pH-triggered solubility and cytotoxicity changes of malachite green derivatives incorporated in liposomes for killing cancer cells†
Received
27th May 2020
, Accepted 27th July 2020
First published on 28th July 2020
Abstract
Three different malachite green leuco derivatives (MG-Xs) are incorporated in liposomes. In all three cases, a substituent (X) is covalently linked to the central carbon atom, abbreviated as MG-OH, MG-OCH3, and MG-CN. The three MG-X compounds are solubilized separately in liposome membranes and become cationic (MG+) and water soluble under acidic conditions. MG+ is consequently released from the liposome to the aqueous exterior. Their release behavior corresponds to their ionization ability: MG-OH > MG-OCH3 > MG-CN. The cellular uptake of the liposomes, the cytotoxic effect, and the location of MG+ in cancer cells are investigated using murine cells derived from colon cancer (Colon 26 cells) and human embryonic kidney cells (HEK 293 cells). The toxic effect on cancer cells is correlated to the ionization ability of MG-Xs. The liposomes effectively deliver MG+via the endocytic pathway, resulting in the cytotoxicity of liposomes containing MG-OH which is higher than that of free MG-OH and MG+. The difference in the phospholipids constituting the liposome membranes barely had an effect on the ionization ratio and the cytotoxicity of MG-OH. Confocal fluorescence microscopic observations revealed that MG+ is ultimately transported into the nuclei after being released in acidic cellular compartments.
Introduction
Liposomes, which are microscopic spheroidal capsules composed of a phospholipid bilayer, are among the most investigated synthetic carriers for drugs for cancer therapeutics because they have many features desirable for drug-delivery systems including low toxicity, modifiable surfaces to achieve specificity for diseased cells, and tunable nanometer sizes (<200 nm) to exhibit the enhanced permeability and retention (EPR) effect for accumulation in tumor sites.1 The controlled release of a drug from liposomes is one approach to avoid toxic side effects manifesting in normal cells. Ideally, liposomes should retain a drug until needed which is after the liposomes are endocytosed by cancer cells or after external stimuli are applied. The pH value in bloodstreams is approximately 7.4 whereas the tumor microenvironment and endocytic compartments of cells show relatively acidic pH.2 Therefore, acidic pH levels have been used as signals for cancer-targeted delivery.3
Various pH-sensitive drug-delivery systems have been developed using liposomes, polymeric micelles,4–6 nanogels,7,8 and inorganic nanomaterials.9 Their pH sensitivity is based on the cleavage of acid-labile bonds,5 shrinkage and swelling,6,7,10 conformational change in capping agents,11 disassembling,12 and electrostatic interaction/repulsion.13 These pH-sensitive reactions are paired with drug carriers to result in the release of encapsulated drugs such as doxorubicin hydrochloride.6,8,9,12 In contrast, conventional chemotherapeutic agents have been conjugated with polymers to achieve sensitivity to acidic pH, where the cleavage of the conjugated bond triggers drug release in endocytic compartments.14 Because cationic lipids show high cytotoxicity toward mammalian cells,15 several attempts have been made to develop new lipids that become cationic in acidic cancer microenvironments while remaining non-cytotoxic in normal cells.16 Alternative novel antitumor drugs for targeted delivery are highly valued if they are prepared with ease and at a low cost. From this point, we focused on malachite green leuco derivatives (MG-Xs, Fig. 1) which are prepared through a one-step synthesis process from malachite green cations (MG+) which are widely used in the dye industry and as an antifungal agent. Acidic conditions promote the conversion of MG-X to MG+. For example, malachite green leuco carbinol base (MG-OH) exists solely as a carbinol at pH 10.1 and completely ionizes at pH 4.0 or lower.17 Early studies reported that MG+ was strongly cytotoxic to mammalian cells while leuco malachite green (X = H) exhibited low cytotoxicity.18 MG+ was found to induce free radical formation which creates reactive oxygen species, resulting in the observed toxicity of lipid peroxidation and damage to the mitochondria and nucleus.19,20 Although MG+ is soluble in water, to the extent of 66.67 g L−1 as the chloride salt,21 MG-Xs are lipophilic and relatively insoluble in water, with a solubility of 500 μg L−1 for MG-OH.17 Therefore, a strategy for pH-targeted delivery can be derived from the solubility change attributed to the conversion from its neutral (MG-X) to cationic (MG+) form, as well as the cytotoxic difference between them. In this work, we designed liposomes containing MG-Xs as shown in Fig. 1. MG-Xs are solubilized in the lipid membrane of the liposome until stimulated by acidic pH. They become cationic in the acidic compartments of cancer cells after endocytosis and are consequently released from the lipid membrane to the exterior, exhibiting cytotoxicity.
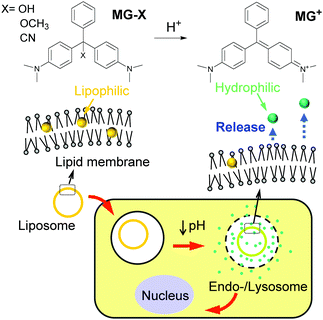 |
| Fig. 1 Chemical structure of malachite green leuco derivatives (MG-Xs) and their plausible anti-cancer behavior induced by the acidic microenvironment in cancer cells. | |
Results and discussion
Ionization of MG-Xs induced by acidic conditions
MG+ absorbs light in the visible light region while MG-Xs do not. The ionization of MG-Xs solubilized in the liposome membrane was thereby detected based on the absorbance at 622 nm (Fig. S1 in the ESI†). Fig. 2(A) shows that the ionization of MG-OH was enhanced with decreasing pH values and that the ionization reached a plateau within 40 min. MG-OCH3 exhibited similar pH-sensitive ionization which was modest compared to that of MG-OH (Fig. 2(B)). On the contrary, Fig. 2C shows that the absorbance of MG-CN was almost zero and remained unchanged even after 1 week under acidic conditions (pH 5.0–6.5). From these results, it can be stated that MG-OH and MG-OCH3 undergo ionization that is sensitive to the lowering of pH values while MG-CN ionization does not have pH sensitivity. The ionization difference can be attributed to the protonation ability of –OH and –OCH3 and the fact that –OH2+ and –OHCH3+ are good leaving groups. The MG-X derivatives exhibited similar pH-sensitive ionization in solutions excluding liposomes (Fig. S2 in the ESI†). The ionization ratio of MG-OH and MG-OCH3 in Fig. 2 was calculated using the calibration curves prepared for N,N,N′,N′-tetramethyl-4,4′-diaminotriphenylcarbenium oxalate (MG oxalate), as shown in Fig. S3 (ESI†). The lipophilic environment of MG-OH and MG-OCH3 in the liposome membrane was indicated by the formation of an exciplex with pyrene (Fig. S4 in the ESI†). The different lipids constituting the liposomes do not induce changes in the ionization ratio but they affect the ionization speed. A comparison between Fig. 2(A), (D) and (E) shows that 1-palmitoyl-2-oleoyl-sn-glycero-3-phosphocholine (POPC) liposomes induce ionization that is more rapid than that exhibited by 1,2-dimyristoyl-sn-glycero-3-phosphocholine (DMPC) liposomes and 1,2-dipalmitoyl-sn-glycero-3-phosphocholine (DPPC) liposomes. We attribute this to the mobility of MG-OH in the liposome membrane which is dependent on the phase transition temperature from gel phase to liquid crystalline phase: −3 °C for POPC, 23 °C for DMPC, and 41 °C for DPPC.22
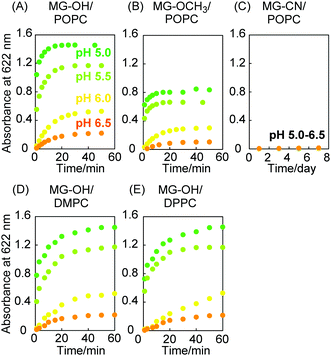 |
| Fig. 2 Time dependent absorbance at 622 nm for MG-X liposomes (50 μL) after mixing with buffer solutions (950 μL) at various pH levels. The liposomes were prepared from PBS (pH 7.4) and mixed with buffer solutions at pH 5.0, 5.5, 6.0, and 6.5. [MG-X]/[Lipid] = 0.1. [Lipid] = 9.3–9.8 mM. The measurements were taken at room temperature. (A) MG-OH/POPC liposome, (B)MG-OCH3/POPC liposome, (C) MG-CN/POPC liposome, (D) MG-OH/DMPC liposome, and (E) MG-OH/DPPC liposome. | |
Release of MG+ from liposomes
After MG-OH and MG-OCH3 were found to be pH-sensitive, we investigated the release of MG+ from liposomes under acidic conditions (Fig. 3). The release was dependent on pH; the release percentage at pH 5.0 was bigger than that at pH 6.0. We did not find any significant difference among the lipids constituting the liposomes (Fig. 3(A)). Therefore, the phase transition temperature of the liposome membrane is considered to not have a dominating effect on the MG+ release. MG-OH/POPC liposomes show a higher release percentage compared to MG-OCH3/POPC liposomes (Fig. 3(B)). These results are in agreement with the fact that MG-OH/POPC liposomes show higher pH-sensitivity to ionization compared to MG-OCH3/POPC liposomes (Fig. 2). We also performed the releasing experiments with MG-CN/POPC liposomes, but the released MG+ was hardly detected (data not shown). The difference in the molar ratio of [MG-X]/[lipid] (between 0.1 and 0.03) did not induce any change in the release percentage under a pH of 5.0. At pH 6.0, the higher ratio may increase the release percentage slightly in the cases of both MG-OH/POPC liposomes and MG-OCH3/POPC liposomes. It may be explained by the increase in the ratio of [MG-X]/[POPC] which shifts the distribution of MG+ from the lipophilic membrane to the aqueous solution.
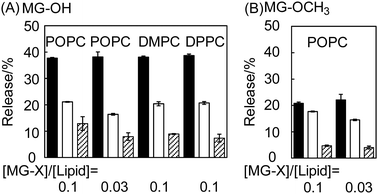 |
| Fig. 3 Released MG+ from MG-OH liposomes (A) and MG-OCH3 liposomes (B) under acidic conditions. Closed bars: at pH 5.0, open bars: at pH 6.0, hatched bars: at pH 6.5. Data are presented as the average (n = 3) ± standard deviation. | |
Viability of cancer cells
The cytotoxicity of MG-X liposomes was evaluated using an MTT assay. MG oxalate, as a cationic form, was examined to compare with MG-Xs. From Fig. 4(A), MG-OCH3/POPC liposomes displayed decreased cell viability with increasing malachite green concentration while MG-CN/POPC liposomes showed a slight decrease in cell viability. It is well explained by the pH sensitivity of these liposomes as shown in Fig. 2. Neutral malachite green (MG-CN) was found to be less cytotoxic than MG+. MG-OH liposomes, which exhibited the highest pH sensitivity and releasing properties among liposomes in this work, showed the highest cytotoxicity (Fig. 4(B) and (C)). It should be noted that the cytotoxicity of both MG-OH/POPC liposomes and MG-OCH3/POPC liposomes is remarkably higher than that of MG oxalate (IC50 = 6 μM for the MG-OH/POPC liposome, 9 μM for the MG-OCH3/POPC liposome, and 30 μM for MG oxalate). In other words, effective delivery of malachite green was carried out using liposomes. In order to investigate the effect of the liposome carrier, we conducted experiments using MG-OH and MG-OCH3 solutions (free MG-OH and MG-OCH3 in Fig. 4(B)). It is interesting to note that cell viability was almost 100% in the concentration range measured even though these compounds are pH sensitive and ionized under acidic conditions. It means that these compounds, which are originally non-cytotoxic, became cytotoxic when they were incorporated in the membrane and stimulated by acidic conditions. As a result of the significantly increased acidity reported for the cell endosome/lysosome compartments,23 the MG-OH/POPC liposomes and MG-OCH3/POPC liposomes were considered to be endocytosed while free MG-OH and MG-OCH3 were not. We then examined the endocytosis of the MG-OH/POPC liposome using flow cytometry (Fig. 4(D)). Colon 26 cells were incubated with liposomes at 4 °C to inhibit all endocytic pathways. The fluorescence intensity of rhodamine, used for labeling liposomes, in Colon 26 cells was reduced by incubation at 4 °C. This result indicated that MG-OH/POPC liposomes were internalized by Colon 26 cells via endocytosis.
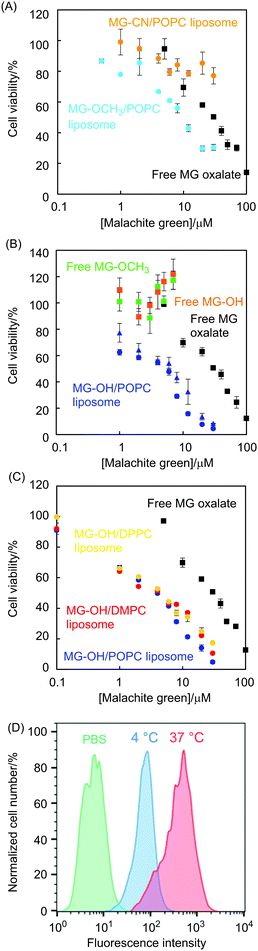 |
| Fig. 4 Cell viability of Colon 26 cells (A and B) and HEK 293 cells (C) treated with MG oxalate solution, MG-OH solution, MG-OCH3 solution, and MG-X liposomes. The MG-OH and MG-OCH3 samples were prepared by dissolving them in PBS solution (pH 7.4) containing 1 v/v% DMSO. Circles: [MG-X]/[lipid] = 0.1, triangles: [MG-X]/[lipid] = 0.03. The concentration of malachite green is expressed as the total concentration of cationic and neutral forms. Each data point is the average ± standard deviation of three different experiments. Flow cytometric measurement of the MG-OH/POPC liposome labeled by rhodamine uptake by Colon 26 cells (D). | |
Although free MG oxalate is cytotoxic, it was not endocytosed and its transportation to the nucleus was considered to be less effective than that of MG+ resulting from the MG-OH/POPC liposomes. As shown from the comparison of the MG-OH/POPC liposomes with [MG-OH]/[POPC] = 0.1 and 0.03, the molar ratio did not affect their cytotoxicity (blue circles and triangles in Fig. 4(B)). This result corresponds to the releasing properties of MG-OH/POPC liposomes at pH 5.0 observed from Fig. 3(A). We also examined the viability of HEK 293 cells treated with MG-OH/POPC liposomes, MG-OH/DMPC liposomes, and MG-OH/DPPC liposomes (Fig. 4(C)). Any significant difference between liposome lipids was not found to affect the cell viability, which is also in agreement with the results observed from Fig. 3(A).
Observation of Colon 26 cells
After being internalized into the cells, the MG-OH/POPC liposomes can release MG+ into the cytoplasm when triggered by an acidic pH signal at the endosomes/lysosomes. We further evaluated the internalization of MG+ released from the liposomes using confocal laser microscopy (Fig. 5). Triphenylmethane dye cations, including MG+, exhibit weak fluorescence, and viscous, cold environments or aptamers have been reported to enhance the fluorescence.24 We also found that the neutral malachite green derivatives showed no fluorescence peak via excitation at 590 nm while MG+ exhibited a peak at 656 nm in the presence of DNA.25 We obtained the fluorescence spectra of the MG-OH/POPC liposomes after incubation with Colon 26 cells and confirmed that a peak appeared at approximately 670 nm (Fig. S5 in the ESI†). Fig. 5 indicates that the majority of MG+ was localized in the nucleus and weak emissions from MG+ were observed from the acidic compartments stained using LysoTracker. This indicates that MG+ was finally transported into the nucleus after it was released in the acidic compartments such as endosomes/lysosomes. The MG+ in the nucleus is also in agreement with the reported evidence that triphenylmethane dyes can interact with DNA after entering the cell.26Fig. 6 shows the confocal laser microscope images of Colon 26 cells incubated with various amounts of MG-OH/POPC liposomes. The red spots in the nucleus corresponding to MG+ were hardly observed at the amount of MG-OH/POPC liposome less than 25 μL, above which the number of red spots increased with increasing MG-OH concentration. A good relationship between DNA damage and cytotoxicity to Chinese hamster ovary cells was also reported,20 and, therefore, the red spots in the nucleus may explain the effective cytotoxicity of MG-OH/POPC liposomes which is higher than that of free MG oxalate observed in Fig. 4(B) and (C).
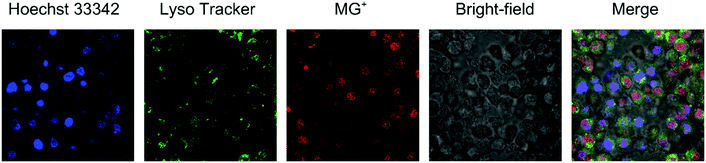 |
| Fig. 5 Confocal laser microscope images of Colon 26 cells incubated with MG-OH/POPC liposomes at 37 °C for 2 h. The amount of MG-OH/POPC liposome = 100 μL. The pH value of the medium was 7.25. | |
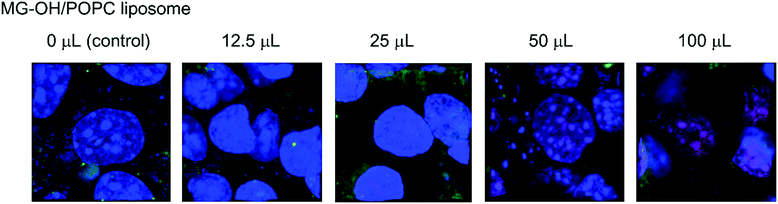 |
| Fig. 6 Confocal laser microscope images of Colon 26 cells treated with varying amounts of MG-OH/POPC liposomes. The pH value of the medium was 7.25. | |
Experimental
Materials
POPC, DMPC, and DPPC were purchased from NOF Corporation (Tokyo, Japan). Bis(4-(dimethylamino)phenyl)(4-phenyl)methyl cyanide (MG-CN) was synthesized as reported previously.27 Malachite green carbinol base (MG-OH) was obtained from the hydroxylation of malachite green carbinol hydrochloride and recrystallized from hexane.28 To obtain bis(4-(dimethylamino)phenyl)(4-phenyl)methyl methoxide (MG-OCH3), malachite green carbinol hydrochloride (2.48 g) was solubilized in distilled methanol (55 mL), and then 8 mL of a methanol solution containing 28% NaOCH3 was added to the solution. After the green solution turned colorless while mixing for about 1 h at room temperature, the resulting crude precipitate was recrystallized from hexane. MG-OCH3 (0.499 g, 40%) as a white solid. 1H NMR (400 MHz, CDCl3, δ): 2.92 (s, 12H, NCH3), 3.04 (s, 3H, OCH3), 6.63 (d, J = 9.2 Hz, 4H; o-H of NPh), 7.13 (t, J = 7.0 Hz, 1H; p-H of CPh), 7.23 (t, J = 4.0 Hz, 2H; m-H of CPh), 7.24(d, J = 9.2 Hz, 4H; m-H of NPh), 7.45 (d, J = 7.2 Hz, 2H; o-H of CPh).
Preparation of liposomes containing MG-X (MG-X liposomes)
Liposomes were prepared using the film hydration method, and MG-X (5.0 μmol or 1.7 μmol) and lipids (50 μmol) were dissolved in chloroform (30 mL). The chloroform was removed via evaporation in a rotary evaporator. The residual lipid film was dried for 2 h under vacuum conditions and was hydrated using a phosphate buffered saline (PBS) solution (0.1 M, pH 7.4) (5.0 mL). The suspension was subjected to five cycles of freezing and thawing prior to extrusion to achieve a diameter of 100 nm. The concentrations of the lipids were measured using the LabAssay™ Phospholipid kit (Wako Pure Chemical Industries, Osaka, Japan) and the final lipid concentration of liposomes was 9.3–9.8 mM. The size and structure of the prepared liposomes were observed using cryo-transmission electron microscopy (TEM). Closed unilamellar structures with diameters ranging from 50 to 120 nm were confirmed (see Fig. S6 in the ESI†). The size distribution of the liposomes was in agreement with the results obtained using dynamic light scattering (see Fig. S7 in the ESI†).
Ionization of MG-X under acidic conditions
The MG-X liposome sample (50 μL) was mixed with a buffer solution (950 μL) at various pH levels and the absorption spectra of the mixture was immediately measured at room temperature. Acetate buffer solutions (0.1 M, pH 5.0 and 5.5) and phosphate buffer solutions (0.1 M, pH 6.0 and 6.5) were used. The final pH values of the mixtures were almost unchanged from those of the original buffer solutions (ΔpH is within ±0.05).
Measurement of MG+ released from liposomes
The measurements were made at room temperature and performed using a gel chromatograph (AKTAprime plus; GE Healthcare, Fairfield, CT, USA) equipped with a column packed with a Sephacryl™ S-400 HR (GE Healthcare, Fairfield, CT, USA). The MG-X liposome (1.5 mL) was added to a buffer solution (3.5 mL) at various pH levels. After 30 min, the mixture was applied to the chromatograph and the buffer solutions used for the mixture were used as the mobile phase with a flow rate of 5.0 mL min−1. The detection wavelength was 546 nm. The MG+ encapsulated in the liposome was detected at a retention time of 15–25 min while the peak of free MG+ appeared at approximately 90 min (the typical chromatogram is shown in Fig. S8, ESI†). The amount of MG+ released was calculated from the peak area at approximately 90 min using the calibration curves prepared for MG oxalate. The percentage released is given by the relationship: %release = amount of released MG+/amount of MG-X in original liposomes.
Cells and cell culture
Colon 26 cells and HEK 293 cells were provided by the RIKEN BRC through the National Bio-Resource Project of the MEXT, Japan and National Institutes of Biomedical Innovation, Health and Nutrition, Japan, respectively. The cells were cultured with Eagle's minimum essential medium (E-MEM) containing 10% fetal bovine serum (FBS) in a humidified atmosphere of 5.0% CO2 at 37 °C.
Cytotoxicity assay
The cytotoxicity of MG-X liposomes, MG-OH, MG-OCH3, and MG oxalate was evaluated using an MTT assay. The samples for MG-OH and MG-OCH3 were prepared by dissolving them in a PBS solution containing 1 v/v% DMSO. MG oxalate was dissolved in a PBS solution. An MTT assay was performed using a CellTiter 96® Non-Radioactive Cell Proliferation Assay kit (Promega, Fitchburg, WI, USA). Colon 26 cells and HEK 293 cells were used for this assay. The cells were seeded on 96-well culture plates (100 μL, 2.0 × 105 cells per mL) and cultured for 24 h in E-MEM supplemented with 10% FBS in an incubator under a humidified atmosphere of 5% CO2 at 37 °C. The cell culture medium was removed and the sample solutions (10 μL) at various concentrations of malachite green derivatives and E-MEM with 10% FBS (90 μL) were added to the wells. Instead of the sample solution, a PBS solution was added as a control. After further incubation for 24 h in a humidified atmosphere of 5% CO2 at 37 °C, MTT solution (5 mg mL−1, 15 μL) was added to each well, and the plate was incubated for 4 h. After the addition of the solubilization solution/stop mix (100 μL), the plates were kept for 1 h under dark conditions at room temperature, and the absorbance of each well was measured using a microplate reader (Bio-Rad, CA, USA) at 550 nm and 655 nm. The cell viability was calculated using the following equation: %cell viability = (A550 − A655)/(A550(0) − A655(0)), where A550 and A655 are the absorbance values at 550 nm and 655 nm, respectively, when the cells were treated with the sample, and A550(0) and A650(0) are the absorbance values at 550 nm and 655 nm, respectively, when the cells were treated with PBS.
Flow cytometric measurement
For this measurement, liposomes were prepared from POPC (10 mM), MG-OH (10 μM), and N-(lissamine rhodamine B sulfonyl)phosphatidylethanolamine (10 μM). Colon 26 cells were seeded on 6-well culture plates (2 mL, 2.0 × 105 cells per mL) and cultured for 24 h in E-MEM supplemented with 10% FBS in an incubator (37 °C, 5% CO2, and a humidified atmosphere). After the culture medium was replaced, the mixture of the fresh medium (1.8 mL) and the liposome sample (200 μL) was added to the cells. The cells were then further incubated for 2 h. In order to inhibit the endocytic pathways, separate experiments were performed with the cells incubated at 4 °C. The control experiment was also performed using PBS instead of liposomes with an incubation temperature of 37 °C. These cells were then treated with trypsin, followed by double washing with PBS. The fluorescence intensities of rhodamine in the cells were measured using a flow cytometer Muse® Cell Analyzer (Merck Millipore, Darmstadt, Germany).
Observations of MG+ in cells using a confocal laser microscope
Colon 26 cells (2 × 105 cells per mL) were cultured in 10% FBS containing E-MEM (2 mL). After incubation for 24 h in a humidified atmosphere of 5% CO2 at 37 °C, the culture medium was replaced, and then 10% FBS containing E-MEM (1.8 mL) and MG-OH/POPC liposome samples (200 μL) were added to Colon 26 cells and further incubated for 2 h. The MG-OH/POPC liposome samples were prepared by mixing MG-OH/POPC liposomes ([MG-OH]/[POPC] = 0.1) and the PBS solution at various ratios. Additional incubation for 30 min was performed after staining with Hoechst 33342 (DOJINDO, Kumamoto, Japan) and LysoTracker™ Green DND-26 (Thermo Fisher, MA, USA), and then Colon 26 cells were observed via confocal laser microscopy (A1R +; Nikon, Tokyo, Japan) using the following filters: λex = 352 nm and λem = 461 nm for Hoechst 33342; λex = 504 nm and λem = 511 nm for LysoTracker; and λex = 590–595 nm and λem = 655–660 nm for MG+.
Conclusions
We reported on using pH-sensitive liposomes containing neutral MG-Xs to kill cancer cells. The pH-sensitivity was based on the differences in solubility and cytotoxicity between MG-Xs and MG+. MG-OH liposomes exhibited the highest ionization and MG+ releasing efficiency and, consequently, the highest cytotoxicity among the MG-X liposomes in this work: 38% release at pH 5.0 and IC50 = 4–6 μM for Colon 26 cells and HEK 293 cells. MG-OH liposomes were internalized by cancer cells via the endocytic pathway and MG+ cations were located in the nucleus. The fluorescence intensity of MG+ in the nucleus depended on the concentration of the treated MG-OH liposomes. These findings suggest that the pH-triggered release of cytotoxic MG+ could be a new approach for anticancer therapy with reduced systemic side effects. An improvement of the retention and targeting properties of MG-X liposomes by conjugation with hydrophilic polymers and antibodies is planned. Future work will include an investigation into their therapeutic performance in vivo.
Conflicts of interest
There are no conflicts to declare.
Acknowledgements
Funding for this study was provided by the National Institute of Technology, Nara College. A part of this work was conducted at the Nara Institute of Science and Technology in the Nara Institute of Science and Technology Nano Technology Hub for a “Nanotechnology Platform Project” sponsored by the Ministry of Education, Culture, Sports, Science, and Technology (MEXT), Japan. R. M. Uda also acknowledges the JST Initiative for Realizing Diversity in the Research Environment, Japan for financial support.
Notes and references
- Y. Matsumura and H. Maeda, Cancer Res., 1986, 46, 6387 Search PubMed
; J. Fang, H. Nakamura and H. Maeda, Adv. Drug Delivery Rev., 2011, 63, 136 CrossRef CAS PubMed
.
- C. Wang, T. Zhao, Y. Li, G. Huang, M. A. White and J. Gao, Adv. Drug Delivery Rev., 2017, 113, 87 CrossRef CAS PubMed
.
- E. S. Lee, Z. Gao and Y. H. Bae, J. Controlled Release, 2008, 132, 164 CrossRef CAS PubMed
.
- J. D. Wallat, J. K. Harrison and J. K. Pokorski, Mol. Pharmaceutics, 2018, 15, 2954 CrossRef CAS PubMed
.
- X. Zhang, Y. Huang, M. Ghazwani, P. Zhang, J. Li, S. H. Thorne and S. Li, ACS Macro Lett., 2015, 4, 620 CrossRef CAS
.
- J. Liu, Q. Liu, C. Yang, Y. Sun, Y. Zhang, P. Huang, J. Zhou, Q. Liu, L. Chu, F. Huang, L. Deng, A. Dong and J. Liu, ACS Appl. Mater. Interfaces, 2016, 8, 10726 CrossRef CAS PubMed
.
- L. Ding, Y. Jiang, J. Zhang, H.-A. Klok and Z. Zhong, Biomacromolecules, 2018, 19, 555 CrossRef CAS PubMed
.
- E. S. Lee, D. Kim, Y. S. Youn, K. T. Oh and Y. H. Bae, Angew. Chem., Int. Ed., 2008, 47, 2418 CrossRef CAS PubMed
.
- W. Cheng, J. Nie, L. Xu, C. Liang, Y. Peng, G. Liu, T. Wang, L. Mei, L. Huang and X. Zeng, ACS Appl. Mater. Interfaces, 2017, 9, 18462 CrossRef CAS PubMed
; Y.-J. Cheng, S.-Y. Qin, Y.-H. Ma, X.-S. Chen, A.-Q. Zhang and X.-Z. Zhang, ACS Biomater. Sci. Eng., 2019, 5, 1878 CrossRef
.
- S.-M. Lee, R. W. Ahn, F. Chen, A. J. Fought, T. V. O’Halloran, V. L. Cryns and S. T. Nguyen, ACS Nano, 2010, 4, 4971 CrossRef CAS PubMed
; R. Kouser, A. Vashist, M. Zafaryab, M. A. Rizvi and S. Ahad, ACS Appl. Bio. Mater., 2018, 1, 1810 CrossRef
.
- X. Chen, T. Chen, L. Ren, G. Chen, X. Gao, G. Li and X. Zhu, ACS Nano, 2019, 13, 7333 CrossRef CAS PubMed
; R. Guillet-Nicolas, A. Popat, J.-L. Bridot, G. Monteith, S. Z. Qiao and F. Kleitz, Angew. Chem., Int. Ed., 2013, 52, 2318 CrossRef PubMed
.
- X. Zhou, L. Xu, J. Xu, J. Wu, T. B. Kirk, D. Ma and W. Xue, ACS Appl. Mater. Interfaces, 2018, 10, 35812 CrossRef CAS PubMed
.
- Q. Zhang, F. Liu, K. T. Nguyen, X. Ma, X. Wang, B. Xing and Y. Zhao, Adv. Funct. Mater., 2012, 22, 5144 CrossRef CAS
; S. Angelos, N. M. Khashab, Y.-W. I. Yang, A. Trabolsi, H. A. Khatib, J. F. Stoddart and J. I. Zink, J. Am. Chem. Soc., 2009, 131, 12912 CrossRef PubMed
; L. Han, C. Tang and C. Yin, ACS Appl. Mater. Interfaces, 2016, 8, 23498 CrossRef PubMed
.
- J. Su, F. Chen, V. L. Cryns and P. B. Messersmith, J. Am. Chem. Soc., 2011, 133, 11850 CrossRef CAS PubMed
; J.-Z. Du, X.-J. Du, C.-Q. Mao and J. Wang, J. Am. Chem. Soc., 2011, 133, 17560 CrossRef PubMed
; Y. Ma, P. He, X. Tian, G. Liu, X. Zeng and G. Pan, ACS Appl. Mater. Interfaces, 2019, 11, 23948 CrossRef PubMed
; L. Huang, K. Tao, J. Liu, C. Qi, L. Xu, P. Chang, J. Gao, X. Shuai, G. Wang, Z. Wang and L. Wang, ACS Appl. Mater. Interfaces, 2016, 8, 6577 CrossRef PubMed
.
- S. Zhang, S. Ding, J. Yu, X. Chen, Q. Lei and W. Fang, Langmuir, 2015, 31, 12161 CrossRef CAS PubMed
; N. H. Park, W. Cheng, F. Lai, C. Yang, P. Florez de Sessions, B. Periaswamy, C. W. Chu, S. Bianco, S. Liu, S. Venkataraman, Q. Chen and Y. Y. Yang, J. Am. Chem. Soc., 2018, 140, 4244 CrossRef PubMed
.
- Y. Chang, Z. Huang, Y. Jiao, J.-F. Xu and X. Zhang, ACS Appl. Mater. Interfaces, 2018, 10, 21191 CrossRef CAS PubMed
; A. Garu, G. Moku, S. K. Gulla, D. Pramanik, B. K. Majeti, P. P. Karmali, H. Shaik, B. Sreedhar and A. Chaudhuri, ACS Biomater. Sci. Eng., 2015, 1, 646 CrossRef
.
- S. J. Culp and F. A. Beland, J. Am. Coll. Toxicol., 1996, 15, 219 CrossRef
.
- S. Clemmensen, J. C. Jensen, N. J. Jensen, O. Meyer, P. Olsen and G. Würtzen, Arch. Toxicol., 1984, 56, 43 CrossRef CAS PubMed
; C. Fernandes, V. S. Lalitha and K. V. K. Rao, Carcinogenesis, 1991, 12, 839 CrossRef PubMed
; L. Radko, M. Minta and S. Stypula-Trebas, Bull. Vet. Inst. Pulawy, 2011, 55, 347 Search PubMed
.
- A. Panandiker, G. B. Maru and K. V. K. Rao, Carcinogenesis, 1994, 15, 2445 CrossRef CAS PubMed
; G. Werth and A. Boituex, Arzneim. Forsch., 1967, 17, 1231 Search PubMed
; A. J. Kowaltowski, J. Turin, G. L. Indig and A. E. Vercesi, J. Bioenerg. Biomembr., 1999, 31, 581 CrossRef PubMed
; R. A. Mittelstaedt, N. Mei, P. J. Webb, J. G. Shaddock, V. N. Dobrovolsky, L. J. McGarrity, S. M. Morris, T. Chen, F. A. Beland, K. J. Greenlees and R. H. Heflich, Mutat. Res., 2004, 11, 127 Search PubMed
; T. Zahn and T. Braunbeck, Toxicol. In Vitro, 1995, 9, 729 CrossRef PubMed
.
- V. Fessard, T. Godard, S. Huct, A. Mourot and J. M. Poul, J. Appl. Toxicol., 1999, 19, 421 CrossRef CAS PubMed
.
-
J. E. F. Reynolds, Martindale. The Extra Pharmacopoeia, Pharmaceutical Press, London, 28th edn, 1982, p. 568 Search PubMed
.
-
D. Marsh, Handbook of Lipid Bilayers, CRC Press, Boston, 1990, pp. 135–158 CrossRef CAS
; R. Koynova and M. Caffrey, Biochim. Biophys. Acta, 1998, 1376, 91 CrossRef CAS
.
- S. Ohkuma and B. Poole, Proc. Natl. Acad. Sci. U. S. A., 1978, 75, 3327 CrossRef CAS PubMed
; A. Abiko and S. Masamune, Tetrahedron Lett., 1996, 37, 1081 CrossRef
.
- M. S. Baptista and G. L. Indig, J. Phys. Chem.
B, 1998, 102, 4678 CrossRef CAS
; J. R. Babendure, S. R. Adams and R. Y. Tsien, J. Am. Chem. Soc., 2003, 125, 14716 CrossRef PubMed
; L. A. Brey, G. B. Schuster and H. G. Drickamer, J. Chem. Phys., 1977, 67, 2648 CrossRef
.
- R. M. Uda and M. Ohshita, Biomacromolecules, 2012, 13, 1510 CrossRef CAS PubMed
; R. M. Uda, N. Nishimoto, T. Matsui and S. Takagi, Soft Matter, 2019, 15, 4454 RSC
.
- H. S. Rosenkranz and H. S. Carr, Br. Med. J., 1971, 3, 702 CrossRef CAS PubMed
.
- M. L. Hertz, J. Am. Chem. Soc., 1975, 97, 6777 CrossRef
.
- A. E. Karr, Text. Color., 1937, 59, 661 CAS
.
Footnote |
† Electronic supplementary information (ESI) available: Absorption spectra of MG-OH liposomes, time dependent absorption of MG-X in DMSO after mixing with a buffer solution, ionization ratio of MG-Xs in liposomes, fluorescence spectra of liposomes containing MG-X and pyrene, MG+ fluorescence in Colon 26 cells, cryo-TEM images of liposomes, DLS results of liposomes, chromatogram of MG-OH liposomes. See DOI: 10.1039/d0tb01346c |
|
This journal is © The Royal Society of Chemistry 2020 |