DOI:
10.1039/D1AY01184G
(Critical Review)
Anal. Methods, 2021,
13, 5120-5143
Monitoring of emerging contaminants of concern in the aquatic environment: a review of studies showing the application of effect-based measures
Received
12th July 2021
, Accepted 20th October 2021
First published on 2nd November 2021
Abstract
Water scarcity is increasingly a global cause of concern mainly due to widespread changes in climate conditions and increased consumptive water use driven by the exponential increase in population growth. In addition, increased pollution of fresh water sources due to rising production and consumption of pharmaceuticals and organic chemicals will further exacerbate this concern. Although surface water contamination by individual chemicals is often at very low concentration, pharmaceuticals for instance are designed to be efficacious at low concentrations, creating genuine concern for their presence in freshwater sources. Furthermore, the additive impact of multiple compounds may result in toxic or other biological effects that otherwise will not be induced by individual chemicals. Globally, different legislative frameworks have led to pre-emptive efforts which aim to ensure good water ecological status. Reports detailing the use and types of effect-based measures covering specific bioassay batteries that can identify specific mode of actions of chemical pollutants in the aquatic ecosystem to evaluate the real threat of pollutants to aquatic lives and ultimately human lives have recently emerged from monitoring networks such as the NORMAN network. In this review, we critically evaluate some studies within the last decade that have implemented effect-based monitoring of pharmaceuticals and organic chemicals in aquatic fauna, evaluating the occurrence of different chemical pollutants and the impact of these pollutants on aquatic fauna with special focus on pollutants that are contaminants of emerging concern (CEC) in urban wastewater. A critical discussion on studies that have used effect-based measures to assess biological impact of pharmaceutical/organic compound in the aquatic ecosystem and the endpoints measurements employed is presented. The application of effect-based monitoring of chemicals other than assessment of water quality status is also discussed.
Introduction
A large proportion of fresh water sources in Europe are heavily polluted mainly due to anthropogenic influences in the form of point or diffuse pollution sources from industrial waste, household/sewage and most importantly agricultural sources.1–4 The European Environment Agency (EEA) reports that only 40% of European water bodies including lakes, rivers and coastal waters are of good ecological status,5 which may further reduce due to uncontrolled human activities.
One of the major anthropogenic factors significantly contributing to surface and underground pollution in Europe is pharmaceutical in origin. According to the European Federation Pharmaceutical Industry and Associations (EFPIA), about €200 billion worth of active pharmaceutical ingredients (APIs) were produced in Europe in 2016 alone.6 Besides considering local consumption, production waste from pharmaceutical industries can will diffuse into surface and ground waters. In fact, APIs are one of the few known pollutants known to permeate through into ground water sources.7 Unfortunately, while many of the APIs might be present in water sources in very small concentrations, the design of pharmaceuticals to be efficacious at very low concentration creates concern about possible biological consequences on both aquatic and human lives. Pre-emptive efforts have been made globally by different governmental bodies attempting to ensure recovery of surface and ground waters.8,9
Effect-based measures in assessing water quality status
More than 100
000 chemicals are registered with the European Chemical Agency (ECHA) with known safety data sheets and about 30
000 of these chemicals are sold and highly consumed annually across Europe. These chemicals eventually end up in thousands of surface waters across Europe.10 Monitoring this high volume of pollutants is an arduous task, although high resolution mass spectrometry (HRMS) in conjunction with gas or liquid chromatography has allowed high throughput monitoring of hundreds of chemicals at a time. This has resulted in an update and revision of existing priority lists or watch list, developed to monitor water quality, with the inclusion of new chemicals that are derived from the contaminants of emerging concerns (CECs) list (Table 1).7,11
Table 1 Major pharmaceuticals in the CEC list and their reported mode of action
Pharmaceutical |
log Kowa |
MW (g mol−1) |
Mode of action (MOA) |
Reference |
log Kow: n-octanol/water partition coefficient.
|
|
4.32 |
785.0 |
Binds and inhibits assembly of large ribosome subunit 50S to prevent bacteria protein synthesis |
12
|
|
4.51 |
334.23 |
Inhibits Cox1 and 2 to prevent prostaglandin synthesis, blocking pain signalling |
13
|
|
3.16 |
747.953 |
Prevents bacteria growth and division by binding to 23S rRNA, a component of the 50S subunit of bacterial ribosome, inhibiting peptides translation |
14
|
|
3.06 |
733.93 |
Binds and inhibits assembly of large ribosome subunit 50S to prevent bacteria protein synthesis |
15
|
|
0.87 |
365.4 |
Binds to penicillin-binding proteins that inhibit transpeptidation, leading to activation of autolytic enzymes e.g. autolysins in the bacterial cell wall. This leads to lysis of the cell wall, and thus, the destruction of the bacterial cell |
16
|
|
0.28 |
331.4 |
Inhibits DNA replication by inhibiting activity of DNA gyrase and topoisomerase IV preventing unwinding of DNA in bacteria |
17
|
|
0.65 |
290.32 |
Inhibit dihydrofolate reductase synthesis of tetrahydrofolate which is required for thymidine biosynthesis and thus inhibits DNA synthesis |
18
|
|
0.48 |
253.28 |
Competitively inhibits dihydropteroate synthase preventing the formation of dihydropteroic acid, a precursor of folic acid which is required for bacterial growth |
19
|
|
2.47 |
236.3 |
Sodium channel blocker. It binds preferentially to voltage-gated sodium channels in their inactive conformation, which prevents repetitive and sustained firing of an action potential |
20
|
|
4.7 |
250.34 |
Activates PPAR-α to stimulate peroxisomal β-oxidation by up-regulating the expression of all three important peroxisomal β-oxidation enzymes (acyl-coa oxidase, 2-trans-enoyl-coa hydratase, and thiolase) |
21
|
|
3.2 |
277.4 |
Increases serotonin, norepinephrine, and dopamine levels in the brain by blocking transport proteins that facilitate its reuptake at the presynaptic terminal |
22
|
Legislation leading to effect-based monitoring of water quality status
The European Union Water Framework Directive (WFD) was established with the aim of encouraging stakeholders to set targets and meet them so as to achieve good water ecological status.23,24 This directive was also promulgated to ensure protection and sustainability of fresh water resources in Member States. However, one of the major challenges in implementing the European Union WFD is achieving a reduced chemical footprint in freshwater sources by minimizing the leaching of toxic chemical cocktails.24 Chemical status of European fresh water bodies was initially defined on the basis of compliance to selected environmental quality standards (EQS) for a finite number of pollutants in the priority substance list, although European water bodies were heavily polluted with a mixture of tens of thousands of chemical pollutants. Regulations to manage these chemicals as priority substances achieved their reduction, but this was not sustained due to introduction of replacement chemicals which were also toxic. This, in addition to the growing list of other undocumented chemicals, resulted in inaccurate representation of water quality.2,25 Even with the priority substance list being constantly updated, the effect of the expanding list of possible chemical mixtures that may produce a concentration addition, makes its use in the assessment of water quality status difficult.
Policy transition and formation of standards for effect-based monitoring
Although the chemical status of surface waters in Europe is constantly being updated with new pollutants added to the CEC list, there is an increasing need to evaluate the biological implication of the chemical mixtures on aquatic ecosystems and human health. The European subgroup Chemical Monitoring and Emerging Pollutants (CMEP) legislated within the Common Implementation Strategy (CIS) was tasked with developing a technical report that identifies effect-based measures (EBMs) for chemical pollution in the aquatic biota. The directive from the CMEP indicated that the report was to identify EBM tools such as bioassays and biomarkers that would be applicable in surveillance, operational and investigative programmes that assesses ecological status, to provide useful data that drives hypothesis generation and correlation with ecological observations.26,27 Based on the result of EBM-based studies, the toxicity index of a water body can be understood in the context of a dynamic monitoring outcome, complemented with a battery of in vitro and in vivo assays that can evaluate short- and long-term effects on aquatic organisms like algae, invertebrates and fish which are the major representatives of WFD Biological Quality Elements (BQE). A consequence of this was the establishment of novel research activities leading to the funding of a large EU-wide Project SOLUTIONS. This project drafted a common position on the application of EBMs in diagnosis and water quality monitoring, such as the use of effect-based trigger values to determine water quality.28 In addition, the activities of the European monitoring network (NORMAN) led to the agreement and establishment of a unified battery of bioassays that will cover modes of actions of all chemicals that pose harm to aquatic ecosystems and human health.26 Standard operating procedures for in vitro and in vivo bioassay batteries were published for different mode of actions (MOAs) such as chemicals' estrogenicity, androgenicity and ability to modulate immune response via activation of aryl hydrocarbon receptor or activation of glucocorticoid receptor and the peroxisome activated receptor, for instance.26,28
This review is an assessment of some studies using effect-based monitoring tools as a snapshot to evaluate the biological impact of pharmaceutical pollutants on aquatic fauna with special highlight of pharmaceutical contaminants that are CEC in urban wastewater. A brief discussion on the findings of studies that implemented effect-based measures in the assessment of the biological effect of pharmaceutical and organic compound contaminants in the aquatic ecosystem as well as the types of endpoints investigated are presented. The application of effect-based monitoring of chemicals other than for the assessment of water quality status is also discussed.
Literature search strategy and selection criteria
The published literature evaluated in this study were systematically retrieved from Scopus® (Elsevier, Netherlands) and PubMed databases in December of 2020. Published studies between 2011 and 2020 that investigated some form of effect-based monitoring of pharmaceutical and organic chemical pollution in different water bodies were selected based on the search criteria below. Several keywords were used to search for effect-based monitoring of pharmaceutical: “bioassay” or “biomonitoring” or “effect-based monitoring” and “pharmaceutical(s)” or “drug(s)” or “chemical” and “water” or “freshwater” or “surface water” or “aquatic” and “pollution” or “contamination”. These terms were searched across keywords, titles and abstracts and only research articles were included.
Workflow for MOA determination
The general workflow for the determination of the MOA of chemicals, based on the studies reviewed here, generally follow four predominant stages, which involves (1) identification and extraction of target chemical(s) from matrix, (2) pre-concentration of target chemical(s) and processing of samples, (3) bioassay set up for hypothesised MOA and (4) data processing and interpretation of study findings. The sample extraction entails all processes involving sample collection from the matrix which were mainly wastewater treatment plants (WWTP). Other sources of sample collection include river sediments or water, freshwater sources and sewage treatment plant (STP) (Fig. 1 and 2). Analyte extraction methods from a sample is predetermined by the analytes physicochemical properties. A common trend in studies that carry out extraction of chemical contaminants or sample collection from WWTP and river sources is the use of a grab sampling technique mainly through automated sampling methods or manual composite sample collection at specific time intervals. Passive sample collection when carried out was mainly through the use of polar organic chemical integrative samplers (POCIS) for polar compounds or semipermeable membrane devices (SPMD) for hydrophobic contaminants or both. Analysis of extracted chemical pollutants were mainly carried out by gas chromatography (GC) or liquid chromatography (LC) coupled with mass spectrometry (MS) owing to the sensitivity and applicability of the techniques for both quantitative and qualitative analyses. One study, however, used both grab and passive sampling.29
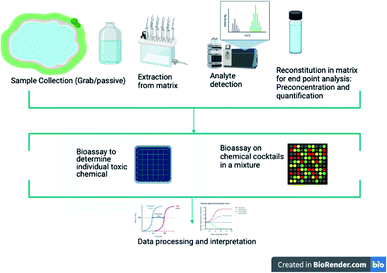 |
| Fig. 1 A typical workflow for mode of action determination of chemical contaminants from sample collection to data on effects. | |
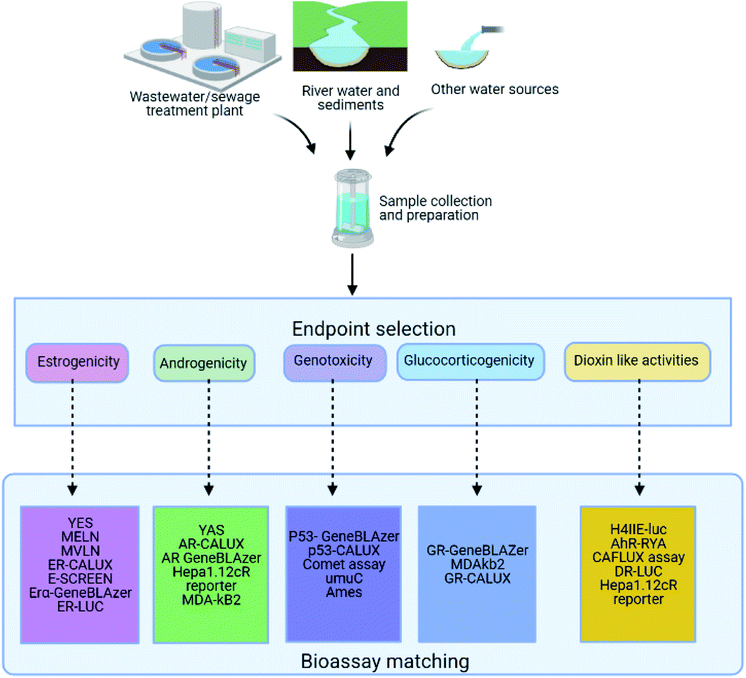 |
| Fig. 2 Illustration of the types of effect-based bioassays and their reported endpoint application: Selecting bioassay for effect-based monitoring often start with identification of appropriate matrix for sample collection. Collected samples by either passive of grab sampling are prepared for assay either through water reconstitution with pure chemical samples or spiking of collected water samples. This is then followed by endpoint selection and bioassay matching. | |
Sampling at WWTPs and rivers using river sediments or water samples was a common practice with some studies sampling at river sites that are proximal to WWTP.29–31 This is because WWTPs are discharge points for chemicals that may influence the composition of adjacent or nearby surface water sources. On the contrary, there are also studies reporting bio-effect of individual and cocktail mixture of pharmaceuticals using reconstituted analytical grade samples of the pharmaceuticals in specific matrices such as water.32–37
Major mode of actions and endpoints
The importance of the need for effect based monitoring as an alternative to generic assessment of risks for individual chemical for instance, has been previously suggested.38 Busch et al. (2016) provided a synopsis of the possible MOAs available for documented chemicals, while noting that there is limited knowledge on possible MOAs for water quality monitoring.38 However, there has been significant change in knowledge since then. The use of bioassays as a measure of water quality status is inherently dependent on the biological effects of pharmaceuticals and other organic chemicals that can be induced in biological faunas. Respectively, this design is also dependent on the chemistry of each individual chemical. There are myriads of bioassays that have been recommended by, for instance, the NORMAN network or the SOLUTIONS project26,28 and the different types of MOAs investigated in some of the studies evaluated here are shown in Tables 2–4.
Table 2 Summary data from studies investigating MOA of sample mixtures from WWTP using grab sampling
Sample type |
Sampling method |
Bioassay |
MOA |
BEQ/EEQa |
Major analytes (total target analytes) |
Reference |
BEQ/EEQ are bioequivalence/estrogenic equivalence, * values are Toxic Mode of Action (TMoA) calculated as no-observed effect concentration transformed to bioanalytical equivalent of reference compounds, DGT = diffusive gradient in thin film, E2 = estradiol.
|
WWTP |
Grab |
E-screen |
Estrogenicity |
WWTP1 = 28.5 ± 10.6 ng E2 L−1 |
Diclofenac, naproxen, bisphenol A (15) |
40
|
WWTP2 = 6.0 ± 3.2 ng E2 L−1 |
MELN |
Estrogenicity |
WWTP1 = 21.7 ± 4.2 ng E2 L−1 |
WWTP2 = 6.7 ± 1.7 ng E2 L−1 |
WWTP |
Grab |
H4IIE-luc |
Dioxin-like activity |
0.1 to 0.7 ng TCDD L−1 |
Diclofenac, ketoprofen, acetaminophen, carbamazepine, atenolol, clarithromycin, amoxicillin, doxycycline |
76
|
MVLN |
Estrogenicity |
0.1 to 5.1 ng E2 L−1 |
MDA-kb2 cells with endogenous AR |
Androgenicity |
1–4 ng testosterone L−1 |
WWTP |
Grab |
YES |
Estrogenicity |
0.83 ng E2 L−1![[thin space (1/6-em)]](https://www.rsc.org/images/entities/char_2009.gif) |
Atrazine, bisphenol A, nonylphenol |
30
|
Algae growth |
— |
— |
Acetylcholinesterase (AChE) activity |
— |
— |
ERα-CALUX |
Estrogenicity |
1 ng E2 L−1 |
WWTP |
Grab and passive (DGT) sampling |
ERE-CALUX |
Estrogenicity |
0.05 ± 0.01 ng E2 L−1 to 29 ± 4 ng E2 L−1 |
— |
77
|
WWTP |
Grab |
Algal growth inhibition |
— |
— |
Terbuthylazine, prometryn, caffeine, azithromycin, levometiomeprazin (25) |
47
|
WWTP |
Fish collection from sites |
VTG assay |
Endocrine disruption |
— |
— |
78
|
WWTP |
Grab |
Yeast bio-reporter |
Estrogenicity |
0.7–14.0 ng E2 L−1 |
— |
79
|
WWTP |
Grab |
DR-LUC (H4L1.1c4) |
Dioxin-like activity |
5.7–10.2a |
Benzo[a]pyrene, fluoranthene, and tributyltin (205) |
41
|
ER-LUC (VM7Luc4E2) |
Estrogenicity |
0.11–7.1a |
AR-EcoScreen |
Androgenicity/Anti-androgenicity |
0.38–3.9a |
5.7–26a (antiandrogenicity) |
TTR-binding |
Thyroxine displacement from TTR protein |
2–200 ng of thyroid hormone (T4) L−1 |
Ames II |
Mutagenicity |
— |
Thamnotoxkit F™ |
Juvenile mortality of thamnocephalus platyurus |
— |
WWTP |
Grab |
YES |
Estrogenicity |
Reported as percentage receptor activation or inhibition |
— |
80
|
YAS |
Androgenicity |
Ames |
Mutagenicity |
— |
WWTP |
Grab |
ER-CALUX |
Estrogenicity |
0.925–23.8 |
Bisphenol A, E1, E2, EE2 (6) |
81
|
WWTP sediments and river basin |
Grab |
Antioxidant biomarkers |
Superoxide dismutase (SOD), glutathione S-transferase activity (GST) |
— |
Salicylic acid, 4-tert-octylphenole, p,p-dichlorodiphenyldichloroethene (168) |
42
|
H4IIE-luc |
Dioxin-like activity |
0.1–0.8 ng of sediment per mL |
|
Genotoxicity |
0.9–3.6 ng of sediment/mL |
WWTP |
Grab |
MVLN reporter gene assay |
Estrogenicity |
0.53 to 17.9 ng of E2 L−1 |
(75) |
43
|
WWTP |
Grab |
Recombinant yeast assay (RYA) |
ER-RYA |
Estrogenicity |
0.05 to 0.35 ng of E2 L−1 |
Parabens, bisphenol A, alkylphenolic compounds, benzotriazoles (31) |
82
|
AhR-RYA |
Dioxin-like activity |
≤ 50 ng benzo[a]pyrene L−1 |
WWTP |
Grab |
E-screen |
Estrogenicity |
0.03–0.56 ng E2 L−1 |
Nonylphenol, nonylphenol monoethoxylate, nonylphenol diethoxylate and bisphenol A |
50
|
Ames |
Genotoxicity |
— |
WWTP |
Grab |
MELN |
Estrogenicity |
2.53 ± 2.48 ng E2 L−1 |
— |
83
|
E-screen |
0.72 ± 0.58 ng E2 L−1 |
WWTP |
Grab |
YES |
Estrogenicity |
0.3–8.6 ng E2 L−1 |
— |
84
|
YAS |
Androgenicity |
— |
Ames |
Genotoxicity |
— |
WWTP |
Grab |
E-screen |
Estrogenicity |
0.034–2.4 ng E2 L−1 |
Metformin, carbamazepine, caffeine, melamine (276) |
44
|
ERα GeneBLAzer |
Estrogenicity |
0.0053–0.26 ng E2 L−1 |
AhR-CAFLUX |
Dioxin-like activity |
0.012 to 0.082 ng TCCD L−1 |
ARE GeneBLAzer |
Oxidative stress |
1.3 × 104–5.6 × 104 ng tBHQ L−1 |
AR GeneBLAzer |
Androgenicity |
0.051–5.8 ng R1881 L−1−1 |
p53 GeneBLAzer |
Genotoxicity |
< 0.24 ng mitomycin |
PPARγ-GeneBLAzer |
Peroxisome proliferator activity |
0.059–0.089 ng Rosi L−1 |
GR GeneBLAzer |
Glucocorticogenicity |
<1.1 ng Dex L−1 |
WWTP secondary site |
Grab and passive |
In situ VTG assay |
Endocrine disruption |
— |
Venlafaxine, trimethoprim, atrazine, sulfamethoxazole, diclofenac, caffeine (424) |
85
|
YES |
Estrogenicity |
Grab = 0.01 to 0.11 ng E2 L−1 |
Passive = 0.03 to 0.17 ng E2 L−1 |
WWTP |
Grab/automated sampler |
AR-CALUX |
Androgenicity |
|
|
86
|
ER-CALUX |
Estrogenicity |
0.45–42 ng E2 L−1 |
umuC |
Genotoxicity |
— |
P53-CALUX |
Genotoxicity |
53–6200 μg cyclophosphamide L−1 |
Fish embryo toxicity test (FET) |
|
— |
STP |
Grab |
MELN |
Estrogenicity |
1.2–35 ng E2 L−1 |
|
87
|
YES |
|
1.5–50 ng E2 L−1 |
Hepa1.12cR reporter |
Dioxin-like activites |
1.4–8.8 ngTCDD L−1 |
|
|
|
Table 3 Some studies investigating MOA of sample mixtures from WWTP using passive sampling techniquea
Sample type |
Sampling method |
Bioassay |
MOA |
BEQ/EEQ |
Major analytes (total target analytes) |
Reference |
TCDD = 2,3,7,8-tetrachlorodibenzo-p-dioxin, DHT = dihydrotestosterone.
|
WWTP |
Passive/POCIS |
Nrf2 reporter |
Oxidative stress, |
— |
Sulfamethoxazole, trimethoprim, metronidazole, erythromycin, clarithromycin, venlafaxine, (80) |
31
|
NFκB reporter |
Inflammation |
— |
ER reporter |
Estrogenicity |
1.3 ng E2 L−1 |
AR reporter |
Androgenicity |
4.2–7.8 ng DHT L−1 |
WWTP |
Passive (large volume solid phase extraction) |
Transcriptional changes of Danio rerio |
pepck1, g6pca, socs3 and slco2a1 genes |
— |
Estrone, 17β-estradiol, mifepristone, pregnanediol, androstenedione, androsterone (15) |
60
|
Behavioural changes (swimming of Danio rerio) |
— |
|
Developmental changes in Danio rerio embryo |
— |
— |
WWTP |
Passive (POCIS) |
Comet assay |
Genotoxicity |
— |
Acebutolol, bisoprolol, venlafaxine, carbamazepine (19) |
61
|
WWTP |
Passive (SPMD and POCIS) |
H4IIE-luc |
Dioxin-like activity |
0.008 to 2.0 ng TCDD L−1 |
Ciprofloxacin, erythromycin, trimethoprim, carbamazepine, diclofenac (72) |
45
|
MVLN |
Estrogenicity |
0.1 to 5.4 ng E2 L−1 |
MDA-kb2 cells with endogenous AR |
Androgenicity |
1.3 to 193 ng testosterone L−1 |
WWTP |
Passive/POCIS (compared POCIS pest with POCIS 20 d) |
MVLN |
Estrogenicity |
0.5 to 4.2 ng/POCIS |
Carbamazepine, ciprofloxacin, diclofenac, erythromycin, sulfamethoxazole, trimethoprim (79) |
88
|
H4IIE-luc |
Dioxin-like activity |
0.08 to 0.39 ng TCDD/POCIS |
WWTP |
Passive |
Ames |
Genotoxicity |
— |
Ciprofloxacin, Azithromycin’ erythromycin, clarithromycin, sulfamethoxazole (100) |
46
|
YES |
Estrogenicity |
0.3–10.8 ng E2 L−1 |
Table 4 Studies investigating MOA of sample mixtures from river water and sediments and surface water
Sample type |
Sampling method |
Bioassay |
MOA |
BEQ/EEQ |
Major analytes (total target analytes) |
Reference |
nd = no detection.
|
River |
Passive/POCIS and SPMD |
CAFLUX assay |
Dioxin-like activity |
SPMD = 0.0029–0.0073 ng TCDD L−1POCIS = 0.031–0.22 ng TCDD L−1 |
Carbamazepine, citalopram, codeine, diclofenac, diltiazem, irtesartan, trimethoprim and venlafaxine (77) |
137
|
MVLN |
Estrogenicity |
SPMD = 0.37–0.78 pg E2 L−1 |
POCIS= < 30 pg E2 L−1 −1.1 ng E2 L−1 |
MDA-kb2 cells with endogenous AR |
Androgenicity |
SPMD = 1 × 10−4–0.0035 ng DHT L−1POCIS = 0.59–55 pg DHT L−1 |
River sediment |
Passive/POCIS and chemcather |
Planar-YES |
Estrogenicity |
Quantified estrogens |
— |
157
|
YES |
3.4 ± 0.2–20.1 ± 2.5 μg E2 kg−1 |
River sediment |
Grab |
Gene expression analysis targeting |
EcR |
Ecdysone receptor activity |
— |
Propyzamide, napropamide, prochloraz and anthraquinone. (231) |
68
|
InR |
Insulin receptor activity |
Heat shock proteins |
Stress response |
Cyp450, SOD, |
Biotransformation reactions |
River water and sediment |
Grab |
ERE-CALUX |
Estrogenicity |
200–937 pg E2 L−1 |
— |
97
|
DRE-CALUX |
Dioxin-like activity |
0.6–42 pg TCDD g−1 sediment |
River |
Grab |
YES |
Estrogenicity |
0.021–0.13 ng E2 L−1 |
|
133
|
VTG assay |
Endocrine disruption |
|
River |
Grab |
YES |
Estrogenicity |
0.09 ng L−1 (antiestrogenic) |
— |
120
|
YAS |
Androgenicity |
1.96–3.13 ng DHT L−1 |
River |
Passive/SPMD and POCIS |
POCIS YES |
Estrogenicity |
0.03–0.28 pM E2 L−1 |
— |
107
|
SPMD YES |
Estrogenicity |
nda |
River |
Grab |
ER-LUC |
Estrogenicity |
0.01–0.84 ng E2-EQ g−1 |
Naphthalene, phenanthrene, Anthracene, fluoranthene, pyrene |
158
|
DR-LUC |
Dioxin-like activity |
0.05–50.6 ng TCDD-EQ g−1 |
TTR-binding |
Thyroxine displacement from TTR protein |
0.4–36.7 μg t4-EQ g−1 |
Ames II |
Mutagenicity |
≤0.221 l g−1−1 |
River |
Passive/POCIS |
MVLN |
Estrogenicity |
0.014–0.791 ng E2 L−1 |
Bromoxynil (31) |
48
|
Hepa1.12cR reporter |
Androgenicity |
0.01–0.064 ng R1881 L−1−1 |
Transiently transfected CHO-K1 |
Dioxin-like activites |
0.154–1.089 ng TCDD L−1 |
River |
Grab and passive/POCIS |
Grab YAS |
Androgenicity |
≤50 μg flutamide L−1 |
Diclofenac, trimethoprim, venlafaxine, carbamazepine, tramadol (>31) |
29
|
POCIS |
≤80 μg flutamide L−1 |
River sediment |
Passive/POCIS |
MELN |
Estrogenicity |
1.0–3.2 ng E2 g−1 |
Carbamazepine, gemfibrozil, pregnenolone, prednisone, prednisolone |
150
|
MDA-kb2 |
Glucocortigenicity |
11.1–180.3 μg Dex g−1 |
Surface water |
Grab |
GR-CALUX |
Glucocortigenicity |
>0.4–<3.0 ng Dex L−1 |
— |
59
|
Major primary endpoints assessment from samples at WWTPs and rivers were estrogenicity and androgenicity and analyses of such samples containing different chemical mixtures29,39–57 (Fig. 2). For example, diclofenac, carbamazepine, ciprofloxacin and venlafaxine are some of the commonly analysed compounds for estrogenic and/or androgenic activities. Most of these chemicals with the exception of diclofenac have log
Kow of <4.5 indicating a relatively water soluble nature (Table 1). On the other hand, diclofenac has a log
Kow of 4.51 indicating a lipophilic nature, which explain the estrogenicity exhibited by diclofenac as demonstrated by Klopcic et al.34 Interestingly, diclofenac log
Kow lies in the range of log
Kow that has been demonstrated to belong to estrogenic compounds.58 Some other studies investigated mutagenicity, aryl hydrocarbon receptor activation in detecting dioxin-like activities, glucocorticogenicity and genotoxicity.42,47,51,59–67 While these are specific endpoints that have been somewhat legislated for in biomonitoring of water quality, the list of endpoints that are measurable is open-ended. Chemicals modifying biological processes do so at the molecular level through activation of specific proteins that may be modified by perturbed gene expression. Such outcome measures are often investigated using in vivo tests although some instances of in vitro gene expression assays exists.60,68–75 For instance, Zhang et al. (2017) measured the alteration of glucose metabolism and gene expression in zebrafish embryos and were able to detect significant alteration in expression of genes involved in lipid synthesis upon exposure to environmentally relevant concentration of target analytes including estradiol, mifepristone and pregnanediol in river water samples.60 Arambourou et al. (2019) monitored whole organism response of Chironomus riparius to river sediments contaminated with heavy metals and polyaromatic hydrocarbons (PAHs) in addition to evaluation of gene expression changes such as genes involved in stress gene response, those modulated by insulin receptor activities and ecdysone receptor activities.68 Another major endpoint often measured is the detection of specific established biomarkers, and these tend to vary from study to study. Interestingly, the main set of biomarkers often investigated are antioxidant biomarkers or biomarkers of metabolic pathways. Although these in vivo tests demonstrate a quantifiable biological response, it must be noted that such systems are not easy to set-up and may be time consuming compared to bioassays like the luciferase based assays such as CALUX and ER/DR-LUC bioassays.
Determining the endpoint measure may be influenced by the type of analytes expected to be present within the sample mixtures, i.e. only determining endpoint measures such as estrogenicity or androgenicity for suspected endocrine disrupting chemicals (EDCs).30,40 Predominantly, studies looking into possible MOAs of compounds within the CEC list such as diclofenac, carbamazepine, venlafaxine and trimethoprim primarily investigated estrogenicity and androgenicity of the sample mixtures wherein they are present. While some studies have not clearly stated reason for the choice of bioassay, this may also be influenced by the regulations in the WFD, listing possible bioassays that should be tested rather than careful analysis of the chemistry of the analytes. The implication of this may be the observations of non-estrogenicity or non-androgenicity of samples while the sample may induce activation of other biological pathways. In a similar fashion, MOAs for organic chemicals in pesticides were mainly evaluated through genotoxicity, thyroxine displacement from transerythrin and glucocorticogenicity in addition to estrogenicity and androgenicity.
Bioassays utilised to measure endpoints
Directives such as the WFD or recommendations from different studies have aggregated into listing series of endpoints that can be measured by a battery of bioassays.26,28 It is suggested that these should be implemented in guaranteeing accurate determination of water quality status through biomonitoring. However, there are no details on what type of bioassays must be used to determine such endpoints. In general, bioassays are reporter gene based as seen in the CALUX system, DR/ER-LUC, MDA-kb2, MELN, MVLN or H4IIE-luc systems,89–102 where genes specific to the endpoint such as estrogen receptor for estrogenicity, and a response element for that receptor are engineered in a plasmid vector located upstream of a luciferase enzyme. Activation of the receptor by the ligand, which in this case is the chemical contaminant, results in activation of the receptor and binding with the response element. This facilitates transcription of the luciferase enzyme that can catalyse a non-fluorescent substrate to a luminescent product (Fig. 3).
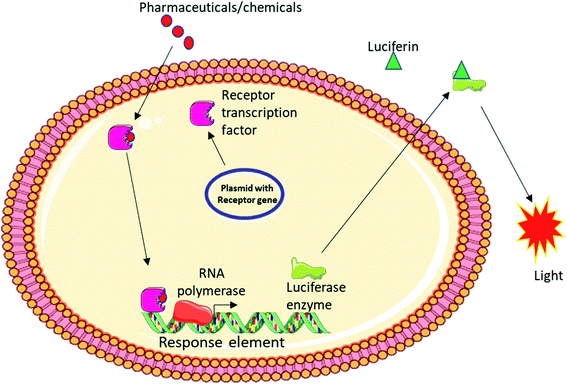 |
| Fig. 3 Schematic showing the luciferase-based bioassay system: the reporter gene assay utilises the fusion of putative regulatory elements, involving the response elements and transcription factor to a reporter gene to facilitate transcriptional activation of downstream gene. Since the expression of the gene product (reporter gene, luciferase enzyme in this case) is under the control of the fused response element, luciferin expression is directly correlated with the activity of the response and can be quantified by the activity of luciferase in converting luciferin to a coloured product. | |
Different bioassays have been used in studies reviewed here to assess estrogenicity of chemicals like diclofenac, trimethoprim, carbamazepine and venlafaxine and some include the yeast based assay (YES), E-SCREEN, MELN and MVLN reporter cell lines and ER-CALUX while androgenicity was mainly evaluated using YAS, which is the androgenic assay equivalent of the YES assay (Tables 2 and 3). Based on this, estrogenicity appeared to be the endpoint with the most diversified bioassay. This is also evident in the different versions of specific tests that exist. As an example, YES assay is a chromogenic test that depends on conversion of a substrate into a coloured product and appeared to be a popular choice amongst researchers. A bioluminescent version of this assay similar to the H4IIE-luc test was used in the study by Omoruyi and Pohjanvitra79 where activation of the endogenic ER result in expression of a luciferase enzyme that can convert the substrate luciferin into a luminescent product (Fig. 3). AR-CALUX and AR-GeneBLAzer were also used in monitoring sample androgenicity. One main reason for the popularity of YAS assay amongst these studies may be due to the low cost compared to the other androgenicity assays such as AR-CALUX. Of all the systems used for endpoint measurement, the MDA-kb2 reporter cell line seem to be the most versatile system as it contains reporter genes for the androgen and glucocorticoid receptors that can detect androgenicity and glucocorticogenicity respectively. This type of system may help circumvent the costly nature of most bioassays if more reporter genes can be incorporated.
Detected estrogenic activity in all matrices
For most relevant endpoints, there are a multitude of bioassays that are employed in evaluating such endpoints. For example, estrogenicity is a measure of the activation of the ER and this endpoint can be evaluated using YES, ER-CALUX, MELN or MVLN bioassays. Some of the EEQ values reported for estrogenicity for individual bioassays were in line or well below the calculated effect-based trigger values (EBT) values of estrogenicity while some were considerably higher according to previously reported data.103 For example, the EBT value reported for MELN assay by Escher et al. (2018) was 0.68 ng E2 L−1 while many studies reported EEQ values for estrogenicity using MELN bioassay above 1.0 ng E2 L−1.40,103–106 Thus, the problem of the acceptability of these values from experimental data arises when considering wider applications in water quality assessment. Unfortunately, without a universally acceptable EBT values that can be referenced for a particular endpoint, correlating data from different bioassays on the same endpoint may become cumbersome, requiring caution during data interpretation. This phenomenon was observed in some of the studies reviewed here, in which different bioassays were used to evaluate the same endpoint with some differences in the EEQ values obtained.30,40,44,83 The difference between the values recorded by the different bioassays for the same endpoint in a particular study may not be large enough to be significant, in which case such differences may be ignored as observed, for instance, in the data reported by Konig et al. (2017) and Kienle et al. (2019) (< 0.2 ng E2 L−1).30,44 This becomes problematic in instances where such difference is considerably large as in the studies by Schiliro et al. (2012) and Spina et al. 2020 (1.7 ng E2 L−1 and 6.8 ng E2 L−1 respectively between E-Screen and MELN assays).40,83 Therefore, it becomes pertinent to develop ways of deriving EBT values for individual bioassays for a given endpoint. According to Escher et al. (2018), this should be an average generic EBT value per endpoint considering all possible bioassay data and such value should be adjusted with a bioassay-specific sensitivity factor that makes it relevant across the board.103
As stated earlier, a high proportion of studies that sampled at WWTP, rivers and other water sources (Tables 2–4), investigated estrogenicity. The limit of detection of estrogenicity varies between all these studies and even at this, estrogenic activities is more likely to be detected than any other mode of actions, although some studies reported no detection of estrogenic activities is some of the sampled locations.93,107 This may be attributed to the chemical properties of the analytes in the collected samples. For short and long term exposure, EEQ values <0.5 ng of E2 L−1 is considered to be the safe level.108 Interestingly, while some studies reported estrogenic activities at values below 0.5 ng of E2 L−1,53,82,97,109–111 many others reported a range of EEQ values above 0.5 ng of E2 L−1.30,100,106,110,112–118 Overall, a wide range of estrogenic activity were detected from WWTP, both influents and effluents. Lower values of estrogenic activities were generally recorded in WWTP effluents for studies applying estrogenicity assessment to evaluate water quality and waste treatment efficiency.80,109,110,119 In these studies, the low estrogenic activities were linked to elimination of chemical pollutants in the treatment processes. Conversely, high estrogenicity values well above 100 ng E2 L−1 were also recorded in other studies while some others reported anti-estrogenic activities.44,45,51,80,120–123 Quantitating anti-estrogenicity in these studies corresponds to measurement of the decrease in detected fluorescence, luminescence or absorbance signal given by a specified amount of competing standard ligand for the estrogen receptor, i.e. as EEQ of the antagonist in ng of reference antagonist L−1. These findings can potentially impact decision making supporting the need for effect-based monitoring of pollutants or contaminants as a standard assessment of water quality at WWTPs or some other water bodies. However, recorded estrogenicity in samples collected upstream from some WWTP discharge points may be found to exceeded some calculated risk trigger values or values obtained at the WWTP by same assay as noted by Archer et al. (2020).121 This highlights the significance of how alternative pollution sources may be contributing towards accumulation of estrogenic or xenoestrogenic contaminants in the environment and ultimately the interpretation of results from assays at specific locations.
In general, the estrogenic activities recorded downstream of WWTPs were lower compared to those measured at the WWTP, for obvious reasons. WWTP, especially the influents, are hotspots containing higher concentration of chemicals. Even though the effluents discharged into other surface water bodies such as rivers and streams typically have lower CEC concentrations than influent samples, effluent CEC concentrations are generally higher than in the secondary sites due to dilution that occurs when effluents mix with surface waters. WWTPs serve residential, hospital and industrial buildings where direct human activities impact the level of estrogens and xenoestrogens in household or commercial wastes. For instance, excreted estrogens and unused estrogenic drugs as well as other xenoestrogenic drugs are disposed through the sewage systems,124 and this leads to an increase the pressure of these chemicals on the STPs. Although YES assay recorded by higher estrogenicity compared to MELN assay, this is likely dependent on the sensitivity of the assays as explained earlier.
In situ quantification of VTG is an efficient way to detect induction of estrogenic pathways and to assess the real life impact of xenoestrogens. VTG secretion, unique to female fish species, has been widely used in different studies as a marker of feminisation in different male fish species due to estrogenic influence of contaminants in water bodies.78,85,125–133 Assessment of VTG expression is often used in conjunction with other estrogenicity bioassays as a complementary method for assessment of endocrine disruption within aquatic fauna. Such application was employed by Geraudie et al. (2017) and Ganser et al. (2019) in their studies where YES assay was used to evaluate estogenicity of water samples in addition to the VTG assay.85,133 Unexpectedly, while Ganser et al.85 found significant induction of estrogenic signals from the YES assay, VTG level was not significantly induced in the male fishes sampled. On the contrary, Geraudie et al.133 discovered significant increase in VTG levels complemented by positive estrogenic activity as detected by the YES assay even though both studies reported similar ranges of E2 equivalence in the sample mixtures (Tables 2 and 3). Many factors could be responsible for this disparity in the two studies, all of which are required to be considered when correlating findings of such complementary assays. Some of these include length of exposure of sampled fish to estrogens and xenoestrogens within the aquatic environment and species-specific response of fishes to chemicals, as different sampled species are bound to have different responses to xenoestogens,134 especially when the concentration of contaminants is high enough to induce response in the bioassay but not enough to cause VTG secretion.
Grab vs. passive sampling and estrogenicity in context of WWTP
Of the major sampling methods available, composite and passive sampling were the most common in the studies reviewed. Interestingly, “grab sampling” where one sample is taken at a location for one specific time point time was often synonymously used for composite sampling in which case samples are grabbed from same location but over a period of time (Tables 2 and 3). The choice of employing passive sampling or grab sampling seem to be dictated by the type and nature of the contaminants that are expected to be present in the water body that is sampled. For instance, most persistent organic contaminants within the aquatic ecosystem are hydrophobic in nature, while other commonly used pharmaceuticals or pesticides are hydrophilic. These types of chemicals can be possibly sampled using various passive samplers based on their hydrophobic or hydrophilic properties.135 Based on this application of passive sampling requires some level of prior knowledge of the chemicals that are present in the sampling location, to allow for compatibility between the passive sampler and the contaminants to be sampled. On the other hand, application of grab/composite sampling does not require prior information. This may have reflected in the fact that more studies in general employed grab over passive sampling. On average, from a short survey of selected studies (Tables 2 and 3), estrogenicity recorded from passive sampling (range = 0.06–10.8 ng E2 L−1) was not markedly different from those from grab samples (range = 0.05–42 ng E2 L−1), indicating likelihood of no difference in the sensitivity of both passive and grab sampling. This notion is supported by findings of Guo et al. (2019), where samples from passive and grab samples provided not only similar estrogenic activities, but similar results in other endpoints investigated.77 This was also similar to the findings of Ganser et al. (2019), where grab samples were taken on two separate days, while passive sampling using solid phase extraction, was use to obtain samples in the time period between those two days.85 Although the passive sampler could not detect estrogenicity at the initial time point, the detection of estrogenic activity in the grab sample may be as a result of possible high concentration of analyte in the grab sample, which needs time to reach the same levels in a passive sampler.136 In addition, the WWTP influents are hotspots that contain high concentrations of chemical pollutants which can be easily detected without the need of passive sampling for extended periods. Extreme conditions in the WWTP influents can also make application of passive sampling difficult, thus favouring the use of grab sampling over passive sampling.
Variation in results from samples collected by different methods was also a phenomenon that was observed. Jalova et al. (2013) used SPMD and POCIS samplers in sample collection.45 POCIS sample showed estrogenic activities which were not detected in samples collected by SPMD. A similar finding was reported by Tousova et al. (2019), where the highest estrogenicity detected for POCIS was more than a thousand fold what was detected for SPMD.137 This can be explained by the difference in the pollutants chemistry that adsorb to the surface of the samplers. POCIS was developed to adsorb polar organic chemicals while SPMD adsorbs hydrophobic chemicals. As such, the polar solvent collected by the POCIS is likely more estrogenic. It is known that a polar group that is capable of donating hydrogen bonds on an aromatic system such as in the hydroxyl group is the most prominent structural feature that is crucial for estrogenic activity.138 This is not likely to be present on a hydrophobic compound.
Other measured endpoints in all matrices
Another common mode of actions is androgenicity, which is mainly evaluated by YAS yeast-based assay and AR-based reported assays like AR-GeneBLAzer, AR-EcoSCREEN and AR-CALUX. Unlike estrogenicity, where the main reference chemical was E2, different reference chemicals like DHT and metribolone (R1881) were used by different studies making comparative analysis difficult. Nonetheless, the androgenic activity detected varied across all studies that used DHT as reference chemical. The lowest recorded values were often recorded in river samples as observed for estrogenicity, which was also observed for other endpoints like dioxin-like activities. This is expected because most water from different point sources entering the river would have been treated at a WWTP to reduce the level of contaminants. The same variability phenomenon for studies using different sample collection types such as SPMD vs. POCIS was observed and this is due to the difference in the chemistry of the collected chemicals regarding the polarity and hydrophobicity of the target analytes. Although most studies detected some level of androgenic activities, some studies recorded no androgenic activities. This lack of activity may be due to the pre-treatment of the water samples as found in the study by Wildhaber et al. (2015) where effluent samples that had been ozonated were tested.84 This is especially as ozonation is a widely used method for decontaminating wastewater, proven to remove steroids from waste water.60,139 This lack of activities not only indicate the application of effect monitoring in assessing the effectiveness of waste water treatment but also reiterate the importance of incorporating such bioassay in water treatment protocols for routine assessment.
Aryl hydrocarbon receptor activation (dioxin-like activities) was another common endpoint tested due to the implication of aryl hydrocarbon receptor activation in cancer development and progression.140 Like estrogenicity, dioxin-like activities were evaluated by numerous bioassays. Most of the studies interestingly used TCDD as the reference chemical but the difference in the sensitivities of these bioassays will also still require some caution in comparing the data. One study did not use TCDD, but benzo[a]pyrene (B[a]P) as the reference compound.82 This is because polycyclic aromatic hydrocarbons (PAH) are known activators of the AhR, and while they do not exhibit the toxic effect of dioxin-like chemicals, their application as a monitoring tool to activate AhR receptor is the main objective.
Assessment of changes to the genetic make-up of aquatic organisms is one of the debilitating effect of chemical contaminants, which is why genotoxicity assessment has been widely investigated globally.66,73,141–149 Genotoxicity was mainly evaluated by Ames, comet and p53-based reporter assay. However, whole organism assessments for DNA damage were also common. Genotoxicity was not detected in some studies and those that detected some genotoxic activities did not use the same data report format. This was based on the variation in the types of assays and the application, making the genotoxicity measurement not uniform across all studies as seen for bioassays like estrogenicity or androgenicity. As a result, the findings of these studies are open to different interpretations. For instance, mitomycin and cyclophosphamide were the reference chemicals used by Konig et al. (2017) and Valitalo et al. (2017), respectively.44,86 Maier et al. (2015) reported the quantity of WWTP sediment that induced the LOD signal.42 The main set back to this disparity is the inability to make comparative analysis or generalised inference that can inform decision making.
Less specific endpoints such as glucocorticogenicity and gene expression profiling were observed.44,59,60,68,69,150–156 Glucocorticogenicity was mainly evaluated by reporter based bioassay and all three samples used dexamethasone as the reference compound. Some level of glucocorticogenic activities were recorded in all studies but as with other studies, the problem encountered is the quantification of BEQ in sediment samples as compared with water samples, which are not easily comparable and requires taking the matrix into consideration. For studies looking at gene expression, this bioassay is tailored to specific research questions, and as such are not generally relatable. However, the possible deduction from the findings of these two studies is the significant changes in transcriptional expression of key genes that affect molecular pathways can be successfully detected in a carefully designed bioassay.
Endpoints on individually targeted analytes
Besides evaluating the biological effect of a mixture of analytes in different water and sediment samples, there are also studies that investigated the biological effect of single pharmaceuticals using a myriad of bioassays (Table 5). These are usually focused on understanding the biological effect of single compounds (independent action) or mixture of chemicals (concentration addition) through modelling toxicities of individual contaminants.159 Pure samples (analytical grade) of these pharmaceuticals was reconstituted in different solvents such as DMSO, water or methanol rather than extraction from collected water samples. Diclofenac, gemfibrozil, venlafaxine, and carbamazepine were the assayed pharmaceuticals all of which are contained in the CEC list.7,11 Diclofenac is one of the most common pharmaceuticals often studied for its independent action. Investigation of its anti-estrogenic, androgenic and anti-androgenic effects, toxic effects of plant growth, mortality and DNA damaging effect on different aquatic organisms have been carried out.32–34,37,160 Interestingly, there are some pharmaceuticals found to have beneficial effects, such as venlafaxine and carbamazepine that were found to reduce abnormal embryo development in zebrafish at an environmentally-relevant concentration of 0.5 μg L−1.36 This is in line with the report of a previous study that documented the highest carbamazepine concentration of 5 μg L−1 in a German wastewater.161 As such, extrapolation of this result to what was observed in the study by Galus et al. (2013) would present the possible danger of such concentration to zebrafish in aquatic environments.36 The applicability of the results from the examples of studies in Table 5, is however questionable as most of the effective and inhibitory concentrations reported are quite above environmentally relevant concentration. For instance, the highest concentration of diclofenac that has been reported in a WWTP was below 2 μg L−1,162 which is more than 1000 folds lower than most EC50 or IC50 reported here.
Table 5 Common endpoints evaluated for selected important pharmaceuticals
Pharmaceutical |
Endpoint |
Organism/bioassay |
Effective concentration |
Reference |
High concentration did not cause mortality.
CBZ concentration did not correspond with the estrogenicity of water sample.
|
Diclofenac |
Loss of shoot |
Solanum lycopersicum
|
5 mg L−1 (EC50) |
32
|
Diclofenac |
Mobility |
Ceriodaphnia silvestrii
|
37.9 mg L−1 (EC50) |
33
|
Diclofenac |
Estrogenicity |
YES
|
Anti-estrogenicity = 3.8 × 10−5 M (IC50) |
34
|
Androgenicity |
YAS
|
Androgenicity = 5.2 × 10−5 M (EC50) |
Anti-androgenicity = 6.3 × 10−5 M (IC50) |
Glucocorticogenicity |
MDA-kb2 cell line
|
GR = 4.6 × 10−8 M (EC50) |
Anti-GR = 2.1 × 10−8 M (IC50) |
Diclofenac |
Genotoxicity (DNA strand breaks) |
M. galloprovincialis
|
25 μg L−1 |
35
|
Gemfibrozil |
Mortality, embryo development |
Danio rerio
|
Mortality = 0.5 and 10 μg L−1 |
36
|
Developmental abnormality = reduced 0.5 μg L−1 |
Venlafaxine |
Mortality = 0.5 μg L−1a |
Developmental abnormality = reduced 0.5 and 10 μg L−1 |
Carbamazepine |
Mortality = 0.5 μg L−1a |
Developmental abnormality = reduced 10 μg L−1 |
Diclofenac |
Proteomics of DNA strand breaks, energy metabolism, oxidative stress response and biomarkers of lipid peroxidation, glutathione transferase (GST) activity |
Blue mussels (Mytilus spp) |
1 and 1000 μg L−1 |
37
|
Gemfibrozil |
Carbamazepine |
AChE activity |
S. vittatum
|
No effect up to 25 mg L−1 |
163
|
Cocktail of 26 PCP including acetaminophen, ciprofloxacin, clarithromycin, clofibrate, ibuprofen, erythromycin |
Ecotoxicity by means of in vitro bioluminescence and respirometry assays |
Vibrio fischeri
|
— |
151
|
Venlafaxine |
Circadian rhythm |
Gambusia holbrooki
|
100 μg L−1 |
164
|
Carbamazepine |
Estrogenicity |
YES |
|
165
|
Interpretation of the results from these studies portend a species-, pathway- and concentration-specific implication. As shown in Table 5, Sousa et al.,32 Damasceno et al.,33 and Klopcic et al.34 investigated different endpoints for diclofenac in different species or in vitro bioassay (evaluating estrogenicity, androgenicity and glucocorticogenicity) as in the case with Klopcic et al.,34 reporting different effective or inhibitory concentrations. Galus et al.36 on other hand evaluated the bio-effect gemfibrozil, venlafaxine and carbamazepine on the same endpoint. Pestana et al.163 showed no effect of carbamazepine exposure in S. vittatum acetylcholinesterase up to a concentration of 25 mg L−1. In contrast to this, 0.5 μg L−1 of carbamazepine induced cell death in zebrafish. This is true for most bioassays but especially in studies where concentration addition effect is not implied. Most bioassays are specific for an endpoint and the concentration addition effect of analyte mixture in the water samples is expressed as BEQ or EEQ of specific reference chemicals making data interpretation with respect to the effective concentration and effect measure universally relevant to some level.
Application of effect-based monitoring assays in assessing chemical pollution and water quality
There is increasing argument for the incorporation of effect-based monitoring of chemical contaminants in assessment of water quality.2,28,90 As evidenced in some of the studies that have been reviewed here (Tables 2–5), these arguments highlight the importance of using effect monitoring to identify possible biological impact of chemical contaminants at environmentally relevant concentration. However, effect based methods have potential applicability that is beyond just detecting pollutants in water samples. A sophisticated application of estrogenicity measurement was observed in the studies by Spina et al.40 and Omoruyi and Pohjanvitra.79 Spina et al.40 employed measurement of estrogenic activity level to assess the efficiency of wastewater treatment by an eco-friendly method involving the use of mutant laccase enzyme from Trametes pubescens. In the same way Omoruyi and Pohjanvitra79 used a yeast bio-reporter assay similar to YES assay to measure the effectiveness of the Helsinki WWTP in water treatment by evaluating estrogenic activities from WWTP influent and effluent samples as well as tap water in residential residences and commercial bottled still and mineral water. By quantifying estrogenicity of WWTP samples before and after the treatment, it was possible to evaluate the water quality status after treatment which translated to the effectiveness of the novel eco-friendly method and the WWTP respectively in wastewater treatment. In the same way, Giebner et al.80 also used the effect measures at different stages of wastewater treatment to determine the percentage removal of chemical waste by quantifying the estrogenicity, anti-estrogenicity, androgenicity and anti-androgenicity at each stages. For instance, anti-estrogenicity of the wastewater was found to reduce from 65% inhibition of the ER from primary treatment of the influent to 39% inhibition of the ER after the second clarifier stage. De Baat et al.166 used a battery of in vitro and in vivo bioassays to test extracts from passive sampling to deduce the associated ecotoxicological risks of metal and organic polar compounds as a ratio the bioanalytical responses observed to the published effect based trigger values to determine location specific contamination. By this, the researchers were able to identify pollution sources in different water bodies, making this strategy a potentially useful application to assess water quality status.
While studies like those of Geraudie et al. (2017) and Ganser et al. (2019) used VTG secretion only to assess feminisation of male fish species or endocrine disruption activities of contaminants,85,133 quantification of VTG level in fish has been applied in the assessment of anthropogenic influence on changes in chemical pollution profile in water bodies. Similar to the application of Spina et al. (2020) as discussed above, Morthorst et al.78 quantified VTG levels in juvenile brown trout over two periods, between 2000 through 2004 and 2010 through 2016 to determine trend of chemical pollution in the river body. It was found that average VTG levels in the fishes between 2000 and 2004 was significantly higher than the quantified level between 2010 and 2016. This was attributed to the reduced estrogenicity of the wastewater as a consequence of the improved wastewater treatment commitment of Danish government.
One of the major reasons for advocating for the implementation of effect-based monitoring of water quality assessment was to address the setbacks of chemical analytical strategies. Chemical analytical techniques are considered to be inadequate to quantify the ever decreasing concentrations of chemical pollutants in the aquatic ecosystem, and some chemicals alone or in mixtures may induce biological effect even at very minimal concentrations that are undetectable by chemical analytical tools. With this in mind, it is advised that a more complete and effective strategy for measuring water quality status is to couple chemical analyses with effect based monitoring.28 While EBM studies often use both chemical analytical tools of different kinds to determine the pollutants within the matrix they investigated in addition to biomonitoring of the pollutants, concentrations of individual chemicals within a mixture are sometimes not reported. This results in a recurring problem: failure to measure individual concentrations of the chemical pollutants will not allow for correlation of the concentrations of these compounds within the mixture with the effects observed. Even when the concentrations are measured, this in addition to the documentation of BEQ or EEQ of the sample extracts as evaluated for specific MOAs (Tables 2–4), does not do justice to identify which of the chemicals within the mixture or which combination of chemicals and at what different concentrations these chemicals are able to produce such effect. This poses the major problem of causality facing the application of effect biomonitoring. To address this, Xia et al. (2020) in a recent study used high throughput transcriptomic approach to monitor the pathways activated by investigating the independent actions and concentration addition effects of single chemicals and chemical cocktails respectively in a concentration dependent manner.167 This strategy was used to create a model that can further predict, using independent actions of single chemicals, the concentration addition effects that is possible for combination of different chemicals that are present in the sample. While this strategy may still be at its infancy, it may be the way forward in consolidating the application of EBM techniques with the concentration effect of single compounds and chemical mixtures in attempt to assess water quality status.
Conclusions and recommendations on adoption of effect-based methods for pharmaceutical and organic chemical application
This review has highlighted that endpoint measurements with the aid of developed bioassays have proven useful in the determination of the biological effects of chemical contaminants in aquatic ecosystems. Although this can translate to possible water quality status, the different bioassays that are possible for one endpoint and the individual variations between any two bioassays will limit the ability to generalise or extrapolate results from different studies for informed decision or policy making. These individual variations include the type of reference chemicals, sensitivity of the bioassay and the type of data reporting format. To overcome this, different bioassays for individual endpoints can be standardised with the same samples and reference chemicals to calculate the sensitivity factor of each bioassay. Such calculated sensitivity factor can be taken into account for any bioassay to have a universally acceptable BEQ/EEQ value of any sample. In addition, bioassays alone are not suitable to attribute observed effect with causative chemicals. As such, there is need to accompany bioassays with quantitative techniques such as such as chromatographic and mass spectrometric techniques will allow identification of specific chemicals in samples and possibility of linking observed effects from field samples with that produced from strategically testing pure and mixture of chemical samples in the lab.
Several studies reviewed here have successfully demonstrated that effect-based monitoring is not limited to assessment of water quality status but also in determining the efficiency of water treatment strategies as well as in the quantification of contaminants in aquatic environments. As noted by Omoruyi and Pohjanvitra,79 the application of bioassay in quantification of analytes may be problematic. Bioassays are only able to measure or quantify bioactive substances, and unable to detect compounds that require metabolism for activity. This however, must be taken into consideration, as is the case with carbamazepine, which has been reported to have five active metabolites that may be detected during wastewater treatment.161 Complementing bioassays with existing quantitative techniques will prevent any omissions.
From the studies reviewed here, it is evident that battery of bioassays evaluating endpoints such as estrogenicity, androgenicity, genotoxicity, aryl hydrocarbon receptor activation/dioxin-like activities and glucocorticogenicity to mention a few are valuable in water quality assessment based on biological activities that are elicited by these chemicals. While grab sampling was more practiced amongst the studies, evidence from studies that employed both sampling methods showed similarities in the effect detected in the samples. This will be provided if appropriate control measures are put in place such as frequency of sampling in grab sampling for instance. However, the use of sampling by POCIS or SPMD may produce different results dependent on the types of analytes picked up by the devices. Employment of either of SPMD and POCIS should thus be driven by carefully designed research question. This is well exemplified by the studies reviewed here. Most of the CEC (Table 1) that were analysed in samples collected by passive method utilised POCIS, which was likely based on the device compatibility with the polar groups present on such chemicals like carbamazepine, ciprofloxacin, diclofenac, erythromycin, sulfamethoxazole and venlafaxine. However, this consideration will not apply to grab sampling and this convenience might explain the reason for most studies employing this sampling method.
Evaluating the biological effect of a single individual chemical rather than mixture of analytes was a theme reviewed here. However, this is an unlikely scenario in real life as contaminated water bodies are often polluted with a mixture of chemicals rather than individual chemicals. While it is informative to know, for instance, the estrogenicity of diclofenac, the contribution of diclofenac amongst a mixture of hundreds of chemicals will be significantly influenced in the presence of these other chemicals such as carbamazepine, trimethoprim, ciprofloxacin, venlafaxine as common in studies reviewed here29,43,45,47,76,85 or any other possible combination of chemical cocktails. In addition, the causality effect of a specific chemical within a mixture of pollutant in a water sample in producing a particular effect is currently impossible with effect-based monitoring. As such, effect-based monitoring of pure samples of chemicals may have to be accompanied by determined, possible or suspected mixture of chemicals to paint the real life scenario.
Ultimately, consistent and robust bio-analytical methods for effect measurement underpin effective determination of bioactivities of pharmaceutical and organic compound contaminants in aquatic fauna. Assay development for biological endpoints is an unending endeavour for targeted and broad-scope effect-based measurement. This is applicable in an interdisciplinary approach to understand pharmaceutical and other organic pollutant activities within surface waters as a measure of water quality status. As toxicological research into biomonitoring of aquatic chemical contaminants continues to grow, standardisation of effect measures that will allow unification of results from different studies that will facilitate informed decision making both at local, continental and global scale remains pertinent.
Conflicts of interest
There are no conflicts to declare.
Acknowledgements
The authors are grateful to the Irish Research Council and the Irish Environmental Protection Agency (EPA Research Grant number 2018-W-MS-36) for financial support.
References
- A. Bielen, A. Šimatović, J. Kosić-Vukšić, I. Senta, M. Ahel, S. Babić, T. Jurina, J. J. González Plaza, M. Milaković and N. Udiković-Kolić, Negative environmental impacts of antibiotic-contaminated effluents from pharmaceutical industries, Water Res., 2017, 126, 79–87 CrossRef CAS PubMed.
- W. Brack, B. I. Escher, E. Müller, M. Schmitt-Jansen, T. Schulze, J. Slobodnik and H. Hollert, Towards a holistic and solution-oriented monitoring of chemical status of European water bodies: how to support the EU strategy for a non-toxic environment?, Environ. Sci. Eur., 2018, 30, 33 CrossRef PubMed.
- D. J. Lapworth, N. Baran, M. E. Stuart and R. S. Ward, Emerging organic contaminants in groundwater: A review of sources, fate and occurrence, Environ. Pollut., 2012, 163, 287–303 CrossRef CAS PubMed.
- R. Loos, R. Carvalho, D. C. António, S. Comero, G. Locoro, S. Tavazzi, B. Paracchini, M. Ghiani, T. Lettieri, L. Blaha, B. Jarosova, S. Voorspoels, K. Servaes, P. Haglund, J. Fick, R. H. Lindberg, D. Schwesig and B. M. Gawlik, EU-wide monitoring survey on emerging polar organic contaminants in wastewater treatment plant effluents, Water Res., 2013, 47, 6475–6487 CrossRef CAS PubMed.
-
E. Miljøagentur, European waters – assessment of status and pressures 2018, European Environment Agency, 2018 Search PubMed.
-
EFPIA, The Pharmaceutical Industry in Figures: Key Data, The European Federation of Pharmaceutical Industries and Associations, 2019 Search PubMed.
- D. J. Lapworth, B. Lopez, V. Laabs, R. Kozel, R. Wolter, R. Ward, E. V. Amelin, T. Besien, J. Claessens, F. Delloye, E. Ferretti and J. Grath, Developing a groundwater watch list for substances of emerging concern: a European perspective, Environ. Res. Lett., 2019, 14, 035004 CrossRef.
- L. Carvalho, E. B. Mackay, A. C. Cardoso, A. Baattrup-Pedersen, S. Birk, K. L. Blackstock, G. Borics, A. Borja, C. K. Feld and M. T. Ferreira, Protecting and restoring Europe's waters: an analysis of the future development needs of the Water Framework Directive, Sci. Total Environ., 2019, 658, 1228–1238 CrossRef CAS PubMed.
-
M. Pulido-Velazquez and F. Ward, in Competition for Water Resources, Elsevier, 2017, pp. 423–441 Search PubMed.
- V. Armstrong, N. A. Karyakina, E. Nordheim, I. Arnold and D. Krewski, Intent and Provision of REACH: an Overview, Neurotoxicology, 2021, 83, 186–198 CrossRef CAS PubMed.
-
T. Lettieri, D. Napierska, R. Loos, D. Marinov and I. Sanseverino, European Commission and Joint Research Centre, Review of the 1st Watch List under the Water Framework Directive and Recommendations for the 2nd Watch List, European Commission: Luxembourg, DOI: DOI:10.2760/701879.
- M. J. Parnham, V. Erakovic Haber, E. J. Giamarellos-Bourboulis, G. Perletti, G. M. Verleden and R. Vos, Azithromycin: mechanisms of action and their relevance for clinical applications, Pharmacol. Ther., 2014, 143, 225–245 CrossRef CAS PubMed.
- T. J. Gan, Diclofenac: an update on its mechanism of action and safety profile, Curr. Med. Res. Opin., 2010, 26, 1715–1731 CrossRef CAS PubMed.
- J. Alarcón-Millán, G. Fernández-Tilapa, E. M. Cortés-Malagón, C. A. Castañón-Sánchez, J. De Sampedro-Reyes, I. Cruz-Del Carmen, R. Betancourt-Linares and A. Román-Román, Clarithromycin resistance and prevalence of Helicobacter pylori virulent genotypes in patients from Southern México with chronic gastritis, Infect., Genet. Evol., 2016, 44, 190–198 CrossRef PubMed.
- T. Gaillard, J. Dormoi, M. Madamet and B. Pradines, Macrolides and associated antibiotics based on similar mechanism of action like lincosamides in malaria, Malar J., 2016, 15, 85 CrossRef PubMed.
- D. J. Weber, N. E. Tolkoff-Rubin and R. H. Rubin, Amoxicillin and potassium clavulanate: an antibiotic combination. Mechanism of action, pharmacokinetics, antimicrobial spectrum, clinical efficacy and adverse effects, Pharmacotherapy, 1984, 4, 122–136 CrossRef CAS PubMed.
- E. C. L. Cazedey and H. R. N. Salgado, A novel and rapid microbiological assay for ciprofloxacin hydrochloride, J. Pharm. Anal., 2013, 3, 382–386 CrossRef CAS PubMed.
- P. A. Masters, T. A. O'Bryan, J. Zurlo, D. Q. Miller and N. Joshi, Trimethoprim-sulfamethoxazole revisited, Arch. Intern. Med., 2003, 163, 402–410 CrossRef CAS PubMed.
- L. C. Chio, L. A. Bolyard, M. Nasr and S. F. Queener, Identification of a class of sulfonamides highly active against dihydropteroate synthase form Toxoplasma gondii, Pneumocystis carinii, and Mycobacterium avium, Antimicrob. Agents Chemother., 1996, 40, 727–733 CrossRef CAS PubMed.
- J. Gierbolini, M. Giarratano and S. R. Benbadis, Carbamazepine-related antiepileptic drugs for the treatment of epilepsy – a comparative review, Expert Opin. Pharmacother., 2016, 17, 885–888 CrossRef PubMed.
- K. Kim, H. K. Kleinman, H.-J. Lee and K. Pahan, Safety and potential efficacy of gemfibrozil as a supportive treatment for children with late infantile neuronal ceroid lipofuscinosis and other lipid storage disorders, Orphanet J Rare Dis, 2017, 12, 113 CrossRef PubMed.
- M. A. Saad, A. E. El-Sahar, R. H. Sayed, E. M. Elbaz, H. S. Helmy and M. A. Senousy, Venlafaxine Mitigates Depressive-Like Behavior in Ovariectomized Rats by Activating the EPO/EPOR/JAK2 Signaling Pathway and Increasing the Serum Estradiol Level, Neurotherapeutics, 2019, 16, 404–415 CrossRef CAS PubMed.
- A. Akhmouch, D. Clavreul and P. Glas, Introducing the OECD Principles on Water Governance, Water International, 2018, 43, 5–12 CrossRef.
- W. Brack, V. Dulio, M. Ågerstrand, I. Allan, R. Altenburger, M. Brinkmann, D. Bunke, R. M. Burgess, I. Cousins, B. I. Escher, F. J. Hernández, L. M. Hewitt, K. Hilscherová, J. Hollender, H. Hollert, R. Kase, B. Klauer, C. Lindim, D. L. Herráez, C. Miège, J. Munthe, S. O'Toole, L. Posthuma, H. Rüdel, R. B. Schäfer, M. Sengl, F. Smedes, D. van de Meent, P. J. van den Brink, J. van Gils, A. P. van Wezel, A. D. Vethaak, E. Vermeirssen, P. C. von der Ohe and B. Vrana, Towards the review of the European Union Water Framework Directive: Recommendations for more efficient assessment and management of chemical contamination in European surface water resources, Sci. Total Environ., 2017, 576, 720–737 CrossRef CAS PubMed.
- R. Altenburger, M. Scholze, W. Busch, B. I. Escher, G. Jakobs, M. Krauss, J. Krüger, P. A. Neale, S. Ait-Aissa, A. C. Almeida, T.-B. Seiler, F. Brion, K. Hilscherová, H. Hollert, J. Novák, R. Schlichting, H. Serra, Y. Shao, A. Tindall, K. E. Tollefsen, G. Umbuzeiro, T. D. Williams and A. Kortenkamp, Mixture effects in samples of multiple contaminants - An inter-laboratory study with manifold bioassays, Environ. Int., 2018, 114, 95–106 CrossRef CAS PubMed.
- V. Dulio, B. van Bavel, E. Brorström-Lundén, J. Harmsen, J. Hollender, M. Schlabach, J. Slobodnik, K. Thomas and J. Koschorreck, Emerging pollutants in the EU: 10 years of NORMAN in support of environmental policies and regulations, Environ. Sci.
Eur., 2018, 30, 5 CrossRef PubMed.
- A.-S. Wernersson, M. Carere, C. Maggi, P. Tusil, P. Soldan, A. James, W. Sanchez, V. Dulio, K. Broeg, G. Reifferscheid, S. Buchinger, H. Maas, E. Van Der Grinten, S. O'Toole, A. Ausili, L. Manfra, L. Marziali, S. Polesello, I. Lacchetti, L. Mancini, K. Lilja, M. Linderoth, T. Lundeberg, B. Fjällborg, T. Porsbring, D. J. Larsson, J. Bengtsson-Palme, L. Förlin, C. Kienle, P. Kunz, E. Vermeirssen, I. Werner, C. D. Robinson, B. Lyons, I. Katsiadaki, C. Whalley, K. den Haan, M. Messiaen, H. Clayton, T. Lettieri, R. N. Carvalho, B. M. Gawlik, H. Hollert, C. Di Paolo, W. Brack, U. Kammann and R. Kase, The European technical report on aquatic effect-based monitoring tools under the water framework directive, Environ. Sci. Eur., 2015, 27, 7 CrossRef.
- W. Brack, S. A. Aissa, T. Backhaus, V. Dulio, B. I. Escher, M. Faust, K. Hilscherova, J. Hollender, H. Hollert, C. Müller, J. Munthe, L. Posthuma, T.-B. Seiler, J. Slobodnik, I. Teodorovic, A. J. Tindall, G. de Aragão Umbuzeiro, X. Zhang and R. Altenburger, Effect-based methods are key. The European Collaborative Project SOLUTIONS recommends integrating effect-based methods for diagnosis and monitoring of water quality, Environ. Sci. Eur., 2019, 31, 10 CrossRef.
- C. Liscio, A. Abdul-Sada, R. Al-Salhi, M. H. Ramsey and E. M. Hill, Methodology for profiling anti-androgen mixtures in river water using multiple passive samplers and bioassay-directed analyses, Water Res., 2014, 57, 258–269 CrossRef CAS PubMed.
- C. Kienle, E. L. M. Vermeirssen, A. Schifferli, H. Singer, C. Stamm and I. Werner, Effects of treated wastewater on the ecotoxicity of small streams - Unravelling the contribution of chemicals causing effects, PLoS One, 2019, 14, e0226278 CrossRef CAS PubMed.
- A. K. Rosenmai, J. Lundqvist, P. Gago-Ferrero, G. Mandava, L. Ahrens, K. Wiberg and A. Oskarsson, Effect-based assessment of recipient waters impacted by on-site, small scale, and large scale waste water treatment facilities - combining passive sampling with in vitro bioassays and chemical analysis, Sci. Rep., 2018, 8, 17200 CrossRef PubMed.
- B. Sousa, J. Lopes, A. Leal, M. Martins, C. Soares, I. M. Valente, J. A. Rodrigues, F. Fidalgo and J. Teixeira, Response of Solanum lycopersicum L. to diclofenac – Impacts on the plant's antioxidant mechanisms, Environ. Pollut., 2020, 258, 113762 CrossRef CAS PubMed.
- L. L. Damasceno de Oliveira, B. Nunes, S. C. Antunes, R. Campitelli-Ramos and O. Rocha, Acute and Chronic Effects of Three Pharmaceutical Drugs on the Tropical Freshwater Cladoceran Ceriodaphnia silvestrii, Water, Air, Soil Pollut., 2018, 229, 116 CrossRef.
- I. Klopčič, T. Markovič, I. Mlinarič-Raščan and M. Sollner Dolenc, Endocrine disrupting activities and immunomodulatory effects in lymphoblastoid cell lines of diclofenac, 4-hydroxydiclofenac and paracetamol, Toxicol. Lett., 2018, 294, 95–104 CrossRef PubMed.
- M. Mezzelani, S. Gorbi, Z. Da Ros, D. Fattorini, G. d'Errico, M. Milan, L. Bargelloni and F. Regoli, Ecotoxicological potential of non-steroidal anti-inflammatory drugs (NSAIDs) in marine organisms: Bioavailability, biomarkers and natural occurrence in Mytilus galloprovincialis, Mar. Environ. Res., 2016, 121, 31–39 CrossRef CAS PubMed.
- M. Galus, N. Kirischian, S. Higgins, J. Purdy, J. Chow, S. Rangaranjan, H. Li, C. Metcalfe and J. Y. Wilson, Chronic, low concentration exposure to pharmaceuticals impacts multiple organ systems in zebrafish, Aquat. Toxicol., 2013, 132–133, 200–211 CrossRef CAS PubMed.
- W. Schmidt, L.-C. Rainville, G. Mceneff, D. Sheehan and B. Quinn, A proteomic evaluation of the effects of the pharmaceuticals diclofenac and gemfibrozil on marine mussels (Mytilus spp.): evidence for chronic sublethal effects on stress-response proteins, Drug Test. Anal., 2014, 6, 210–219 CrossRef CAS PubMed.
- W. Busch, S. Schmidt, R. Kühne, T. Schulze, M. Krauss and R. Altenburger, Micropollutants in European rivers: a mode of action survey to support the development of effect-based tools for water monitoring, Environ. Toxicol. Chem., 2016, 35, 1887–1899 CrossRef CAS PubMed.
- P. Cocci, F. A. Palermo, L. Quassinti, M. Bramucci, A. Miano and G. Mosconi, Determination of estrogenic activity in the river Chienti (Marche Region, Italy) by using in vivo and in vitro bioassays, J. Environ. Sci., 2016, 43, 48–53 CrossRef CAS PubMed.
- F. Spina, M. Gea, C. Bicchi, C. Cordero, T. Schiliro and G. C. Varese, Ecofriendly laccases treatment to challenge micropollutants issue in municipal wastewaters, Environ. Pollut., 2020, 257, 113579 CrossRef CAS PubMed.
- T. Hamers, J. Legradi, N. Zwart, F. Smedes, J. de Weert, E.-J. van den Brandhof, D. van de Meent and D. de Zwart, Time-Integrative Passive sampling combined with TOxicity Profiling (TIPTOP): an effect-based strategy for cost-effective chemical water quality assessment, Environ. Toxicol. Pharmacol., 2018, 64, 48–59 CrossRef CAS PubMed.
- D. Maier, L. Blaha, J. P. Giesy, A. Henneberg, H.-R. Kohler, B. Kuch, R. Osterauer, K. Peschke, D. Richter, M. Scheurer and R. Triebskorn, Biological plausibility as a tool to associate analytical data for micropollutants and effect potentials in wastewater, surface water, and sediments with effects in fishes, Water Res., 2015, 72, 127–144 CrossRef CAS PubMed.
- B. Jarosova, A. Ersekova, K. Hilscherova, R. Loos, B. M. Gawlik, J. P. Giesy and L. Blaha, Europe-wide survey of estrogenicity in wastewater treatment plant effluents: the need for the effect-based monitoring, Environ. Sci. Pollut. Res. Int., 2014, 21, 10970–10982 CrossRef CAS PubMed.
- M. Konig, B. I. Escher, P. A. Neale, M. Krauss, K. Hilscherova, J. Novak, I. Teodorovic, T. Schulze, S. Seidensticker, M. A. Kamal Hashmi, J. Ahlheim and W. Brack, Impact of untreated wastewater on a major European river evaluated with a combination of in vitro bioassays and chemical analysis, Environ. Pollut., 2017, 220, 1220–1230 CrossRef PubMed.
- V. Jalova, B. Jarosova, L. Blaha, J. P. Giesy, T. Ocelka, R. Grabic, J. Jurcikova, B. Vrana and K. Hilscherova, Estrogen-, androgen- and aryl hydrocarbon receptor mediated activities in passive and composite samples from municipal waste and surface waters, Environ. Int., 2013, 59, 372–383 CrossRef CAS PubMed.
- T. Smital, S. Terzic, R. Zaja, I. Senta, B. Pivcevic, M. Popovic, I. Mikac, K. E. Tollefsen, K. V. Thomas and M. Ahel, Assessment of toxicological profiles of the municipal wastewater effluents using chemical analyses and bioassays, Ecotoxicol. Environ. Saf., 2011, 74, 844–851 CrossRef CAS PubMed.
- Z. Tousova, J. Froment, P. Oswald, J. Slobodnik, K. Hilscherova, K. V. Thomas, K. E. Tollefsen, M. Reid, K. Langford and L. Blaha, Identification
of algal growth inhibitors in treated waste water using effect-directed analysis based on non-target screening techniques, J. Hazard. Mater., 2018, 358, 494–502 CrossRef CAS PubMed.
- M. Long, J. Strand, P. Lassen, T. Kruger, I. Dahllof, R. Bossi, M. M. Larsen, P. Wiberg-Larsen and E. C. Bonefeld-Jorgensen, Endocrine-disrupting effects of compounds in Danish streams, Arch. Environ. Contam. Toxicol., 2014, 66, 1–18 CrossRef PubMed.
-
J. L. Campbell, M. Yoon and H. J. Clewell, A case study on quantitative in vitro to in vivo extrapolation for environmental esters: methyl-, propyl- and butylparaben, Jun 2015, vol. 332, pp. 67-76 Search PubMed.
- G. Bertanza, M. Papa, R. Pedrazzani, C. Repice, G. Mazzoleni, N. Steimberg, D. Feretti, E. Ceretti and I. Zerbini, EDCs, estrogenicity and genotoxicity reduction in a mixed (domestic + textile) secondary effluent by means of ozonation: a full-scale experience, Sci. Total Environ., 2013, 458–460, 160–168 CrossRef PubMed.
- B. Wetterauer, M. Ricking, J. C. Otte, A. V. Hallare, A. Rastall, L. Erdinger, J. Schwarzbauer, T. Braunbeck and H. Hollert, Toxicity, dioxin-like activities, and endocrine effects of DDT metabolites-DDA, DDMU, DDMS, and DDCN, Environ. Sci. Pollut. Res. Int., 2012, 19, 403–415 CrossRef PubMed.
- S. Nadzialek, S. Depiereux, S. N. M. Mandiki and P. Kestemont, In vivo biomarkers of estrogenicity: limitation of interpretation in wild environment, Arch. Environ. Contam. Toxicol., 2011, 60, 471–478 CrossRef PubMed.
- K. D. Daniels, D. VanDervort, S. Wu, F. D. L. Leusch, J. P. van de Merwe, A. Jia and S. A. Snyder, Downstream trends of in vitro bioassay responses in a wastewater effluent-dominated river, Chemosphere, 2018, 212, 182–192 CrossRef PubMed.
- R. Quiroz, J. O. Grimalt and P. Fernandez, Toxicity assessment of polycyclic aromatic hydrocarbons in sediments from European high mountain lakes, Ecotoxicol. Environ. Saf., 2010, 73, 559–564 CrossRef PubMed.
- N. Creusot, N. Tapie, B. Piccini, P. Balaguer, J.-M. Porcher, H. Budzinski and S. Ait-Aissa, Distribution of steroid- and dioxin-like activities between sediments, POCIS and SPMD in a French river subject to mixed pressures, Environ. Sci. Pollut. Res. Int., 2013, 20, 2784–2794 CrossRef CAS PubMed.
- M. L. De Baat, M. H. S. Kraak, R. Van der Oost, P. De Voogt and P. F. M. Verdonschot, Effect-based nationwide surface water quality assessment to identify ecotoxicological risks, Water Res., 2019, 159, 434–443 CrossRef CAS PubMed.
- X. Y. Ma, Y. Wang, K. Dong, X. C. Wang, K. Zheng, L. Hao and H. H. Ngo, The treatability of trace organic pollutants in WWTP effluent and associated biotoxicity reduction by advanced treatment processes for effluent quality improvement, Water Res., 2019, 159, 423–433 CrossRef CAS PubMed.
- A. C. Rastall, D. Getting, J. Goddard, D. R. Roberts and L. Erdinger, A biomimetic approach to the detection and identification of estrogen receptor agonists in surface waters using semipermeable membrane devices (SPMDs) and bioassay-directed chemical analysis, Environ. Sci. Pollut. Res. Int., 2006, 13, 256–267 CrossRef CAS PubMed.
- M. Schriks, S. C. van der Linden, P. G. M. Stoks, B. van der Burg, L. Puijker, P. de Voogt and M. B. Heringa, Occurrence of glucocorticogenic activity in various surface waters in The Netherlands, Chemosphere, 2013, 93, 450–454 CrossRef CAS PubMed.
- K. Zhang, Y. Zhao and K. Fent, Occurrence and Ecotoxicological Effects of Free, Conjugated, and Halogenated Steroids Including 17alpha-Hydroxypregnanolone and Pregnanediol in Swiss Wastewater and Surface Water, Environ. Sci. Technol., 2017, 51, 6498–6506 CrossRef CAS PubMed.
- R. Turja, K. K. Lehtonen, A. Meierjohann, J.-M. Brozinski, E. Vahtera, A. Soirinsuo, A. Sokolov, P. Snoeijs, H. Budzinski, M.-H. Devier, L. Peluhet, J.-P. Paakkonen, M. Viitasalo and L. Kronberg, The mussel caging approach in assessing biological effects of wastewater treatment plant discharges in the Gulf of Finland (Baltic Sea), Mar. Pollut. Bull., 2015, 97, 135–149 CrossRef CAS PubMed.
- F. D. L. Leusch, P. A. Neale, C. Arnal, N. H. Aneck-Hahn, P. Balaguer, A. Bruchet, B. I. Escher, M. Esperanza, M. Grimaldi, G. Leroy, M. Scheurer, R. Schlichting, M. Schriks and A. Hebert, Analysis of endocrine activity in drinking water, surface water and treated wastewater from six countries, Water Res., 2018, 139, 10–18 CrossRef CAS PubMed.
- N. Zwart, W. Jonker, R. T. Broek, J. de Boer, G. Somsen, J. Kool, T. Hamers, C. J. Houtman and M. H. Lamoree, Identification of mutagenic and endocrine disrupting compounds in surface water and wastewater treatment plant effluents using high-resolution effect-directed analysis, Water Res., 2020, 168, 115204 CrossRef CAS PubMed.
- S. Yue, T. Zhang, Q. Shen, Q. Song, C. Ji, Y. Chen, M. Mao, Y. Kong, D. Chen, J. Liu, Z. Sun and M. Zhao, Assessment of endocrine-disrupting effects of emerging polyhalogenated carbazoles (PHCZs): In vitro, in silico, and in vivo evidence, Environ. Int., 2020, 140, 105729 CrossRef CAS PubMed.
- K. T. Ho, I. M. Konovets, A. V. Terletskaya, M. V. Milyukin, A. V. Lyashenko, L. I. Shitikova, L. I. Shevchuk, S. A. Afanasyev, Y. G. Krot, K. Y. Zorina-Sakharova, V. V. Goncharuk, M. M. Skrynnyk, M. A. Cashman and R. M. Burgess, Contaminants, mutagenicity and toxicity in the surface waters of Kyiv, Ukraine, Mar. Pollut. Bull., 2020, 155, 111153 CrossRef CAS PubMed.
- D. Feretti, M. Acito, M. Dettori, E. Ceretti, C. Fatigoni, S. Posadino, I. Zerbini, M. Villarini, M. Moretti, P. Castiglia and A. Azara, Genotoxicity of source, treated and distributed water from four drinking water treatment plants supplied by surface water in Sardinia, Italy, Environ. Res., 2020, 185, 109385 CrossRef CAS PubMed.
- X. Wang, G. Zhao, H. Wang, J. Liang, S. Xu, S. Chen, A. Xu and L. Wu, Assessment of the cytotoxic and mutagenic potential of the Jialu River and adjacent groundwater using human-hamster hybrid cells, J. Environ. Sci., 2018, 70, 133–143 CrossRef PubMed.
- H. Arambourou, R. Planello, L. Llorente, I. Fuertes, C. Barata, N. Delorme, P. Noury, O. Herrero, A. Villeneuve and C. Bonnineau, Chironomus riparius exposure to field-collected contaminated sediments: From subcellular effect to whole-organism response, Sci. Total Environ., 2019, 671, 874–882 CrossRef CAS PubMed.
- J. R. Loughery, J. R. Marentette, R. A. Frank, L. M. Hewitt, J. L. Parrott and C. J. Martyniuk, Transcriptome Profiling in Larval Fathead Minnow Exposed to Commercial Naphthenic Acids and Extracts from Fresh and Aged Oil Sands Process-Affected Water, Environ. Sci. Technol., 2019, 53, 10435–10444 CrossRef CAS PubMed.
- E. M. Happel, T. Markussen, J. E. Teikari, V. Huchaiah, J. Alneberg, A. F. Andersson, K. Sivonen, M. Middelboe, V. Kisand and L. Riemann, Effects of allochthonous dissolved organic matter input on microbial composition and nitrogen-cycling genes at two contrasting estuarine sites, FEMS Microbiol. Ecol., 2019, 95(9), fiz123 CrossRef CAS PubMed.
- S. Zhou, Z. Wei, T. Chu, H. Yu, S. Li, W. Zhang and W. Gui, Transcriptomic analysis of zebrafish (Danio rerio) embryos to assess integrated biotoxicity
of Xitiaoxi River waters, Environ. Pollut., 2018, 242, 42–53 CrossRef CAS PubMed.
- N. Casatta, F. Stefani and L. Viganò, Hepatic gene expression profiles of a non-model cyprinid (Barbus plebejus) chronically exposed to river sediments, Comp. Biochem. Physiol., Part C: Toxicol. Pharmacol., 2017, 196, 27–35 CAS.
- F. Stefani, N. Casatta, C. Ferrarin, A. Izzotti, F. Maicu and L. Viganò, Gene expression and genotoxicity in Manila clam (Ruditapes philippinarum) modulated by sediment contamination and lagoon dynamics in the Po river delta, Mar. Environ. Res., 2018, 142, 257–274 CrossRef CAS PubMed.
- I. A. Rodriguez-Jorquera, K. J. Kroll, G. S. Toor and N. D. Denslow, Transcriptional and physiological response of fathead minnows (Pimephales promelas) exposed to urban waters entering into wildlife protected areas, Environ. Pollut., 2015, 199, 155–165 CrossRef CAS PubMed.
- V. Lorenzi, A. C. Mehinto, N. D. Denslow and D. Schlenk, Effects of exposure to the β-blocker propranolol on the reproductive behavior and gene expression of the fathead minnow, Pimephales promelas, Aquat. Toxicol., 2012, 116–117, 8–15 CrossRef CAS PubMed.
- L. Palli, F. Spina, G. C. Varese, M. Vincenzi, M. Aragno, G. Arcangeli, N. Mucci, D. Santianni, S. Caffaz and R. Gori, Occurrence of selected pharmaceuticals in wastewater treatment plants of Tuscany: An effect-based approach to evaluate the potential environmental impact, Int. J. Hyg. Environ. Health, 2019, 222, 717–725 CrossRef CAS PubMed.
- W. Guo, K. Van Langenhove, T. Vandermarken, M. S. Denison, M. Elskens, W. Baeyens and Y. Gao, In situ measurement of estrogenic activity in various aquatic systems using organic diffusive gradients in thin-film coupled with ERE-CALUX bioassay, Environ. Int., 2019, 127, 13–20 CrossRef CAS PubMed.
- J. E. Morthorst, K. K. Mathiesen, H. Holbech, K. L. Pedersen and P. Bjerregaard, Vitellogenin concentrations in feral Danish brown trout have decreased: An effect of improved sewage treatment in rural areas?, Environ. Toxicol. Chem., 2018, 37, 839–845 CrossRef CAS PubMed.
- I. M. Omoruyi and R. Pohjanvirta, Estrogenic activity of wastewater, bottled waters and tap water in Finland as assessed by a yeast bio-reporter assay, Scand J Public Health, 2015, 43, 770–775 CrossRef PubMed.
- S. Giebner, S. Ostermann, S. Straskraba, M. Oetken, J. Oehlmann and M. Wagner, Effectivity of advanced wastewater treatment: reduction of in vitro endocrine activity and mutagenicity but not of in vivo reproductive toxicity, Environ. Sci. Pollut. Res. Int., 2018, 25, 3965–3976 CrossRef CAS PubMed.
- P. Valitalo, N. Perkola, T.-B. Seiler, M. Sillanpaa, J. Kuckelkorn, A. Mikola, H. Hollert and E. Schultz, Estrogenic activity in Finnish municipal wastewater effluents, Water Res., 2016, 88, 740–749 CrossRef CAS PubMed.
- C. Rivetti, J. J. Lopez-Perea, C. Laguna, B. Pina, R. Mateo, E. Eljarrat, D. Barcelo and C. Barata, Integrated environmental risk assessment of chemical pollution in a Mediterranean floodplain by combining chemical and biological methods, Sci. Total Environ., 2017, 583, 248–256 CrossRef CAS PubMed.
- T. Schiliro, A. Porfido, F. Spina, G. C. Varese and G. Gilli, Oestrogenic activity of a textile industrial wastewater treatment plant effluent evaluated by the E-screen test and MELN gene-reporter luciferase assay, Sci. Total Environ., 2012, 432, 389–395 CrossRef CAS PubMed.
- Y. Schindler Wildhaber, H. Mestankova, M. Schärer, K. Schirmer, E. Salhi and U. von Gunten, Novel test procedure to evaluate the treatability of wastewater with ozone, Water Res., 2015, 75, 324–335 CrossRef CAS PubMed.
- B. Ganser, M. Bundschuh, I. Werner, N. Homazava, E. L. M. Vermeirssen, C. Moschet and C. Kienle, Wastewater alters feeding rate but not vitellogenin level of Gammarus fossarum (Amphipoda), Sci. Total Environ., 2019, 657, 1246–1252 CrossRef CAS PubMed.
- P. Valitalo, R. Massei, I. Heiskanen, P. Behnisch, W. Brack, A. J. Tindall, D. Du Pasquier, E. Kuster, A. Mikola, T. Schulze and M. Sillanpaa, Effect-based assessment of toxicity removal during wastewater treatment, Water Res., 2017, 126, 153–163 CrossRef CAS PubMed.
- K. O. Kusk, T. Kruger, M. Long, C. Taxvig, A. E. Lykkesfeldt, H. Frederiksen, A.-M. Andersson, H. R. Andersen, K. M. S. Hansen, C. Nellemann and E. C. Bonefeld-Jorgensen, Endocrine potency of wastewater: contents of endocrine disrupting chemicals and effects measured by in vivo and in vitro assays, Environ. Toxicol. Chem., 2011, 30, 413–426 CrossRef CAS PubMed.
- B. Jarosova, L. Blaha, B. Vrana, T. Randak, R. Grabic, J. P. Giesy and K. Hilscherova, Changes in concentrations of hydrophilic organic contaminants and of endocrine-disrupting potential downstream of small communities located adjacent to headwaters, Environ. Int., 2012, 45, 22–31 CrossRef CAS PubMed.
- P. Berckmans, H. Leppens, C. Vangenechten and H. Witters, Screening of endocrine disrupting chemicals with MELN cells, an ER-transactivation assay combined with cytotoxicity assessment, Toxicol. In Vitro, 2007, 21, 1262–1267 CrossRef CAS PubMed.
- C. Di Paolo, R. Ottermanns, S. Keiter, S. Ait-Aissa, K. Bluhm, W. Brack, M. Breitholtz, S. Buchinger, M. Carere and C. Chalon, Bioassay battery interlaboratory investigation of emerging contaminants in spiked water extracts–towards the implementation of bioanalytical monitoring tools in water quality assessment and monitoring, Water Res., 2016, 104, 473–484 CrossRef CAS PubMed.
- S. Schmitt, G. Reifferscheid, E. Claus, M. Schlusener and S. Buchinger, Effect directed analysis and mixture effects of estrogenic compounds in a sediment of the river Elbe, Environ. Sci. Pollut. Res. Int., 2012, 19, 3350–3361 CrossRef CAS PubMed.
- A. D. Vethaak, T. Hamers, C. Martínez-Gómez, J. H. Kamstra, J. de Weert, P. E. Leonards and F. Smedes, Toxicity profiling of marine surface sediments: a case study using rapid screening bioassays of exhaustive total extracts, elutriates and passive sampler extracts, Mar. Environ. Res., 2017, 124, 81–91 CrossRef CAS PubMed.
- H. Serra, F. Brion, C. Chardon, H. Budzinski, T. Schulze, W. Brack and S. Aït-Aïssa, Estrogenic activity of surface waters using zebrafish- and human-based in vitro assays: The Danube as a case-study, Environ. Toxicol. Pharmacol., 2020, 78, 103401 CrossRef CAS PubMed.
- C. J. Houtman, J. Kroesbergen, K. Lekkerkerker-Teunissen and J. P. van der Hoek, Human health risk assessment of the mixture of pharmaceuticals in Dutch drinking water and its sources based on frequent monitoring data, Sci. Total Environ., 2014, 496, 54–62 CrossRef CAS PubMed.
- E. Simon, A. Schifferli, T. B. Bucher, D. Olbrich, I. Werner and E. L. M. Vermeirssen, Solid-phase extraction of estrogens and herbicides from environmental waters for bioassay analysis-effects of sample volume on recoveries, Anal. Bioanal. Chem., 2019, 411, 2057–2069 CrossRef CAS PubMed.
- N. A. Alygizakis, H. Besselink, G. K. Paulus, P. Oswald, L. M. Hornstra, M. Oswaldova, G. Medema, N. S. Thomaidis, P. A. Behnisch and J. Slobodnik, Characterization of wastewater effluents in the Danube River Basin with chemical screening, in vitro bioassays and antibiotic resistant genes analysis, Environ. Int., 2019, 127, 420–429 CrossRef PubMed.
- T. Vandermarken, K. Croes, K. Van Langenhove, I. Boonen, P. Servais, T. Garcia-Armisen, N. Brion, M. S. Denison, L. Goeyens and M. Elskens, Endocrine activity in an urban river system and the biodegradation of estrogen-like endocrine disrupting chemicals through a bio-analytical approach using DRE- and ERE-CALUX bioassays, Chemosphere, 2018, 201, 540–549 CrossRef PubMed.
- M. Muschket, C. Di Paolo, A. J. Tindall, G. Touak, A. Phan, M. Krauss, K. Kirchner, T.-B. Seiler, H. Hollert and W. Brack, Identification of Unknown Antiandrogenic Compounds in Surface Waters by Effect-Directed Analysis (EDA) Using a Parallel Fractionation Approach, Environ. Sci. Technol., 2018, 52, 288–297 CrossRef PubMed.
- M. Avberšek, B. Žegura, M. Filipič, N. Uranjek-Ževart and E. Heath, Determination of estrogenic potential in waste water without sample extraction, J. Hazard. Mater., 2013, 260, 527–533 CrossRef PubMed.
- J. Sun, X. Ji, R. Zhang, Y. Huang, Y. Liang, J. Du, X. Xie and A. Li, Endocrine disrupting compounds reduction and water quality improvement in reclaimed municipal wastewater: A field-scale study along Jialu River in North China, Chemosphere, 2016, 157, 232–240 CrossRef PubMed.
- A. Henneberg, K. Bender, L. Blaha, S. Giebner, B. Kuch, H.-R. Köhler, D. Maier, J. Oehlmann, D. Richter, M. Scheurer, U. Schulte-Oehlmann, A. Sieratowicz, S. Ziebart and R. Triebskorn, Are in vitro methods for the detection of endocrine potentials in the aquatic environment predictive for in vivo effects? Outcomes of the Projects SchussenAktiv and SchussenAktivplus in the Lake Constance Area, Germany, PLoS One, 2014, 9, e98307 CrossRef PubMed.
- J. C. Otte, S. Keiter, C. Faßbender, E. B. Higley, P. S. Rocha, M. Brinkmann, D.-S. Wahrendorf, W. Manz, M. A. Wetzel, T. Braunbeck, J. P. Giesy, M. Hecker and H. Hollert, Contribution of priority PAHs and POPs to Ah receptor-mediated activities in sediment samples from the River Elbe Estuary, Germany, PLoS One, 2013, 8, e75596 CrossRef CAS PubMed.
- B. I. Escher, S. Aїt-Aїssa, P. A. Behnisch, W. Brack, F. Brion, A. Brouwer, S. Buchinger, S. E. Crawford, D. Du Pasquier, T. Hamers, K. Hettwer, K. Hilscherová, H. Hollert, R. Kase, C. Kienle, A. J. Tindall, J. Tuerk, R. van der Oost, E. Vermeirssen and P. A. Neale, Effect-based trigger values for in vitro and in vivo bioassays performed on surface water extracts supporting the environmental quality standards (EQS) of the European Water Framework Directive, Sci. Total Environ., 2018, 628–629, 748–765 CrossRef CAS PubMed.
- P. Y. Kunz, E. Simon, N. Creusot, B. S. Jayasinghe, C. Kienle, S. Maletz, A. Schifferli, C. Schönlau, S. Aït-Aïssa, N. D. Denslow, H. Hollert, I. Werner and E. L. M. Vermeirssen, Effect-based tools for monitoring estrogenic mixtures: Evaluation of five in vitro bioassays, Water Res., 2017, 110, 378–388 CrossRef CAS PubMed.
- H. Serra, M. Scholze, R. Altenburger, W. Busch, H. Budzinski, F. Brion and S. Aït-Aïssa, Combined effects of environmental xeno-estrogens within multi-component mixtures: Comparison of in vitro human- and zebrafish-based estrogenicity bioassays, Chemosphere, 2019, 227, 334–344 CrossRef CAS PubMed.
- F. Brion, V. De Gussem, S. Buchinger, H. Hollert, M. Carere, J.-M. Porcher, B. Piccini, C. Féray, V. Dulio, S. Könemann, E. Simon, I. Werner, R. Kase and S. Aït-Aïssa, Monitoring estrogenic activities of waste and surface waters using a novel in vivo zebrafish embryonic (EASZY) assay: Comparison with in vitro cell-based assays and determination of effect-based trigger values, Environ. Int., 2019, 130, 104896 CrossRef CAS PubMed.
- D. B. Pickford, A. Jones, A. Velez-Pelez, F. Orton, T. Iguchi, N. Mitsui and O. Tooi, Screening breeding sites of the common toad (Bufo bufo) in England and Wales for evidence of endocrine disrupting activity, Ecotoxicol. Environ. Saf., 2015, 117, 7–19 CrossRef CAS PubMed.
- B. Jarošová, L. Bláha, J. P. Giesy and K. Hilscherová, What level of estrogenic activity determined by in vitro assays in municipal waste waters can be considered as safe?, Environ. Int., 2014, 64, 98–109 CrossRef PubMed.
- F. Itzel, N. Baetz, L. L. Hohrenk, L. Gehrmann, D. Antakyali, T. C. Schmidt and J. Tuerk, Evaluation of a biological post-treatment after full-scale ozonation at a municipal wastewater treatment plant, Water Res., 2020, 170, 115316 CrossRef CAS PubMed.
- S. Xiao, X. Lv, Y. Lu, X. Yang, X. Dong, K. Ma, Y. Zeng, T. Jin and F. Tang, Occurrence and change of estrogenic activity in the process of drinking water treatment and distribution, Environ. Sci. Pollut. Res. Int., 2016, 23, 16977–16986 CrossRef CAS PubMed.
- X. Ke, C. Wang, H. Zhang, Y. Zhang and S. Gui, Characterization of estrogenic receptor agonists and evaluation of estrogenic activity in the sediments of Liaohe River protected areas, Mar. Pollut. Bull., 2015, 100, 176–181 CrossRef PubMed.
- M. Čelić, B. D. Škrbić, S. Insa, J. Živančev, M. Gros and M. Petrović, Occurrence and assessment of environmental risks of endocrine disrupting compounds in drinking, surface and wastewaters in Serbia, Environ. Pollut., 2020, 262, 114344 CrossRef PubMed.
- Y. Huang, X. Xie, L. J. Zhou, X. Ji, B. Gao, G. Z. Xu and A. Li, Multi-phase distribution and risk assessment of endocrine disrupting chemicals in the surface water of the Shaying River, -Huai River Basin, China, Ecotoxicol. Environ. Saf., 2019, 173, 45–53 CrossRef PubMed.
- R. Tan, R. Liu, B. Li, X. Liu and Z. Li, Typical Endocrine Disrupting Compounds in Rivers of Northeast China: Occurrence, Partitioning, and Risk Assessment, Arch. Environ. Contam. Toxicol., 2018, 75, 213–223 CrossRef PubMed.
- D. Liu, S. Wu, H. Xu, Q. Zhang, S. Zhang, L. Shi, C. Yao, Y. Liu and J. Cheng, Distribution and bioaccumulation of endocrine disrupting chemicals in water, sediment and fishes in a shallow Chinese freshwater lake: Implications for ecological and human health risks, Ecotoxicol. Environ. Saf., 2017, 140, 222–229 CrossRef PubMed.
- X.-W. Chen, J.-L. Zhao, Y.-S. Liu, L.-X. Hu, S.-S. Liu and G.-G. Ying, Evaluation of estrogenic activity in the Pearl River by using effect-directed analysis, Environ. Sci. Pollut. Res. Int., 2016, 23, 21692–21702 CrossRef PubMed.
- Y. Li, S. Gao, S. Liu, B. Liu, X. Zhang, M. Gao, L. Cheng and B. Hu, Excretion of manure-borne estrogens and androgens and their potential risk estimation in the Yangtze River Basin, J. Environ. Sci., 2015, 37, 110–117 CrossRef CAS PubMed.
- J. Yang, H. Li, Y. Ran and K. Chan, Distribution and bioconcentration of endocrine disrupting chemicals in surface water and fish bile of the Pearl River Delta, South China, Chemosphere, 2014, 107, 439–446 CrossRef CAS PubMed.
- A. C. V. Dias, F. W. Gomes, D. M. Bila, G. L. J. Sant'Anna and M. Dezotti, Analysis of estrogenic activity in environmental waters in Rio de Janeiro state (Brazil) using the yeast estrogen screen, Ecotoxicol. Environ. Saf., 2015, 120, 41–47 CrossRef CAS PubMed.
- K. Hassell, V. Pettigrove, N. Beresford, S. Jobling and A. Kumar, No evidence of exposure to environmental estrogens in two feral fish species sampled from the Yarra River, Australia: A comparison with Northern Hemisphere studies, Ecotoxicol. Environ. Saf., 2016, 131, 104–117 CrossRef CAS PubMed.
- E. Archer, G. M. Wolfaardt, J. H. van Wyk and N. van Blerk, Investigating (anti)estrogenic activities within South African wastewater and receiving
surface waters: Implication for reliable monitoring, Environ. Pollut., 2020, 263, 114424 CrossRef CAS PubMed.
- K.-Y. Chen and P.-H. Chou, Detection of endocrine active substances in the aquatic environment in southern Taiwan using bioassays and LC-MS/MS, Chemosphere, 2016, 152, 214–220 CrossRef CAS PubMed.
- A. G. M. Osman, K. Y. AbouelFadl, A. Krüger and W. Kloas, Screening of multiple hormonal activities in water and sediment from the river Nile, Egypt, using in vitro bioassay and gonadal histology, Environ. Monit. Assess., 2015, 187, 317 CrossRef PubMed.
- N. H. Tran, M. Reinhard and K. Y.-H. Gin, Occurrence and fate of emerging contaminants in municipal wastewater treatment plants from different geographical regions-a review, Water Res., 2018, 133, 182–207 CrossRef CAS PubMed.
- Y. Gross-Sorokin Melanie, D. Roast Stephen and C. Brighty Geoffrey, Assessment of Feminization of Male Fish in English Rivers by the Environment Agency of England and Wales, Environ. Health Perspect., 2006, 114, 147–151 CrossRef PubMed.
- M. E. Franco, K. M. Stroski, J. L. Sims, S. R. Burket, C. Ashcroft, M. Luers, B. W. Brooks and R. Lavado, Plasma Vitellogenin Reveals Potential Seasonal Estrogenicity in Fish from On-Site Wastewater Treatment Systems in Semi-Arid Streams Influenced by Snowmelt, Bull. Environ. Contam. Toxicol., 2020, 105, 692–698 CrossRef CAS PubMed.
- M. M. Ali, M. L. Ali, M. S. Islam and M. Z. Rahman, Assessment of toxic metals in water and sediment of Pasur River in Bangladesh, Water Sci. Technol., 2018, 77, 1418–1430 CrossRef CAS PubMed.
- G. V. Aguirre-Martínez, H. C. Reinardy, M. L. Martín-Díaz and T. B. Henry, Response of gene expression in zebrafish exposed to pharmaceutical mixtures: Implications for environmental risk, Ecotoxicol. Environ. Saf., 2017, 142, 471–479 CrossRef PubMed.
- A. Abdel-Moneim, D. Deegan, J. Gao, C. De Perre, J. S. Doucette, B. Jenkinson, L. Lee and M. S. Sepúlveda, Gonadal intersex in smallmouth bass Micropterus dolomieu from northern Indiana with correlations to molecular biomarkers and anthropogenic chemicals, Environ. Pollut., 2017, 230, 1099–1107 CrossRef CAS PubMed.
- M. F. Pimentel, É. P. Damasceno, P. C. Jimenez, P. F. R. Araújo, M. F. Bezerra, P. C. V. de Morais, R. M. Cavalcante, S. Loureiro and L. V. C. Lotufo, Endocrine disruption in Sphoeroides testudineus tissues and sediments highlights contamination in a northeastern Brazilian estuary, Environ. Monit. Assess., 2016, 188, 298 CrossRef PubMed.
- M. Houde, M. Douville, M. Giraudo, K. Jean, M. Lépine, C. Spencer and A. O. De Silva, Endocrine-disruption potential of perfluoroethylcyclohexane sulfonate (PFECHS) in chronically exposed Daphnia magna, Environ. Pollut., 2016, 218, 950–956 CrossRef CAS PubMed.
- F. Gagné, M. Douville, C. André, T. Debenest, A. Talbot, J. Sherry, L. M. Hewitt, R. A. Frank, M. E. McMaster, J. Parrott and G. Bickerton, Differential changes in gene expression in rainbow trout hepatocytes exposed to extracts of oil sands process-affected water and the Athabasca River, Comp. Biochem. Physiol., Part C: Toxicol. Pharmacol., 2012, 155, 551–559 Search PubMed.
- P. Geraudie, M. Gerbron and C. Minier, Endocrine disruption effects in male and intersex roach (Rutilus rutilus L.) from French rivers: An integrative approach based on subcellular to individual responses, Comp. Biochem. Physiol., Part B: Biochem. Mol. Biol., 2017, 211, 29–36 CrossRef CAS PubMed.
- P. I. S. Pinto, M. D. Estêvão and D. M. Power, Effects of Estrogens and Estrogenic Disrupting Compounds on Fish Mineralized Tissues, Mar. Drugs, 2014, 12, 4474–4494 CrossRef PubMed.
- X. Gao, Y. Xu, M. Ma, K. Rao and Z. Wang, Simultaneous passive sampling of hydrophilic and hydrophobic emerging organic contaminants in water, Ecotoxicol. Environ. Saf., 2019, 178, 25–32 CrossRef CAS PubMed.
- H. Ahkola, J. Juntunen, K. Krogerus, T. Huttula, S. Herve and A. Witick, Suitability of Chemcatcher® passive sampling in monitoring organotin compounds at a wastewater treatment plant, Environ. Sci.: Water Res. Technol., 2016, 2, 769–778 RSC.
- Z. Tousova, B. Vrana, M. Smutna, J. Novak, V. Klucarova, R. Grabic, J. Slobodnik, J. P. Giesy and K. Hilscherova, Analytical and bioanalytical assessments of organic micropollutants in the Bosna River using a combination of passive sampling, bioassays and multi-residue analysis, Sci. Total Environ., 2019, 650, 1599–1612 CrossRef CAS PubMed.
- E. L. Hamblen, M. T. D. Cronin and T. W. Schultz, Estrogenicity and acute toxicity of selected anilines using a recombinant yeast assay, Chemosphere, 2003, 52, 1173–1181 CrossRef CAS PubMed.
- C. Wei, F. Zhang, Y. Hu, C. Feng and H. Wu, Ozonation in water treatment: the generation, basic properties of ozone and its practical application, Rev. Chem. Eng., 2017, 33, 49–89 CAS.
- P. Xue, J. Fu and Y. Zhou, The Aryl Hydrocarbon Receptor and Tumor Immunity, Front. Immunol., 2018, 9 DOI:10.3389/fimmu.2018.00286.
- M. A. Tripodi, N. B. Andrioli and O. V. Suárez, Genotoxicity evaluation using micronucleus test in Rattus norvegicus captured in urban ecosystems of Buenos Aires, Argentina, Environ. Sci. Pollut. Res. Int., 2020, 27, 27626–27634 CrossRef CAS PubMed.
- A. Rybakovas, K. Arbačiauskas, V. Markovskienė and K. Jokšas, Contamination and genotoxicity biomarker responses in bivalve mussels from the major Lithuanian rivers, Environ. Mol. Mutagen., 2020, 61, 338–354 CrossRef CAS PubMed.
- Y. Risjani, G. Loppion, J. Couteau, Y. Yunianta, I. Widowati, A. Hermawati and C. Minier, Genotoxicity in the rivers from the Brantas catchment (East Java, Indonesia): occurrence in sediments and effects in Oreochromis niloticus (Linnæus 1758), Environ. Sci. Pollut. Res. Int., 2020, 27, 21905–21913 CrossRef CAS PubMed.
- M. Iqbal, L. Taylor-Edmonds, S. Ebrahimi, N. Zollbrecht and R. C. Andrews, Low toxicological impact of wastewaters on drinking water sources, Water Res., 2020, 171, 115376 CrossRef CAS PubMed.
- Z. Kljaković-Gašpić, S. Herceg Romanić, T. Bituh, V. Kašuba, I. Brčić Karačonji, N. Brajenović, I. Franulović, J. Jurasović, D. Klinčić, N. Kopjar, G. Marović, M. Milić, T. Orct, A. Sekovanić and D. Želježić, Assessment of multiple anthropogenic contaminants and their potential genotoxicity in the aquatic environment of Plitvice Lakes National Park, Croatia, Environ. Monit. Assess., 2018, 190, 694 CrossRef PubMed.
- A. L. Hilario Garcia, C. A. Matzenbacher, M. S. Santos, L. Prado, J. N. Picada, S. M. Premoli, D. S. Corrêa, L. Niekraszewicz, J. F. Dias, I. Grivicich and J. da Silva, Genotoxicity induced by water and sediment samples from a river under the influence of brewery effluent, Chemosphere, 2017, 169, 239–248 CrossRef CAS PubMed.
- S. Kolarević, M. Kračun-Kolarević, J. Kostić, J. Slobodnik, I. Liška, Z. Gačić, M. Paunović, J. Knežević-Vukčević and B. Vuković-Gačić, Assessment of the genotoxic potential along the Danube River by application of the comet assay on haemocytes of freshwater mussels: The Joint Danube Survey 3, Sci. Total Environ., 2016, 540, 377–385 CrossRef PubMed.
- S. Kolarević, J. Knežević-Vukčević, M. Paunović, M. Kračun, B. Vasiljević, J. Tomović, B. Vuković-Gačić and Z. Gačić, Monitoring of DNA damage in haemocytes of freshwater mussel Sinanodonta woodiana sampled from the Velika Morava River in Serbia with the comet assay, Chemosphere, 2013, 93, 243–251 CrossRef PubMed.
- Y. Han, N. Li, Y. Oda, M. Ma, K. Rao, Z. Wang, W. Jin, G. Hong, Z. Li and Y. Luo, Evaluation of genotoxic effects of surface waters using a battery of bioassays indicating different mode of action, Ecotoxicol. Environ. Saf., 2016, 133, 448–456 CrossRef CAS PubMed.
- N. Creusot, S. Ait-Aissa, N. Tapie, P. Pardon, F. Brion, W. Sanchez, E. Thybaud, J.-M. Porcher and H. Budzinski, Identification of synthetic steroids in river water downstream from pharmaceutical manufacture discharges based on a bioanalytical approach and passive sampling, Environ. Sci. Technol., 2014, 48, 3649–3657 CrossRef CAS PubMed.
- S. A. Ortiz de Garcia, G. Pinto Pinto, P. A. Garcia-Encina and R. Irusta-Mata, Ecotoxicity and environmental risk assessment of pharmaceuticals and personal care products in aquatic environments and wastewater treatment plants, Ecotoxicology, 2014, 23, 1517–1533 CrossRef CAS PubMed.
- A. Eadie, I. C. Vásquez, X. Liang, X. Wang, C. L. 2nd Souders, J. E. Chehouri, R. Hoskote, A. Feswick, A. M. Cowie, J. R. Loughery and C. J. Martyniuk, Transcriptome network data in larval zebrafish (Danio rerio) following exposure to the phenylpyrazole fipronil, Data Brief, 2020, 33, 106413 CrossRef PubMed.
- J. M. Ali, A. Montecinos, T. T. Schulze, L. G. Allmon, A. T. Kallenbach, G. F. Watson, P. H. Davis, D. D. Snow, A. Bertin, N. Gouin and A. S. Kolok, Assessment of Gene Expression Biomarkers in the Chilean Pencil Catfish, Trichomycterus areolatus, from the Choapa River Basin, Coquimbo Chile, Arch. Environ. Contam. Toxicol., 2020, 78, 137–148 CrossRef CAS PubMed.
- J. C. V. Sposito, C. C. Montagner, M. Casado, L. Navarro-Martín, J. C. Jut Solórzano, B. Piña and A. B. Grisolia, Emerging contaminants in Brazilian rivers: Occurrence and effects on gene expression in zebrafish (Danio rerio) embryos, Chemosphere, 2018, 209, 696–704 CrossRef CAS PubMed.
- S. E. Hook, F. J. Kroon, S. Metcalfe, P. A. Greenfield, P. Moncuquet, A. McGrath, R. Smith, M. S. J. Warne, R. D. Turner, A. McKeown and D. A. Westcott, Global transcriptomic profiling in barramundi (Lates calcarifer) from rivers impacted by differing agricultural land uses, Environ. Toxicol. Chem., 2017, 36, 103–112 CrossRef CAS PubMed.
- L. Besaury, B. Pawlak and L. Quillet, Expression of copper-resistance genes in microbial communities under copper stress and oxic/anoxic conditions, Environ. Sci. Pollut. Res. Int., 2016, 23, 4013–4023 CrossRef CAS PubMed.
- A.-K. Muller, K. Leser, D. Kampfer, C. Riegraf, S. E. Crawford, K. Smith, E. L. M. Vermeirssen, S. Buchinger and H. Hollert, Bioavailability of estrogenic compounds from sediment in the context of flood events evaluated by passive sampling, Water Res., 2019, 161, 540–548 CrossRef PubMed.
- T. Hamers, J. H. Kamstra, J. van Gils, M. C. Kotte and A. G. M. van Hattum, The influence of extreme river discharge conditions on the quality of suspended particulate matter in Rivers Meuse and Rhine (The Netherlands), Environ. Res., 2015, 143, 241–255 CrossRef CAS PubMed.
- B. Escher, G. Braun and C. Zarfl, Exploring the concepts of concentration addition and independent action using a linear low-effect mixture model, Environ. Toxicol. Chem., 2020, 39, 2552–2559 CrossRef CAS PubMed.
- N. J. Efosa, W. Kleiner, W. Kloas and F. Hoffmann, Diclofenac can exhibit estrogenic modes of action in male Xenopus laevis, and affects the hypothalamus-pituitary-gonad axis and mating vocalizations, Chemosphere, 2017, 173, 69–77 CrossRef CAS PubMed.
- A. Bahlmann, W. Brack, R. J. Schneider and M. Krauss, Carbamazepine and its metabolites in wastewater: Analytical pitfalls and occurrence in Germany and Portugal, Water Res., 2014, 57, 104–114 CrossRef CAS PubMed.
- Y. Zhang, S.-U. Geißen and C. Gal, Carbamazepine and diclofenac: Removal in wastewater treatment plants and occurrence in water bodies, Chemosphere, 2008, 73, 1151–1161 CrossRef CAS PubMed.
- J. L. T. Pestana, S. C. Novais, M. F. L. Lemos and A. M. V. M. Soares, Cholinesterase activity in the caddisfly Sericostoma vittatum: Biochemical enzyme characterization and in vitro effects of insecticides and psychiatric drugs, Ecotoxicol. Environ. Saf., 2014, 104, 263–268 CrossRef CAS PubMed.
- S. D. Melvin, Effect of antidepressants on circadian rhythms in fish: Insights and implications regarding the design of behavioural toxicity tests, Aquat. Toxicol., 2017, 182 DOI:10.1016/j.aquatox.2016.11.007.
- J. Wolz, K. Grosshans, G. Streck, T. Schulze, A. Rastall, L. Erdinger, W. Brack, M. Fleig, D. Kuhlers, T. Braunbeck and H. Hollert, Estrogen receptor mediated activity in bankside groundwater, with flood suspended particulate matter and floodplain soil - an approach combining tracer substance, bioassay and target analysis, Chemosphere, 2011, 85, 717–723 CrossRef CAS PubMed.
- M. L. De Baat, R. Van der Oost, G. H. Van der Lee, N. Wieringa, T. Hamers, P. F. M. Verdonschot, P. De Voogt and M. H. S. Kraak, Advancements in effect-based surface water quality assessment, Water Res., 2020, 183, 116017 CrossRef CAS PubMed.
- P. Xia, H. Zhang, Y. Peng, W. Shi and X. Zhang, Pathway-based assessment of single chemicals and mixtures by a high-throughput transcriptomics approach, Environ. Int., 2020, 136, 105455 CrossRef CAS PubMed.
|
This journal is © The Royal Society of Chemistry 2021 |