Human health risk assessment of atmospheric mercury inhalation around three artisanal small-scale gold mining areas in Indonesia†
Received
10th December 2020
, Accepted 4th August 2021
First published on 23rd August 2021
Abstract
To clarify the human health risk of inhalation of gaseous elemental mercury (GEM), we measured GEM concentrations in three artisanal small-scale gold mining (ASGM) areas of Palu (Central Sulawesi, 342
000 residents), Muara Aman (Benkulu in Sumatra, about 1000 residents), and Palangka Raya (Central Kalimantan, 236
000 residents). These sites differed with regard to the scale and method of gold mining (ball mill and dredging systems). Although the scale of ASGM activity at these three sites differed for both the ball mill and dredging methods, gold amalgams were always made during the refining process. The air in these cities was therefore polluted by the mercury used in extracting the gold, although the levels of pollution differed. The highest GEM concentrations were measured at gold shops (5 × 105 ng m−3). In the ASGM activity area, the measured GEM concentrations were high (100–14
000 ng m−3) near grinding drums. GEM concentrations in Palu and Palangka Raya ranged from 2.06 to 375 ng m−3 and 2.04 to 25.3 ng m−3, respectively. GEM concentrations within an ASGM community area in Muara Aman ranged from 4.10 to 11.5 ng m−3. Our results indicated that the health of residents of Palu—not only in the ASGM area but also in the city—may be at risk due to GEM inhalation. In Muara Aman, the health of people who engage in gold refining and of gold shop employees may be at risk, whereas the health of residents of Palangka Raya appears not to be at risk.
1. Introduction
Even in very small amounts, mercury is toxic to humans and other animals. Emissions of gaseous elemental mercury (GEM) from artisanal small-scale gold mining (ASGM) activities were estimated to account for 40% of total global GEM emissions in 2015 (ref. 1) and ranked first among all GEM emissions.
There have been many reports of the mercury pollution of environmental media such as soil and water2,3 caused by ASGM activities, but the current spatial resolution and range of reported GEM concentrations are limited because of the high costs associated with instruments, electricity, and argon gas, as well as the technical training required for use of automated monitoring techniques.4 Generally, such equipment cannot be installed in ASGM areas. Because of the difficulty of measuring atmospheric mercury, relatively few studies have focused on concentrations of GEM in the atmosphere5–13 compared to studies of mercury in other environmental media and its level of risk to humans.
In ASGM areas, miners dig gold ore illegally using relatively primitive techniques. The reports in several studies of environmental pollution caused by mercury at ASGM sites worldwide6,7,14–17 have raised concerns of harmful effects on vulnerable groups such as women and children.18–20 In areas where ASGM operations are closely spaced, nearby residents may be affected because most of the environment can be contaminated by mercury.21 The price of gold increased sevenfold between 2001 (average gold price 230 USD per oz) and 2013 (average gold price 1600 USD per oz). Although it has since decreased to 1100–1300 USD per oz, the price of gold is still much higher than it was in 2001.22 This increase in the price of gold has led to a resurgent gold rush in developing counties that has resulted in an increase of both organic and inorganic mercury pollution.23–25 Seccatore et al.26 have estimated that the more than 16 million artisanal miners involved in ASGM worldwide in 2011 were producing 380–450 tonnes of gold per year. A global mercury assessment has estimated that ASGM releases 830 tonnes (range 675–1000 tonnes) of mercury into the environment per year.27 The United Nation's Minamata Convention on Mercury, which became effective in August 2017,28 requires a reduction in the use of mercury and mercury compounds at mining sites. However, ASGM activities are closely related to poverty and thus are likely to continue.
The scale of ASGM activity varies between ASGM sites. Some ASGM activity is conducted on a relatively large scale, whereas other ASGM activity is conducted by single families scattered throughout an area. The tendency of scattered ASGM activities to be conducted in remote areas makes it difficult to determine the level of ASGM activity in such areas. Nakazawa et al.7 have reported that not only residents in communities next to ASGM activities but also people who live in urban areas more than 5 km away may be at risk. There are various scales and methods of ASGM, however, and the associated degrees of pollution and levels of risk are rarely reported. There is thus an urgent need to clarify the human health risk associated with ASGM.
The purpose of this study was to clarify the human health risk associated with inhalation of GEM at three ASGM sites in Indonesia. The three sites differed from each other with respect to the scale of ASGM activities and the gold-processing method used. In this study, we focused on ASGM operations that used the dredging and ball mill methods which are the most popular methods used in Indonesia.27,29
2. Materials and methods
2.1 Experimental sites
We observed ASGM activities at three sites in Indonesia: the city of Palu and the village of Muara Aman, where the ball mill method was used, and the city of Palangka Raya, where the dredging method was used (Fig. SI1†).
To clarify the human health risk due to GEM inhalation around the ASGM sites (described in Section 2.4), we assigned people to four categories: workers in gold processing (group 1), workers in gold shops (group 2), residents in the ASGM community (group 3), and residents in a city near the ASGM site (group 4). We then measured the GEM concentrations in the ambient air at the following four sites: (1) gold processing sites; (2) gold shop sites, where the gold–mercury amalgam was heated with an acetylene torch, and each gold miner was paid money for the gold that was obtained; (3) ASGM community sites; and (4) central city sites. We could not measure GEM concentrations at some sites (indicated by dashes in Table 1) because there was no contact person who could provide access to the site.
Table 1 Exposure concentration of each site
Parameter |
Typea |
Value or probability distribution function (ng m−3) |
Datab |
St. Noc |
Methodd |
D deterministic value, P probabilistic distribution function.
Alphabet corresponds to Fig. 4 and SI3.
St. No. (station number) is shown in Fig. SI1.
Corresponds to Fig. 3(a) portable handheld mercury analyser method, (b) high-resolution measurement method, (c) gold trap method, and (d) passive sampling method.
“—” we could not obtain a dataset.
|
Exposure concentration (EC)
|
Palu—ball mill method with large ASGM area
|
Gold processing |
D
|
4080 |
Fig. 4(a)
|
4 |
a |
Gold shop |
— |
— |
— |
— |
—e |
Community area |
P
|
Geometric mean 5.11; geometric standard deviation 1.70 |
Fig. SI3(a) |
5 |
b |
Central city area |
P
|
Geometric mean 4.23; geometric standard deviation 1.56 |
Fig. SI3(b) and (c) |
1, 2 and 3 |
b |
![[thin space (1/6-em)]](https://www.rsc.org/images/entities/char_2009.gif) |
Muara Aman—ball mill method with small ASGM |
Gold processing |
D
|
1300 |
Fig. 4(b)
|
10 |
a |
Gold shop |
D
|
177 × 103 |
Fig. 4(c)–(e)
|
11 and 12 |
a |
Community area |
P
|
Geometric mean 2.46; geometric standard deviation 1.37 |
Fig. SI3(d) |
13 and 15–18 |
c and d |
Central city area |
— |
— |
— |
— |
— |
![[thin space (1/6-em)]](https://www.rsc.org/images/entities/char_2009.gif) |
Parangka Raya—dreadging system method |
Gold processing |
— |
— |
— |
— |
— |
Gold shop |
— |
— |
— |
— |
— |
Community area |
— |
— |
— |
— |
— |
Central city area |
P
|
Geometric mean 1.94; geometric standard deviation 0.91 |
Fig. SI3(e) |
8 and 9 |
c and d |
2.1.1 ASGM area in city of Palu.
The city of Palu (0°53′58′′S, 119°51′59′′E) is the capital of Central Sulawesi (Fig. SI1†). It has a population of 340
000 residents and a total area of 395 km2.30,31 The city, which has a dry climate, is situated between hills and lies 130–190 m above sea level around the estuary of the Palu River at the tip of the long and narrow Palu Bay (Fig. SI2(a)†). A very large area of ASGM activity, Poboya, is located about 5 km northeast from the center of Palu.30,31 At Poboya, miners use the ball mill method to process gold ore (Fig. 1 (a)), and there are gold shops in Poboya (Fig. SI2(b)†). The total area associated with ASGM activity is approximately 7000 ha; some of the mining sites are located within the Poboya Panki Grand Forest Park, where a large gold-mining company holds a concession granted by the Ministry of Energy and Mineral Resources. In addition, there is much family-run, small-scale gold processing activity in this area. A worker at one gold-processing site reported that about 1500 ball mills were used in the area.
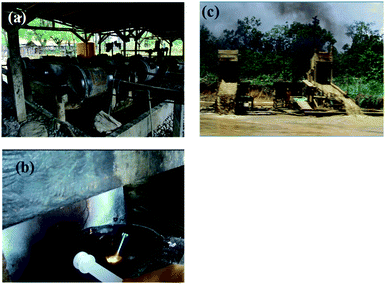 |
| Fig. 1 Details of the measurement sites. (a) Ball mill method in Poboya near Palu, (b) Mercury–gold amalgam is heated, evaporating the mercury and leaving gold at a gold shop, (c) dredging operation in the Kahayan River. | |
The survey was conducted at a gold-processing site, an ASGM community site, and at a central city site in the northern part of Palu. We were unable to survey a gold shop site in the city of Palu.
2.1.2 ASGM area in the village of Muara Aman.
The village of Muara Aman is located in the Benkulu district of Sumatra Island, Indonesia, about 80 km north-northeast of the city of Benkulu, the district's capital. The village is in a valley that runs from northeast to southwest between two mountains. Our survey was conducted in the town area about 4.5 km from the base of the south side of a mountain (3°6′24.1′′–3°7′45.5′′S, 102°11′0.01′′–102°11′50.6′′E). The estimated population of Revon County is 106
000, and the population density is 55 people km−2.32,33 About 1000 people live in the village of Muara Aman. Near this community, there is a gold-mining site that was started by the Dutch a century ago but has since closed.34 However, the people who live in the village of Muara Aman continue to work this gold-mining site illegally. The ball mill method was used in this village. The ball mill used at Muara Aman was smaller than the ball mill used at Poboya and was motorized by hydropower from a waterwheel (Fig. SI2(c)†). The sizes of the ball mills are positively correlated with the amount of mercury used. In many cases, ball mills are operated directly adjacent to a housing area.
In contrast to the ASGM activity in the city of Palu, the ASGM activity in the village of Muara Aman was conducted near the homes of several families. The only ASGM activity we could find in this village was conducted by five or six families, and in most cases those families used only a few ball mills (Fig. SI2(c)†). We could not determine how many families were conducting ASGM activities because they did their work in their backyards. However, the presence of a ball mill manufacturing company in the village suggested that more families may have been involved in ASGM activities in this area.
In the village of Muara Aman, we measured GEM concentrations in the air at a gold-processing site (Fig. SI2(c)†), a gold shop site (Fig. 1(b)), and a site in the community surrounding the ASGM activity (Fig. SI2(d)†).
2.1.3 ASGM area in city of Palangka Raya.
The city of Palangka Raya (2°12′27′′S, 113°54′59′′E) is the capital of Central Kalimantan Province and is located in the Kahayan River basin. The Kahayan River is one of the widest rivers (average width of 450 m) in Central Kalimantan and is 600 km long. The 82 km-long Rungan River flows into the Kahayan River just north of the city of Palangka Raya, and the Kahayan River then flows into the Java Sea (Fig. SI2(e)†). There was no ASGM site in the city of Palangka Raya. However, there were numerous sites of ASGM activity along the Kahayan River and Rungan River. The nearest ASGM site was about 6 km from Palangka Raya. We travelled by boat along the Kahayan River 150 km downstream from Palangka Raya and found that ASGM activity along the river was common. In this area gold mining is conducted via sluices. There are two types of operations. One is an open-pit operation, and the other involves dredging (Fig. 1(c) and SI2(f)†). Open-pit operations are conducted as follows. Soils are excavated and sprayed with a jet of water. Dilute soil is pumped into carpeted sluice boxes, in which gold particles are expected to settle to the carpet because of their high density. In the dredging method, bottom sediments from the river are pumped through a pipe and drained into carpeted sluices boxes. In both operations, people bring the carpets to a gold shop and wash them into a bowl. They then add mercury to form an amalgam. Less mercury is used with the sluice method than with the ball mill method. In 2004, thousands of gold dredging operations were being conducted in the Kahayan watershed, and there were 200 more in the Rungan River watershed.35 Mori et al.36 have estimated that the total outflows of suspended solids from the Kahayan River and Rungan River are 5.22 × 108 kg per year and 2.06 × 108 kg per year, respectively. Such high discharges of suspended solids with elemental mercury from ASGM activities has contaminated the downstream reaches of the rivers.35 Because miners are constantly moving from site to site along the streams, mercury is dispersed along the streams, and it may settle into sediments of the rivers.
Although there is no gold-processing site within the city of Palangka Raya, GEM emitted from ASGM activities conducted in the upper Kahayan River and Rungan River may affect the air in Palangka Raya.
In the city of Palangka Raya, we were able to measure GEM concentrations only at sites in the central city. However, to measure concentrations of GEM that were emitted from gold processing or gold shop sites, we measured GEM concentrations from a boat on the Rungan River.
2.2 Field measurements
Field surveys of GEM concentrations were conducted from 21 to 26 December 2017 in the city of Palu, from 6 to 8 March 2011 in the village of Muara Aman, and in July and August 2007,35, January and February 2008, and January, August, September, November, and December 2009 in central Kalimantan (Fig. SI1†).
In ASGM areas, we usually did not have access to electricity or gas, and it was therefore difficult to conduct fixed point monitoring at a site. Because ASGM activities are usually illegal, areas associated with ASGM are sometimes unsafe. We therefore had to be mindful of the safety of the researchers who were conducting the field surveys. In this case, we used four mobile type survey instruments (Fig. 2): (a) a portable, handheld mercury analyser; (b) a high-resolution, portable, handheld mercury analyser; (c) a gold trap method; and (d) a passive sampling method.
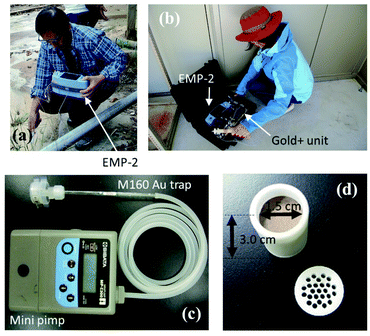 |
| Fig. 2 Atmospheric mercury measured by using four methods: (a) portable handheld mercury analysing method, (b) high-resolution portable handheld mercury analysing method, (c) gold trap method, and (d) passive sampling method. | |
2.2.1 Portable, handheld mercury analyser.
We used a portable handheld mercury analyser (Cold Vapor Atomic Absorption Spectroscopy CVAAS, EMP-2, Nippon Instruments Co., Ltd. Osaka, Japan) to measure high concentrations of GEM (Fig. 2(a)). The device internally computes an average concentration from values obtained at a sampling frequency of 1 s−1. The coefficient of variation was less than 10% at a GEM of 400 ng m−3. The measurement range of the handheld mercury analyser was from <100 to 999
000 ng m−3. This method was used mainly for GEM measurements at gold-processing sites and gold-shop sites. Also, because the instrument was portable, we could measure GEM concentrations as we walked (Fig. 3). The equipment was calibrated by the manufacturer prior to use.
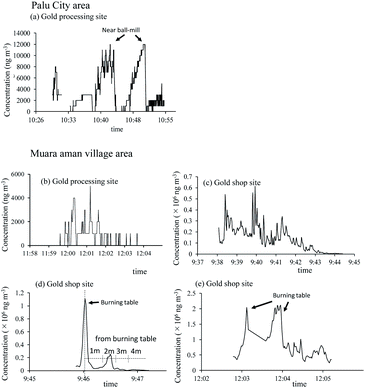 |
| Fig. 3 Fluctuation of GEM concentrations at ASGM sites. Gold shop and gold processing sites. (a) GEM concentration of a gold processing site using the ball mill method in Poboya near Palu (St. 4, Fig. SI1†); (b) GEM concentration of a gold processing site using the ball mill method in Muara Aman; (c–e) Inside Muara Aman gold shops6 and for (c), GEM concentrations when gold amalgam was not being burned. | |
2.2.2 High-resolution portable handheld mercury analysing method.
The high-resolution portable handheld mercury analyser used the EMP-2 with a Gold+ unit (Fig. 2(b)). This portable, handheld, mercury analyser (CVAAS EMP-2 Gold+, Nippon Instruments) could detect concentrations as low in 10–20 min at a flow rate of 0.5 L min−1. Because this analyser could measure low GEM concentrations, we used it to measure GEM concentrations at ASGM community sites and central city sites. We were able to place this analyser on a car and measure GEM concentrations while driving (Fig. SI3(a) and (b)†). We could also put this analyser near, for example, a ball mill operation area (Fig. SI3(c)†).
2.2.3 Gold trap method.
We used the gold trap method6,7,37–40 to determine 12 h to 24 h average atmospheric mercury concentrations (Fig. 2(c)). The device consisted of M-160 Au traps (Nippon Instruments) made with gold-coated diatomaceous earth packed in a quartz tube. GEM was collected using gold traps via gold–mercury amalgamation. GEM in ambient air was sampled for 12–24 h at a flow rate of 0.5 L per minute with a mini-pump connected to an M-160 Au trap. GEM absorbed on the M-160 Au traps was quantified with a mercury analyser (model MA-2000, Nippon Instruments). In the case of high GEM concentrations in the ambient air, the sampling time of about 10 min was sufficient to maintain the accuracy of the analysis. However, this equipment could measure GEM concentrations over a broad range from low to high. The Au trap was able to absorb atmospheric mercury from ∼0 pg to >70
000 pg. In this study, we used this equipment at gold processing sites, ASGM community sites, and central city sites (Fig. SI3(e) and (f)†). The instruments were calibrated by the manufacturer prior to use.
2.2.4 Passive sampling method.
The passive sampling method37–39 was used to determine 24 h average atmospheric mercury concentrations (Fig. 2(d)). The sampler had two independent cavities (inner diameter, 1.4 cm), each of which contained a diffusion bar sampler end cap and filter. The GEM sampling filter was a 15 mm φ quartz filter (Tokyo Dylec Co., Tokyo, Japan) that was coated with gold by means of a vacuum-deposition process. To prevent the filters from absorbing stray GEM from the atmosphere, we stored them in a vacuum desiccator until use. Before each use, the filters were heated for 30 min at 850 °C in a muffle furnace to completely remove any contaminating GEM. Whether we used this passive sampling equipment depended on the circumstances at the observation site, such as the expected GEM concentration range and whether we were able to obtain permission from the miners and residents to install the equipments. We used this equipment at community sites (Fig. SI3(d)†) to characterize the spatial distribution of GEM in the air. After sampling was conducted by the gold trap method and passive sampling method, we analysed the samples via heat-vaporization atomic absorption spectrometry (model MA-2000, Nippon Instruments).
2.3 Quality assurance/quality control
Quality assurance/quality control was carried out by analysing standard reference materials obtained from the National Institute of Standards and Technology: trace elements in pine needles (Pinus taeda) 1575a and reference material no. 482 trace elements in lichens. The percentage recoveries for Hg were 89.3% and 91.4%, respectively, of the reference material values.
2.4 Human health risk assessment method
The human health risk associated with the GEM concentrations measured at the survey sites was evaluated based on the Hazard Quotient (HQ),41–43 which was equated to the ratio of the average inhalation concentration to the reference concentration as follows: |  | (1) |
where HQinh is the hazard quotient for contaminated air via inhalation, EC is the average exposure concentration (ng m−3), and RfC is the reference concentration (ng m−3).
The potential for effects other than cancer to occur was assessed by comparing the exposure concentration or average concentration during inhalation of GEM with the corresponding reference concentration (RfC). The RfC (mg m−3 in ambient air) was considered to be a safe threshold for the general population. The non-carcinogenic health risk was expressed in terms of the HQ for GEM.41 In this study, the reference concentration associated with chronic exposure via inhalation was set to the general atmospheric value of 300 ng m−3 as elemental mercury, as reported in the Integrated Risk Information System of the U.S. Environmental Protection Agency.44 That publication defines a critical effect as a hand tremor, increase in memory disturbance, or slight subjective or objective evidence of autonomic dysfunction.
The average daily exposure concentration for a person who lives in the same place for a whole year was calculated as follows:
| 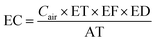 | (2) |
where EC (μg m
−3) = exposure concentration;
Cair (μg m
−3) = contaminant concentration in air; ET (hours per day) = exposure time; EF (days per year) = exposure frequency; ED (years) = exposure duration; and AT (ED in years × 365 days per year × 24 hours per day) = averaging time (hours).
However, when a person stayed at several places, the average EC for the period of residency was calculated using a Cair value that was an average of the mercury concentrations in the air of each microenvironment (ME) weighted by the amount of time spent in each ME:
|  | (3) |
where EC
j (μg m
−3) is the average exposure concentration for the period of exposure
j;
Cair j (μg m
−3) is the contaminant concentration in air in ME
i; ET
i (hours per day) is the exposure time spent in ME
i; EF
i (days per year) is the exposure frequency for ME
i; ED
j (years) is the exposure duration for exposure period
j; and AT
j (hours) is the averaging time and equals ED
j × 24 hours per day × 365 days per year.
2.5 Monte Carlo simulations
Because the parameters that described the GEM concentrations in the study areas were not constant, we performed a Monte Carlo simulation to determine the confidence limits of the calculated GEM concentrations and the associated risks. To perform the probabilistic risk assessment, we assumed a log-normal distribution for each parameter. Using the average and standard deviation (SD) of the log-normal distribution of the dataset for each parameter, we conducted random Monte Carlo simulations of the GEM concentrations at the gold processing sites, gold shop sites, ASGM community site, and central city site. Random Monte Carlo simulations were performed with Crystal Ball software (Oracle Co., KKE Inc., Japan, Tokyo). We used a Latin square sampling method with 10
000 iterations to define the probabilistic distribution.45 The 95th percentile value was generally used as the reasonable maximum exposure value, and we used that value in this study for risk assessment.
3. Results and discussion
3.1 GEM concentrations in study areas
3.1.1 City of Palu.
On the roadside in the ASGM area within the city of Palu, the GEM concentrations tended to be high (St. 5; Fig. SI3(a)†). The GEM concentrations near a ball mill varied from <100 to 14
000 ng m−3 (Fig. 3(a)). Because a refinery with a ball mill is often operated in a hut with only a simple roof, the GEM emitted from the ball mill can easily be dispersed over a wide area, and the concentrations vary greatly. GEM concentrations along roadsides in the areas where ball mill refineries operated were as high as 5500 ng m−3 (Fig. SI3(a)†). At Poboya, the ASGM community site near the city of Palu (St. 5 in Fig. 1), many ball mills operated side by side, and there were small communities and city residents nearby. At the site of the ASGM community, there were more than 10 gold shops on one street. In front of the shops, children were playing, as shown in Fig. SI2(b).† Children at the ASGM community site may have been at risk from exposure to high GEM concentrations.
The GEM concentration at St. 3 (city of Palu) ranged between 2.05 and 376 ng m−3 (mean ± SD = 155 ± 152 ng m−3, n = 18; Fig. SI3(c)†). The GEM concentrations that we measured while driving along the shore from St. 1 to St. 3 were quite variable, but we could not detect a trend in the concentrations (Fig. SI3(b)†). During 2010–2012 (ref. 7) and even in 2017, ASGM activity continued in Poboya. The daily average concentration observed during 2010–2012 in this area was 524 ± 420 ng m−3.7 We observed GEM concentrations during the daytime in this study. The direction of the wind in Palu is determined by the strengths of the sea breeze and mountain breeze. GEM concentrations would probably tend to be relatively low in the daytime,7 when the sea breeze is dominant. The concentrations observed in this study may therefore have been lower than the average daily concentration.
3.1.2 Village of Muara Aman.
GEM concentrations in the two gold shops (St. 10 and St. 11) in Muara Aman fluctuated greatly (Fig. 3(c)–(e)). The highest concentration of 6.13 × 105 ng m−3 was measured around the burning table when amalgam was not being burned (Fig. 3(c)). The concentration was 2.12 × 106 ng m−3 when an acetylene torch was being used to heat the mercury–gold amalgam (Fig. 1(b)). The measured concentration was 1.11 × 106 ng m−3 above the bowl and 2.19 × 105 ng m−3 near the burning table (Fig. 3(d)). At 1, 2, and 3 m away from the burning table, the concentration decreased to 0.80–2.19 × 105 ng m−3, 1.50–2.53 × 105 ng m−3, and 0.01–1.11 × 105 ng m−3, respectively (Fig. 3(d)). At 4 m away from the burning table, the concentration dramatically decreased to below the limit of detection of the portable, handheld mercury analyser.
These measurements indicate that the health risk was high for employees of the two gold shops, each of which was only about 25 m2 in area. The employees were working without any protective device. Because of various limitations, measurements could not be made at gold shops in Palu and Palangka Raya. However, we confirmed that amalgam was burned indoors or in front of a store using a drum can as a chimney.
A family of gold smelters lived next to the ball mill operation. Similarly, the families of some gold shop owners lived in the shops and may have been exposed to high concentrations of GEM. In the area of Muara Aman where there was no ASGM activity, GEM concentrations ranged from 4.10 to 11.5 ng m−3 (mean ± SD = 6.84 ± 2.72 ng m−3; Fig. SI3(d)†).
3.1.3 City of Palangka Raya.
Because there is much gold dredging activity in the basins of the Kahayan River and Rungan River upstream of Palangka Raya, both river water and sediments may have been contaminated by mercury.35 ASGM activity may also have contaminated the air along both rivers.
The GEM concentrations measured by the gold trap method on a boat in the Rungan River were 122 and 1470 ng m−3 (Fig. SI3(f),† St. 6 and 7). There were more dredging operations in the Kahayan River basin than in the Rungan River basin. GEM was also detectable in the air above the mainstream of the Kahayan River. The wind along the river usually blows from upstream to downstream from midnight to early in the morning. Therefore, a plume of air containing high concentrations of GEM due to upstream ASGM activity may flow downstream to Palangka Raya. This possibility raises concerns about the human health risk associated with inhalation of GEM. We therefore established two observation sites in the center of Palangka Raya (St. 8 and St. 9).
The GEM concentrations at St. 8 and St. 9 ranged from 2.04 to 25.3 ng m−3 (mean ± SD = 6.65 ± 5.41 ng m−3) and 8.90 to 167 ng m−3 (mean ± SD = 44.3 ± 68.8 ng m−3), respectively, with the exception of one anomalous measurement of 167 ng m−3 at St. 9 (Fig. SI3(e)†). At St. 8, GEM concentrations were 1.85–19.5 times the background level of 1.10–1.30 ng m−3 in an uncontaminated area of the Southern Hemisphere.50 We observed high GEM concentrations, regardless of the method and scale of ASGM activity.
3.2 Parameterization of probabilistic distribution for input variables
Parameterization of the exposure assessment model involved the selection of a dataset and derivation of the probability density function for the input variables. In eqn (2) and (3), the concentration of GEM in the air (Cair) is the critical input variable for assessing the average daily exposure concentration (EC). The datasets for exposure estimation were obtained from our field surveys. Table 1 lists the GEM concentrations at the gold processing sites, gold shop site, ASGM community sites, and three ASGM areas in central city sites. Each EC value was estimated from a deterministic equation or log-normal probabilistic distribution function. The Hg concentrations at gold processing facilities and gold shops were measured over a time interval of one second with a portable handheld mercury analyser. We therefore averaged the values and reported them as deterministic values. For Hg concentrations in community areas and central city areas, we estimated probabilistic values. Before calculating the EC values, we applied a Shapiro–Wilk test to confirm that the log-transformed GEM atmospheric concentrations were normally distributed (i.e. that the original datasets followed a log-normal distribution). We log-transformed the GEM data and then applied the Shapiro–Wilk test to the dataset. The hypothesis of normality was rejected. However, the dataset of GEM concentrations that were obtained in 2010–2012 in Palu (n = 60)7 were judged to be normally distributed. Concentrations in environmental media are usually log-normally distributed after dilution.46 In general, a log-normal distribution is empirically useful for risk assessment because it cannot take negative values and should fit the data better than a normal distribution.47
Based on this logic, we therefore assumed that the dataset was log-normally distributed.
3.3 Framework of risk analysis
Based on a preliminary survey, we categorized the people who worked in areas surrounding ASGM operations into four groups. Workers who engaged in gold processing (group 1) and gold shop employees (group 2) may have been directly exposed to GEM during their work. In many cases, ASGM activity was conducted at a residential site, where residents who lived near sites of ASGM activity (group 3) may have been exposed to GEM. In places like Palu and Palangka Raya, there were very large urban sites next to ASGM operations, and people who lived in such urban areas were defined as Group 4. To estimate human health risks, we used the measured atmospheric GEM concentrations and the results of informal interviews. Based on this information, the distribution of each parameter (Cair and ET in eqn (1)–(3)) for risk analysis was determined.
As discussed in Section 3.2, the average daily concentration was used for the value of the exposure concentration. Because the concentrations observed at the gold processing site (Fig. 3(a) and (b)) and gold shop site (Fig. 3(c)–(e)) were instantaneous values, we averaged all the data and defined the average to be the average daily exposure concentration (EC). We monitored the ASGM community site (Fig. SI3(a) and (d)†) in a spatially uniform manner. The gold processing operation continued for 24 h throughout the day. The spatial distribution of GEM that we documented may therefore apply to the entire ASGM community area. Although the GEM concentration that we measured was a 15 min average value, we assumed that this value was the average daily exposure concentration (EC). Likewise, at the ASGM community site, the GEM concentration observed at the central city site (Fig. SI3(b) and (e)†) was used as the average daily exposure concentration (EC). Table 1 provides details about the GEM concentration dataset and exposure time data used for the risk assessment.
To calculate exposure times (ETs), we assumed that workers in gold processing (group 1) stayed 24 h per day in the same place (gold processing site). Because a gold processing operation is a family-run business, the workplace is located next to the living space. Gold processing usually continues for 24 h per day. The climate of Indonesia is characterized by a rainy season and a dry season, and because the temperature and humidity are always high, housing in this area is well ventilated. Also, residents of the ASGM community as well as residents of the city area were assumed to stay in the same place 24 h per day. The workers in the gold shop (group 2) were assumed to work 8 h in the gold shop and to return to their homes in the city area for 16 h per day. Table 2 provides details of assumed exposure times. Interview surveys must generally be conducted by appropriate methods and require appropriate samples. However, in this study, we could not obtain an appropriate number of samples for interviews. To conduct pre-screening risk assessment, we set ET values based on information from the residents.
Table 2 Exposure time used for human health risk estimation
ET: (hours per day) |
Person |
Group |
Typea |
Place: hours per day |
D deterministic value.
|
Palu city area
|
Worker in gold processing |
1 |
D
|
Gold processing: 24 |
Worker in gold processing |
2 |
— |
— |
Residents in community |
3 |
D
|
Community area: 24 |
Residents in central city area |
4 |
D
|
City area: 24 |
![[thin space (1/6-em)]](https://www.rsc.org/images/entities/char_2009.gif) |
Muara Aman village area
|
Worker in gold processing |
1 |
D
|
Gold processing: 24 |
Worker in gold processing |
2 |
D
|
Gold shop: 8 |
City area: 16 |
Residents in community |
3 |
D
|
Community area: 24 |
Residents in central city area |
4 |
— |
— |
![[thin space (1/6-em)]](https://www.rsc.org/images/entities/char_2009.gif) |
Palanka Raya city area
|
Worker in gold processing |
1 |
— |
— |
Worker in gold processing |
2 |
— |
— |
Residents in community |
3 |
— |
— |
Residents in central city area |
4 |
D
|
City area: 24 |
3.4 Assessment of the human health risk from inhalation of mercury
The results of our assessment of human health risk due to exposure to GEM via inhalation are shown in Fig. 4 and Table 3. The HQinh (the 95th percentile of the probabilistic value or the deterministic value) for groups 1, 3, and 4 in Palu were 7.82, 4.93, and 1.67, respectively. The HQinh for groups 1 and 2 in Muara Aman were 4.33 and 197. All of these groups were at risk of experiencing health effects from GEM inhalation.
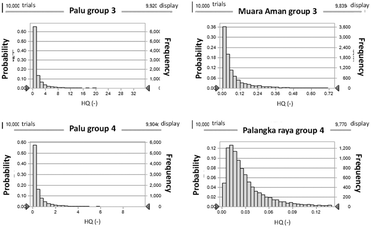 |
| Fig. 4 Estimation of human health risk caused from GEM inhalation exposure. In the figure, extremely high values were excluded, but about 99% of predicted values are included. | |
Table 3 Summary of the risk analysis
Site |
Group |
HQ |
Risk concern |
The HQ value is the hazard quotient when the probabilistic distribution function is adopted for the gaseous elemental mercury concentrations. In such cases, the 95 percentile value of HQ is shown.
|
Palu |
1 |
7.82 |
Yes |
2 |
— |
Unknown |
3 |
4.93a |
Yes |
4 |
1.67a |
Yes |
Muara Aman |
1 |
4.33 |
Yes |
2 |
197 |
Yes |
3 |
0.24a |
No |
4 |
— |
— |
Paranka Raya |
1 |
— |
Unknown |
2 |
— |
Unknown |
3 |
— |
Unknown |
4 |
0.07a |
No |
For group 1, the differences between the gold shop sites in the Palu and Muara Aman areas were the size and number of ball mills. The ball mill method is used at both of these sites, but the ball mill that is used in the Muara Aman area is smaller than that in the Poboya area of Palu. The difference of the size of the ball mill is related to the amount of mercury that is used. Usually, Regardless of ball mill size, people in group 1 at both sites were at risk. Most miners believe that the more mercury they add to the mixture, either in the ball mill or during panning, the more gold they will recover. In reality, that is not the case.48 Although we did not gather data on the amount of mercury being used at each site, there is a need for appropriate amounts of mercury to be used. For group 2, we could observe only the Muara Aman area, where there was a concern about human health risk. In this paper, we estimated the human health risk of chronic inhalation of GEM. The United States (US) Occupational Safety and Health Administration (OSHA) has promulgated a permissible exposure limit (PEL) of 0.10 mg m−3 as total mercury.50 Some of our measured concentrations were higher than the US OSHA PEL. In addition, OSHA recognizes that many of its PELs are outdated and inadequate for ensuring protection of worker health. Most of OSHA's PELs were issued shortly after adoption of the Occupational Safety and Health Act in 1970 and have not been updated since that time.51 The concentration of mercury in the air that is fatal to humans has not been reported, but for animals (dog) the fatal concentration has been reported to be 15–20 × 106 ng m−3.49 The threshold value for an acceptable GEM concentration is 0.05 × 106 ng m−1,3 but mercury-induced acute pneumonia occurs when a person is exposed to a concentration of 1–2 × 106 ng m−3 for several hours.49 The highest concentration measured inside a gold shop was equal to the concentration that induces pneumonia. There should also be concern about the acute toxicity of the GEM in gold shops to workers.
For group 3, a human health risk was noted in the Palu area (Poboya) but not in the Muara Aman area. This difference may reflect the difference in the number of ball mill operations in each area, because the ASGM area is more extensive in Poboya than in Muara Aman. For group 2, regardless of ball mill size or number of ball mills, there were concerns about human health risks, and for group 3, who live in areas of very high ASGM activity (Poboya, Palu area), there were concerns about human health risks.
For group 4, there was concern about human health risks only in Palu. Although there were no concerns about human health for group 4 in Palangka Raya, the observed GEM concentrations there ranged from 2.04 to 167 ng m−3, and those concentrations were 1.85 to 152 times the background level of 1.10–1.30 ng m−3.43 There is much ASGM dredging activity conducted in the Kahayan and Rungan River basins just upstream of Palangka Raya. We measured GEM concentrations in the center of Palangka Raya, and we assumed that the dataset represented the GEM concentrations in the center of Palangka Raya. In this study, we could not observe the seasonal variations that would be revealed by a more extensive study. Immediate measures should be undertaken to reduce GEM concentrations in these areas.
GEM concentrations should continue to be monitored to lessen the human health risk from GEM inhalation in urban areas. Even in the urban area of Palu, human health may be at risk. The residents who lived in Palu may have been at risk in 2012,7 and our findings revealed that this risk has persisted to the present day (2021).
Some of the datasets we have reported were collected as long ago as 2007 and 2011. Satellite photographs,52 however, have indicated that ASGM activity still continues and is expanding around Poboya, and ASGM activity is still continuing in the Palangka Raya area.27 Although the Minamata convention on mercury became effective in August 2017, these facts indicate the difficulty of reducing ASGM activities. Our survey was conducted in the Palu area in 2017, where people have used the same method for ASGM since we first visited Palu in 2010. Such observations indicate that GEM emissions to the atmosphere due to ASGM may be increasing.
4. Conclusions
GEM pollution is associated with all scales and methods of ASGM activity. Our research revealed human health risk via GEM inhalation. At gold shops, gold amalgam is burned using an acetylene torch in a simple draft to obtain crude gold. We observed high GEM concentrations in these shops, and there was reason for concern about the risks to human health in such places. Immediate measures, such as preparing a draft and collecting mercury using a small retort29,53,54 should be taken to minimize the risks to human health from such activity.
Many environmental and social problems have been reported at sites of large-scale ASGM activity.17,19,23,55–57 In developing countries, ASGM activity occurs at various scales. Large ASGM operations can be found easily, but small, family-operated ASGM facilities are not obvious, and the associated human health problem is thus easily overlooked. Unfortunately, it is difficult to stop ASGM activity in impoverished regions. Improved mercury recovery systems and environmental education programs will be needed to reduce the human health risk associated with these operations.57
Authors contributions
Koyomi Nakazawa: conceptualization, field measurement, sample analysis, data analysis, writing, Osamu Nagafuchi: conceptualization, field measurement, sample analysis, Tomonori Kawakami: field measurement, data analysis, Takanobu Inoue: field measurement, writing, Rosana Elvince: field measurement, survey arrangement, Koji Kanefuji: data analysis, Isrun Nur: field measurement, Mery Napitululu: survey arrangement, Muhammad Basir-Cyio: survey arrangement, Hazumu Kinoshita: field measurement, sample analysis, Ken'ichi Shinozuka: data analysis.
Conflicts of interest
The authors declare no conflicts of interest in this research.
Acknowledgements
The authors wish to thank Dr Hery Suhartoyo, Dr Faiz Bachia, and the students in Bengkulu University, Indonesia. Also, we would like to thank Nippon Instruments., Co. Ltd., Japan, for use of the EMP-2. We also thank the following foundations for their support: Environmental Research and Technology Development Fund of Japan (B-1008; 2010–2012, JPMEERF20205R03; 2020–2022); Mitsui Co., Ltd Environmental fund (grant No. 07-290) (2008–2010); Japan Society for the Promotion of Science KAKENHI (Grants 224013, 2051007 and 26257301); Steel Foundation for Environmental Protection Technology; and The Institute of Statistical Mathematics (H30-2-2058, H28-J-4308, H29-J-4110).
References
-
UNEP Global Mercury Assessment, 2018 Search PubMed.
- C. Salazar-Camacho, M. Salas-Moreno, S. Marrugo-Madrid, J. José Marrugo-Negrete and S. Díez, Dietary human exposure to mercury in two artisanal small-scale gold mining communities of northwestern Colombia, Environ. Int., 2017, 107, 47–54 CrossRef CAS PubMed.
- M. M. Sari, T. Inoue, Y. Matsumoto and K. Yokota, Relating food and human hair to assess mercury exposure levels in Poboya, Central Sulawesi, Indonesia, Int. J. Environ. Sci. Technol., 2017, 14, 463–472 CrossRef CAS.
- D. S. McLagan, D. P. J. Michell, H. Huang, Y. D. Lei, A. S. Cole, A. Steffen, H. Hung and F. Wania, A High precision passive air for gaseous mercury, Environ. Sci. Technol. Lett., 2016, 3, 24–29 CrossRef CAS.
- P. Cordy, M. Veiga, L. Bernaudat and O. Gracia, Successful airborne mercury reductions in Colombia, J. Cleaner Prod., 2015, 108, 992–1001 CrossRef CAS.
- O. Nagafuchi, K. Nakazawa, T. Inoue, R. Elvince, T. Kawakami, K. Osaka and O. Kanefuji, Mercury concentration in environmental media and its human health risk observed in Artisanal very Small Gold Mining site, Bengkulu Sumatra, Indonesia, J. Environ. Sci., 2018, 31(2), 43–48 Search PubMed.
- K. Nakazawa, O. Nagafuchi, T. Kawakami, T. Inoue, K. Yokota, Y. Serikawa, B. Cyio and R. Elvince, Human health risk assessment of mercury vapor around artisanal small-scale gold mining area, Palu, Central Sulawesi, Indonesia, Ecotoxicol. Environ. Saf., 2016, 124, 155–162 CrossRef CAS PubMed.
- Y. Serikawa, T. Kawakami, B. Cyio, I. Nur, R. Elvince and T. Inoue, 2011. Emission and dispersion of gaseous mercury from artisanal small-scale gold mining plants in Palu, Environ. Sci., 2011, 24(4), 269–274 Search PubMed.
- V. Conzalez-Carrasco, C. Patricio, P. C. Velasquez-Lopez, J. Olivero-Verbel and N. Pajaro-Castro, Air mercury contamination in the gold mining town of Prtovelo, Ecuador, Bull. Environ. Contam. Toxicol., 2011, 87, 250 CrossRef PubMed.
-
A. M. Kiefer, K. Drrace, S. C. Seney and M. M. Veiga, Challenges associated with using retorts to limit mercury exposure in artisanal and small-scale gold mining: vase studies from Mozambique, Ecuador, and Guyana, Trace Materials in Air, Soil, and Water, 2015, pp. 51–77, ch. 3 Search PubMed.
- T. S. Brown, K. M. Hasan, K. H. Moody, C. LovingD, K. E. Howe, A. G. Dawson, K. Drace, J. D. Hugdahl, C. S. Seney, C. M. Vega, L. E. Fernandez and A. M. Kiefer, Method for mapping Hg0 emissions from gold shops in artisanal and small-scale gold mining communities, Methods X, 2020, 7, 101060 Search PubMed.
- T. S. Brown, L. L. Bandoo, S. S. Agard, S. T. Thom, T. E. Gilhuys, G. K. Mudireddy, A. V. Eechampati, K. M. Hasan, D. C. Loving and C. S. Seney, A collaborative training program to assess mercury pollution from gold shops in Guyana's artisanal and small-scale gold mining sector, Atmosphere, 2020, 11, 719 CrossRef.
- K. H. Moody, K. M. Hasan, S. Aljic, V. M. Blakeman, L. P. Hicks, D. C. Loving, M. E. Moore, B. S. Hammett, M. Silva-Gonzalez, C. S. Seney and M. A. Kiefer, Mercury emissions from Peruvian gold shops: Potential ramifications for Minamata compliance in artisanal and small-scale gold mining communities, Environ. Res., 2020, 182, 109042 CrossRef CAS PubMed.
- T. Kawakami, M. Konishi, Y. Imai and S. P. Soe, Diffusion of mercury from artisanal small-scale gold mining sites in Myanmar, Int. J. GEOMATE, 2019, 17(61), 228–235 Search PubMed.
- B. Niane, S. Guédron, F. Feder, S. Legros, P. K. Ngom and R. Moritz, Impact of recent artisanal small-scale gold mining in Senegal: Mercury and methylmercury contamination of terrestrial and aquatic ecosystems, Sci. Total Environ., 2019, 669(15), 185–193 CrossRef CAS PubMed.
- T. Tomiyasu, Y. K. Hamada, H. Kodamatani, N. Hidayati and J. S. Rahajoe, Transport of mercury species by river from artisanal and small-scale gold mining in West Java, Indonesia, Environ. Sci. Pollut. Res., 2019, 26(24), 25262–25274, DOI:10.1007/s11356-019-05718-6.
- P. Cordy, M. M. Veiga, I. Salih, S. Al-Saadi, S. Console, O. G. Luis Alberto Mesa, P. C. Velásquez-López and M. Roeser, Mercury contamination from artisanal gold mining in Antioquia, Colombia: The world's highest per capita mercury pollution, Sci. Total Environ., 2011, 410–411, 154–160 CrossRef CAS PubMed.
- S. Bose-O’Reilly, B. Lettmeier, G. Roider, U. Siebert and G. Drasch, Mercury in breast milk – A health hazard for infants in gold mining areas?, Int. J. Hyg. Environ. Health, 2008, 211, 615–623 CrossRef PubMed.
- Z. Castilhos, S. Rodrigues-Filho, A. P. C. Rodrigues, R. C. Villas-Boas, S. Siegel, M. M. Veiga and C. Beinhoff, Mercury contamination in fish from gold mining areas in Indonesia and human health risk assessment, Sci. Total Environ., 2006, 368(1), 320–325 CrossRef CAS PubMed.
- Z. Castilhos, S. Rodrigues-Filho, R. Cesar, A. Paula Rodrigues, R. Villas-Bôas, I. de Jesus, M. Lima, K. Faial, A. Miranda, E. Brabo, C. Beinhoff and E. Santos, Human exposure and risk assessment associated with mercury contamination in artisanal gold mining areas in the Brazilian Amazon, Environ. Sci. Pollut. Res., 2015, 22, 11255–11264 CrossRef CAS PubMed.
- Y. M. Arifin, M. Sakakibara and K. Sera, Impacts of Artisanal and Small-Scale Gold Mining (ASGM) on Environment and Human Health of Gorontalo Utara Regency, Gorontalo Province, Indonesia, Geosciences, 2015, 5, 160–176 CrossRef CAS.
-
Goldprice homepage, accessed 2019.9.17, https://goldprice.org/.
- U. Arroyo-Abed, M. Pfeifer, S. Monthes, H. Stark, C. Piechotta, J. Mattusch and T. Reemtsma, Determination of moderately polar arsenolipids and mercury speciation in freshwater fish of the river elbe (Saxony, Germany), Environ. Pollut., 2016, 208, 458–466 CrossRef PubMed.
- M. Casey, Toxic gold: Using mercury in Third World countries to mine gold is fast and cheap, but poses a real threat, The Sault Star January, 2009, 10 Search PubMed.
- A. J. Reichelt-Brushett, P. Stone, B. Howe, M. Thomas, Y. Clark, A. N. Male and P. Butcher, Geochemistry and mercury contamination inreceiveing environments of artisanal mining wasters and identified concerns for food safety, Environ. Res., 2017, 152, 407–418 CrossRef CAS PubMed.
- J. Seccatore, M. Veiga, C. Origliasso, T. Marin and D. G. Tomi, An estimation of the artisanal small-scale production of gold in the world, Sci. Total Environ., 2014, 496, 662–667 CrossRef CAS PubMed.
-
UNITAR UNITAR's Operational Satellite Applications Programme, Satellite Mapping of Artisanal and Small Scale Gold Mining in Central Kalimantan, Indonesia, 2016 Search PubMed.
- Minamata convention on mercury < Minamata Convention on Mercury > Home (https://www.mercuryconvention.org)> (accessed 2021-4-14).
-
UNEP A Practical Guide Reducing Mercury Use in Artisanal and Small-Scale Gold Mining. 2012 Search PubMed.
-
Balifokus, Rapid Assessment of the Socio-Economic Impact and Human Rights Aspect of Mercury Use in Artisanal and Small-Scale Gold Mining Hot Spots in Indonesia, 2012 Search PubMed.
-
Balifokus, Mercury Hotspots in Indonesia ASGM Sites: Poboya and Sekotong in Indonesia, IPEN mercury-free campaign report, 2013 Search PubMed.
-
Anton, 2008 Muara Aman, The Gold and Historical City, https://callhavid.wordpress.com/my-hometown-2/muara-aman-the-gold-and-historical-city/, accessed 2019/11/3 Search PubMed.
-
Census Data of Lebong Regency, Sumatra, Indonesia, 2014 Search PubMed.
-
T. Leeuwen, A Brief History of Mineral Exploration and Mining in Sumatra, Proceeding of Sundland Resources, 2014 Mgei Annual Convention, 17-18 Novenber 2014, Palembang, South Sumatra, Indonesia, 2014 Search PubMed.
-
T. Yamada, T. Inoue, S. Dohong, and U. Darung, Mercury contamination in river water and sediment in Central Kalimantan, Indonesia, Annual Report for April 2004 –March 2005 on Environmental Conservation and Land Use Management of Wetland Ecosystems in Southeast Asia, Core University Program between Hokkaido University, Japan, and Research Center for Biology, LIPI, Indonesia. Sponsored by japan Society for Promotion of science, 2005, pp. 115–120 Search PubMed.
-
A. Mori, H. Tachibana and H. TakahashiDeposition of Wash Load Observed in Kahayan and Rungan Rivers. Annuual Report for April 2003-March 2004 on Environmental Conservation and Land Use Management of Wetland Ecosystems in Southeast Asia, Core University Program between Hokkaido University, Japan, and Research Center for Biology, Lipi, Indonesia. Sponsored by Japan Society for Promotion of Science, 2004, pp. 109–117 Search PubMed.
- H. Kinoshita, O. Nagafuchi, K. Nakazawa and K. Yokota, Relationship between origin of atmospheric mercury and pathway of air mass observed at free troposphere-observation on Mt. Fuji, J. Environ. Sci., 2016, 29(6), 275–282 Search PubMed.
- R. Kuniki, T. Kawakami, S. Kagaya, T. Inoue, E. Rosana and O. Nagafuchi, Measurements of gaseous mercury concentration in the atmosphere, Environ. Eng. Research, 2009, 46, 355–359 Search PubMed.
- T. Matsui, N. Harai, K. Saitho, K. Koyomi and O. Nagafuchi, Measurement of gaseous elemental mercury concentration by passive sampler, J. Environ. Conserv. Eng., 2017, 46, 208–214 CrossRef CAS.
- A. Yamakawa, A. Takami, Y. Takeda, S. Kato and Y. Kajii, Emerging investigator series: investigation of mercury emission sources using Hg isotopic compositions of atmospheric mercury at the Cape Hedo Atmosphere and Aerosol Monitoring Station (CHAAMS), Japan, Environ. Sci.: Processes Impacts, 2019, 21, 809–818 RSC.
-
Risk Assessment and Management Handbook for Environmental, Health, and Safety Professionals, ed. V. K. Kolluru, chapter 4 Health risk assessment: principles and practices, McFraw-Hill Inc., New York, 1996, pp. 4.1-4.68 Search PubMed.
-
US EPA, Risk Assessment Guidance for Superfund Volume 1 Human Health Evaluation Manual (Part A), United States Environmental Protection Agency, 1989, EPA/540/1-89/002, http://www.epa.gov/oswer/riskassessment/ragsa/pdf/preface.pdf, accessed 2016-12-15 Search PubMed.
-
US EPA, Risk Assessment Guidance for Superfund (RAGS), Volume I: Human Health Evaluation Manual (Part F, Supplemental Guidance for Inhalation Risk Assessment), United States Environmental Protection Agency, 2010, http://www/oswer/riskassessment/ragsf/index.htm, accessed 2016-12-15 Search PubMed.
-
US EPA, Integrated Risk Information System, IRIS, 2016, https://www.epa.gov/iris, accessed 2016-12-15 Search PubMed.
-
US EPA, Guiding Principles for Monte Carlo Analysis, United States Environmental Protection Agency, 2014, EPA/630/R-97/001, https://www.epa.gov/sites/production/files/2014-11/documents/montecar.pdf, accessed 2016-12-15 Search PubMed.
-
A. C. Cullen and H. C. Frey, Probabilistic Techniques in Exposure Assessment a Handbook for Dealing with Variability and Uncertainty in Models and Inputs, Plenum Press, 1999 Search PubMed.
-
D. M. Kammen and D. M. Hassenzahl, Should We Risk it?, Princeton University Press, Princeton, New Jersey, 1999 Search PubMed.
-
BaliFokus Foundation, Illegal and illicit mercury trade in Indonesia, 2016, available at https://docs.wixstatic.com/ugd/13eb5b_f0dd64cbceda413e8c3dbb3943e1975f.pdf Search PubMed.
- S. Asano, K. Eto, E. Kurisaki, H. Gunji, K. Hiraiwa and M. Sato, Acute inorganic mercury vapor inhalation poisoning, Pathol. Int., 2000, 50, 169–174 CrossRef CAS PubMed.
- United States Department of Labour Occupational Safety and Health Administration Permissible Exposure Limits – Annotated Tables Permissible Exposure Limits – Annotated Tables|Occupational Safety and Health Administration (osha.gov) accessed 2021/5/1.
-
United States Department of Labour Occupational Safety and Health Administration, MERCURY VAPOR IN WORKPLACE ATMOSPHERES, https://www.osha.gov/dts/sltc/methods/inorganic/id140/id140.pdf, accessed 2021/5/1 Search PubMed.
- Google earth pro < Google Earth > accessed 2021/5/1.
- F. Sprovieri, N. Pirrone, R. Ebinghaus, H. Kock and A. Dommergue, A review of worldwide atmospheric mercury measurements, Atmos. Chem. Phys., 2010, 10, 8245–8265 CrossRef CAS.
- L. J. Esdaile and J. M. Chalker, The mercury problem in Artisanal and Small-Scale Gold mining, Chem. - Eur. J., 2018, 24, 6905–6916 CrossRef CAS PubMed.
- N. Basu, E. P. Renne and R. N. Long, An integrated assessment approach to address artisanal and small-scale
gold mining in Ghana, Int. J. Environ. Res. Public Health, 2015, 12(9), 11683–11698 CrossRef PubMed.
- S. J. Turner, P. A. Flindell, D. Hendri, I. Hardjana, P. F. Lauricella, R. P. Lindsay, B. Marpaung and G. P. White, Sediment-hosted gold mineralization in the Ratatotok district, North Sulawesi, Indonesia, J. Geochem. Explor., 1994, 50(1–3), 317–336 CrossRef CAS.
- I. B. Ottenbros, R. Z. Boerleider, B. Jubitan, N. Roelevelad and P. T. J. Scheepers, Knowledge and awareness of health effects related to the use of mercury in artisanal and small-scale gold mining in Suriname, Environ. Int., 2019, 122, 142–150 CrossRef CAS PubMed.
Footnote |
† Electronic supplementary information (ESI) available. See DOI: 10.1039/d0ea00019a |
|
This journal is © The Royal Society of Chemistry 2021 |