from: http://rsif.royalsocietypublishing.org/content/10/87/20130396.full.pdf+html%0Ahttp://ovidsp.ovid.com/ovidweb.cgi?T=JS&PAGE=reference&D=emed14&NEWS=N&AN=369697722.
- T. Y. Sun, N. A. Bornhöft, K. Hungerbühler and B. Nowack, Dynamic Probabilistic Modeling of Environmental Emissions of Engineered Nanomaterials, Environ. Sci. Technol., 2016, 50(9), 4701–4711, DOI:10.1021/acs.est.5b05828.
- M. E. Vance, T. Kuiken, E. P. Vejerano, S. P. McGinnis, M. F. Hochella and D. Rejeski,
et al. Nanotechnology in the real world: Redeveloping the nanomaterial consumer products inventory, Beilstein J. Nanotechnol., 2015, 1769–1780 CrossRef CAS , Available from: http://www.beilstein-journals.org/bjnano/content/6/1/181.
- P. S. Tourinho, C. A. M. van Gestel, A. J. Morgan, P. Kille, C. Svendsen and K. Jurkschat,
et al. Toxicokinetics of Ag in the terrestrial isopod Porcellionides pruinosus exposed to Ag NPs and AgNO3 via soil and food, Ecotoxicology, 2016, 25(2), 267–278, DOI:10.1007/s10646-015-1585-7.
- A. E. Pradas del Real, H. Castillo-Michel, R. Kaegi, B. Sinnet, V. Magnin and N. Findling,
et al. Fate of Ag-NPs in Sewage Sludge after Application on Agricultural Soils, Environ. Sci. Technol., 2016, 50(4), 1759–1768, DOI:10.1021/acs.est.5b04550.
- S. Makama, J. Piella, A. Undas, W. J. Dimmers, R. Peters and V. F. Puntes,
et al. Properties of silver nanoparticles influencing their uptake in and toxicity to the earthworm Lumbricus rubellus following exposure in soil, Environ. Pollut., 2016, 218, 870–878, DOI:10.1016/j.envpol.2016.08.016.
- Y. Chen, Y. Si, D. Zhou and F. Dang, Differential bioaccumulation patterns of nanosized and dissolved silver in a land snail Achatina fulica, Environ. Pollut., 2017, 222, 50–57, DOI:10.1016/j.envpol.2017.01.007.
- R. A. González-Fuenzalida, L. Sanjuan-Navarro, Y. Moliner-Martínez and P. Campíns-Falcó, Quantitative study of the capture of silver nanoparticles by several kinds of soils, Sci. Total Environ., 2018, 630, 1226–1236, DOI:10.1016/j.scitotenv.2018.02.307.
- A. R. Whitley, C. Levard, E. Oostveen, P. M. Bertsch, C. J. Matocha, F. Kammer and Von Der,
et al. Behavior of Ag nanoparticles in soil: Effects of particle surface coating, aging and sewage sludge amendment, Environ. Pollut., 2013, 182, 141–149, DOI:10.1016/j.envpol.2013.06.027.
- R. C. Bicho, T. Ribeiro, N. P. Rodrigues, J. J. Scott-Fordsmand and M. J. B. Amorim, Effects of Ag nanomaterials (NM300K) and Ag salt (AgNO3) can
be discriminated in a full life cycle long term test with Enchytraeus crypticus, J. Hazard. Mater., 2016, 318, 608–614 CrossRef CAS PubMed , Available from: http://www.ncbi.nlm.nih.gov/pubmed/27474850.
- S. I. L. Gomes, D. Hansen, J. J. Scott-Fordsmand and M. J. B. Amorim, Effects of silver nanoparticles to soil invertebrates: Oxidative stress biomarkers in Eisenia fetida, Environ. Pollut., 2015, 199, 49–55 CrossRef CAS PubMed , Available from: https://linkinghub.elsevier.com/retrieve/pii/S0269749115000159.
- A. H. Jesmer, J. R. Velicogna, D. M. Schwertfeger, R. P. Scroggins and J. I. Princz, The toxicity of silver to soil organisms exposed to silver nanoparticles and silver nitrate in biosolids-amended field soil, Environ. Toxicol. Chem., 2017, 36(10), 2756–2765, DOI:10.1002/etc.3834.
- M. J. C. van der Ploeg, R. D. Handy, P. L. Waalewijn-Kool, J. H. J. van den Berg, Z. E. Herrera Rivera and J. Bovenschen,
et al. Effects of silver nanoparticles (NM-300K) on Lumbricus rubellus earthworms and particle characterization in relevant test matrices including soil, Environ. Toxicol. Chem., 2014, 33(4), 743–752, DOI:10.1002/etc.2487.
- OECD. Test No. 220: Enchytraeid Reproduction Test [Internet]. OECD; 2016, (OECD Guidelines for the Testing of Chemicals, Section 2), Available from: https://www.oecd-ilibrary.org/environment/test-no-220-enchytraeid-reproduction-test_9789264264472-en.
- OECD. Test No. 232: Collembolan Reproduction Test in Soil [Internet]. Test No. 232: Collembolan Reproduction Test in Soil. OECD Publishing; 2009, pp. 1–19, Available from: https://www.oecd-ilibrary.org/environment/test-no-232-collembolan-reproduction-test-in-soil_9789264076273-en.
- OECD. Test No. 222: Earthworm Reproduction Test (Eisenia fetida/Eisenia andrei) [Internet]. OECD Guidelines for the Testing of Chemicals. OECD Publishing; 2004, pp. 1–18, Available from: http://www.oecd-ilibrary.org/environment/test-no-222-earthworm-reproduction-test-eisenia-fetida-eisenia-andrei_9789264070325-en.
- G. Cornelis, C. DooletteMadeleine Thomas, M. J. McLaughlin, J. K. Kirby, D. G. Beak and D. Chittleborough, Retention and Dissolution of Engineered Silver Nanoparticles in Natural Soils, Soil Sci. Soc. Am. J., 2012, 76(3), 891–902 CrossRef CAS , Available from: https://www.soils.org/publications/sssaj/abstracts/76/3/891.
- S. K. Misra, A. Dybowska, D. Berhanu, S. N. Luoma and E. Valsami-Jones, The complexity of nanoparticle dissolution and its importance in nanotoxicological studies, Sci. Total Environ., 2012, 438, 225–232, DOI:10.1016/j.scitotenv.2012.08.066.
- N. Chung and M. Alexander, Effect of soil properties on bioavailability and extractability of phenanthrene and atrazine sequestered in soil, Chemosphere, 2002, 48(1), 109–115 CrossRef CAS , Available from: https://linkinghub.elsevier.com/retrieve/pii/S0045653502000450.
- M. Alexander, Aging, bioavailability, and overestimation of risk from environmental pollutants, Environ. Sci. Technol., 2000, 34(20), 4259–4265 CrossRef CAS.
- M. Diez-Ortiz, E. Lahive, S. George, A. Ter Schure, C. A. M. Van Gestel and K. Jurkschat,
et al. Short-term soil bioassays may not reveal the full toxicity potential for nanomaterials; bioavailability and toxicity of silver ions (AgNO3) and silver nanoparticles to earthworm Eisenia fetida in long-term aged soils, Environ. Pollut., 2015, 203, 191–198, DOI:10.1016/j.envpol.2015.03.033.
- J. R. Velicogna, D. M. Schwertfeger, A. H. Jesmer, R. P. Scroggins and J. I. Princz, The bioaccumulation of silver in Eisenia andrei exposed to silver nanoparticles and silver nitrate in soil, NanoImpact, 2017, 6, 11–18, DOI:10.1016/j.impact.2017.03.001.
- M. Baccaro, A. K. Undas, J. de Vriendt, J. H. J. van den Berg, R. J. B. Peters and N. W. van den Brink, Ageing, dissolution and biogenic formation of nanoparticles: how do these factors affect the uptake kinetics of silver nanoparticles in earthworms?, Environ. Sci.: Nano, 2018, 5(5), 1107–1116, 10.1039/C7EN01212H.
- B. Reidy, A. Haase, A. Luch, K. Dawson and I. Lynch, Mechanisms of Silver Nanoparticle Release, Transformation and Toxicity: A Critical Review of Current Knowledge and Recommendations for Future Studies and Applications, Materials, 2013, 6(6), 2295–2350 CrossRef CAS PubMed , Available from: http://www.mdpi.com/1996-1944/6/6/2295/.
- A. Fairbrother, R. Wenstel, K. Sappington and W. Wood, Framework for Metals Risk Assessment, Ecotoxicol. Environ. Saf., 2007, 68(2), 145–227 CrossRef CAS PubMed , Available from: http://www.epa.gov/raf/metalsframework/index.htm.
- M. C. P. Mendonça, M. B. de Jesus and C. A. M. van Gestel, Protective effect of N-acetylcysteine on the toxicity of silver nanoparticles: Bioavailability and toxicokinetics in Enchytraeus crypticus, Sci. Total Environ., 2020, 715, 136797, DOI:10.1016/j.scitotenv.2020.136797.
- E. Topuz and C. A. M. van Gestel, Toxicokinetics and toxicodynamics of differently coated silver nanoparticles and silver nitrate in Enchytraeus crypticus upon aqueous exposure in an inert sand medium, Environ. Toxicol. Chem., 2015, 34(12), 2816–2823, DOI:10.1002/etc.3123.
- P. L. Waalewijn-Kool, K. Klein, R. M. Forniés and C. A. M. van Gestel, Bioaccumulation and toxicity of silver nanoparticles and silver nitrate to the soil arthropod Folsomia candida, Ecotoxicology, 2014, 23(9), 1629–1637, DOI:10.1007/s10646-014-1302-y.
- M. Diez-Ortiz, E. Lahive, P. Kille, K. Powell, A. J. Morgan and K. Jurkschat,
et al. Uptake routes and toxicokinetics of silver nanoparticles and silver ions in the earthworm Lumbricus rubellus, Environ. Toxicol. Chem., 2015, 34(10), 2263–2270, DOI:10.1002/etc.3036.
- Z. Khodaparast, C. A. M. van Gestel, A. G. Papadiamantis, S. F. Gonçalves, I. Lynch and S. Loureiro, Toxicokinetics of silver nanoparticles in the mealworm Tenebrio molitor exposed via soil or food, Sci. Total Environ., 2021, 777, 146071, DOI:10.1016/j.scitotenv.2021.146071.
- M. P. Castro-Ferreira, D. Roelofs, C. A. M. van Gestel, R. A. Verweij, A. M. V. M. Soares and M. J. B. Amorim, Enchytraeus crypticus as model species in soil ecotoxicology, Chemosphere, 2012, 87(11), 1222–1227, DOI:10.1002/cbdv.200490137/abstract.
- W. Didden and J. Römbke, Enchytraeids as Indicator Organisms for Chemical Stress in Terrestrial Ecosystems, Ecotoxicol. Environ. Saf., 2001, 50(1), 25–43 CrossRef CAS PubMed , Available from: http://www.ncbi.nlm.nih.gov/pubmed/11534950.
- S. Jänsch, M. J. Amorim and J. Römbke, Identification of the ecological requirements of important terrestrial ecotoxicological test species, Environ. Rev., 2005, 13(2), 51–83, DOI:10.1139/a05-007.
- N. P. Rodrigues, J. J. Scott-Fordsmand and M. J. B. Amorim, Novel understanding of toxicity in a life cycle perspective – The mechanisms that lead to population effect – The case of Ag (nano)materials, Environ. Pollut., 2020, 262, 114277 CrossRef CAS , Available from: https://linkinghub.elsevier.com/retrieve/pii/S0269749119375700.
- M. C. P. Mendonça, N. P. Rodrigues, J. J. Scott-Fordsmand, M. B. Jesus and M. J. B. Amorim, The toxicity of silver nanomaterials (NM 300K) is reduced when combined with N-Acetylcysteine: Hazard assessment on Enchytraeus crypticus, Environ. Pollut., 2020, 256, 113484 CrossRef PubMed , Available from: https://linkinghub.elsevier.com/retrieve/pii/S0269749119350948.
- S. I. L. Gomes, C. P. Roca, J. J. Scott-Fordsmand and M. J. B. Amorim, High-throughput transcriptomics reveals uniquely affected pathways: AgNPs, PVP-coated AgNPs and Ag NM300K case studies, Environ. Sci.: Nano, 2017, 4(4), 929–937 RSC , Available from: http://xlink.rsc.org/?DOI=C6EN00652C.
- V. L. Maria, M. J. Ribeiro, S. Guilherme, A. M. V. M. Soares, J. J. Scott-Fordsmand and M. J. B. Amorim, Silver (nano)materials cause genotoxicity in Enchytraeus crypticus , as determined by the comet assay, Environ. Toxicol. Chem., 2018, 37(1), 184–191, DOI:10.1002/etc.3944.
-
C. L. Klein, S. Comero, B. Stahlmecke, J. Romazanov, T. A. J. Kuhlbusch, E. Van Doren, P.-J. Mast, J. De Temmerman, P. Wick, H. Krug, G. Locoro, K. Hund-Rinke, W. Kördel, S. Friedrichs, G. Maier, J. Werner, Th. Linsinger and B. M. Gawlik, NM-series of Representative Manufactured Nanomaterials. NM-300 Silver. Characterisation, Stability, Homog, Publ Off Eur Union, 2011 Search PubMed.
- OECD. Test No. 317: Bioaccumulation in Terrestrial Oligochaetes [Internet]. Organisation for Economic Co-operation and Development,. Paris, France: OECD; 2010, p. 30, (OECD Guidelines for the Testing of Chemicals, Section 3). Available from: http://www.oecd-ilibrary.org/environment/test-no-317-bioaccumulation-in-terrestrial-oligochaetes_9789264090934-en%5Cnhttp://www.oecd-ilibrary.org/environment/test-no-316-phototransformation-of-chemicals-in-water-direct-photolysis_9789264067585-en.
- OECD. Test No. 202: Daphnia sp. Acute Immobilisation Test [Internet]. OECD Guideline for the Testing og Chemicals, Section 2. OECD; 2004, pp. 1–12, (OECD Guidelines for the Testing of Chemicals, Section 2). Available from: http://www.oecd-ilibrary.org/content/book/9789264069947-en.
- M. M. Ardestani, N. M. van Straalen and C. A. M. van Gestel, Uptake and elimination kinetics of metals in soil invertebrates: A review, Environ. Pollut., 2014, 193, 277–295, DOI:10.1016/j.envpol.2014.06.026.
-
SigmaPlot 14.0. Statistical Package for the Social Sciences - SigmaPlot for Windows 14.0 ed. Systat Software, Chicago, 2017 Search PubMed.
- J. Mariyadas, M. J. B. Amorim, J. Jensen and J. J. Scott-Fordsmand, Earthworm avoidance of silver nanomaterials over time, Environ. Pollut., 2018, 239, 751–756 CrossRef CAS , Available from: https://linkinghub.elsevier.com/retrieve/pii/S0269749118306869.
- F. C. F. Santos, S. I. L. Gomes, J. J. Scott-Fordsmand and M. J. B. Amorim, Hazard assessment of nickel nanoparticles in soil-The use of a full life cycle test with Enchytraeus crypticus, Environ. Toxicol. Chem., 2017, 36(11), 2934–2941, DOI:10.1002/etc.3853.
- W. Peijnenburg, A. Praetorius, J. Scott-Fordsmand and G. Cornelis, Fate assessment of engineered nanoparticles in solids dominated media – Current insights and the way forward, Environ. Pollut., 2016, 218, 1365–1369, DOI:10.1016/j.envpol.2015.11.043.
- S. Klitzke, G. Metreveli, A. Peters, G. E. Schaumann and F. Lang, The fate of silver nanoparticles in soil solution — Sorption of solutes and aggregation, Sci. Total Environ., 2015, 535, 54–60, DOI:10.1016/j.scitotenv.2014.10.108.
- R. C. Bicho, F. C. F. Santos, J. J. Scott-Fordsmand and M. J. B. Amorim, Effects of copper oxide nanomaterials (CuONMs) are life stage dependent – full life cycle in Enchytraeus crypticus, Environ. Pollut., 2017, 224, 117–124 CrossRef CAS PubMed , Available from: https://linkinghub.elsevier.com/retrieve/pii/S0269749116323788.
Footnote |
† Electronic supplementary information (ESI) available. See DOI: 10.1039/d1en00338k |
|
This journal is © The Royal Society of Chemistry 2021 |