Concentrations of halogenated flame retardants and polychlorinated biphenyls in house dust from Lagos, Nigeria†
Received
29th July 2021
, Accepted 27th September 2021
First published on 28th September 2021
Abstract
Polychlorinated biphenyls (PCBs), polybrominated diphenyl ethers (PBDEs), and hexabromocyclododecane (HBCDD) are regulated under the Stockholm Convention of the United Nations' Environment Programme; with similar concerns emerging about alternative halogenated flame retardants (alt-HFRs), the use of which is increasing as replacements for PBDEs and HBCDD. While the presence in indoor dust of PCBs, PBDEs, and HBCDDs has been reported previously in a few African locations including Lagos, Nigeria, we are unaware of similar data for alt-HFRs. The present study thus aimed to provide the first information on alt-HFRs in indoor dust in sub-Saharan Africa, and to evaluate the impact of restrictions on the use of PBDEs, HBCDD, and PCBs on their concentrations in house dust in Lagos, Nigeria. Concentrations of ∑8PBDEs, ∑HBCDDs, ∑7alt-HFRs, and ∑8PCBs in 15 samples of dust from homes in Lagos, Nigeria were found to be: 43–810 (median = 300) ng g−1, <dl – 66 (median = <dl) ng g−1, 32–2600 (median = 320) ng g−1 and 3.8–61 (median = 18) ng g−1 respectively. The dominant PBDE was BDE-209, its replacement decabromodiphenyl ethane (DBDPE) was the predominant alt-HFR, while PCB-138 displayed the highest concentration of the 8 PCBs targeted. Likely due to their higher vapour pressures, concentrations of the non-arochlor PCB 11, as well as those of PCB 28, and PBDE 28 were below detection limits. Concentrations of PBDEs and PCBs reported are generally below those reported previously for Lagos, Nigeria; suggesting restrictions on their manufacture and use have been effective. In contrast, while concentrations of BDE-209 in this study were lower than in one previous study in Lagos, they exceeded those in another; implying that the more recent restrictions on the deca-BDE product have yet to be fully effective. The evidence presented here of concentrations of alt-HFRs in Nigerian house dust provide a valuable benchmark against which future trends in their concentrations may be evaluated.
Environmental significance
Polybrominated diphenyl ethers (PBDEs) and hexabromocyclododecane (HBCDD) found extensive past use as flame retardants, with polychlorinated biphenyls (PCBs) also used widely, leading to substantial environmental contamination and human exposure. Alongside toxicity concerns, this resulted in bans on their manufacture. Despite this, emissions continue from products remaining in use, with new concerns raised about exposure to non-regulated, structurally similar alternative halogenated flame retardants (alt-HFRs). This study augments the sparse database on PBDEs, HBCDD, and PCBs in African house dust and provides the first information on alt-HFRs in indoor dust from sub-Saharan Africa. Encouraging declines in PCBs and some PBDEs indicate a beneficial impact of past restrictions; and we provide a benchmark against which future trends in alt-HFRs may be evaluated.
|
1. Introduction
While polychlorinated biphenyls (PCBs) and brominated flame retardants (BFRs) like polybrominated diphenyl ethers (PBDEs) and hexabromocyclododecane (HBCDD) are not known to have been produced in Africa;1 due to inter alia their use in imported goods, their presence is widely reported in diverse environmental compartments across the African continent.2
Both ‘legacy’ brominated flame retardants (BFRs) like PBDEs and HBCDD, as well as alternative halogenated flame retardants (alt-HFRs) were or are applied principally as additives in a wide range of consumer products including electronics, fabrics, and furniture foams.3–5 As a consequence of concerns over their toxicity, environmental persistence, capacity for bioaccumulation, and potential for long range atmospheric transport; both PBDEs and HBCDD are listed as persistent organic pollutants (POPs) under the Stockholm Convention of the United Nations Environment programme.6
Alt-HFRs encompass a range of chemicals that are potential replacements for the regulated PBDEs and HBCDD. Those targeted in this study are: decabromodiphenyl ethane (DBDPE), dechlorane plus (DP), hexabromobenzene (HBBz), pentabromobenzene (PBBz), pentabromoethylbenzene (PBEB), and pentabromotoluene (PBT).3 These are targeted here due to concerns over their toxicity, persistence and bioaccumulation potential.7,8 For example, DBDPE, a potential BDE 209 replacement, was highlighted as bioaccumulative9 and a potential thyroid hormone disruptor.10 Moreover, the USEPA has highlighted DBDPE to display similar developmental toxicity to that of decaBDE.11 This is of concern as the EPA's reference dose for decaBDE (7 μg per kg per body weight per day) is based on its neurodevelopmental toxicity.12
Up until widespread bans on their manufacture and new use in the late 1970s-early 1980s; the commercial mixtures of PCBs produced under different trade names such as arochlor, were applied in many jurisdictions as dielectric fluids in electrical transformers and capacitors, alongside other applications in: adhesives, paints, building sealants, fire proofing agents, inks, and paints.1,13 Furthermore, recent studies have reported substantial concentrations of congeners such as PCB 11, which are not present in technical commercial mixtures like aroclors. Sources of PCB 11 and other so-called non-aroclor PCBs include diarylide dyes and degradation of higher chlorinated PCB congeners.14 Current knowledge of the toxicity of PCB 11 is limited, but the arochlor PCBs have been regulated since 2001 under the UNEP Stockholm Convention on POPs.6
Ingestion of domestic dust is an established pathway of human exposure to organic contaminants, particularly for toddlers due to their greater hand-to-mouth behaviour compared to adults combined with their low body weight. There are several studies targeting PBDEs,15–19 HBCDDs,17,20 and PCBs15,16,21 in domestic dust from a number of locations in the African continent. However, data on concentrations of alt-HFRs in African domestic dust are scarce. Against this backdrop, this study's objectives were to evaluate temporal trends in concentrations of PBDEs and PCBs in house dust from Lagos, Nigeria by comparing data for samples collected in 2020 with those reported previously for samples collected in 2014,16,20 and to provide baseline data for concentrations of alt-HFRs in the same dust samples.
2. Materials and methods
2.1 Sampling procedures
One convenience sample of indoor dust was collected from each of 15 homes in Lagos, Nigeria, which is one of the most industrialised and urbanised cities in Africa with population of about 24 million. Samples were collected with a hand brush comprising synthetic polyester bristles.22 Dust samples, excluding small stones and heavy materials, were collected from the most-frequented parts of the living room floor, wrapped carefully in hexane-washed aluminium foil, and sealed in a zip lock bag before they were transferred to the laboratory. Samples were then stored at ∼20 °C before shipping to the University of Birmingham for chemical analysis. On receipt at Birmingham, the dust samples were each passed through a hexane – precleaned 500 μm mesh testing sieve (UKGE Limited, UK) prior to extraction and analysis.
2.2 Chemical reagents and standards
All solvents used in this study were of HPLC grade or higher, obtained from Fisher Scientific (Loughborough, UK). Individual stock standard solutions of natives, internal standards, and recovery determination standards (PBDEs – 28, 47, 99, 100, 153, 154, 183, 209, 77, 128, 13C-BDE-100, 13C-BDE-209, α-HBCDD, β-HBCDD, γ-HBCDD, 13C-α-HBCDD, 13C-β-HBCDD, 13C-γ-HBCDD, d18-γ-HBCDD, DBDPE, anti-DP, syn-DP, HBBz, PBBz, PBEB, and PBT) were purchased from Wellington Laboratories (Guelph, ON, Canada). PCBs – 11, 14, 28, 29, 34, 52, 62, 101, 118, 119, 129, 138, 147, 153, 173, and 180 were bought from Greyhound Chromatography (Birkenhead, Merseyside, UK). Pesticide grade florisil (60–100 mesh) and concentrated sulfuric acid, were purchased from Acros Organics (Geel, Belgium) and Sigma-Aldrich (St. Louis, MA, US) respectively.
2.3 Sample extraction and cleanup
Approximately 100 mg (accurately weighed) of dust was spiked with an internal standard mixture comprising: BDE-77, BDE-128, 13C-BDE-209; 13C-α-HBCDD, 13C-β-HBCDD, 13C-γ-HBCDD; PCB-14, PCB-34, PCB-62, PCB-119, PCB-147, and PCB-173. The spiked dust sample was extracted with 5 mL hexane followed by 1 min vortexing, prior to 15 min sonication, followed by 5 min centrifugation at 3500 rpm. This procedure was repeated twice and the supernatants combined in a 100 mL TurboVap® tube (Drage et al., 2020). The crude extract was concentrated to ∼2 mL hexane using a TurboVap® evaporator before acid digestion with ∼2 mL concentrated sulfuric acid overnight.23 After decanting the hexane layer, the cleaned extract was transferred with hexane washings and applied to a 9 mm i.d. chromatographic column containing 1 g florisil topped with 1 cm anhydrous sodium sulphate, pre-washed with 5 mL hexane, before extract elution with 15 mL hexane. The resulting eluate was concentrated to incipient dryness using a TurboVap® before reconstitution in 100 μL toluene containing 20 ng PCB-29 and -129, and 50 ng 13C-BDE-100 and d18-γ-HBCDD as recovery determination (or syringe) standards (RDS).22,23
2.4 Instrumental analysis
Target BFRs were analysed as previously described.23 Briefly, 1 μL of the extract was injected onto a TRACE™ 1310 Gas Chromatograph/ISQ™ single quadrupole mass spectrometer (Thermo Fisher Scientific, Austin, TX, USA) operated in electron ionisation, selected ion monitoring (EI-SIM) mode and fitted with a Restek Rxi-5Sil MS chromatographic column (15 m × 0.25 mm × 0.25 μm film thickness). Analysis of target PCBs was performed on GC Agilent HP6850/5975 MS. One μL of the final sample extract was injected onto a Restek Rxi-5Sil MS column (30 m × 0.25 mm × 0.25 μm film thickness). The carrier gas was pure helium at a flow rate of 1.5 mL min−1 for both BFRs and PCBs, with the column oven temperature programmes and m/z ions monitored provided in Tables S1 and S2a.†
After analysis of BFRs and PCBs was complete, the extract was reconstituted in methanol and HBCDDs measured using a dual pump Shimadzu LC-20AB prominence liquid chromatograph (Shimadzu, Kyoto, Japan) interfaced with a Sciex API 2000 triple quadrupole mass spectrometer (Applied Biosystems, Foster City, CA). Target HBCDD diastereomers were separated on an Agilent Pursuit XRS3 C18 reversed phase analytical column (150 mm × 2 mm i.d., 3 μm particle size). Full details of the ions monitored and the mobile phase elution gradient employed for HBCDD analysis are provided as ESI in Table S2b and Fig. S1.†20,24
2.5 Quality assurance/quality control
All glassware were thoroughly cleaned with tap water, then detergent solution, further with Milli Q water and a solvent mixture of dichloromethane
:
hexane (1
:
1), before finally baked for ∼3 hours at 425 °C before use. Aliquots (n = 10) of the Standard Reference Material NIST SRM 2585 (organics in indoor dust) were analysed to evaluate method accuracy and precision, with the concentrations obtained within acceptable values (Table S3b†). A procedural blank in which the sample was substituted with anhydrous sodium sulfate was analysed for every 5 samples. Only BDE-47 was found in the blank samples at concentrations below 5% of those detected in samples in all cases. Limits of quantifications (LOQs) were 0.19–1.5 ng g−1, 2.4–11 ng g−1, 0.10–54 ng g−1, and 0.19–0.57 ng g−1 for BDEs, HBCDDs, alt-HFRs, and PCBs respectively. Average recoveries of internal standards were 71–110% (BFRs), 75–76% (HBCDD); and 56–89% (PCBs) (Table S4c†).
2.6 Statistical analysis
Box plot and Pearson correlations were performed in Microsoft Excel 365, while one-way ANOVA tests were performed in IBM SPSS Statistics 26. For statistical analysis purposes, non-detect values were replaced by the fractional detection frequency (df) × LOQ. A confidence interval of ≥95% was taken to denote statistical significance.
2.7 Estimation of exposure
Exposures to BDEs, NBFRs, and PCBs via dust ingestion were estimated using the following algorithm (eqn (1)):21,25,26 | Estimated daily intake (EDI) (ng per kg bw per day) = [CH × FH × IR]/body weight | (1) |
where CH is the concentration of target contaminant in dust (ng g−1), F = fractional time spent at home, IR = ingestion rate for dust (mg per day). Average fractional time spent by adults and toddlers at home were assumed to be 0.58 (58%) and 0.92 (92%) respectively, while adult and toddler body weights were assumed to be 60 and 10 kg respectively.27,28 Exposure via house dust ingestion was calculated under eight scenarios: (a) assuming typical dust ingestion rates of 20 mg per day for adults and 50 mg per day for toddlers28 and that ingested dust contained each contaminant at the 5th percentile, arithmetic mean, median, and 95th percentile concentration; and (b) assuming high dust ingestion rates of 50 mg per day for adults and 200 mg per day for toddlers28 and that ingested dust contained each contaminant at the 5th percentile, arithmetic mean, median, and 95th percentile concentration.
3. Results
3.1 Concentrations of the target chemicals in domestic indoor dust from Lagos, Nigeria
3.1.1 Concentrations of PBDEs and HBCDDs in dust from Nigerian homes.
The concentrations of PBDEs and HBCDD measured in indoor dust in this study are summarised in Fig. 1 and Table 1 respectively. Those of ∑8BDEs ranged from 43 ng g−1 to 810 ng g−1 average = 310 ng g−1. As shown in Fig. 1, BDE-209 was the dominant PBDE, comprising on average 87% of ∑8BDEs, with the relative abundance being: BDE-209 (average concentration = 290 ng g−1) > BDE-99 (average concentration = 6.6 ng g−1) > BDE-47 (average concentration = 5.8 ng g−1) > BDE-183 (average concentration = 4.5 ng g−1) > BDE-153 (average concentration = 2.4 ng g−1) > BDE-154 (average concentration = 1.6 ng g−1) > BDE-100 (average concentration = 1.3 ng g−1). BDE-28 was not detected in any sample (<0.78 ng g−1). This dominance of BDE-209 – which has been reported elsewhere16,23,29,30 – suggests decaBDE is the principal PBDE formulation used in the products in the Nigerian homes sampled. Interestingly, while the detection frequency for BDE-209 and BDE-47 was 93% and that for BDE-99, 86%; congeners commonly associated with the octaBDE formulation – i.e. BDE-153, 154, and 183 were detected in all samples (Table S5a†).
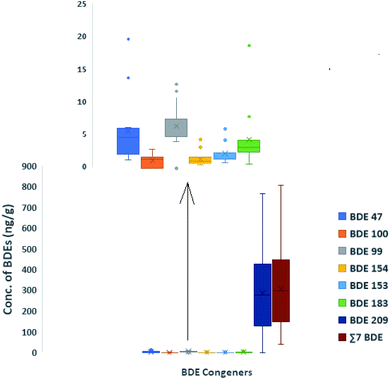 |
| Fig. 1 Concentrations of target BDE congeners in Lagos home settled dust. * – the boxes indicate the 25th and 75th percentiles while the bars and the ‘‘x’’ in the box indicate the median and mean concentration respectively. The whiskers indicate the maximum and minimum concentrations, and the ‘dots’ show the outliers. | |
Table 1 Concentrations of HBCDD in Lagos home dust
|
Concentration (ng g−1) |
α-HBCDD |
β-HBCDD |
γ-HBCDD |
∑HBCDD |
Mean |
5.7 |
<11 |
5.1 |
11 |
Median |
<5.3 |
<11 |
<2.4 |
<dl |
Range |
<5.3–40 |
<11 |
<2.4–19 |
<dl – 66 |
Concentrations of ∑HBCDD range from <2.4 to 66 ng g−1 (mean = 11, median = <2.4) (Table 1), with HBCDD detected in only 4 samples. Although HBCDD has some minor applications in consumer products such as electronics and furniture fabric covers, its predominant application is as an additive to polystyrene building thermal insulation foam.24,31 Hence, the low concentrations of HBCDD detected here are unsurprising given the tropical climate of Lagos. With respect to the diastereomer pattern, γ-HBCDD was observed in four samples, α-HBCDD was observed in two with β-HBCDD not detected in any samples.
3.1.2 Concentration of alt-HFRs in dust from Nigerian homes.
Concentrations of alt-HFRs are presented in Fig. 2 and S7b.† Those of ∑alt-HFRs are in the range 32–2600 ng g−1 (mean = 560, median = 320). DBDPE was the dominant alt-HFR targeted, on average comprising 68% of ∑alt-HFRs (Table S7c†). Average concentrations of the alt-HFRs targeted are: DBDPE (480 ng g−1) > anti-DP (36 ng g−1) >PBEB (27 ng g−1) > syn-DP (9.1 ng g−1) > PBT (0.96 ng g−1) > HBBz (0.93 ng g−1) > PBBz (0.1 ng g−1). PBBz was only found only in one sample while syn-DP and anti-DP display the highest detection frequencies of 93% and 87% respectively. The target alt-HFRs are all classified by the USEPA as low production volume chemicals, with the exceptions of DBDPE and DP which are classified as high production.3,32 This is consistent with the relative abundance in this study of both DBDPE and anti-DP. The fractional contribution of anti-DP to ∑DP (fanti-DP) fell in the range 0.26–0.96 (mean = 0.76, median = 0.79). Similar wide variations have been documented previously.33
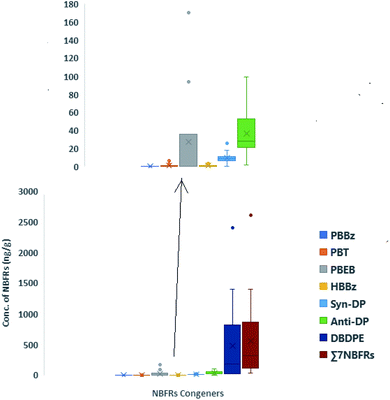 |
| Fig. 2 Concentrations of target alt-HFRs in Lagos home settled dust. * – the boxes indicate the 25th and 75th percentiles while the bars and the ‘‘x’’ in the box indicate the median and mean concentration respectively. The whiskers indicate the maximum and minimum concentrations, and the ‘dots’ show the outliers. | |
3.1.3 Concentration of PCBs in dust from Nigerian homes.
Concentrations of target PCBs detected in indoor dust in this study are summarised in Fig. 3 and S8b,† with those of ∑7PCBs ranging from 3.8 ng g−1 to 61 ng g−1 (mean = 22 ng g−1, median = 18) ng g−1. Likely reflecting their comparatively high vapour pressures, neither PCB-28 or the non-arochlor PCB-11 were detected in any sample. Of the remaining target congeners, only PCBs 138 and 180 were detected in all samples, while average concentrations were in the order: PCB-138 (9.0 ng g−1) > PCB-180 (4.5 ng g−1) > PCB-153 (3.7 ng g−1) > PCB-52 (2.8 ng g−1) > PCB-118 (1.0 ng g−1) > PCB-101 (0.55 ng g−1).
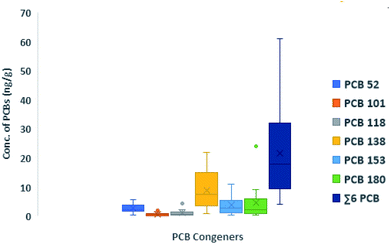 |
| Fig. 3 Concentrations of target PCB congeners in Lagos home settled dust. * – the boxes indicate the 25th and 75th percentiles while the bars and the ‘‘x’’ in the box indicate the median and mean concentration respectively. The whiskers indicate the maximum and minimum concentrations, and the ‘dots’ show the outliers. | |
3.2 Comparison of concentrations of target contaminants in dust from Nigerian homes with similar reports elsewhere
3.2.1 PBDEs and HBCDD.
Table 2 compares the concentrations of PBDEs and HBCDD detected in this study with those from selected other studies in home dust. Concentrations in our study were lower than those reported previously for Nigeria,16,19,20 with the exception that concentrations of BDE-209 in this study exceeded those reported.19 This may suggest that restrictions on the manufacture and use of the penta- and octa-BDE formulations have lowered concentrations of associated PBDE congeners like BDE-47, BDE-99, BDE-153, BDE-154, and BDE-183. However, concentrations of BDE-209 have declined from the levels reported in Harrad et al.,16 but not compared to those reported by Olukunle et al.19 This is consistent with the more recent restrictions on the manufacture and use of the deca-BDE commercial product. In general, comparing with data obtained further afield in other locations in Africa and elsewhere, concentrations in our study are very much at the low end of those reported. Overall, the available data suggest that PBDE and HBCDD use in Nigerian homes is lower than in many other countries.
Table 2 Comparison of concentrations of PBDEs and HBCDDs in domestic indoor dust from this study with those reported elsewherea
Location |
Sampling year(s) (sample number) |
Concentration (ng g−1) – range (mean, median) |
BDE-28 |
BDE-47 |
BDE-99 |
BDE-100 |
BDE-153 |
BDE-154 |
BDE-183 |
BDE-209 |
∑8PBDEs |
∑HBCDD |
dl – detection limit, # – median, * –mean.
|
Lagos, Nigeria (this study) |
2019 (n = 15) |
<0.79 |
<1.5–20 (5.8, 4.8) |
<0.19–13 (6.6, 6.9) |
<0.21–3.1 (1.3, 1.5) |
1–6.2 (2.4, 1.9) |
0.64–4.6 (1.6, 1.2) |
0.71–19 (4.5, 3.4) |
<0.16–770 (290, 280) |
43–810 (310, 300) |
<2.4–66 (11, <2.4) |
Lagos, Nigeria16 |
2014 (n = 12) |
<0.04–3.1 (1, 0.5) |
2.2–50 (13, 8) |
1.5–31 (17, 14) |
<0.03–44 (8, 4) |
<0.04–22 (5, 4) |
<0.04–3500 (450, 4) |
2.9–90 (26, 18) |
77–940 (420, 390) |
— |
— |
Lagos, Nigeria20 |
2014 (n = 10) |
— |
— |
— |
— |
— |
— |
— |
— |
— |
41–1900 (659, 394) |
Makurdi, Nigeria19 |
2012 (n = 10) |
— |
26–51 (43, 47) |
0.24–62 (99, 46) |
0.1–56 (39, 43) |
0.03–74 (39, 50) |
0.03–72 (35, 35) |
38.8–85 (62, 30) |
79–202 (141, 139) |
#45 |
— |
Cairo, Egypt17 |
2013 (n = 17) |
0.15–4 (0.7, 0.3) |
0.34–380 (29, 1.7) |
0.53–510 (35, 2.7) |
0.07–98 (7.2, 0.4) |
0.54–200 (28, 6.3) |
<0.04–7.9 (4.9, 0.4) |
0.27–5.2 (1.7, 1.1) |
2.2–590 (130, 40) |
5.0–1900 (250, 57) |
(21, 6.2) |
Pretoria, South Africa18 |
2010–2011 (n = 31) |
— |
#2.6 |
2.6 |
<0.13 |
<0.13 |
<0.13 |
— |
<1.8 |
— |
— |
Durban, South Africa15 |
2012 (n = 10) |
1.1–30 (15, 12) |
56–470 (180, 160) |
150–1100 (540, 510) |
<dl – 150 (47, 37) |
34–180 (78, 65) |
<dl – 130 (76, 97) |
5.6–110 (44, 45) |
59–2200 (730, 660) |
690–3900 (1700, 1600) |
— |
Birmingham, UK23 |
2020 (n = 14) |
<0.1 |
<0.1–37 (6, 0.93) |
<0.1–61 (11, 2.9) |
<0.1–6.9 (1.5, 0.29) |
<2.0 |
<2.0 |
<2.0 |
21–18 000 (4800, 1600) |
21–18 000 (4800, 1700) |
76–570 000 (460 000, 280) |
Annecy, France20 |
2008 (n = 9) |
— |
— |
— |
— |
— |
— |
— |
— |
— |
360–1900 (1200, 1400) |
Australia34 |
2009–2011 (n = 30) |
(<dl – 10) 0.7 |
(2.6–390) 37 |
(2.9–170) 57 |
(0.52–71) 9.1 |
(<dl – 60) 6.4 |
(<dl – 32) 5.1 |
(0.53–44) 4.6 |
(<dl – 82 000) *420 |
— |
— |
Ireland30 |
2016–2017 (n = 26) |
— |
0.6–240 (26, 8) |
<0.2–500 (45, 13) |
— |
— |
— |
<0.3–33 (4, 1) |
140–650 000 (58 000, 1300) |
— |
1.3–430 000 (2900, 490) |
Stockholm, Sweden35 |
2006 (n = 10) |
<0.1–5.6 (#1.3) |
<0.5–230 (42) |
<1–140 (52) |
— |
0.61–23 (6.6) |
— |
<0.7–49 (12) |
53–4000 (510) |
— |
15–990 (100) |
Beijing, China13 |
2014 (n = 30) |
0.34–3.8 (1.1, 0.68) |
0.67–4.2 (1.6, 1.3) |
1.2–25 (6.3, 4.9) |
<dl − 1.9 (0.37, 0.44) |
0.27–3.5 (1.4, 0.89) |
0.25–1.6 (0.70, 0.53) |
0.21–3.7 (0.84, 0.37) |
69–410 (190, 150) |
90–430 (200, 160) |
110–390 (253, 258) |
Toronto, Canada29 |
2006 (n = 10) |
1.4–20 (6.6, 4.1) |
47–20 (300, 140) |
80–1800 (510, 330) |
14–420 (120, 65) |
9.4–260 (71, 43) |
6.2–280 (69, 39) |
7–30 (13, 9) |
290–1100 (670, 560) |
160–3600 (1100, 620) |
— |
3.2.2 Alt-HFRs.
To our knowledge, this is the first report of concentrations of alt-HFRs in indoor dust in sub-Saharan Africa. We nonetheless compare our data for these contaminants with those reported in other locations (Table 3). In keeping with studies elsewhere, DBDPE is the dominant alt-BFR in our study, and in general, concentrations of our other target alt-HFRs fall within the range reported previously in other locations.
Table 3 Comparison of concentrations of alt-HFRs in domestic indoor dust from this study with those reported elsewherea
Location |
Sampling period (sample number) |
Concentrations (ng g−1) – range (mean, median) |
PBBz |
PBT |
PBEB |
HBBz |
syn-DP |
anti-DP |
DBDPE |
dl – detection limit, * – median, ** – mean.
|
Lagos, Nigeria (this study) |
2019 (n = 15) |
<0.7–0.89 (0.10, <0.7) |
<0.59–6.3 (0.96, <0.59) |
<0.71–170 (27, <0.71) |
<0.76–3.5 (0.93, <0.76) |
<0.1–26 (9.1, 8.7) |
<2.4–99 (36, 28) |
<54–2400 (480, 180) |
Birmingham, UK23 |
2020 (n = 14) |
— |
— |
— |
— |
— |
— |
<50–7500 (1500, 660) |
Ireland30 |
2016–2017 (n = 29) |
— |
— |
— |
— |
— |
— |
410–460 000 (39 000, 4200) |
Melbourne, Australia26 |
2016 (n = 24) |
— |
<dl – 8.0 |
<dl |
<dl – 4.6 (1.0, 0.71) |
— |
— |
<dl – 9000 (2000, 1600) |
Birmingham, UK36 |
2013–2015 (n = 30) |
— |
<0.01–90 (7.1, 1.8) |
<0.01–21 (2.3, 0.78) |
— |
— |
— |
<1.2–2300 (240, 41) |
Alexandria, Egypt37 |
2014 (n = 12) |
<dl – 0.20 (0.10, 0.08) |
<dl–0.08 (<dl, <dl) |
<dl – 0.10 (<dl, <dl) |
<dl – 0.70 (0.30, 0.29) |
— |
— |
— |
Beijing, China13 |
2014 (n = 30) |
— |
<dl – 4.9 (1.1, 0.72) |
<dl – 2.1 (0.53, 0.20) |
0.10–5.4 (2.0, 1.4) |
— |
— |
220–3000 (770, 560) |
Basrah, Irag38 |
2013 (n = 18) |
— |
— |
<0.1–0.55 (0.14, <0.1) |
— |
— |
— |
33–270 (130, 130) |
Oslo, Norway39 |
2013–2014 (n = 60) |
— |
<5.2–250 |
<0.37–12 |
<0.21–110 |
— |
— |
11–430 |
Cairo, Egypt17 |
2013 (n = 17) |
— |
— |
— |
0.1* |
0.3* |
0.01* |
— |
Kuwait21 |
2011 (n = 15) |
— |
— |
— |
0.2–3.6 (1.4, 1.2) |
— |
— |
40–2200 (510, 220) |
Antwerp, Belgium21 |
2008 (n = 39) |
— |
— |
— |
— |
— |
— |
55–2100 (300, 150) |
Hanoi, Vietnamese41 |
2008 (n = 6) |
— |
— |
— |
— |
— |
— |
17–150 (median = 40) |
Vancouver, Canada33 |
2007–2008 (n = 116) |
— |
— |
<0.1–4.1 (**0.5) |
<0.02–130 (6.8, 3.7) |
<0.70–170 (11, 0.9) |
<0.70–170 (11, 4) |
— |
3.2.3 PCBs.
Concentrations detected in this study together with those from selected similar studies are summarised in Table 4. Concentrations of individual PCBs are a little lower but within the range reported previously for homes in Lagos, Nigeria.16 In general, concentrations in this study are at the lower end of those reported previously elsewhere, possibly reflecting a general temporal decline in PCB contamination as samples reported previously were collected 6–13 years before those in this study. Interestingly, concentrations of PCB 28, 153, and 180 detected in 10 house dust samples collected in 2012 in Durban, South Africa15 exceed substantially (by up to two orders of magnitude) those found in Lagos in our study. Notwithstanding likely temporal declines in PCB contamination, this suggests lower PCB use in Nigeria than South Africa.
Table 4 Comparison of concentrations of PCBs in domestic indoor dust from this study with those reported elsewherea
Location |
Sampling year (sample number) |
Concentration (ng g−1) – range (mean, median) |
PCB-28 |
PCB-52 |
PCB-101 |
PCB 118 |
PCB-138 |
PCB-153 |
PCB-180 |
∑7PCBs |
dl – detection limit, * PCBs – 28 + 31, ** – median.
|
Lagos, Nigeria (this study) |
2019 (n = 15) |
<0.31 |
<0.49–7.6 (2.8, 2.1) |
<0.32–1.9 (0.55, 0.51) |
0.59–4.2 (1.0, 0.62) |
0.82–22 (9.0, 7.5) |
<0.46–11 (3.7, 2.8) |
0.27–24 (4.5, 2.2) |
3.8–61 (22, 18) |
Lagos, Nigeria16 |
2014 (n = 12) |
1.5–7.8 (3.8, 3.9) |
0.8–8.6 (4.2, 4.5) |
0.4–9.4 (2.7, 3.7) |
— |
0.3–22 (6.0, 7.5) |
0.2–22 (5.8, 1.3) |
4.4–24 (10, 10) |
— |
Durban, South Africa15 |
2012 (n = 10) |
16, 11 |
— |
— |
— |
— |
173, 150 |
702, 585 |
— |
Kuwait21 |
2011 (n = 15) |
— |
— |
<0.2–835 (63, <0.2) |
<0.2–560 (35, <0.2) |
<0.1–435 (28, <0.1) |
<0.2–310 (20, <0.2) |
<0.1–105 (7, 0.4) |
— |
Faisalabad, Pakistan21 |
2011 (n = 15) |
— |
— |
<0.2 |
<0.2–0.8 (<0.2, 0.2) |
<0.1–2.2 (0.5, <0.1) |
<0.1–4.0 (0.8, 0.4) |
<0.1–11 (1.2, 0.3) |
— |
Texas, US28 |
2006 (n = 20) |
*1.6–37 (9.5, 5.1) |
1.7–28 (7.9, 6.2) |
1.9–29 (10, 8.7) |
1,8–44 (10, 5.5) |
1.1–31 (8.6, 6.5) |
1–22 (8.4, 7.1) |
0.7–20 (4.5, 2.6) |
— |
Birmingham, UK28 |
2006 (n = 20) |
*0.5–39 (6.3, 3.9) |
0.3–53 (5.6, 1.8) |
0.1–73 (6.1, 1.2) |
0.06–56 (4.3, 0.92) |
0.1–50 (4.1, 1.1) |
0.1–32 (3.3, 1.2) |
0.1–8.1 (1.8, 0.89) |
— |
Toronto, Canada28 |
2006 (n = 10) |
*3.5–29 (10, 7.3) |
3.4–37 (12, 7.2) |
1.9–60 (15, 8.8) |
1.1–55 (13, 8.7) |
1.9–60 (15, 8.8) |
1–49 (12, 9.5) |
0.9–36 (11, 9.9) |
— |
Wellington, New Zealand28 |
2006 (n = 20) |
*0.8–11 (3.3, 2.3) |
0.4–13 (2.6, 1.4) |
0.4–21 (3.1, 1.6) |
<dl – 14 (1.9, 0.95) |
0.4–21 (3.1, 1.6) |
0.3–11 (2.8, 1.8) |
0.4–12 (2.7, 1.4) |
— |
Hanoi, Vietnamese41 |
2008 (n = 6) |
0.18–0.59 (**0.49) |
0.17–2.7 (0.40) |
0.35–3.3 (0.93) |
0.38–8.4 (0.64) |
0.09–1.2 (0.36) |
0.62–18 (1.2) |
0.18–2.3 (0.31) |
— |
Kuwait40 |
n = 15 (2011) |
— |
— |
<0.2–840 (63, <0.2) |
<0.2–560 (35, <0.2) |
<0.2–310 (20, <0.2) |
<0.1–440 (28, <0.1) |
0.1–110 (7, 0.4) |
— |
Canada42 |
n = 16 (2013) |
— |
— |
— |
— |
— |
— |
— |
<dl – 521 (69.1, <dl) |
Czech Republic42 |
n = 28 (2013) |
— |
— |
— |
— |
— |
— |
— |
11–360 (79, 75) |
3.3 Implication of exposure risks of BFRs and PCBs in Lagos home settled dust
Estimated exposures to our target compounds via ingestion of indoor dust were calculated for a range of scenarios as outlined in Section 2.6. The resulting estimates for toddlers and adults are summarised in Tables 5 and 6 respectively. In general, the order of exposures for both age groups is: ∑alt-HFRs > ∑PBDEs > ∑6PCBs > HBCDD. DBDPE and BDE-209 dominate estimated exposures for ∑alt-HBFRs and ∑PBDEs respectively.
Table 5 Estimated exposure of toddlers to BFRs and PCBs (ng per kg bw per day)a
Compounds |
Toddlers |
Average dust ingestion |
High dust ingestion |
5th |
Mean |
Median |
95th |
5th |
Mean |
Median |
95th |
∑alt-HFRs = DBDPE + anti-DP + syn-DP + PBEB + PBT + HBBz + PBBz, ∑BDEs = BDE 47 + BDE 99 + BDE 100 + BDE 153 + BDE 154 + BDE 183 + BDE 209, ∑PCBs = PCB 52 + PCB 101 + PCB 118 + PCB 138 + PCB 153 + PCB 180.
|
BDE-209 |
0.22 |
1.3 |
1.3 |
2.8 |
0.87 |
5.3 |
5.0 |
11 |
DBDPE |
0.13 |
2.2 |
0.82 |
7.74 |
0.53 |
8.8 |
3.2 |
31 |
∑HBCDD |
<0.0006 |
0.0052 |
<0.0006 |
0.028 |
0.024 |
0.20 |
0.024 |
1.0 |
∑alt-HFRs |
0.15 |
2.5 |
1.4 |
8.1 |
0.61 |
10 |
5.8 |
32 |
∑PCBs |
0.024 |
0.098 |
0.083 |
0.25 |
0.097 |
0.38 |
0.33 |
0.98 |
∑PBDEs |
0.36 |
1.4 |
1.3 |
2.9 |
1.4 |
5.7 |
5.4 |
11 |
Table 6 Estimated exposure of adults to BFRs and PCBs (ng per kg bw per day)a
Compounds |
Adults |
Average dust ingestion |
High dust ingestion |
5th |
Mean |
Median |
95th |
5th |
Mean |
Median |
95th |
∑alt-HFRs = DBDPE + anti-DP + syn-DP + PBEB + PBT + HBBz + PBBz, ∑BDEs = BDE 47 + BDE 99 + BDE 100 + BDE 153 + BDE 154 + BDE 183 + BDE 209, ∑PCBs = PCB 52 + PCB 101 + PCB 118 + PCB 138 + PCB 153 + PCB 180.
|
BDE-209 |
0.0093 |
0.056 |
0.053 |
0.12 |
0.023 |
0.14 |
0.13 |
0.29 |
DBDPE |
0.0056 |
0.093 |
0.035 |
0.33 |
0.014 |
0.23 |
0.087 |
0.82 |
∑HBCDD |
<0.0003 |
0.0021 |
<0.0003 |
0.011 |
<0.0006 |
0.0052 |
<0.0006 |
0.028 |
∑alt-HFRs |
0.0065 |
0.11 |
0.061 |
0.34 |
0.016 |
0.27 |
0.15 |
0.86 |
∑PCBs |
0.001 |
0.004 |
0.004 |
0.01 |
0.003 |
0.01 |
0.009 |
0.026 |
∑PBDEs |
0.015 |
0.060 |
0.057 |
0.12 |
0.038 |
0.15 |
0.14 |
0.30 |
The USEPA43 have published reference dose (RfD) values for: BDE 209 (7000 ng per kg per day), BDE 47 (100 ng per kg per day), and BDE 99 (100 ng per kg per day). These RfD values substantially exceeded the exposure levels observed in this study.
4. Conclusion
This study reports concentrations of BFRs and PCBs in floor dust samples from homes in Lagos, Nigeria. The general order of concentrations of our target chemicals was: ∑7alt-HFRs > ∑7BDEs > ∑6PCBs > HBCDD. DBDPE and BDE 209 were the dominant alt-HFR and PBDE respectively. The concentrations of PBDEs and PCBs reported are below those reported previously for house dust from Lagos, Nigeria, suggesting that restrictions on the manufacture and use of such chemicals have had beneficial effects. However, while concentrations of BDE-209 in this study were lower than in one previous study in Lagos, they exceeded those in another; this suggests that the more recent restrictions on the deca-BDE product have yet to lead to a consistent reduction in environmental concentrations of BDE-209. To our knowledge, we report the first evidence of the presence of alt-HFRs in house dust from Africa; these data will provide a valuable benchmark against which possible future trends in concentrations of these replacements for banned BFRs may be evaluated.
Funding sources
Olumide Emmanuel Akinrinade is a Commonwealth Scholar, funded by the UK government via a Commonwealth Scholarship split-site scholarship (NGCN-2019-46).
Author contributions
The manuscript was written through the contributions of all authors. All authors have given approval to the final version of the manuscript. OEA and WS designed and performed the experiments, drafted, and revised the manuscript. SH designed the experiments, drafted, revised, and edited the manuscript. MAA drafted, revised, and edited the manuscript. OA and RA revised, and edited the manuscript.
Conflicts of interest
The authors declare no conflicts of interest.
References
- K. Breivik, J. M. Armitage, F. Wania, A. J. Sweetman and K. C. Jones, Tracking the Global Distribution of Persistent Organic Pollutants Accounting for E-Waste Exports to Developing Regions, Environ. Sci. Technol., 2016, 50, 798–805, DOI:10.1021/acs.est.5b04226.
- O. E. Akinrinade, W. Stubbings, M. A. Abdallah, O. Ayejuyo, R. Alani and S. Harrad, Status of brominated flame retardants, polychlorinated biphenyls, and polycyclic aromatic hydrocarbons in air and indoor dust in AFRICA: a review, Emerging Contam., 2020, 6, 405–420, DOI:10.1016/j.emcon.2020.11.005.
- A. Covaci, S. Harrad, M. A.-E. Abdallah, N. Ali, R. J. Law, D. Herzke and C. A. de Wit, Novel brominated flame retardants: a review of their analysis, environmental fate and behaviour, Environ. Int., 2011, 37(2), 532–556, DOI:10.1016/j.envint.2010.11.007.
- O. T. Fatunsin, T. O. Oluseyi, D. Drage, M. A. Abdallah, A. Turner and S. Harrad, Children's exposure to hazardous brominated flame retardants in plastic toys, Sci. Total Environ., 2020, 720, 137623, DOI:10.1016/j.scitotenv.2020.137623.
- E. A. R. Zuiderveen, J. C. Slootweg and J. de Boer, Novel brominated flame retardants – a review of their occurrence in indoor air, dust, consumer goods and food, Chemosphere, 2020, 255, 126816, DOI:10.1016/j.chemosphere.2020.126816.
-
United Nation Environment Programme (UNEP), Stockholm Convention on POPs, 2020, available online: http//:chm.pops.int/Theconvention/ThePOPs/AllPOPs/tabid/2509/Default.aspx Search PubMed.
- P. Gramatica, S. Cassani and A. Sangion, Are some “safer alternatives” hazardous as PBTs? The case study of new flame retardants, J. Hazard. Mater., 2016, 306, 237–246, DOI:10.1016/j.jhazmat.2015.12.017.
- E. Sverko, G. T. Tomy, E. J. Reiner, Y.-F. Li, B. E. McCarry, J. A. Arnot, R. J. Raw and R. A. Hites, Dechlorane Plus and Related Compounds in the Environment: A Review, Environ. Sci. Technol., 2011, 45(12), 5088–5098, DOI:10.1021/es2003028.
- X. B. Zheng, X. J. Luo, Y. H. Zeng, J. P. Wu, S. J. Chen and B. X. Mai, Halogenated flame retardants during egg formation and chicken embryo development: maternal transfer, possible biotransformation, and tissue distribution, Environ. Toxicol. Chem., 2014, 33(8), 1712–1719, DOI:10.1002/etc.2588.
- T. Chen, D. Yu, L. Yang, S. Sui, S. Lv, Y. Bai, W. Sun, Y. Wang, L. Chen, Z. Sun, L. Tian, S. Tian, D. Wang, P. Niu and Z. Shi, Thyroid function and decabromodiphenyl ethane (DBDPE) exposure in Chinese adults from a DBDPE manufacturing area, Environ. Int., 2019, 133, 105179, DOI:10.1016/j.envint.2019.105179.
-
United States Environmental Protection Agency (US EPA), An alternatives assessment for the flame retardants decabromodiphenyl ether (DecaBDE) – Final report, 2014, available online, https://www.epa.gov/sites/default/files/2014-05/documents/decabde-final.pdf Search PubMed.
-
United States Environmental Protection Agency (US EPA), Toxicological Review of Decabromodiphenyl ether (BDE – 209), EPA/635/R-07/008F, 2008, in support of summary information on the Integrated Risk Information System (IRIS), available online: https://iris.epa.gov/static/pdfs/0035tr.pdf Search PubMed.
- J. Wang, Y. Wang, Z. Shi, X. Zhou and Z. Sun, Legacy and novel brominated flame retardants in indoor dust from Beijing, China: Occurrence, human exposure assessment and evidence for PBDEs replacement, Sci. Total Environ., 2018, 618, 48–59, DOI:10.1016/j.scitotenv.2017.11.049.
- H. Q. Anh, I. Watanabe, T. B. Minh and S. Takahashi, Unintentionally produced polychlorinated biphenyls in pigments: an updated review on their formation, emission sources, contamination status, and toxic effects, Sci. Total Environ., 2021, 755, 142504, DOI:10.1016/j.scitotenv.2020.142504.
- O. A. Abafe and B. S. Martincigh, Polybrominated diphenyl ethers and polychlorinated biphenyls in indoor dust in Durban, South Africa, Indoor Air, 2015, 25(5), 547–556, DOI:10.1111/ina.12168.
- S. Harrad, M. A.-E. Abdallah and T. Oluseyi, Polybrominated diphenyl ethers and polychlorinated biphenyls in dust from cars, homes, and offices in Lagos, Nigeria, Chemosphere, 2016, 146, 346–353, DOI:10.1016/j.chemosphere.2015.12.045.
- Y. Hassan and T. Shoeib, Levels of polybrominated diphenyl ethers and novel flame retardants in microenvironment dust from Egypt: an assessment of human exposure, Sci. Total Environ., 2015, 505, 47–55, DOI:10.1016/j.scitotenv.2014.09.080.
- K. K. Kefeni, J. O. Okonkwo and B. M. Botha, Concentrations of polybromobiphenyls and polybromodiphenyl ethers in home dust: relevance to socio-economic status and human exposure rate, Sci. Total Environ., 2014, 470–471, 1250–1256, DOI:10.1016/j.scitotenv.2013.10.078.
- O. I. Olukunle, O. J. Okonkwo, R. Sha'ato and G. A. Wase, Levels of polybrominated diphenyl ethers in indoor dust and human exposure estimates from Makurdi, Nigeria, Ecotoxicol. Environ. Saf., 2015, 120, 394–399, DOI:10.1016/j.ecoenv.2015.06.023.
- M. A.-E. Abdallah, M. Bressi, T. Oluseyi and S. Harrad, Hexabromocyclododecane and tetrabromobisphenol, 2016 tetrabromobisphenol-A in indoor dust from France, Kazakhstan and Nigeria: Implications for human exposure, Emerging Contam., 2016, 2(2), 73–79, DOI:10.1016/j.emcon.2016.03.006.
- N. Ali, L. Ali, T. Mehdi, A. C. Dirtu, F. Al-Shammari, H. Neels and A. Covaci, Levels and profiles of organochlorines and flame retardants in car and house dust from Kuwait and Pakistan: implication for human exposure via dust ingestion, Environ. Int., 2013, 55, 62–70, DOI:10.1016/j.envint.2013.02.001.
- B. T. Folarin, M. A.-E. Abdallah, T. Oluseyi, K. Olayinka and S. Harrad, Concentrations of polychlorinated biphenyls in soil and indoor dust associated with electricity generation facilities in Lagos, Nigeria, Chemosphere, 2018, 207, 620–625, DOI:10.1016/j.chemosphere.2018.05.110.
- D. S. Drage, S. Waiyarat, S. Harrad, M. A. Abdallah and S. K. Boontanon, Temporal trends in concentrations of legacy and novel brominated flame retardants in house dust from Birmingham in the United Kingdom, Emerging Contam., 2020, 6, 323–329, DOI:10.1016/j.emcon.2020.08.003.
- W. A. Stubbings and S. Harrad, Laboratory studies on leaching of HBCDD from building insulation foams, Emerging Contam., 2019, 5, 36–44, DOI:10.1016/j.emcon.2019.02.003.
- S. Harrad, R. Wijesekera, S. Hunter, C. Halliwell and R. Baker, Preliminary Assessment of U.K. Human Dietary and Inhalation Exposure to Polybrominated Diphenyl Ethers, Environ. Sci. Technol., 2004, 38(8), 2345–2350, DOI:10.1021/es0301121.
- T. J. McGrath, P. D. Morrison, A. S. Ball and B. O. Clarke, Concentrations of legacy and novel brominated flame retardants in indoor dust in Melbourne, Australia: An assessment of human exposure, Environ. Int., 2018, 55113, 191–201, DOI:10.1016/j.envint.2018.01.026.
- S. C. Walpole, D. Prieto-Merino, P. Edwards, J. Cleland, G. Stevens and I. Roberts, The weight of nations, 2012 weight of nations: an estimation of adult human biomass, BMC Public Health, 2012, 12(1), 439, DOI:10.1186/1471-2458-12-439.
- S. Harrad, C. Ibarra, M. Robson, L. Melymuk, X. Zhang, M. Diamond and J. Douwes, Polychlorinated biphenyls in domestic dust from Canada, New Zealand, United Kingdom United States, 2009 and United States: Implications for human exposure, Chemosphere, 2009, 76(2), 232–238, DOI:10.1016/j.chemosphere.2009.03.020.
- S. Harrad, C. Ibarra, M. Diamond, L. Melymuk, M. Robson, J. Douwes, L. Roosens, A. C. Dirtu and A. Covaci, Polybrominated diphenyl ethers in domestic indoor dust from Canada, New Zealand, United Kingdom and United States, Environ. Int., 2008, 34(2), 232–238, DOI:10.1016/j.envint.2007.08.008.
- N. Wemken, D. S. Drage, M. A.-E. Abdallah, S. Harrad and M. A. Coggins, Concentrations of Brominated Flame Retardants in Indoor Air and Dust from Ireland Reveal Elevated Exposure to Decabromodiphenyl Ethane, Environ. Sci. Technol., 2019, 53(16), 9826–9836, DOI:10.1021/acs.est.9b02059.
- J. Desborough, T. Evans, J. Müller and S. Harrad, Polychlorinated biphenyls (PCBs), hexabromocyclododecanes (HBCDDs) and degradation products in topsoil from Australia and the United Kingdom, Emerging Contam., 2016, 2(1), 37–41, DOI:10.1016/j.emcon.2016.03.003.
- J. K. Schuster, T. Harner and E. Sverko, Dechlorane Plus in the Global Atmosphere, Environ. Sci. Technol. Lett., 2020, 8(1), 39–45, DOI:10.1021/acs.estlett.0c00758.
- M. Shoeib, T. Harner, G. M. Webster, E. Sverko and Y. Cheng, Legacy and current-use flame retardants in house dust from Vancouver, Canada, Environ. Pollut., 2012, 169, 175–182, DOI:10.1016/j.envpol.2012.01.043.
- A. Stasinska, A. Reid, A. Hinwood, G. Stevenson, A. Callan, J. Ø. Odland and J. Heyworth, Concentrations of polybrominated diphenyl ethers (PBDEs) in residential dust samples from Western Australia, Chemosphere, 2013, 91(2), 187–193, DOI:10.1016/j.chemosphere.2012.12.044.
- K. Thuresson, J. A. Bjorklund and C. A. de Wilt, Tri-decabrominated diphenyl ethers and hexabromocyclododecane in indoor air and dust from Stockholm microenvironment 1: Level and profiles, Sci. Total Environ., 2012, 414, 713–721, DOI:10.1016/j.scitotenv.2011.11.016.
- F. Tao, M. A.-E. Abdallah and S. Harrad, Emerging and Legacy Flame Retardants in UK Indoor Air and Dust: Evidence for Replacement of PBDEs by Emerging Flame Retardants?, Environ. Sci. Technol., 2016, 50(23), 13052–13061, DOI:10.10.1021/acs.est.6b02816.
- M. A. Khairy and R. Lohmann, Selected organohalogenated flame retardants in Egyptian indoor and outdoor environments: levels, sources and implications for human exposure, Sci. Total Environ., 2018, 633, 1536–1548, DOI:10.1016/j.scitotenv.2018.03.243.
- L. S. Al-Omran and S. Harrad, Polybrominated diphenyl ethers and “novel” brominated flame retardants in floor and elevated surface house dust from Iraq: implications for human exposure assessment, Emerging Contam., 2016, 2(1), 7–13, DOI:10.1016/j.emcon.2015.10.001.
- J. H. Tay, U. Sellström, E. Papadopoulou, J. A. Padilla-Sánchez, L. S. Haug and C. A. de Wit, Human Exposure to Legacy and Emerging Halogenated Flame Retardants via Inhalation and Dust Ingestion in a Norwegian Cohort, Environ. Sci. Technol., 2017, 51(14), 8176–8184, DOI:10.1021/acs.est.7b02114.
- N. Ali, S. Harrad, E. Goosey, H. Neels and A. Covaci, “Novel” brominated flame retardants in Belgian and UK indoor dust: Implications for human exposure, Chemosphere, 2011, 83(10), 1360–1365, DOI:10.1016/j.chemosphere.2011.02.078.
- N. M. Tue, S. Takahashi, G. Suzuki, T. Isobe, P. H. Viet, Y. Kobara, N. Seike, G. Zhang, A. Sudaryanto and S. Tanabe, Contamination of indoor dust and air by polychlorinated biphenyls and brominated flame retardants and relevance of non-dietary exposure in Vietnamese informal e-waste recycling sites, Environ. Int., 2013, 51, 160–167, DOI:10.1016/j.envint.2012.11.006.
- O. Audy, L. Melymuk, M. Venier, S. Vojta, J. Becanova, K. Romanak, M. Vykoukalova, R. Prokes, P. Kukucka, M. L. Diamond and J. Klanova, PCBs and organochlorine pesticides in indoor environments – a comparison of indoor contamination in Canada and Czech Republic, Chemosphere, 2018, 206, 622–631, DOI:10.1016/j.chemosphere.2018.05.016.
-
United States Environmental Protection Agency (US EPA), Technical Fact Sheet – Polybrominated Diphenyl Ethers (PBDEs), EPA 505-F-17-015, United States Office of Land and Emergency, Environmental Protection Agency Management (5106P) – November 2017, 2017, available online: https://www.epa.gov/fedfac/technical-fact-sheet-polybrominated-diphenyl-ethers-pbdes-and-polybrominated-biphenyls-pbbs Search PubMed.
Footnote |
† Electronic supplementary information (ESI) available. See DOI: 10.1039/d1em00316j |
|
This journal is © The Royal Society of Chemistry 2021 |