Polyvinyl chloride in consumer and environmental plastics, with a particular focus on metal-based additives
Received
3rd June 2021
, Accepted 29th July 2021
First published on 30th July 2021
Abstract
Polyvinyl chloride (PVC) is one of the most widely used thermoplastics but is also a material of concern because of the generation and release of harmful chemicals during its life cycle. Amongst the chemicals added to PVC are metal-based stabilisers and Sb-based halogenated flame retardant synergists. However, very little quantitative information exists on these additives, and in particular in PVC lost to the environment. In this study, the distribution of PVC amongst consumer plastics in societal circulation and plastics retrieved from marine and lacustrine beaches and agricultural soils are compared, along with the presence and concentrations of Ba, Cd, Pb, Sb, Sn and Zn as proxies for common metal-based additives and determined by X-ray fluorescence spectrometry. About 10% of consumer plastics and 2% of environmental plastics were constructed of PVC, with the discrepancy attributed to the long service lives and managed disposal of PVC used in the construction sector and the propensity of the plastic to sink in aquatic systems and evade detection. Metal-based additives, defined as having a metal concentration >1000 mg kg−1, were present in about 75% of consumer and environmental PVC, with Ba and Pb most abundant and Cd and Zn least abundant in both types of sample, and median concentrations statistically different only for Ba. Metals also appeared to be present as contaminants (defined as concentrations <1000 mg kg−1) arising from manufacturing or recycling. Metals in PVC are believed to pose little risk when the material is in use, but experimental evidence in the literature suggests that significant mobilisation and exposure may occur from PVC microplastics when ingested by wildlife.
Environmental significance
Polyvinyl chloride (PVC) is a thermoplastic present in many common consumer products ranging from pipes, flooring, window frames and wire insulation to adhesive taping, medicine packing, raincoats and rucksacks. PVC has long suffered from “bad press”, mostly because of the addition of organic compounds as functional additives; however, the possible effects of the presence of metal-based additives has received much less attention. Measurements of chlorine and selected chemical elements in PVC consumer products and in improperly managed and disposed PVC show that the presence and concentrations of potentially hazardous metals are similar in both sample types, but micronization and weathering in the environment might enhance metal mobility.
|
Introduction
Polyvinyl chloride (PVC) is a vinyl polymer with the chemical formula (C2H3Cl)n whose key properties are given in Table 1. First patented in 1913 and commercially produced in 1933, this thermoplastic has had widespread uses in various sectors because of its durability, inherent flame retardancy, excellent chemical and mechanical properties and ability to accommodate a wide range of additives through its high polarity. PVC resins may be either rigid (unplasticised), and produced mainly by extrusion or moulding, or flexible (plasticised), where plasticisers are added in high proportions (up to 50%) to engender softness and elasticity. Recent estimates indicate that PVC accounts for 12–25% of all plastic manufactured globally and is exceeded only by polypropylene and/or polyethylene.2–4 Unplasticised PVC is used in plumbing, construction, cladding, fencing, seating, drainage systems and in hard, interior and exterior automotive parts, while applications of plasticised PVC include hosing, electrical cable insulation, flooring, medical devices, elastic automotive parts, waterproof clothing and inflatable toys.
Table 1 Key properties and characteristics of unplasticised PVC1
CAS number |
9002-86-2 |
Molecular weight of repeat unit, g mol−1 |
62.5 |
Mass percentage of Cl |
56.7 |
Glass transition temperature, K |
344–380 |
Tensile strength, MPa |
56.6 |
Density @ 20 °C, g cm−3 |
1.391–1.431 |
Despite its extensive production and wide applications, PVC has been referred to as a “contested” versatile material because of concerns about risks to human health from its life cycle that have been raised by scientific research and regulatory bodies.5 These concerns mainly result from the generation and release of harmful chemicals during production, usage and disposal of the material. Thus, although PVC is considered relatively inert, the generation of chlorine itself is associated with hazardous by-products, while the long-term physical and chemical breakdown of the plastic and its disposal via combustion are responsible for environmental emissions of various toxic organochlorine substances and additives.6 Regarding the latter, of greatest concern has been the use of phthalic acid esters as plasticisers, and cadmium- and lead-based primary thermal stabilisers to prevent dehydrohalogenation and to neutralise hydrochloric acid (HCl).7,8 Use of the most harmful phthalates is subject to various recent or pending restrictions. For instance, in Europe, one of the phthalates most widely used in PVC (di(2-ethylhexyl)phthalate) was registered under the European Union's REACH legislation in 2010, with use after 2015 requiring authorisation.9 Regarding cadmium (Cd) and lead (Pb) compounds, European producers and stakeholders voluntarily phased out their intentional introduction into PVC in 2007 and 2015, respectively.10 Preferred alternative, phthalate-free plasticisers that have been used or are being developed include citrates, benzoates, aliphatic esters and bio-based compounds,11 and, while safer metal-based stabilisers are still in use (such as salts of barium, Ba, and zinc, Zn, and organotin compounds), there have been efforts to replace all metal compounds with organophosphites.12
Despite its widespread use and the presence of harmful additives, there is relatively little documentation of the occurrence, distribution and characterisation of PVC in the environment compared with other plastics,13,14 with many reviews failing to discuss or even mention the polymer.15,16 Moreover, where the toxicity of the plastic is studied empirically, there is often a distinct lack of material characterisation and identification or quantification of the additives present.17–21 To this end, we present a compilation of data derived in our laboratory from X-ray fluorescence analyses on the relative abundance of PVC in both consumer plastics and plastics lost to the environment (environmental plastics). Specifically, we report and compare the degree of chlorination and concentrations of metals (including the metalloid antimony, Sb) used in stabilising additives and halogenated flame retardant synergists in consumer and environmental samples of PVC. We use this information and the more general properties of the plastic to gain insights into its environmental behaviour, fate and impacts.
Materials and methods
Samples
Moulded hard and soft plastics, excluding foams, paints, rubbers, waxes and textiles, were considered in the present study. Consumer plastics (n = 1056) were accessed or sourced from households, university offices, schools, shops and restaurants in Plymouth (SW England) and as described in Turner and Filella.22 Items or distinct components thereof were grouped as electronic (mainly housings and wire insulation of small and large domestic appliances), food-hygiene (food-contact and packaging for food, cosmetics and medicines), construction-storage (plumbing, fixtures, flooring, frames, cans, cases, hangers), tools-office (stationery, DIY, adhesive taping, book covers), leisure (toys, games, sports gear, hobbies, crafts) or clothing-accessories (raincoats, jewellery, straps, rucksacks, shoes, buttons).
Environmental plastics (n = 1980) were collected and analysed as part of previous studies23–25 or for the purposes of the present research. Plastics were grouped as: beached plastic litter (mainly articles and fragments >5 mm in size), beached fishing equipment (mainly netting and rope fragments) and beached “small” plastics (visible, primary and secondary particles <5 mm in length or diameter) retrieved from the coastal strandlines of southwest England; beached litter obtained from the shores of Lake Geneva (mainly articles and fragments >5 mm; note that fishing gear and small plastics were largely absent here); and agricultural plastic waste (>5 mm) sampled from fields of Luxembourg and central Spain. Environmental samples were cleaned under tap water and dried before being analysed.
XRF analysis
Samples were analysed by standardless energy-dispersive field-portable-XRF using a Niton XL3t 950 He GOLDD+ that was operated in a low-density “plastics” mode and employed a thickness correction algorithm down to 50 μm. Most samples were analysed in the laboratory within an accessory stand and via a laptop after thickness of the measurement surface had been measured with digital callipers or estimated from the known thickness of similar products. An initial, ∼2 s analysis of characteristic chlorine peaks determined whether the sample was constructed of PVC or an alternative (and usually lighter) plastic, with PVC operationally defined as having a chlorine content >15% by weight. This was succeeded by counting periods up to 200 seconds (depending on sample size and thickness) distributed equally or in a 2
:
1 ratio between a “main” energy range (50 kV and 40 μA) and a “low” energy range (20 kV and 100 μA). Spectra were quantified by fundamental parameter coefficients to yield elemental concentrations on a dry weight basis (in mg kg−1) and with a measurement counting error of 2σ (95% confidence) that were downloaded to the laptop via Niton data transfer software. In addition to Cl, elements of interest were those used in metal-based stabilisers in PVC (Ba, Cd, Pb, Sn, Zn) and the halogenated flame retardant synergist, Sb.
For performance checks, Niton (UK) and Modern Analytical Techniques (New Jersey) polyethylene and PVC reference discs certified for different combinations and concentrations of Ba, Cd, Pb and Sb (PN 180-554, batch SN PE-071-N; PN 180-619, LOT#T-81; SN PVC-4C80, cal set #16) were analysed during each sample measurement session. Concentrations returned were always within 15% and usually within 10% of certified values.
For permanent fixtures or items too large to be contained within the accessory stand, the XRF was employed handheld under the operating conditions described above. The detector window was placed firmly over the measurement surface and the instrument was operated using the trigger mechanism and touch-screen control panel. Reference discs placed on a solid, attenuating surface were analysed at regular intervals, with concentrations returned that were always within 20% and usually within 15% of certified values.
XRF detection limits for a given element, and based on three counting errors, vary according to the composition, thickness and density of material and the mode of operation of the instrument. However, detection limits for the plastic samples in the present study usually ranged from about 5 to 15 mg kg−1 for Pb, 20 to 50 mg kg−1 or Cd, Sb, Sn and Zn, and 50 to 300 mg kg−1 for Ba and Cl.
FTIR analysis
As a check on the ability of the XRF to discriminate PVC from other plastics, selected samples returning a range of Cl concentrations (n = 41) were analysed by Fourier transform infra-red (FTIR) spectroscopy using a Bruker ALPHA Platinum attenuated total reflection QuickSnap A220/D-01 spectrometer. Flakes of ∼1 mg were sliced from each sample in the region analysed by XRF using a stainless steel scalpel before being clamped down on to the ATR diamond crystal. Measurements, consisting of 16 scans in the range 4000 to 400 cm−1 and at a resolution of 4 cm−1, were activated via Bruker OPUS spectroscopic software. Polymer identification involved a comparison of sample transmittance spectra with libraries of reference spectra and a hit quality of >70%.
Results and discussion
Identification of PVC
Fig. 1 shows the results of FTIR analysis of selected consumer and environmental samples along with the corresponding Cl concentrations determined by XRF. In red (and hatched) and blue are samples defined as PVC and non-PVC, respectively, according to XRF criteria. In the majority of cases, there is a clear distinction in Cl content between PVC and other plastics and XRF correctly identifies PVC. The one false negative involved a complex, multi-component fragment of beach litter in which the different resolutions of each technique may have contributed to the discrepancy (FTIR requires a surface flake of ∼1 mm while XRF collimation is either 3 mm or 8 mm in diameter). In support of XRF as a rapid and non-destructive means of identifying PVC, a recent study found that an Oxford Instruments portable device operated in a “soils” mode and tested on various consumer and environmental samples was 100% accurate for this plastic.26
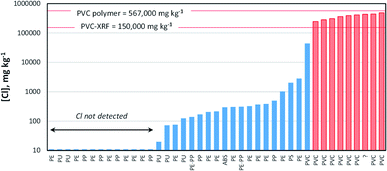 |
| Fig. 1 A selection of plastics analysed by XRF and shown in ascending order of Cl content along with the polymer identified by FTIR (ABS = acrylonitrile butadiene styrene; PE = polyethylene; PP = polypropylene; PS = polystyrene; PU = polyurethane; ? = uncertain and hit quality <70%). Data shown in red and hatched are samples defined as PVC by XRF ([Cl] >150 000 mg kg−1). Also annotated is the theoretical Cl content of pure PVC. | |
PVC amongst consumer and environmental samples
Within the constraints above, Table 2 presents a summary of the PVC samples encountered in each group of the consumer and environmental plastics considered in the present study. Note that XRF is unable to directly discriminate plasticised and unplasticised forms of the polymer, but samples were readily defined as “flexible” or “rigid” based on their appearance and elasticity. Amongst the plastics in circulation with consumers (n >1000), the storage-construction group exhibited the greatest percentage contribution of PVC to total plastic samples (∼20), with PVC articles including hosing and piping, frames for windows and doors, vinyl flooring and storage wallets, while the food-hygiene group displayed the lowest percentage contribution (∼1), with PVC items limited to two tablet blister packs. Overall, about 9% of plastics on a number basis were constructed of PVC. However, given that many of these samples were relatively large construction items, the percentage of the polymer in consumer circulation on a mass or volume basis is likely to be closer to the production figure of 20% reported by the British Plastics Federation.4 Regarding plastics lost to the environment (n ∼ 2000), about 2% overall, and mainly comprised of relatively small (<10 cm) objects and fragments, were constructed of PVC, with percentage contributions in the different groups ranging from less than one for beached marine fishing gear (rope) and small plastics to about five for beached lake samples.
Table 2 Total number of consumer and environmental plastics (by group) analysed by XRF and the corresponding number of samples defined as PVC and as rigid PVC. Also shown are the numbers of PVC samples containing functional metal-based stabilisers, no metal-based stabilisers (“absent”) and Sb as a flame retardant synergist
|
Samples |
PVC |
Rigid |
PVC with metal-based stabiliser |
Sb |
Ba |
Cd |
Pb |
Sn |
Zn |
Absent |
Consumer
|
Electronic |
267 |
16 |
3 |
10 |
0 |
11 |
2 |
2 |
1 |
6 |
Food-hygiene |
172 |
2 |
2 |
0 |
0 |
0 |
2 |
0 |
0 |
0 |
Clothing-accessories |
78 |
9 |
2 |
4 |
0 |
0 |
2 |
1 |
2 |
0 |
Office-tools |
118 |
17 |
9 |
8 |
0 |
3 |
3 |
2 |
4 |
3 |
Storage-construction |
130 |
25 |
13 |
11 |
2 |
8 |
4 |
2 |
5 |
2 |
Leisure |
291 |
23 |
10 |
12 |
0 |
1 |
5 |
1 |
9 |
0 |
Total |
1056 |
92 |
39 |
45 |
2 |
23 |
18 |
8 |
21 |
11 |
![[thin space (1/6-em)]](https://www.rsc.org/images/entities/char_2009.gif) |
Environmental
|
Beached (marine) |
306 |
10 |
4 |
3 |
0 |
2 |
2 |
3 |
2 |
2 |
Beached (marine, fishing) |
91 |
0 |
0 |
0 |
0 |
0 |
0 |
0 |
0 |
0 |
Beached (marine, micro-) |
923 |
3 |
3 |
0 |
0 |
0 |
0 |
0 |
1 |
0 |
Beached (lacustrine) |
603 |
31 |
20 |
9 |
2 |
13 |
3 |
1 |
7 |
3 |
Agricultural soils |
57 |
2 |
1 |
0 |
0 |
0 |
1 |
0 |
1 |
0 |
Total |
1980 |
46 |
28 |
12 |
2 |
15 |
6 |
4 |
11 |
5 |
Chlorine content and metal-based functional additive concentrations in consumer and environmental PVC
Also shown in Table 2 is a summary of the number of samples in each category and group containing functional and deliberately added stabilisers based on the five metals: Ba, Cd, Pb, Sn, Zn. Here, the presence of a functional stabiliser was assumed when the concentration of an individual metal exceeded 1000 mg kg−1; this is equivalent to a minimum metal-based stabilising compound content in PVC of about 2000 mg kg−1 reported by Baitz et al.27 Note that the presence of each metal in this form is not mutually exclusive and that “absent” means that there was no evidence of any functional metal-based stabiliser. Specifically, Ba and Pb exhibited the greatest number of co-associations as additives while Sn exhibited the fewest number of associations with any other metal. Note also that metal concentrations lower than 1000 mg kg−1 were assumed to be present as contaminants of PVC manufacture or recycling or through acquisition from the environment. The number of PVC samples containing Sb as a halogenated flame retardant synergist (principally in wire insulation28), and likewise defined by its presence above 1000 mg kg−1, is also given in Table 2. A quantitative summary of the concentrations of the different elements present as functional additives in the consumer and environmental PVC samples (and where, by operational definition, [Cl] >150
000 mg kg−1 and other elements >1000 mg kg−1) is provided in Table 3. Also shown are results of a series of Mann–Whitney tests performed in Minitab v19 that compare median concentrations of each element in consumer and environmental samples.
Table 3 Summary statistics for the concentrations of the different elements as functional stabilisers in consumer and environmental samples of PVC (defined as >150
000 mg kg−1 for Cl and >1000 mg kg−1 for metals and Sb) and results of median value comparisons using a series of Mann–Whitney tests
|
|
Cl |
Ba |
Cd |
Pb |
Sn |
Zn |
Sb |
Consumer |
n
|
92 |
45 |
2 |
23 |
18 |
8 |
11 |
Mean, mg kg−1 |
304 000 |
16 800 |
1630 |
11 800 |
2700 |
2120 |
7530 |
Median, mg kg−1 |
286 000 |
9210 |
1630 |
12 100 |
2500 |
1580 |
6730 |
Min, mg kg−1 |
151 000 |
1100 |
1580 |
1610 |
1020 |
1100 |
1390 |
Max, mg kg−1 |
635 000 |
75 500 |
1680 |
25 100 |
5100 |
4350 |
17 700 |
Environmental |
n
|
46 |
12 |
2 |
15 |
6 |
4 |
5 |
Mean, mg kg−1 |
342 000 |
43 900 |
3440 |
10 100 |
2290 |
5000 |
9140 |
Median, mg kg−1 |
360 000 |
3370 |
3440 |
9200 |
2020 |
3750 |
6510 |
Min, mg kg−1 |
151 000 |
1130 |
2640 |
1140 |
1020 |
1260 |
1460 |
Max, mg kg−1 |
532 000 |
319 000 |
4240 |
19 000 |
4550 |
11 200 |
23 000 |
Difference in medians |
p-Value |
0.040 |
0.035 |
0.245 |
0.634 |
0.484 |
0.203 |
0.981 |
Regarding Cl, concentrations ranged from about 150
000 mg kg−1 in both consumer and environmental PVC samples that were flexible to over 600
000 mg kg−1 in two rigid consumer plastics. Assuming that there are no significant contributions from other, chlorine-bearing additives in the samples, the measured Cl content can be used to estimate the PVC content (=[Cl] × 100/56.7, and ranging from 27 to 112%), with the remaining mass contribution providing an estimate of the total additive content of the sample. Thus, lower Cl concentrations are predicted to be associated with a relatively high proportion of plasticisers, stabilisers, fillers, rubbers and pigments (up to 74% by weight), while concentrations approaching the theoretical Cl content of PVC (567
000 mg kg−1) are predicted to contain a relatively small proportion of additives. These estimates are consistent with information provided by Baitz et al.27 that suggests the PVC content of consumer and industrial articles ranges from about 25% for vinyl flooring to 92% for hard food and mechanical packaging and piping (or an additive content ranging from about 75% to 8%, respectively). The makeup of samples whose measured Cl content exceeds the theoretical maximum for PVC cannot, however, be explained on this basis. Here, we suspect that the component polymer is chlorinated PVC, a direct derivative of PVC (containing about 670
000 mg kg−1 of Cl) that offers a higher temperature service range and greater corrosion and chemical resistance.29
Overall, the median Cl concentration in the environmental samples was significantly greater than the median value in the consumer plastics (p = 0.040). Based on the dehydrochlorination of PVC that occurs during photodegradation,30 one might expect environmental plastics to have a lower Cl content than unweathered consumer samples. However, according to Table 2, there was a higher proportion of rigid samples among environmental plastics (about 61%) than consumer plastics (about 42%), suggesting an inherently lower plasticiser content (or higher Cl content) of samples lost to the environment.
Based on the measured concentration of Ba, Cd, Pb, Sn and Zn, functional metal-based stabilisers were evident in about 75% of all consumer samples constructed of PVC (n = 92). Barium-based compounds appeared to be the most commonly employed stabiliser (although other uses of Ba compounds, such as an inert filler, cannot be excluded) and the metal was encountered in all groups with the exception of food-hygiene and up to concentrations of about 75
000 mg kg−1. In flexible and semi-rigid PVC, mixed salts of Ba and Cd were used until safer alternatives based on Ba and Zn salts of fatty acids or phenolates were introduced.8 By contrast, Cd-based stabilising compounds were only present in two samples (and in association with Ba) within the storage-construction group and at concentrations of about 1500 mg Cd per kg, and Zn-based compounds were present in eight samples (and usually in the presence of Ba) across a range of groups at concentrations ranging from about 1000 to 4000 mg Zn per kg. Organotin stabilisers, such as dibutyltin maleate or di-n-alkyltin mercaptides, are usually employed in rigid PVC8 but were encountered in 18 hard and flexible consumer samples at concentrations up to about 5000 mg Sn per kg and were the only stabilisers detected in the food-hygiene group. Lead-based compounds were found in 23 consumer samples over a wide concentration range (about 1600 to 25
000 mg Pb per kg), reflecting the broad array of lead soaps and salts used as stabilisers in PVC, including stearates, phthalates, sulphates, carbonates and phosphites,31 and the durability and long service life of PVC products containing leaded compounds.27 Significantly, and unlike the case for other plastics, including polyethylene, polypropylene and polystyrene,23,32 Pb was never detected in the presence of Cr, suggesting that lead chromate is not commonly employed as a colour pigment in PVC.
A similar percentage of environmental PVC samples (n = 46) contained functional metal-based stabilisers. Distributions and concentration ranges of the different metals were comparable with those of consumer samples, with Ba and Pb most abundant and Cd and Zn least abundant, and a significant difference in median concentrations of environmental and consumer samples was only evident for Ba (p = 0.035). Antimony, as a synergist, exhibited similar distributions between consumer and environmental samples of PVC, with no statistically significant difference in median concentrations. In summary, therefore, it would appear that the stocks of PVC in societal circulation and lost to the environment through littering and poor waste management have broadly common chemical signatures.
Residual metal concentrations
Table 4 shows summary statistics for metals and Sb detected in consumer and environmental PVC samples at concentrations below 1000 mg kg−1. Here, it is assumed that concentrations are too low to function as additives and are therefore present as residues from the manufacturing or recycling of PVC. Note that while acquisition of metals from the environment is possible (through adsorption, for example), concentrations arising from this route are much lower than the detection limit of the XRF.33–35 When PVC is mechanically recycled, functional additives are retained, an approach that is favoured during the controlled reconstitution of the same type of product. However, less controlled and more open mechanical recycling may result in additives being introduced into different types of product (such as Sb in non-electrical goods) or being dispersed at lower concentrations into more general, blended recyclates which are often mixed with virgin PVC.36
Table 4 Summary statistics for the concentrations of the different metals and Sb as residues in consumer and environmental samples of PVC (defined as <1000 mg kg−1) and results of median value comparisons using a series of Mann–Whitney tests (nd = not determined)
|
|
Ba |
Cd |
Pb |
Sn |
Zn |
Sb |
Consumer |
n
|
4 |
10 |
5 |
20 |
23 |
5 |
Mean, mg kg−1 |
628 |
257 |
393 |
300 |
306 |
279 |
Median, mg kg−1 |
643 |
226 |
546 |
226 |
254 |
279 |
Min, mg kg−1 |
373 |
120 |
86 |
39 |
34 |
135 |
Max, mg kg−1 |
852 |
476 |
621 |
913 |
866 |
470 |
Environmental |
n
|
15 |
5 |
1 |
4 |
18 |
5 |
Mean, mg kg−1 |
396 |
300 |
37 |
51 |
330 |
96 |
Median, mg kg−1 |
388 |
250 |
37 |
57 |
274 |
77 |
Min, mg kg−1 |
180 |
36 |
37 |
34 |
36 |
55 |
Max, mg kg−1 |
735 |
857 |
37 |
103 |
805 |
226 |
Difference in medians |
p-Value |
0.147 |
0.668 |
nd |
0.011 |
0.646 |
0.037 |
The data suggest that residual contamination is common and heterogeneous in both consumer and environmental samples of PVC, with a range in elemental concentrations, a variety of co-occurrences amongst the elements, and their presence with stabilisers (above 1000 mg kg−1) in both rigid and flexible forms of the plastic. In total, 47 consumer samples and 27 environmental samples (or about 50% and 60% of total PVC samples, respectively) contained detectable residuals, and median concentrations of Sb and Sn were significantly lower in environmental samples than in consumer samples, possibly because of their greater mobilization under environmental conditions. We note a low incidence of residual Pb amongst the samples compared with its occurrence as a functional additive which, presumably, reflects efforts to eliminate or recover the metal chemically or to recycle it in a more controlled manner.36 Overall, seven consumer samples and two environmental samples were “clean” and free of detectable metals and Sb.
Differences in PVC abundance between consumer and environmental samples
Despite similarities in the abundance and concentration range of the elemental constituents of PVC in consumer goods and environmental samples, the polymer itself is considerably less abundant amongst the plastic groups in the latter category. Moreover, the polymer was entirely absent from the several hundred pre-production pellets retrieved amongst the small beached plastics in southwest England, despite the plastic being processed by injection moulding.37 The low quantities of PVC samples retrieved from the environment compared with its occurrence in consumer plastics and its global production may be attributed to a number of factors.
First, a significant fraction of plastic litter in the environment is derived from food packaging38,39 and the data in Table 2 appear to suggest that the contemporary and recent use of PVC in this sector is limited. More specifically, in the marine environment, large quantities of plastic waste are derived from fishing activities, and in particular fragments and offcuts of cord, netting and rope, where PVC has few uses.40 Secondly, many of the applications of PVC are large, “long-life”, construction articles whose disposal is more carefully managed and regulated than other, smaller or single-use consumer plastics.41 Specifically, Baitz et al.27 report that the lifespans of construction PVC articles may be up to 30 years, and that about 70% of PVC produced has a service life of at least a decade. Thirdly, the higher density of PVC (the polymer density is about 1.4 g cm−3 according to Table 1 but this may be increased by the presence of metal-based additives) compared with other consumer plastics means that a greater proportion of this material that is discharged into surface waters, lost at sea or entering water treatment facilities is likely to sink. In theory, therefore, PVC has a greater propensity to evade both long-range transport in surface waters and beaching along strandlines where plastics are often sampled and studied. Rather, it is predicted to settle relatively rapidly and accumulate in benthic environments that are more inaccessible and less well researched.42,43
PVC microplastics and metal mobilisation and toxicity
Environmental microplastics of smaller (sub-mm) dimensions than the small plastics referred to above have been subject to extensive scientific scrutiny over the past few years and, despite PVC receiving less attention than other plastics, like polyethylene, polypropylene and polystyrene, the recent literature mentioning PVC suggests that it is ubiquitous.44–49 The nature and concentration of additives in microplastics of sub-mm dimensions is difficult to determine readily or accurately. However, it would be reasonable to assume that the chlorine content and signatures of metal- and Sb-based additives and residuals reported above apply to microscopic particles.
Metal-based additives and synergists in PVC products in-life are assumed to be inert and pose no significant health or environmental risks until disposal or unless exposed to fire.27 However, release is more likely from environmental PVC that has weathered and become micronised and that is exposed to physico-chemical conditions favouring the dissolution of inorganic compounds.28,50,51 The inadvertent ingestion and digestion of microplastics by aquatic organisms and birds provides a means by which these conditions are met, and examinations of the gastro-intestinal tracts of bivalves42,52–56 have confirmed that PVC particles are often present. As part of previous research, selected consumer and environmental samples reported in the current paper were micronised (mm-sized) to study the release of metals from PVC (and other plastics) in simulated digestive fluids.57,58 Mobilisation followed diffusion kinetics and mobilities (relative to total contents) ranged from ∼0.01% for Sb from a micronised beached fragment of PVC in a protein-rich invertebrate digestive solution after six hours' incubation to about 10% for Pb from a micronised PVC pipe bend in an acidic avian extraction test after a period of seven days.
Metal mobilisation is not necessarily equivalent to metal accumulation, nor should it imply toxicity. However, two studies have reported a direct link between acute toxicity and the mobilisation of a metal-based additive from PVC particles into the aqueous phase. Thus, first, Lithner et al.59 attributed most of the observed immobility of Daphnia magna to the mobilisation of Zn ions from plasticised PVC (with leachate prepared from 250 g of rubber glove cuttings per L of deionised water). Secondly, Boyle et al.19 showed that zebrafish larvae exposed to 150 μm PVC particles (of unspecified origin and presumably rigid) at up to 500 mg L−1 for 24 h increased expression of metallothionein 2, a metal-binding protein, but not biomarkers of estrogenic or organic contaminants, with subsequent analysis of PVC leachates revealing that the release of Pb was responsible. Although performed under environmentally unrealistic conditions (excessive particle concentrations), these studies suggest the potential for both restricted and unrestricted metal-based additives, and in both plasticised and unplasticised PVC, to exert toxic responses in aquatic organisms. Clearly, a greater understanding of the presence and mobilities of metal additives is required for PVC that is lost to the environment.
Conclusions
The data presented here suggest that, at least on a number basis, PVC is more abundant amongst consumer articles than plastics lost to the environment. This may be attributed to the relatively low contribution of PVC to common litter and fishing gear, the use of PVC in larger, long-lasting products whose disposal is more carefully managed, and the propensity of PVC to sink and evade ready detection in many aquatic systems. Environmental PVC has a greater chlorine content than consumer PVC, possibly because of a greater abundance of rigid (unplasticised) plastics in the environment. However, the concentrations and distributions of metal-based additives and Sb-based flame retardant synergist is similar between the two categories of PVC, despite differences in the relative abundance of the material and regulations that have been introduced to reduce Cd and Pb. These additives are believed to pose little risk when PVC is in use, but experimental evidence indicates that significant mobilisation and exposure may occur from PVC microplastics that are ingested by wildlife.
Conflicts of interest
There are no conflicts to declare.
References
-
A. L. Andrady, Poly(vinyl chloride), in Polymer Data Handbook, ed. M. E. James, Oxford University Press, 1999, pp. 928–934 Search PubMed.
- R. Geyer, J. R. Jambeck and K. L. Law, Production, use, and fate of all plastics ever made, Sci. Adv., 2017, 3, e1700782 CrossRef PubMed.
- L. Ye, C. Qi, J. Hong and X. Ma, Life cycle assessment of polyvinyl chloride production and its recyclability in China, J. Cleaner Prod., 2017, 142, 2965–2972 CrossRef CAS.
-
British Plastics Federation, Polyvinyl chloride PVC, 2021, https://www.bpf.co.uk/plastipedia/polymers/pvc.aspx#:%7E:text=PVC%20is%20a%20versatile%20material,coverings%2C%20fashion%20and%20footwear%2C%20packaging, accessed April 2021 Search PubMed.
-
G. Akovali, Plastic materials: Polyvinyl chloride (PVC), in Toxicity of Building Materials, ed. F. Pacheco-Torgal, S. Jalali and A. Fucic, Woodhead Publishing Series in Civil and Structural Engineering, 2012, pp. 23–53 Search PubMed.
- J. Leadbitter, PVC and sustainability, Prog. Polym. Sci., 2002, 27, 2197–2226 CrossRef CAS.
- A. O. Earls, I. P. Axford and J. H. Braybrook, Gas chromatography-mass spectrometry determination of the migration of phthalate plasticisers from polyvinyl chloride toys and childcare articles, J. Chromatogr. A, 2003, 983, 237–246 CrossRef CAS.
-
J. E. McGrath, M. A. Hickner and R. Höfer, Polymers for a Sustainable Environment and Green Energy, Elsevier, Amsterdam, 2012 Search PubMed.
- European Council for Plasticisers and Intermediates, European Parliament targets DEHP plasticizer in recycled PVC, Addit. Polym., 2016, 2, 11 Search PubMed.
-
VinylPlus, The European PVC industry's experience in replacing lead and cadmium-based stabilisers, 2014, https://www.stabilisers.eu/wp-content/uploads/2015/11/VinylPlus_Contribution-Cefic_Eu-Industry.pdf, accessed April 2021 Search PubMed.
- T. T. Bui, G. Giovanoulis, A. P. Cousins, J. Magner, I. T. Cousins and C. A. de Wit, Human exposure, hazard and risk of alternative plasticizers to phthalate esters, Sci. Total Environ., 2016, 541, 451–467 CrossRef CAS PubMed.
-
V. Ambrogi, C. Carfagna, P. Cerruti and V. Marturano, Additives in polymers, in Modification of Polymer Properties, ed. C. F. Jasso-Gastinel and J. M. Kenny, William Andrew Publishing, 2017, pp. 87–108 Search PubMed.
- H. A. Nel, G. H. S. Smith, R. Harmer, R. Sykes, U. Schneidewind, I. Lynch and S. Krause, Sources, transport, and accumulation of different types of plastic litter in Sediment, Environ. Pollut., 2020, 264, 114696 CrossRef PubMed.
- C. Bertoldi, L. Z. Lara, F. A. D. Mizushima, F. C. G. Martins, M. A. Battisti, R. Hinrichs and A. N. Fernandes, First evidence of microplastic contamination in the freshwater of Lake Guaiba, Porto Alegre, Brazil, Sci. Total Environ., 2021, 759, 143503 CrossRef CAS PubMed.
- G. Erni-Cassola, V. Zadjelovic, M. I. Gibson and J. A. Christie-Oleza, Distribution of plastic polymer types in the marine environment; A meta-analysis, J. Hazard. Mater., 2019, 369, 691–698 CrossRef CAS PubMed.
- M. Padervand, E. Lichtfouse, D. Robert and C. Wang, Removal of microplastics from the environment: A review, Environ. Chem. Lett., 2020, 18, 807–828 CrossRef CAS.
- M. Oliveiro, T. Tato, S. Schiavo, V. Fernandez, S. Manzo and R. Beiras, Leachates of micronized plastic toys provoke embryotoxic effects upon sea urchin Paracentrotus lividus, Environ. Pollut., 2019, 247, 706–715 CrossRef.
- Y. M. Wu, P. Y. Guo, X. Y. Zhang, Y. X. Zhang, S. T. Xie and J. Den, Effect of microplastics exposure on the photosynthesis system of freshwater algae, J. Hazard. Mater., 2019, 375, 219–227 CrossRef.
- D. Boyle, A. I. Catarino, N. J. Clark and T. B. Henry, Polyvinyl chloride (PVC) plastic fragments release Pb additives that are bioavailable in zebrafish, Environ. Pollut., 2020, 263, 114422 CrossRef CAS.
- S. Pignattelli, A. Broccoli and M. Renzi, Physiological responses of garden cress (L. sativum) to different types of microplastics, Sci. Total Environ., 2020, 727, 138609 CrossRef CAS PubMed.
- L. Zimmermann, S. Göttlich, J. Oehlmann, M. Wagner and C. Volker, What are the drivers for plastic toxicity? Comparing the toxicity of plastic chemicals and particles to Daphnia magna, Environ. Pollut., 2020, 267, 115392 CrossRef CAS PubMed.
- A. Turner and M. Filella, Bromine in plastic consumer products – Evidence for the widespread recycling of electronic waste, Sci. Total Environ., 2017, 601–602, 374–379 CrossRef CAS PubMed.
- A. Turner and K. R. Solman, Analysis of the elemental composition of marine litter by field-portable-XRF, Talanta, 2016, 159, 262–271 CrossRef CAS.
- A. Massos and A. Turner, Cadmium, lead and bromine in beached microplastics, Environ. Pollut., 2017, 227, 139–145 CrossRef CAS PubMed.
- M. Filella and A. Turner, Observational study unveils the extensive presence of hazardous elements in beached plastics from Lake Geneva, Front. Environ. Res., 2018, 6, 1, DOI:10.3389/fenvs.2018.00001.
- A. P. M. Michel, A. E. Morrison, V. L. Preston, C. T. Marx, B. C. Colson and H. K. White, Rapid identification of marine plastic debris via spectroscopic techniques and machine learning classifiers, Environ. Sci. Technol., 2020, 54, 10630–10637 CrossRef CAS PubMed.
-
M. Baitz, J. Kreissig, E. Byrne, C. Makishi, T. Kupfer, N. Frees, N. Bey, M. S. Hansen, A. Hansen, T. Bösch, V. Borghi, J. Watson and M. Mirnada, Life Cycle Assessment of PVC and of principal competing materials, Final Report for the European Commission, Brussels, 2004, https://pure.au.dk/portal/files/13386408/pvc-final_report_lca.pdf, accessed May 2021 Search PubMed.
- P. V. Mercea, C. Losher, M. Petrasch and V. Tosa, Migration of stabilizers and plasticizer from recycled polyvinylchloride, J. Vinyl Addit. Technol., 2018, 24, E112–E124 CrossRef CAS.
-
T. Walsh, The plastic piping industry in North America, in Applied Plastics Engineering Handbook, ed. M. Kutz, William Andrew Publishing, 2011, pp. 625–644 Search PubMed.
- C. A. D'Aquino, W. Balmant, R. L. L. Ribeiro, M. Munaro, J. V. C. Vargas and S. C. Amico, A simplified mathematical model to predict PVC photodegradation in photobioreactors, Polym. Test., 2012, 31, 638–644 CrossRef.
- Y. B. Liu, W. Q. Liu and M. H. Hou, Metal dicarboxylates as thermal stabilizers for PVC, Polym. Degrad. Stab., 2007, 92, 1565–1571 CrossRef CAS.
-
C. Maier and T. Calafut, Polypropylene: The Definitive User's Guide and Databook, William Andrew, Norwich NY, 1998, p. 431 Search PubMed.
- K. Ashton, L. Holmes and A. Turner, Association of metals with plastic production pellets in the marine environment, Mar. Pollut. Bull., 2010, 60, 2050–2055 CrossRef CAS PubMed.
- M. Carbery, G. R. MacFarlane, W. O'Connor, S. Afrose, H. Tayloer and T. Palanisami, Baseline analysis of metal(loid)s on microplastics collected from the Australian shoreline using citizen science, Mar. Pollut. Bull., 2020, 152, 110914 CrossRef CAS PubMed.
- I. Martins, Y. Rodriguez and C. K. Pham, Trace elements in microplastics stranded on beaches of remote islands in the NE Atlantic, Mar. Pollut. Bull., 2020, 156, 111270 CrossRef CAS PubMed.
-
J. Ooms and J. G. Cuperus, Impact of Lead Restrictions on the Recycling of PVC, Tauw BV, Deventer, NL, 2013, https://vinylplus.eu/uploads/Modules/Documents/2013_07_13-impact_lead-restrictions_pvc_recycling-tauw.pdf, accessed May 2021 Search PubMed.
- T. Garbacz, Structure and properties of cellular injection molded products, Polimery, 2013, 58, 295–303 CrossRef.
- C. Y. Barlow and D. C. Morgan, Polymer film packaging for food: An environmental assessment, Resour., Conserv. Recycl., 2013, 78, 74–80 CrossRef.
- T. Kiessling, K. Knickmeier, K. Kruse, D. Brennecke, A. Nauendorf and M. Thiel, Plastic Pirates sample litter at rivers in Germany – Riverside litter and litter sources estimated by schoolchildren, Environ. Pollut., 2019, 245, 545–557 CrossRef CAS PubMed.
- N. A. Welden and P. Cowie, Degradation of common polymer ropes in a sublittoral marine environment, Mar. Pollut. Bull., 2017, 118, 248–253 CrossRef CAS PubMed.
- A. E. Schwarz, T. Ligthart, E. Boukris and T. van Harmelen, Sources, transport, and accumulation of different types of plastic litter in aquatic environments: A review study, Mar. Pollut. Bull., 2019, 143, 92–100 CrossRef CAS PubMed.
- C. A. Peters, E. Hendrickson, E. C. Minor, K. Schreiner, J. Halbur and S. P. Bratton, Pyr-GC/MS analysis of microplastics extracted from the stomach content of benthivore fish from the Texas Gulf Coast, Mar. Pollut. Bull., 2018, 137, 91–95 CrossRef CAS PubMed.
- P. Xu, G. Peng, L. Su, Y. Gao, L. Gao and D. Li, Microplastic risk assessment in surface waters: A case study in the Changjiang Estuary, China, Mar. Pollut. Bull., 2018, 133, 647–654 CrossRef CAS PubMed.
- Q. Zhou, C. Tian and Y. Luo, Various forms and deposition fluxes of microplastics identified in the coastal urban atmosphere, Kexue Tongbao, 2017, 62, 3902–3910 CrossRef.
- C. C. Cheang, Y. Ma and L. Fok, Occurrence and composition of microplastics in the seabed sediments of the coral communities in proximity of a metropolitan area, Int. J. Environ. Res. Public Health, 2018, 15, 2270 CrossRef CAS.
- C. Du, H. D. Liang, Z. P. Li and J. Gong, Pollution characteristics of microplastics in soils in southeastern suburbs of Baoding City, China, Int. J. Environ. Res. Public Health, 2020, 17, 845 CrossRef CAS.
- A. A. Franco, J. M. Arellano, G. Albendin, R. Rodriguez-Barroso, S. Zahedi, M. Quiroga and M. D. Coello, Mapping microplastics in Cadiz (Spain): Occurrence of microplastics in municipal and industrial wastewaters, J. Water Process. Eng., 2020, 38, 101596 CrossRef.
- D. Kankanige and S. Babel, Identification of micro-plastics (MPs) in conventional tap water sourced from Thailand, J. Eng. Technol. Sci., 2020, 52, 95–107 CrossRef CAS.
- M. Ghayebzadeh, H. Taghipour and H. Aslani, Abundance and distribution of microplastics in the sediments of the estuary of seventeen rivers: Caspian southern coasts, Mar. Pollut. Bull., 2021, 164, 112044 CrossRef CAS PubMed.
- R. M. Town, H. P. van Leeuwen and R. Blust, Biochemodynamic features of metal ions bound by micro- and nano-plastics in aquatic media, Front. Chem., 2018, 6, 627, DOI:10.3389/fchem.2018.00627.
- J. Meng, B. Xu, F. Liu, W. Li, N. Sy, X. Zhou and B. Yan, Effects of chemical and natural ageing on the release of potentially toxic metal additives in commercial PVC microplastics, Chemosphere, 2021, 283, 131274 CrossRef CAS PubMed.
- C. Fang, R. H. Zheng, H. Z. Chen, F. K. Hong, L. S. Lin, H. Lin, H. G. Guo, C. Bailey, H. Segner, J. L. Mu and J. Bo, Comparison of microplastic contamination in fish and bivalves from two major cities in Fujian Province, China and the implications for human health, Aquaculture, 2019, 512, 734322 CrossRef CAS.
- A. Gomiero, P. Strafella, K. B. Oysaed and G. Fabi, First occurrence and composition assessment of microplastics in native mussels collected from coastal and offshore areas of the northern and central Adriatic Sea, Environ. Sci. Pollut. Res., 2019, 26, 24407–24416 CrossRef CAS PubMed.
- G. Pellini, A. Gomiero, T. Fortibuoni, C. Ferrà, F. Grati, A. N. Tassetti, P. Polidori, G. Fabi and G. Scarcella, Characterization of microplastic litter in the gastrointestinal tract of Solea solea from the Adriatic Sea, Environ. Pollut., 2018, 234, 943–952 CrossRef CAS PubMed.
- S. Avery-Gomm, M. Valliant, C. R. Schacter, K. F. Robbins, M. Liboiron, P. Y. Daoust, L. M. Rios and I. L. Jones, A study of wrecked Dovekies (Alle alle) in the western North Atlantic highlights the importance of using standardized methods to quantify plastic ingestion, Mar. Pollut. Bull., 2016, 113, 75–80 CrossRef CAS PubMed.
- R. Furtado, D. Menezes, C. J. Santos and P. Catry, White-faced storm-petrels Pelagodroma marina predated by gulls as biological monitors of plastic pollution in the pelagic subtropical Northeast Atlantic, Mar. Pollut. Bull., 2016, 112, 117–122 CrossRef CAS PubMed.
- E. James and A. Turner, Mobilisation of antimony from microplastics added to coastal aquatic environments: A review study, Mar. Pollut. Bull., 2020, 161, 111776 CrossRef PubMed.
- E. C. Smith and A. Turner, Mobilisation kinetics of Br, Cd, Cr, Hg, Pb and Sb in microplastics exposed to simulated, dietary-adapted digestive conditions of seabirds, Sci. Total Environ., 2020, 733, 138802 CrossRef CAS PubMed.
- D. Lithner, I. Nordensvan and G. Dave, Comparative acute toxicity of leachates from plastic products made of polypropylene, polyethylene, PVC, acrylonitrile–butadiene–styrene, and epoxy to Daphnia magna, Environ. Sci. Pollut. Res., 2012, 19, 1763–1772 CrossRef CAS PubMed.
|
This journal is © The Royal Society of Chemistry 2021 |