Continuous production of uniform chitosan beads as hemostatic dressings by a facile flow injection method
Received
10th June 2020
, Accepted 14th July 2020
First published on 15th July 2020
Abstract
In this work, we developed a facile flow injection method to fabricate chitosan beads of uniform size in a continuous manner and assessed their properties as hemostatic dressings. Chitosan was dissolved into the ionic liquid 1-ethyl-3-methylimidazolium acetate (EMIM Ac) to form a solution, and then the chitosan/EMIM Ac solution was flow injected into ethanol to form beads of uniform size. The formed chitosan beads were obtained following the exchange of ethanol solvent with water and freeze-drying. The overall process is ecofriendly and scalable without acid/alkali treatment or the use of cross-linking agents. The morphology, swelling, and cytotoxicity of the chitosan beads were characterized. The blood coagulation and whole blood clotting kinetics of the chitosan beads were also studied. The chitosan beads have a significantly high swelling capacity. They can absorb 30 times as much water and 40 times as much PBS/0.9% NaCl solution as their dry mass. Cytotoxicity was not observed on NIH3T3 fibroblast cells after 24 h- or 48 h-incubation, when the concentration of the chitosan beads was 1.2 mg mL−1 or lower. Pig whole blood quickly lost its flowability in 10 min after the blood (1 mL) was incubated with 1 mg of chitosan beads. The whole blood clotting kinetics results showed that the chitosan beads efficiently caused the pig whole blood to clot with the absorbance of red blood cells (RBCs) dramatically reduced in 20 min and further reduced in 1 hour. The chitosan beads were shown to be biocompatible with excellent hemostatic properties. This work can apply a simple method to prepare chitosan beads for trauma hemostasis and broaden chitosan processing such as continuous manufacturing and 3D printing.
1. Introduction
Chitosan has broad biomedical applications and has been used in the form of a scaffold, fiber, film, and foam.1–10 Chitosan-based antibacterial and hemostatic wound dressings have been approved by the Food and Drug Administration (FDA).11 In particular, chitosan beads have received considerable attention for their use in the fields of drug delivery, protein release, adsorption and so on.12–16 Due to the macromolecular hydrogen-bonded network of chitosan, chitosan beads are usually prepared by dissolving chitosan in acids and precipitating in alkalis. Many small molecules/macromolecules are involved in cross-linking. The process is tedious, and the acid and alkali residuals or cross-linkers may affect the biological properties of chitosan materials.
The preparation of chitosan materials by adjusting the chitosan hydrogen-bonded network itself in plain water is an eco-friendly way for its biomedical applications, which needs critical safety demand. Ionic liquids (ILs) are environmentally benign solvents as they have features such as non-volatility, thermal and chemical stability, and ease of recycling.17–20 Many water-miscible ionic liquids can dissolve nature-based polysaccharides. It opens an opportunity to use them as media for breaking and rebuilding the hydrogen-bonded network of chitosan in plain water.21,22 Recently, we developed an eco-friendly method to prepare plain water-based chitosan solution.23 In the method, we first solubilize chitosan in an ionic liquid, freeze the chitosan/IL system, and then subject it to extensive solvent change with water to remove the IL. The method provides a new approach to fabricate chitosan products. A drug-loaded chitosan film has been prepared with the plain-water based chitosan solution for improved drug delivery.24 In this work, we went on to develop a method to fabricate chitosan beads by integrating continuous flow injection into the process. In particular, chitosan/IL solution is flow injected into ethanol to form beads and remove IL, which is then washed with water and freeze-dried. The overall process is ecofriendly and potentially allows for large-scale continuous production. The morphology, swelling, cytotoxicity, blood coagulation, and whole blood clotting kinetics of the resulting chitosan bead were studied for its performance assessment as a potential hemostatic dressing. The chitosan products prepared by this method do not require acid/alkali treatment or the use of cross-linking agents, more appealing for biomedical applications.
2. Materials and methods
Materials
High molecular weight (HMW) chitosan (310
000–375
000 Da), ionic liquid 1-ethyl-3-methylimidazolium acetate (EMIM Ac, ≥95.0%) and WST-1 assay were purchased from Sigma-Aldrich. Ethanol, phosphate-buffered saline (PBS, 1×, pH 7.4), sodium chloride (NaCl), and calcium chloride (CaCl2) were purchased from Thermo Fisher Scientific. Water was ultra-purified by deionization and filtration before use. Pig whole blood (collected following the United States Department of Agriculture (USDA) guidelines with 3.8% anticoagulant sodium citrate solution at 1
:
10 dilution) was purchased from Pel Freez Biologicals.
Preparation of chitosan beads
Chitosan (HMW) was dissolved into ionic liquid EMIM Ac at ∼115 °C to prepare a solution at a concentration of 20 mg mL−1. The chitosan/EMIM Ac solution was then injected into ethanol through a syringe with a 0.33 mm inner-diameter needle at room temperature. The injection speed was set at 10 mL h−1 controlled using a syringe pump (KD Scientific KDS 270, USA). The resulting beads were kept in ethanol overnight and washed with ethanol three times to remove ionic liquid completely. Then the chitosan beads were washed with water three times to allow solvent exchange between ethanol and water in 30 min. Finally, the hydrated chitosan beads were freeze-dried to obtain anhydrous chitosan beads. The sizes of the chitosan beads were measured by using the ImageJ software.25
Scanning electron microscope (SEM)
SEM images of chitosan beads were taken under a scanning electron microscope (Hitachi FE-SEM Su-70 and S-3400N, Japan) after being sputter-coated with gold–platinum for 90 s.
Swelling
Lyophilized chitosan beads were pre-weighted as W0 and then immersed and incubated at 37 °C in 1 mL of water, PBS (pH 7.4), or 0.9% NaCl aqueous solution, respectively. At predetermined time points of 0.25, 0.5, 1, 2, 4, 6, and 24 h, the hydrated chitosan beads were weighed as Wt. The swelling ratio (%) is calculated as (Wt − W0)/W0 ×100.
Cytotoxicity
The cytotoxicity of chitosan beads was evaluated in NIH3T3 mouse embryo fibroblast cells by WST-1 assay as previously reported.26 Briefly, NIH3T3 cells were seeded in 24-well plates at a density of 1 × 105 cells per well. After cell attachment, chitosan beads were added to each well at a density of 0.4 mg mL−1, 0.8 mg mL−1, and 1.2 mg mL−1, respectively. After 24 h and 48 h incubation, the cell viability was tested on WST-1 assay using a microplate spectrophotometer.
Blood coagulation tests
Chitosan beads (1 mg, 2 mg, and 5 mg) were added to 1 mL of pig whole blood as-purchased with an anticoagulant in a 37 °C water bath. Time was recorded right after the addition of 50 μL of 0.1 M CaCl2 aqueous solution to the mixture. The tube was tilted every 30 s to observe if the blood was gelatinized. The clotting time was recorded when the blood completely lost flowability.27
Whole blood clotting kinetics
Clotting of 1 mL of pig whole blood was initiated by adding 50 μL of 0.1 M CaCl2 aqueous solution. The initiated blood (100 μL) was immediately added to a tube containing 1 mg, 2 mg, or 5 mg of chitosan beads maintained at 37 °C. At each time point of 5, 20, 35, and 50 min, 3 mL of water was added to each tube for 5 min to lyse red blood cells (RBCs), which were not trapped within the blood clot. The diluted hemoglobin released from the lysed RBCs was added to a 96 well plate (200 μL per well) in triplicates for each sample and time point. The absorbance at 540 nm was recorded using a microplate reader.28
Statistical analysis.
The data were reported as means ± standard deviation (SD). Student's t-test was conducted for statistical analysis. P values less than 0.05 were considered statistically significant.
3. Results and discussion
The flow injection process for the fabrication of chitosan beads is shown in Fig. 1. A dissolution process is the first step, in which chitosan (HMW) is solubilized in ionic liquid EMIM Ac at 115 °C. Flow injection is subsequently carried out as the chitosan/EMIM Ac solution is dropwise injected to ethanol to form spherical chitosan beads. The resulting chitosan beads are collected, washed with ethanol, and then engaged in the solvent exchange process in water. Finally, freeze-drying is followed to dry chitosan beads.
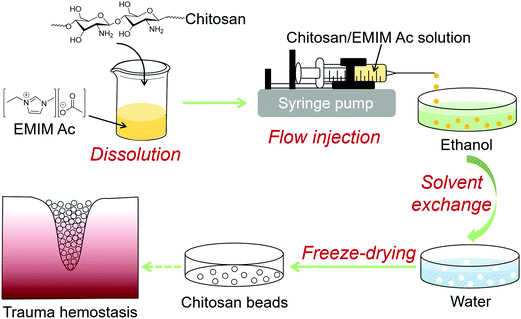 |
| Fig. 1 Schematic illustration of the fabrication of chitosan beads through a flow injection process. | |
The flow injection system is simple to set up and enables the bead fabrication process to be carried out easily. It is composed of a syringe pump and a receiver filled with ethanol, which is positioned on a magnetic stirrer (Fig. 2a). Continuous production of transparent pale yellowish chitosan beads with a diameter of 2.5 ± 0.2 mm is enabled as chitosan/EMIM Ac solution is dispensed into ethanol at a controlled rate (Fig. 2b). The hydrogen-bonded network of chitosan macromolecular chains is disrupted by ionic liquid EMIM Ac through the dissolution process.22 When chitosan/EMIM Ac is added to a nonsolvent like ethanol, chitosan/EMIM Ac drop immediately collapses and stabilizes in spherical shape. As the ionic liquid EMIM Ac was washed out with ethanol and the hydrogen-bonded network was rebuilt, the chitosan beads gradually turned white (Fig. 2c). The chitosan beads retrieved from the ionic liquid maintained relatively stable shape and size (2.6 ± 0.1 mm) in ethanol.
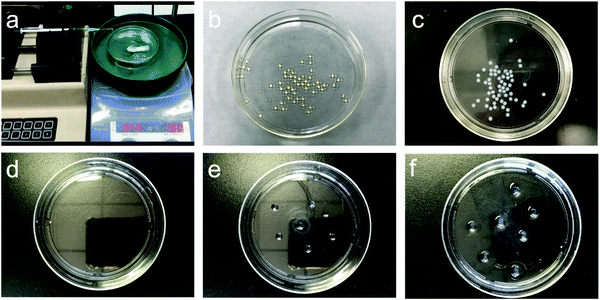 |
| Fig. 2 The flow injection system setup and monitoring of chitosan beads throughout the process. (a) Flow injection experimental setup. (b) Chitosan beads produced upon the injection of chitosan/EMIM Ac to ethanol. (c) Chitosan beads after ethanol wash. The appearance of chitosan beads following ethanol wash for various lengths of time, i.e., 10 min (d), 30 min (e), and overnight (f) and subsequent 30 min solvent exchange with water. | |
The subsequent shape-maintenance of chitosan beads in water depends strongly on its hydrogen-bonded network rebuilding through the ethanol-based washing process. It is especially important to control the duration of the ethanol washing before the solvent exchange with water. If we directly injected chitosan/EMIM Ac into the water without ethanol processing, we could only get a chitosan water solution. This is because the amine groups of chitosan would be gradually protonated during the solvent exchange process from ionic liquid EMIM Ac to water.23 It reduces the cross-linking effect from the hydrogen-bonding interactions of chitosan through electrostatic repulsion. A water-based gel-like solution of chitosan would form, which was also observed in our previous study.23 When the ethanol washing step was less than 10 min, the ionic liquid largely residues in chitosan beads, and there is no shaped chitosan material left after removing the washing water for 30 min solvent exchange with stirring (Fig. 2d). Extending ethanol washing to 30 min helped preserve chitosan beads (4.2 ± 0.2 mm) (Fig. 2e). To further eliminate the effect of ionic liquid residues, we prolonged the ethanol washing overnight, during which ethanol was refreshed three times. As a result, the chitosan beads were maximally preserved in water despite that their size increased to 4.6 ± 0.5 mm due to swelling (Fig. 2f).24 In this process, the hydrogen-bonded network of chitosan was broken by the dissolution of EMIM Ac and successfully rebuilt as a cross-linking effect for the chitosan beads in ethanol. This cross-linking effect has largely remained after the subsequent solvent exchange process with water. A micron-sized bulky structure—chitosan bead was formed in water by the cross-linking effect of chitosan hydrogen-bonding interactions itself (Fig. 3).
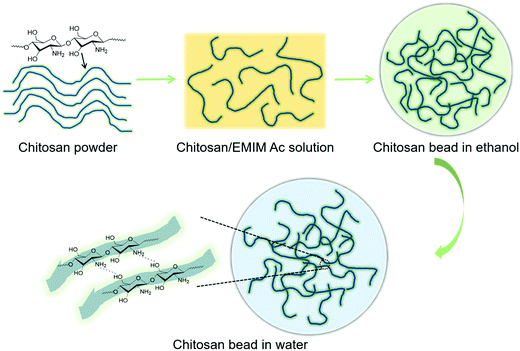 |
| Fig. 3 Proposed chitosan macromolecular chain formation from chitosan powder, chitosan/EMIM Ac solution and formed chitosan bead in ethanol and water. | |
The freeze-dried chitosan beads appear as solid white particles (Fig. 4a) and have an average size of 3.5 ± 0.4 mm (Fig. 4b). The surface of the chitosan bead shrunk and formed a compact wrinkled microstructure (Fig. 4c), which is typically seen in freeze-dried materials.26 The internal microstructure appears to be porous (Fig. 4d). The swelling behaviors of dehydrated chitosan beads were studied in water, PBS, and 0.9% NaCl solution equilibrated at 37 °C. As shown in Fig. 5, chitosan beads reached swelling equilibrium within 60 min regardless of the medium type. Chitosan beads absorbed 30 times as much water as their weight. More impressively, they absorbed nearly 40 times as much PBS or 0.9% NaCl solution as their weight. The significantly high swelling capacity of the chitosan beads is attributed to their high hydrophilicity and porous microstructures. The chitosan beads were shown to have much higher swelling in PBS and 0.9% NaCl solution due to their higher affinity to salt solutions.29–31
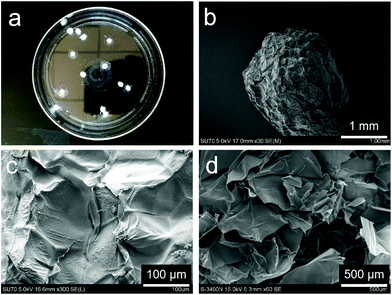 |
| Fig. 4 Photograph and SEM images of freeze-dried chitosan beads. (a) Photograph of chitosan beads. SEM images of a whole chitosan bead (b), the surface morphology (c), and the internal structure (d) of chitosan bead. | |
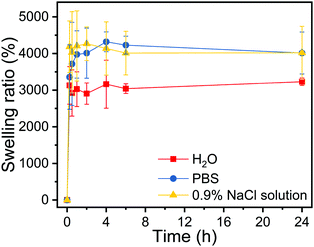 |
| Fig. 5 Swelling behavior of dried chitosan beads in water, PBS (pH 7.4) and 0.9% NaCl solution at 37 °C. | |
The chitosan beads were shown to possess excellent cytocompatibility. NIH3T3 fibroblast cells did not experience viability reduction following 24 h or 48 h incubation with chitosan beads within the concentration range of 0.4 and 1.2 mg mL−1 (Fig. 6). Given the utility of chitosan-based materials for wound healing and hemostasis,22,27,32,33 we tested the hemostatic properties of the chitosan beads prepared by us. Pig whole blood quickly lost its flowability in 10 min upon the addition of 1 mg chitosan beads to 1 mL of the blood (Fig. 7a). Nonetheless, higher amounts of chitosan beads (2 or 5 mg) did not seem to accelerate blood coagulation. The whole blood clotting kinetics induced by chitosan beads was further studied. The absorbance is indicative of the number of red blood cells (RBCs) not trapped within the clot. Therefore, the absorbance of RBCs is used to monitor clotting kinetics. Chitosan beads efficiently caused the pig whole blood to clot, as evidenced by the dramatic reduction in RBC absorbance in 20 min and a further reduction in 1 hour (Fig. 7b). Similar whole blood clotting kinetics was observed among the three treatment groups, consistent with blood coagulation test results.
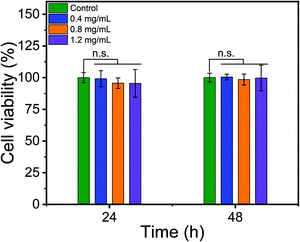 |
| Fig. 6 Cytotoxicity of chitosan beads to NIH3T3 cells. n.s. indicates not significant. | |
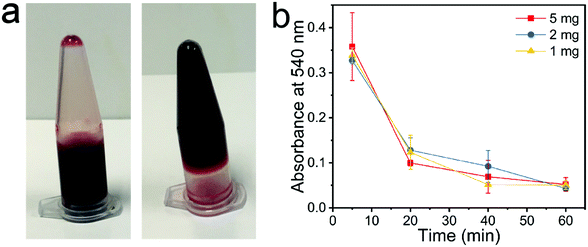 |
| Fig. 7 Hemostatic properties of chitosan beads. (a) Pig whole blood (1 mL) coagulated upon the addition of 1 mg of chitosan beads. Left panel: Untreated pig whole blood; right panel, the blood stopped flowing after 10 min treatment with chitosan beads. (b) Pig whole blood clotting kinetics induced by various amounts of chitosan beads. | |
Our preliminary assessment supports the potential use of chitosan beads as hemostatic dressings prepared using this simple ecofriendly flow injection method. Based on this method, it is possible to prepare chitosan beads of different sizes by adjusting injection parameters, e.g., the needle gauge, flow rate, stirring speed, and rheological properties of chitosan/IL systems. Chitosan beads can be used to deliver drugs. For instance, water-soluble drugs can be loaded on chitosan beads through surface adsorption, and water-insoluble or sparingly soluble drugs can be blended into chitosan during the dissolution by ionic liquids.34,35 The ionic liquid can be recycled and reused for reducing the overall manufacturing cost. Taken together, this simple continuous flow injection method provides a new opportunity to fabricate scalable chitosan-based biomedical materials and drug-loaded formulations.
4. Conclusions
In this work, we developed a simple flow injection method that enables the continuous production of chitosan beads of uniform size. Chitosan is first dissolved in ionic liquid EMIM Ac and injected into ethanol at a controlled rate using an infusion pump for bead forming, followed by solvent exchange with water and freeze-drying. The resulting chitosan beads have uniform size and porous microstructures. Chitosan beads possess high swelling capability and good biocompatibility. They are capable of efficiently inducing blood coagulation and clotting and have the potential use as hemostatic dressings for trauma hemostasis. This flow injection method provides a new way to fabricate chitosan products in a continuous manufacturing fashion.
Conflicts of interest
The authors declare no competing financial interest.
Acknowledgements
This work was supported, in part, by the Fundamental Research Funds for the Central Universities (Grant No. 3332019100 and 2019PT320028) (BL).
References
- M. Rinaudo, Chitin and chitosan: properties and applications, Prog. Polym. Sci., 2006, 31, 603–632 CrossRef CAS.
- M. Dash, F. Chiellini, R. M. Ottenbrite and E. Chiellini, Chitosan—A versatile semi-synthetic polymer in biomedical applications, Prog. Polym. Sci., 2011, 36, 981–1014 CrossRef CAS.
- M. Rodriguez-Vazquez, B. Vega-Ruiz, R. Ramos-Zuniga, D. Alexander Saldana-Koppel and L. Fernando Quinones-Olvera, Chitosan and its potential use as a scaffold for tissue engineering in regenerative medicine, BioMed Res. Int., 2015, 821279 Search PubMed.
- K. Azuma, S. Ifuku, T. Osaki, Y. Okamoto and S. Minami, Preparation and biomedical applications of chitin and chitosan nanofibers, J. Biomed. Nanotechnol., 2014, 10, 2891–2920 CrossRef CAS PubMed.
- G. Cardenas, P. Anaya, C. von Plessing, C. Rojas and J. Sepulveda, Chitosan composite films. Biomedical applications, J. Mater. Sci.: Mater. Med., 2008, 19, 2397–2405 CrossRef CAS PubMed.
- J.-D. Mathias, N. Tessier-Doyen and P. Michaud, Development of a chitosan-based biofoam: application to the processing of a porous ceramic material, Int. J. Mol. Sci., 2011, 12, 1175–1186 CrossRef CAS PubMed.
- P. S. Barber, S. P. Kelley, C. S. Griggs, S. Wallace and R. D. Rogers, Surface modification of ionic liquid-spun chitin fibers for the extraction of uranium from seawater: seeking the strength of chitin and the chemical functionality of chitosan, Green Chem., 2014, 16, 1828–1836 RSC.
- K. Zhu, J. Duan, J. Guo, S. Wu, A. Lu and L. Zhang, High-strength films consisted of oriented chitosan nanofibers for guiding cell growth, Biomacromolecules, 2017, 18, 3904–3912 CrossRef CAS PubMed.
- Y. Yang, X. Wang, F. Yang, H. Shen and D. Wu, A universal soaking strategy to convert composite hydrogels into extremely tough and rapidly recoverable double-network hydrogels, Adv. Mater., 2016, 28, 7178–7184 CrossRef CAS PubMed.
- D. Zhu, X. Jin, X. Leng, H. Wang, J. Bao, W. Liu, K. Yao and C. Song, Local gene delivery via endovascular stents coated with dodecylated chitosan-plasmid DNA nanoparticles, Int. J. Nanomed., 2010, 5, 1095–1102 CrossRef CAS PubMed.
- I. Wedmore, J. G. McManus, A. E. Pusateri and J. B. Holcomb, A special report on the chitosan-based hemostatic dressing: experience in current combat operations, J. Trauma Acute Care Surg., 2006, 60, 655–658 CrossRef PubMed.
- X. Shu and K. J. Zhu, A novel approach to prepare tripolyphosphate/chitosan complex beads for controlled release drug delivery, Int. J. Pharm., 2000, 201, 51–58 CrossRef CAS PubMed.
- F. C. Wu, R. L. Tseng and R. S. Juang, Comparative adsorption of metal and dye on flake- and bead-types of chitosans prepared from fishery wastes, J. Hazard. Mater., 2000, 73, 63–75 CrossRef CAS PubMed.
- A. K. Anal, D. Bhopatkar, S. Tokura, H. Tamura and W. F. Stevens, Chitosan-alginate multilayer beads for gastric passage and controlled intestinal release of protein, Drug Dev. Ind. Pharm., 2003, 29, 713–724 CrossRef CAS PubMed.
- F. L. Mi, H. W. Sung and S. S. Shyu, Drug release from chitosan-alginate complex beads reinforced by a naturally occurring cross-linking agent, Carbohydr. Polym., 2002, 48, 61–72 CrossRef CAS.
- M. Li, Z. Wang and B. Li, Adsorption behaviour of congo red by cellulose/chitosan hydrogel beads regenerated from ionic liquid, Desalin. Water Treat., 2016, 57, 16970–16980 CAS.
- R. D. Rogers and K. R. Seddon, Ionic liquids–solvents of the future?, Science, 2003, 302, 792–793 CrossRef PubMed.
- K. R. Seddon, Ionic liquids: a taste of the future, Nat. Mater., 2003, 2, 363–365 CrossRef CAS PubMed.
- T. Welton, Room-temperature ionic liquids. Solvents for synthesis and catalysis, Chem. Rev., 1999, 99, 2071–2084 CrossRef CAS PubMed.
- C. D. Hubbard, P. Illner and R. van Eldik, Understanding chemical reaction mechanisms in ionic liquids: successes and challenges, Chem. Soc. Rev., 2011, 40, 272–290 RSC.
- R. P. Swatloski, S. K. Spear, J. D. Holbrey and R. D. Rogers, Dissolution of cellose with ionic liquids, J. Am. Chem. Soc., 2002, 124, 4974–4975 CrossRef CAS PubMed.
- S. S. Silva, J. F. Mano and R. L. Reis, Ionic liquids in the processing and chemical modification of chitin and chitosan for biomedical applications, Green Chem., 2017, 19, 1208–1220 RSC.
- B. Li, J. Wang, M. E. Moustafa and H. Yang, Ecofriendly method to dissolve chitosan in plain water, ACS Biomater. Sci. Eng., 2019, 5, 6355–6360 CrossRef CAS.
- B. Li, J. Wang, Q. Gui and H. Yang, Drug-loaded chitosan film prepared via facile solution casting and air-drying of plain water-based chitosan solution for ocular drug delivery, Bioact. Mater., 2020, 5, 577–583 CrossRef PubMed.
- C. A. Schneider, W. S. Rasband and K. W. Eliceiri, NIH image to ImageJ: 25 years of image analysis, Nat. Methods, 2012, 9, 671–675 CrossRef CAS PubMed.
- J. Wang, H. He, R. C. Cooper and H. Yang,
In situ-forming polyamidoamine dendrimer hydrogels with tunable properties prepared via aza-Michael addition reaction, ACS Appl. Mater. Interfaces, 2017, 9, 10494–10503 CrossRef CAS PubMed.
- X. Sun, Z. Tang, M. Pan, Z. Wang, H. Yang and H. Liu, Chitosan/kaolin composite porous microspheres with high hemostatic efficacy, Carbohydr. Polym., 2017, 177, 135–143 CrossRef CAS PubMed.
- C. Liu, X. Liu, C. Liu, N. Wang, H. Chen, W. Yao, G. Sun, Q. Song and W. Qiao, A highly efficient, in situ wet-adhesive dextran derivative sponge for rapid hemostasis, Biomaterials, 2019, 205, 23–37 CrossRef CAS PubMed.
- A. Salam, J. J. Pawlak, R. A. Venditti and K. El-tahlawy, Synthesis and characterization of starch citrate−chitosan foam with superior water and saline absorbance properties, Biomacromolecules, 2010, 11, 1453–1459 CrossRef CAS PubMed.
- A. Salam, R. A. Venditti, J. J. Pawlak and K. El-Tahlawy, Crosslinked hemicellulose citrate–chitosan aerogel foams, Carbohydr. Polym., 2011, 84, 1221–1229 CrossRef CAS.
- A. Ayoub, R. A. Venditti, J. J. Pawlak, A. Salam and M. A. Hubbe, Novel hemicellulose–chitosan biosorbent for water desalination and heavy metal removal, ACS Sustainable Chem. Eng., 2013, 1, 1102–1109 CrossRef CAS.
- T. Dai, M. Tanaka, Y. Y. Huang and M. R. Hamblin, Chitosan preparations for wounds and burns: antimicrobial and wound-healing effects, Expert Rev. Anti-Infect. Ther., 2011, 9, 857–879 CrossRef CAS PubMed.
- R. A. Muzzarelli, Chitins and chitosans for the repair of wounded skin, nerve, cartilage and bone, Carbohydr. Polym., 2009, 76, 167–182 CrossRef CAS.
- M. Moniruzzaman and M. Goto, Ionic liquids: future solvents and reagents for pharmaceuticals., J. Chem. Eng. Jpn., 2011, 44, 370–381 CrossRef CAS.
- N. Adawiyah, M. Moniruzzaman, S. Hawatulailaa and M. Goto, Ionic liquids as a potential tool for drug delivery systems, MedChemComm, 2016, 7, 1881–1897 RSC.
Footnote |
† B. L. and J. W. contributed equally to this work. |
|
This journal is © The Royal Society of Chemistry 2020 |