DOI:
10.1039/D1CC02097H
(Communication)
Chem. Commun., 2021,
57, 5694-5697
A new mode of cyclobutenedione ring opening for the synthesis of 2-oxobut-3-enamides and tetrasubstituted furans†
Received
20th April 2021
, Accepted 5th May 2021
First published on 5th May 2021
Abstract
A dichotomy between the additions of organolithiums and lithium amides to cyclobutenediones is described wherein the former give carbonyl addition products while the latter induce ring opening by enone cleavage via O- to C-lithium transfer. This distinct mode of ring scission gives access to 2-oxobut-3-enamides and tetrasubstituted furans.
Cyclobutenediones and squarates, e.g.1a, are used extensively as precursors to carbocyclic and heterocyclic ring systems through ring expansion (Scheme 1).1–10 Typically, a carbon nucleophile is first added to give an adduct 2 that rearranges to a product on thermolysis or photolysis. Rearrangements triggered by light usually give rise to 5H-furanones 6via vinylketene (E)-4,9,10 while those triggered by heat typically proceed via the isomeric vinylketene (Z)-4 and give products determined by the nature of the residue introduced at C4. The versatility and reliability of the chemistry is evident from the frequent deployment of such rearrangements in natural products total synthesis.5,8
 |
| Scheme 1 The dichotomous reactivity of dimethyl squarate with organolithiums and lithium amides, and a summary of key transformations. | |
Herein we describe a dichotomy between the additions of organo-lithiums and lithium amides to cyclobutenediones (Scheme 1). Thus, while dimethyl squarate 1a gives cyclobutenones 2 on treatment with organolithum reagents its reactions with lithium amides lead to vinyllithium intermediates 5via the corresponding adduct 3. Herein we show how this unprecedented mode of ring opening, involving scission of the C3–C4 bond with concomitant O- to C-lithium transfer, provides access to an array of 2-oxo-but-3-enamides 7 and tetrasubstituted furans 8.
The discovery was made while preparing a series of 3-amino-4-methoxycyclobutenediones by the addition of amines to dimethyl squarate 1a (Scheme 2).9 Though the method worked well for many substrates, e.g.1a to 9a–c, it returned starting materials when applied to 2°-amines with a high steric burden, e.g.iPr2NH.11 This prompted a switch to using LDA as the nucleophile, but instead of delivering the anticipated 3-aminocyclobutenedione 9d, 2-oxo-but-3-enamide 7a was isolated as the major product in 65% yield. Intrigued by this finding, we decided to explore the generality of the reaction, beginning with extensions to diisopropyl and di-tert-butyl squarates, 1b and 1c. Pleasingly, both gave the corresponding 2-oxo-but-3-enamides, 7b and 7c, albeit more slowly due to the increase in steric demand.
 |
| Scheme 2 The discovery of the C3–C4 mode of ring scission. | |
The reaction was next extended to 2-alkoxy-3-amino-cyclobuten-ones 9. All of the cases examined (Table 1) showed excellent regioselectivity leading to the corresponding vinylogous amides 11 exclusively. This outcome can be attributed to a preferred addition of LDA to the vinylogous ester carbonyl in 9, leading to adduct 10, over its addition to the vinylogous amide carbonyl.
Table 1 Reactions of LDA with aminocyclobutenones together with X-ray structures for 11d [CCDC 2059384] and 11f [CCDC 2042398]
Steric influences on the reaction were next examined with respect to the lithium amide (Scheme 3). Notably, while LDA and lithium piperidide each induced ring opening of cyclobutenedione 9a to 2-oxo-but-3-enamides 11a and 12a respectively, starting material was returned when bulkier lithium amides were employed, e.g. LiHMDS, lithium 2-methylpiperidide and LiTMP. By way of contrast, lowering steric demand with lithium dimethylamide promoted substitution of the alkoxide leading to the bis-aminocyclobutenedione 13b.
 |
| Scheme 3 Influence of steric factors on the addition of lithium amides to cyclobutenedione 9a. | |
Lithium amide additions to dimethyl squarate 1a could also be sequenced with substitution of the terminal alkoxide for NH2 through employment of an ammoniacal work up (Scheme 4).12
 |
| Scheme 4 Substitution of the terminal ether for NH2 with an ammoniacal work-up. | |
Reactions of 3-alkoxy-4-alkylcyclobutenediones 16 with LDA and lithium piperidide were next examined and exposed further subtleties (Scheme 5). Thus, while treatment of the tert-butyl derivative 16a with LDA gave the anticipated oxobutenamide 18a, substitution dominated with lithium piperidide leading to amino-cyclobutenedione 17. Reducing steric demand with the methyl analogue 16b led to cleaner reactions and higher yields, with lithium piperidide giving oxobutenamide 18b in 79% yield. Curiously, alkene reduction to the sensitive cyclobutanedione 19 was observed following exposure to LDA.13
 |
| Scheme 5 Reactions of 3-alkoxy-4-alkylcyclobutenediones with lithium piperidide and LDA. | |
A DFT analysis on the opening of adducts 3a (Fig. 1) and 21 (Fig. 2), to vinyllithium intermediates 5a and 23 respectively, identified low energy pathways for ring scission via transition states 20 and 22.14 From these calculations we infer that the facile O- to C-lithium transfer is due to both a relief of ring strain and the formation of an amide that co-ordinates strongly to the resulting vinyllithium.
 |
| Fig. 1 Summary of DFT calculations on the opening of dimethyl squarate 1a with lithium dimethylamide. | |
 |
| Fig. 2 Summary of DFT calculations on the opening of cyclobutenedione 9a with lithium dimethylamide. | |
The intermediacy of vinyllithium species led us to seek an extension of the chemistry for the synthesis of tetrasubstituted furans. To that end, dimethyl squarate 1a, diisopropyl squarate 1b and cyclobutene-dione 9a were each treated sequentially with LDA then an aldehyde. We presume each reaction proceeded via adduct 24, as the corresponding furans 8 were given in modest yields following an aqueous work-up and purification by column chromatography (Table 2). Notably, these furans each bear three electron-donating substituents making them difficult to access by traditional methods.15
Table 2 Three-component coupling reactions leading to tetrasubstituted furans
Finally, we have identified a single example of the C3–C4 mode of ring scission occurring during an amine addition to a cyclobut-enedione.9 It was observed when 3-isoproxycyclobutenedione 25 was treated with benzylamine, where 2-oxo-but-3-enamide 27 (Fig. 3) was as a significant byproduct formed in 25% yield alongside with the anticipated aminocyclobutenedione 26 (40% yield). To the best of our knowledge, this side reaction has not been reported previously (Scheme 6).
 |
| Fig. 3 X-ray crystal structure of 2-oxo-but-3-enamide 27 [CCDC†1500038]. | |
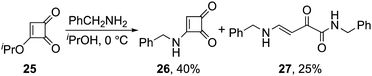 |
| Scheme 6 A related fragmentation of cyclobutenedione 25 triggered with benzylamine. | |
In conclusion, we have uncovered a new mode of cyclobutenedione ring opening that is triggered by lithium amide addition. The reaction proceeds via sequential N- to O- to C-lithium transfer, as detailed in Scheme 1, and gives access to an array of 2-oxo-but-3-enamides (via protonation) and tetrasubstituted furans (via aldehyde addition). We are currently investigating further extensions of the methodology in heterocyclic synthesis.
Dr Wei Sun and Ryan Bennett contributed equally in respect of the experimental work with Dr Mark Light performing the X-ray analyses and Prof. David Harrowven supervising the work.
We gratefully acknowledge financial support from the European Regional Development Fund [ERDF Interreg Va programme (Project 121)] and EPSRC [EP/P013341/1, EP/L003325/1 and EP/K039466/1].
Conflicts of interest
There are no conflicts of interest to declare.
Notes and references
- H. W. Moore and O. H. W. Decker, Chem. Rev., 1986, 86, 821 CrossRef CAS; L. S. Liebeskind, Tetrahedron, 1989, 45, 3053 CrossRef.
- H. W. Moore and S. T. Perri, J. Org. Chem., 1988, 53, 996 CrossRef CAS; J. M. Heerding and H. W. Moore, J. Org. Chem., 1991, 56, 4048 CrossRef; L. S. Liebeskind and J. Zhang, J. Org. Chem., 1991, 56, 6379 CrossRef; L. M. Gayo, M. P. Winters and H. W. Moore, J. Org. Chem., 1992, 57, 6896 CrossRef; D. J. Krysan, A. Gurski and L. S. Liebeskind, J. Am. Chem. Soc., 1992, 114, 1412 CrossRef; P. Turnbull, M. J. Heileman and H. W. Moore, J. Org. Chem., 1996, 61, 2584 CrossRef PubMed; B. Yucel, B. Sanli, H. Soylemez and I. Yilmaz, Tetrahedron, 2011, 67, 1406 CrossRef; E. Packard, D. D. Pascoe, J. Maddaluno, T. P. Goncalves and D. C. Harrowven, Angew. Chem., Int. Ed., 2013, 52, 13076 CrossRef PubMed; Y. Yamamoto, T. Kurohara and M. Shibuya, Chem. Commun., 2015, 51, 16357 RSC; J. T. Hill-Cousins, I.-A. Pop, G. Pileio, G. Stevanato, P. Håkansson, S. S. Roy, M. H. Levitt, L. J. Brown and R. C. D. Brown, Org. Lett., 2015, 17, 2150 CrossRef PubMed.
- L. D. Foland, J. O. Karlsson, S. T. Perri, R. Schwabe, S. L. Xu, S. Patil and H. W. Moore, J. Am. Chem. Soc., 1989, 111, 975 CrossRef CAS; K. Karabelas and H. W. Moore, J. Am. Chem. Soc., 1990, 112, 5372 CrossRef; C. Boullais, C. Rannou, E. Reveillere and C. Mioskowski, Eur. J. Org. Chem., 2000, 723 CrossRef; T. P. Goncalves, M. Mohamed, R. J. Whitby, H. F. Sneddon and D. C. Harrowven, Angew. Chem., Int. Ed., 2015, 54, 4531 CrossRef PubMed; W. Sun, D. C. Wilson, M. E. Light and D. C. Harrowven, Org. Lett., 2018, 20, 4346 CrossRef PubMed.
- L. S. Liebeskind, S. Iyer and C. F. Jewell, J. Org. Chem., 1986, 51, 3065 CrossRef CAS; L. S. Liebeskind and J. Zhang, J. Org. Chem., 1991, 56, 6379 CrossRef; L. S. Liebeskind, K. L. Granberg and J. Zhang, J. Org. Chem., 1992, 57, 4345 CrossRef; F. Liu and L. S. Liebeskind, J. Org. Chem., 1998, 63, 2835 CrossRef; T. Kurohara, J. Jiyue, M. Shibuya and Y. Yamamoto, Synthesis, 2018, 1687 Search PubMed.
- A. G. Birchler, F. Liu and L. S. Liebeskind, J. Org. Chem., 1994, 59, 7737 CrossRef CAS; M. Mohamed, T. P. Goncalves, R. J. Whitby, H. F. Sneddon and D. C. Harrowven, Chem. – Eur. J., 2011, 17, 13698 CrossRef PubMed.
- S. L. Xu and H. W. Moore, J. Org. Chem., 1989, 54, 6018 CrossRef CAS; S. L. Xu, H. Xia and H. W. Moore, J. Org. Chem., 1991, 56, 6094 CrossRef; Y. Yamamoto, M. Ohno and S. Eguchi, Bull. Chem. Soc. Jpn., 1996, 69, 1353 CrossRef; J. M. MacDougall and H. W. Moore, J. Org. Chem., 1999, 64, 7445 CrossRef; S. K. Verma, Q. H. Nguyen, J. M. MacDougall, E. B. Fleischer and H. W. Moore, J. Org. Chem., 2000, 65, 3379 CrossRef PubMed; T. Kurohara, J. Jiyue, M. Shibuya and Y. Yamamoto, Synthesis, 2018, 1687 Search PubMed; M. A. Manning, W. Sun, M. E. Light and D. C. Harrowven, Chem. Commun., 2021, 57, 4556 RSC.
- K. H. Lee and H. W. Moore, J. Org. Chem., 1995, 60, 735 CrossRef CAS; F. Liu and L. S. Liebeskind, J. Org. Chem., 1998, 63, 2835 CrossRef.
- S. T. Perri, H. J. Dyke and H. W. Moore, J. Org. Chem., 1989, 54, 2032 CrossRef CAS; L. D. Foland, O. H. W. Decker and H. W. Moore, J. Am. Chem. Soc., 1989, 111, 989 CrossRef; M. J. Heileman, R. Tiedemann and H. W. Moore, J. Am. Chem. Soc., 1998, 120, 3801 CrossRef; A. Enhsen, K. Karabelas, J. M. Heerding and H. W. Moore, J. Org. Chem., 1990, 55, 1177 CrossRef; S. T. Perri and H. W. Moore, J. Am. Chem. Soc., 1990, 112, 1897 CrossRef; V. J. Santora and H. W. Moore, J. Am. Chem. Soc., 1995, 117, 8486 CrossRef; C. S. Tomooka, H. Liu and H. W. Moore, J. Org. Chem., 1996, 61, 6009 CrossRef; Y. Xiong and H. W. Moore, J. Org. Chem., 1996, 61, 9168 CrossRef; J. M. MacDougall, V. J. Santora, S. K. Verma, P. Turnbull, C. R. Hernandez and H. W. Moore, J. Org. Chem., 1998, 63, 6905 CrossRef PubMed; D. C. Harrowven, D. D. Pascoe, D. Demurtas and H. O. Bourne, Angew. Chem., Int. Ed., 2005, 44, 1221 CrossRef PubMed; D. Knueppel and S. F. Martin, Angew. Chem., Int. Ed., 2009, 48, 2569 CrossRef PubMed; X. Yan and X. Hu, J. Org. Chem., 2014, 79, 5282 CrossRef PubMed; D. Knueppel, J. Yang, B. Cheng, D. Mans and S. F. Martin, Tetrahedron, 2015, 71, 5741 CrossRef; D. J. Asby, M. G. Radigois, D. C. Wilson, F. Cuda, C. L. L. Chai, A. Chen, A. S. Bienemann, M. E. Light, D. C. Harrowven and A. Tavassoli, Org. Biomol. Chem., 2016, 4, 9330 Search PubMed; J. Feng, X. Lei, Z. Guo and Y. Tang, Angew. Chem., Int. Ed., 2017, 56, 7895 CrossRef.
- W. Sun, D. C. Wilson and D. C. Harrowven, Synthesis, 2017, 3091 CAS.
- S. T. Perri, L. D. Foland and H. W. Moore, Tetrahedron Lett., 1988, 29, 3529 CrossRef CAS; Y. Yamamoto, M. Ohno and S. Eguchi, Tetrahedron, 1994, 50, 7783 CrossRef; D. C. Harrowven, M. Mohamed, T. P. Goncalves, R. J. Whitby, D. Bolien and H. F. Sneddon, Angew. Chem., Int. Ed., 2012, 51, 4405 CrossRef . See also D. E. Collin, E. H. Jackman, N. Jouandon, W. Sun, M. E. Light, D. C. Harrowven and B. Linclau, Synthesis, 2021, 1307 Search PubMed.
-
C. P. Krimmel, US Pat., 4036828, 1977 CrossRef CAS PubMed; N. C. Lim, M. D. Morton, H. A. Jenkins and C. Brueckner, J. Org. Chem., 2003, 68, 9233 CrossRef CAS PubMed;
A. Crew, A.-H. Li, L. Qiu, A. L. Castelhano, H. Dong, A. Smith, L. Tardibono and T. Zhang, WO Pat., 34111, 2006 Search PubMed;
J. J. Baldwin, J. Yuan, B. Lawhorn, C. A. Leach, L. S. Kallander, P. T. Flaherty, S. B. Singh, R. D. Simpson, W. Zhao, G. Mcgeehan, Z. Xu, S. Semus, X. Hou, D. Ghirlanda, J. Zhang, B. Ghavini-Alagha, L. R. Terrell, Q. Lu, A. V. Ishchenko, D. A. Claremon, C. M. Tice, S. Cacatian and L. W. Dillard, WO Pat., 117482, 2007 Search PubMed;
J. Chen, Y. Chen, A. Regueiro-Ren, S.-Y. Sit and J. Swidorski, WO Pat., 2847, 2018 Search PubMed;
K. E. Benenato, E. S. Kumarasinghe and M. Cornebise, WO Pat., 170306, 2018 Search PubMed;
Z. Wang, N. Li, J. Ma and Y. Shao, WO Pat., 120296, 2019 Search PubMed;
N. Yu, WO Pat., 160054, 2020 Search PubMed;
Z. Wang, J. Ma, Y. Shao and N. Li, WO Pat., 160707, 2020 Search PubMed.
- W. A. Kinney, N. E. Lee, D. T. Garrison, E. J. Podlesny, Jr., J. T. Simmonds, D. Bramlett, R. R. Notvest, D. M. Kowal and R. P. Tasse, J. Med. Chem., 1992, 35, 4720 CrossRef CAS PubMed; A. P. Davis, S. M. Draper, G. Dunne and P. Ashton, Chem. Commun., 1999, 2265 RSC; Y. Brandy, N. Brandy, E. Akinboye, M. Lewis, C. Mouamba, S. Mack, R. J. Butcher, A. J. Anderson and O. Bakare, Molecules, 2013, 18, 1973 CrossRef PubMed; N. Sim, S. Gottschalk, R. Pal, J. Engelmann, D. Parker and A. Mishra, Chem. Sci., 2013, 4, 3148 RSC; M. Lu, Q.-B. Lu and J. F. Honek, Bioorg. Med. Chem. Lett., 2017, 27, 282 CrossRef.
- Reduction is presumed to occur by a mechanism akin to the Meerwein-Ponndorf-Verley reduction, i.e.
R. N. McDonald, R. N. Steppe and R. C. Cousins, J. Org. Chem., 1975, 40, 1694 CrossRef CAS; C. Kowalski, X. Creary, A. J. Rollin and M. C. Burke, J. Org. Chem., 1978, 43, 2601 CrossRef; M. Majewski and D. M. Gleave, J. Organomet. Chem., 1994, 470, 1 CrossRef.
-
M. J. Frisch, G. W. Trucks, H. B. Schlegel, G. E. Scuseria, M. A. Robb, J. R. Cheeseman, G. Scalmani, V. Barone, B. Mennucci, G. A. Petersson, H. Nakatsuji, M. Caricato, X. Li, H. P. Hratchian, A. F. Izmaylov, J. Bloino, G. Zheng, J. L. Sonnenberg, M. Hada, M. Ehara, K. Toyota, R. Fukuda, J. Hasegawa, M. Ishida, T. Nakajima, Y. Honda, O. Kitao, H. Nakai, T. Vreven, J. A. Montgomery, Jr., J. E. Peralta, F. Ogliaro, M. Bearpark, J. J. Heyd, E. Brothers, K. N. Kudin, V. N. Staroverov, R. Kobayashi, J. Normand, K. Raghavachari, A. Rendell, J. C. Burant, S. S. Iyengar, J. Tomasi, M. Cossi, N. Rega, N. J. Millam, M. Klene, J. E. Knox, J. B. Cross, V. Bakken, C. Adamo, J. Jaramillo, R. Gomperts, R. E. Stratmann, O. Yazyev, A. J. Austin, R. Cammi, C. Pomelli, J. W. Ochterski, R. L. Martin, K. Morokuma, V. G. Zakrzewski, G. A. Voth, P. Salvador, J. J. Dannenberg, S. Dapprich, A. D. Daniels, Ö. Farkas, J. B. Foresman, J. V. Ortiz, J. Cioslowski and D. J. Fox, Gaussian 09, Revision C.01, Gaussian, Inc., Wallingford CT, 2009 Search PubMed.
- P. X. Iten, A. A. Hofmann and C. H. Eugster, Helv. Chim. Acta, 1978, 61, 430 CrossRef CAS; C. H. Eugster, M. Balmer, R. Prewo and J. H. Bieri, Helv. Chim. Acta, 1981, 64, 2636 CrossRef; M. E. Jung and L. J. Street, J. Am. Chem. Soc., 1984, 106, 8327 CrossRef; L. L. Klein and M. S. Shanklin, J. Org. Chem., 1988, 53, 5202 CrossRef; M. E. Jung and C. S. Siedem, J. Am. Chem. Soc., 1993, 115, 3822 CrossRef; G. H. Hakimelahi, M. L. Jain, T. W. Ly, I.-C. Chen, K. S. Ethiraj, J. R. Hwu and A. A. Moshfegh, J. Org. Chem., 2001, 66, 7067 CrossRef; M. Romero, P. Renard, D.-H. Caignard, G. Atassi, X. Solans, P. Constans, C. Bailly and M. D. Pujol, J. Med. Chem., 2007, 50, 294 CrossRef; M.-A. Légaré, E. Rochette, J. L. Lavergne, N. Bouchard and F.-G. Fontaine, Chem. Commun., 2016, 52, 5387 RSC; L. Grau, M. Romero, C. Privat-Contreras, D. Presa, M. Viñas, J. Morral, K. Pors, J. Rubio-Martinez and M. D. Pujol, Eur. J. Med. Chem., 2020, 185, 111807 CrossRef.
Footnote |
† Electronic supplementary information (ESI) available: Experimental accounts with spectral details and copies of NMR spectra are available as supplementary information. CCDC 2059384, 2042398 and 1500038. For ESI and crystallographic data in CIF or other electronic format see DOI: 10.1039/d1cc02097h |
|
This journal is © The Royal Society of Chemistry 2021 |
Click here to see how this site uses Cookies. View our privacy policy here.