Colorimetric detection of urine glucose using a C/CdTe QDs–GOx aerogel based on a microfluidic assay sensor†
Received
6th February 2020
, Accepted 5th June 2020
First published on 5th June 2020
Abstract
A sensitive fluorescent microfluidic sensor based on carbon quantum dots (CQDs), cadmium telluride quantum dots (CdTe QDs) aerogel and glucose oxidase (GOx) for urinal glucose detection was fabricated via a simple method. CQDs, emitting green fluorescence (emission peak at 520 nm) and the CdTe QDs, emitting red fluorescence (emission peak at 620 nm) under 365 nm ultraviolet irradiation, were synthesized. The as-prepared CQDs, CdTe QDs and GOx (C/CdTe QD-GOx) were mixed in a certain ratio and were then introduced into the microfluidic chip to construct a three-dimensional porous aerogel sensor after freeze-drying. A ratiometric fluorescence response was realized through the quenching of the red fluorescence by H2O2 produced from the glucose/GOx reaction, while the green fluorescence intensity stays constant. A small change in the ratio of the two fluorescence intensities led to a clear change in the fluorescent colour of the sensor, which can be easily observed under a UV lamp. The microsensor displayed a serial colour change from red to green with the changes in glucose concentration. The intensity ratio between the red fluorescence and green fluorescence (R/G value) indicates the glucose concentration. In our experiments, the optimal concentration ratio of CdTe QDs and CQDs was 0.5
:
1, and the optimal response time was 5 min. The detection range of glucose concentration was from 0 mM to 13 mM, and the detection limit was 0.223 mM. We also found that this glucose sensor has excellent selectivity, specificity and stability. The as-prepared microsensor can be stably stored for 30 days under −20 °C. Such a C/CdTe QD-GOx aerogel based microfluidic assay sensor provides a new approach towards the optical and quantitative detection of glucose, which enables daily urine glucose monitoring for the diabetic patients in a convenient way.
Introduction
Governments and individuals spend hundreds of billions of dollars annually on diagnosing biopharmaceuticals because of expensive laboratory equipment and human expenditure. For example, more than 400 million people worldwide are suffering from diabetes since 2015, which is expected to be more than 600 million by 2045.1,2 For the self-management of diabetes mellitus and reduction of related complications, the regular monitoring and strict control of the blood glucose level are necessary.3,4 However, for the diabetic patients, it is extremely inconvenient and painful to undergo blood tests every few days. When glucose is present in the urine, it is very dangerous situation, which indicates severe diabetes. Considering convenience, painlessness and affordability, the urine glucose testing should not be completely ignored, particularly in the places where the economy is underdeveloped.
To date, various methods for analyzing glucose have been reported. Methods for detecting glucose by direct electrochemical oxidation have been studied.5–8 However, due to the toxic effects of the reaction medium, the conventional platinum or gold electrode has a low electrocatalytic activity towards the glucose oxidation,9,10 resulting in low sensibility, poor selectivity. In recent years, numerous studies have been conducted for the detection of glucose via fluorescence spectroscopy.11–14 Fluorescence spectroscopy has a relatively high sensitivity and a low detection limit, but the instrument used in this method is very expensive and unrealistic for mass use, and this method requires the complex pretreatment of the sample, which is not convenient. A fast, convenient, and low-cost method for detecting glucose is still needed. With the developments in the micro/nano material synthesis technology during the past decade, colorimetric sensors15–19 are receiving increasing attention because of their low cost, simple structure, and easy readout with the naked eye. Although colorimetric sensors have made great strides in detecting glucose quickly, conveniently, and at low cost, little research has been done on miniaturized optical biosensors.20–24 Compared with the traditional analytical equipment, the micro-biosensor has some advantages. The microsensor has the advantages of low sample consumption, low pollution, small size and convenient carrying,25,26 and also provides some protection for operators in dealing with toxic compounds. These are all very meaningful. So, we have the idea of developing a new miniature colorimetric sensor.
In this study, a ratiometric fluorescent microfluidic sensor compromising the dual-emission quantum dot aerogel with a porous structure and glucose oxidase was developed for glucose. The dual emission quantum dot aerogel was fabricated from two coloured QDs: water-soluble L-cysteine capped CdTe QDs with red emission, which are specifically sensitive to H2O2 and water-soluble amine capped CQDs with green emission, which are insensitive to H2O2. A ratiometric fluorescence response is realized upon the quenching of the red fluorescence by H2O2 produced by the glucose/GOx reaction while the green fluorescence intensity stays constant. A small change in the ratio of the two fluorescence intensities leads to a clear change in the fluorescence colour of the sensor, which can be easily observed with the naked eye under a UV lamp. As for the material selection, the CdTe QD synthesis is simple and the repeatability of the synthesis method is also good. It gives excellent fluorescence at 365 nm UV excitation and has the property of being quenched by the hydrogen peroxide, making it ideal for biosensing indicators. CQDs also have the advantages of simple synthesis and good fluorescence characteristics. It is not quenched by the hydrogen peroxide and is well suited in the colorimetric glucose sensor as the internal reference. We further demonstrate the utility of the ratiometric fluorescent sensor for the visualization of glucose in the urine samples. This microfluidic sensor can be highly promising in the realization of fast, portable, real-time and cheap device for urine glucose. Thus, such microfluidic chips can be conveniently applied for early screening of the diabetic patients.
Experimental
Reagents and materials
All the chemicals were of analytical grade. Triethyl citrate (Alfa Aesar), ethylenediamine (Shanghai Aladdin), 2.5 cadmium chloride hydrate (CdCl2·2.5H2O) (Alfa Aesar), sodium borohydride (NaBH4) (Alfa Aesar), L-glutathione (L-GSH) (Alfa Aesar), sodium tellurate (Na2TeO3) (Alfa Aesar), sodium hydroxide (NaOH) (Chengdu KESHI Company), isopropanol (C3H8O) (Chengdu KESHI Company), glucose (C6H12O6) (Sigma-Aldrich), uric acid (Sigma-Aldrich), urea (Shanghai Aladdin), ascorbic acid (Sigma-Aldrich), sucrose (Shanghai Aladdin), fructose (Shanghai Aladdin), maltose (Shanghai Aladdin), lactose (Shanghai Aladdin), human urine samples (Nanjing Zhongda Hospital), PBS buffer (Biosharp, 10 mM) were used as received. Ultrapure water (≥18 MΩ cm2) was prepared by a Millipore water purification system.
The fluorescence spectra were recorded on a Lengguang Tech F96 pro fluorescence spectrophotometer (Lengguang Tech, Shanghai, China). Fluorescence images were taken with a Canon 7D® digital camera (Canon, Tokyo, Japan) using a Qinke ZF-7A UV lamp (Qinke, Shanghai, China) as the light source.
Construction of the 3D porous C/CdTe quantum dot aerogels in microfluidic chips
The microfluidic sensor arrays contain five identical flow channel reactors to enable parallel measurements (Fig. S3, ESI†). Each flow channel reactor consists of a 12 mm long, 0.5 mm wide and 0.05 mm deep channel with a pair of inlet and outlet ports. The reactor chips outer dimensions are 30 mm in length, 26 mm in width, 1 mm in thickness.
The CdTe QDs and CQDs were synthesized according to the approach described in the literature.27,28 The original CdTe QD solution and CQD solution were diluted by a certain factor with ultrapure water as required, and then the diluted CdTe QD solution and CQD solution were mixed in a ratio of 1
:
1 by volume; 25 U GOx was added to the mixed solution at a rate of 100 μL. 1.4 μL of the mixed C/CdTe QD-GOx solution was injected into the microfluidic chip channel using a microsampler. Microfluidic chip will be done with −20 °C in the refrigerator frozen for 24 h; After the freeze was completed, the removal was immediately put into the freeze vacuum dryer for freeze-drying, which lasted for 3 h, and then the C/CdTe QDs–GOx aerogel microsensors was obtained.
Testing of urine samples
Urine samples of the patients were collected from the Zhongda hospitals. For each test, 0.8 μL sample solution was injected into each channel of the microsensor with a microsampler. Photoshop software was used to analyze the relative R/G value of the samples. The relative glucose concentration of the sample was calculated according to the corresponding detection characteristic curve, and then was compared with the reference value given by the hospitals.
Results and discussion
Detection principle of the C/CdTe QD-GOx aerogel microsensor
In order to construct the ratiometric fluorescent microfluidic sensor for glucose, the 3D porous C/CdTe QD-GOx aerogel was prepared in the microfluidic chip (the detailed information of the green CQDs and red QDs are shown in Fig. S1 (ESI†), the green CQDs show a fluorescence maximum at 520 nm, while the red CdTe QDs show a fluorescence peak at 620 nm). Due to FRET (detailed explanation can be seen in the ESI,† page 4) between the CdTe QDs and CQDs, the aerogel emit strong red fluorescence under 365 nm ultraviolet light, as illustrated in Scheme 1. When the urine glucose sample was added into the microfluidic sensor, glucose was oxidized and decomposed under the action of GOx to produce H2O2. The H2O2 quenches the red fluorescence emitted by the CdTe QDs, while the green fluorescence emitted by the CQDs is not affected by H2O2. The decrease in the red emission of the ratiometric probe results in the color change of the emission, facilitating the visual detection of the urine glucose. Glucose concentration is measured by the colorimetric ratio between the red and green fluorescence.
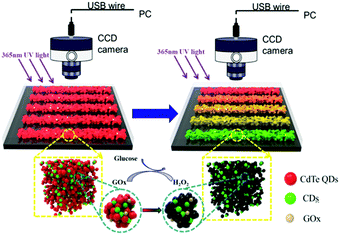 |
| Scheme 1 Principle of the C/CdTe-GOx aerogel microsensor to detect glucose. | |
Synthesis and characterization of the C/CdTe QD-GOx aerogel and the fabrication of microfluidic arrays sensors
As described in the Experiment section, a simple one-step method was utilized to fabricate the C/CdTe QD-GOx structure inside the microfluidic chips. Then, the sensor was characterized by SEM and photographs under the sun light and UV light. Fig. 1(a) is the scanning electron microscopy (SEM) image of the quantum dot aerogels. A note-worthy three dimensional porous structure of the aerogel was observed. This morphology has high porosity, high specific surface area, ultralow density, and could provide a very good catalytic environment for the glucose oxidase. These characteristics greatly increase the response rate and sensitivity of the sensor. Fig. 1(b) is an image of the microchannel sensor without access to any solution under incandescent light. It can be seen that there is no significant difference between each flow. Under the condition of 365 UV lamp irradiation, glucose solutions of different concentrations were introduced into the microchannel, and the microchannels were visually observed to show a gradual change from red to green, as shown in Fig. 1(c).
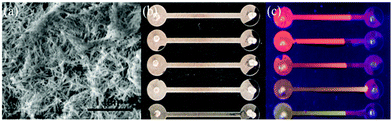 |
| Fig. 1 (a) SEM of aerogel of C/CdTe-GOx, (b) microchannel sensor under incandescent lamp, (c) microchannel sensor under ultraviolet lamp. | |
Validation of the sensing principle and optimization of the C/CdTe QD-GOx aerogel microsensor
Different sensors were prepared to verify the feasibility of detecting glucose by the aerogel microsensor. All the as-prepared aerogel microsensors had C/CdTe, except that some added GOx and some did not. After the preparation, different reagents were introduced therein as a control (the detailed information can be seen in Fig. S5, ESI†). As shown in Fig. S5 (ESI†), the fluorescent colour of the microsensor was changed only when there was GOx and glucose or H2O2. The results of the experiments fully prove that the detection of glucose by the C/CdTe-GOx aerogel microsensor is feasible.
In consideration of the requirement for visual and qualitative detection of the urine glucose, it is necessary to optimize the concentration of the CdTe QDs and CQDs in the sensor. The CdTe QD stock solution and the CQD stock solution were diluted by different factors, and were mixed according to the volume ratio of 1
:
1, followed by the addition of GOx. The concentration ratio of CdTe
:
C was set to 1
:
1; 1
:
0.5; 1
:
0.25; 0.5
:
1; 0.25
:
1; and 0.5
:
0.5. After several sets of control microsensors were made, we studied 0 mM and 10.8 mM glucose solution to observe the sensor's detection performance (the result is shown in the Fig. S6, ESI†). As can be seen from Fig. S6(a) (ESI†), only the C/CdTe-GOx aerogel microsensor fabricated with the concentration ratio of 0.5
:
1, showed red fluorescence in the presence of the 0 mM glucose sample and the colour turned yellow in the presence of 10.8 mM glucose sample; the colour change was not obvious for the other groups. As can be seen from Fig. S6(b) (ESI†), when the concentration ratio of the aerogel is 0.5
:
1, the change in the R/G value between the 0 mM glucose sample and the 10.8 mM glucose sample is the largest. This meant that the microsensor has the highest sensitivity and detection domain at a ratio of 0.5
:
1. So, we chose CdTe
:
C = 0.5
:
1 as the ratio of the C/CdTe-GOx aerogel microsensor and built the C/CdTe-GOx aerogel microsensor in the microfluidic chip.
In order to enable the sensor to quickly detect glucose and ensure its sensitivity, the best detection time was explored. 0.8 μL of 10.8 mM glucose solution was injected into each flow channel of the microsensor with a micro syringe, a fluorescence image was recorded every 2 min and the R/G value was read (the detailed information can be seen in Fig. S7, ESI†). It can be seen that as the reaction time increased, the R/G value gradually decreased and when the reaction time reached 5 min, the R/G value remained basically unchanged. Therefore, 5 min was chosen as the detection time.
Qualitative and quantitative detection of glucose in urine samples
The urine of a healthy volunteer was set as a blank sample and different amount of glucose was added into the healthy urine sample. Urine samples with different glucose concentrations were prepared, including 0 mM, 4.3 mM, 6.3 mM, 8.7 mM, 10.8 mM, 13 mM and 26 mM. These urine samples were then injected into the microsensor flow channel using a micro syringe, the fluorescence image was taken and the R/G values were analyzed. The experimental results are shown in Fig. 2(b). As can be seen from Fig. 2(b), the urine of different glucose concentrations showed significant differences in the fluorescence colour. When the glucose concentration was 10.8 mM, the fluorescence colour changed from red to yellow and when the urine glucose concentration exceeded 10.8 mM, the fluorescence colour changed from yellow to green.
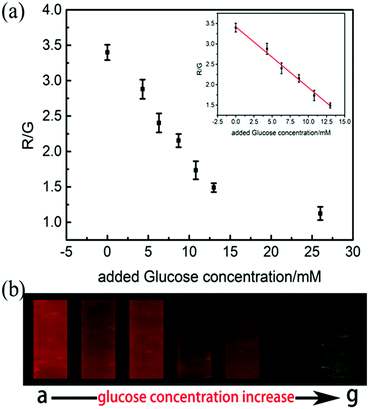 |
| Fig. 2 (a) Linear curve of detecting urine glucose. (b) Fluorescence images obtained by microsensors detecting urine containing different glucose concentrations (from left to right: (a) 0 mM, (b) 4.3 mM, (c) 6.3 mM, (d) 8.7 mM, (e) 10.8 mM, (f) 13 mM, (g) 26 mM). | |
In addition to visual inspection, the quantitative analysis of the urine glucose was also performed. Glucose concentration was defined as C and the R/G value of the microsensor fluorescence image was defined as I, and the I–C linear curve was plotted, as shown in Fig. 2(a). It demonstrated that within 0–13 mM the glucose concentration has a good linear relationship with the R/G value. The regression equation is:
| I = −0.14903C + 3.41992 (R2 = 0.99, n = 5) | (1) |
The sensor has a detection limit of 0.223 mM for urine glucose, which met the needs of urine glucose testing. The detection of glucose using different colorimetic sensor systems was compared (the detailed information can be seen in Table S1, ESI
†). From the Table S1 (ESI
†), we can see that the developed microsensor has a wide linear range and low detection limit.
Sensor selectivity verification
In addition, the selectivity of C/CdTe QD-GOx aerogel microsensors was also studied. A number of substances that may interfere with the microsensor were selected for specific validation, including sucrose, fructose, maltose, lactose, urea, uric acid, ascorbic acid. These sample solutions were separately injected into the microsensor flow path. The result is shown in the Fig. 3(a). It can be clearly seen that other similar interfering substances did not cause a large change in the R/G value of the microsensor fluorescence image. This confirmed that the C/CdTe QD-GOx aerogel microsensor has good selectivity for the human urine glucose detection. There is great potential for the screening of diabetes in clinic and for monitoring blood glucose in diabetic patients.
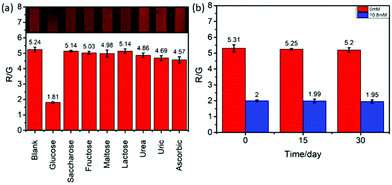 |
| Fig. 3 (a) Sensor selection specific verification, (b) sensor stability verification. | |
Sensor stability verification
For an effective sensor, the stability is also an important property to ensure that it can maintain a relatively stable performance after a period of manufacture. So the stability of the C/CdTe QD-GOx aerogel microsensor was also explored. The sensor placed in a −20 °C refrigerator for the storage and performance of the chip in presence of 0 mM and 10.8 mM glucose solution was tested every 15 days. The results are shown in Fig. 3(b). As can be seen from Fig. 3(b), the fluorescence image of the sensor did not change much even after 30 days of storage. The value of R/G remained basically the same and it can be concluded that the three-dimensional porous C/CdTe QD-GOx aerogel microsensor is very stable and can meet clinical use.
Real urine sample detection
Fresh urine from different healthy volunteers was collected to determine whether the microsensor has the effectiveness and reliability of urine glucose testing for different human urine. Glucose was added to the fresh urine to obtain urine samples with different glucose concentrations. 0.8 μL of these urine samples were injected into the microsensor flow path through a micro syringe, and the obtained results are shown in Table 1. It can be seen from Table 1 that the measured glucose concentration is basically the same as the glucose concentration in the sample, and has a good recovery.
Table 1 Determination of glucose in urine samples of different people (a: blank sample, b–e: urine samples of different volunteers after adding glucose)
Sample |
Spiked (mM) |
Found (mM) |
Recovery (%) |
Photo |
a |
Blank |
— |
— |
|
b |
3.50 |
3.82 |
109.04 |
|
c |
7.00 |
7.02 |
100.24 |
|
d |
10.50 |
11.34 |
108.03 |
|
e |
14.00 |
15.83 |
113.08 |
|
Further more, real urine samples were taken from the Zhongda Hospital to compare our sensors with commercial medical instruments.
The results are shown in Table 2, it can be seen that the microsensor has good consistency with the medical instruments (the sensor was developed to detect glucose in the urine, but in order to verify the superior performance of the sensor, we also measured the real serum samples, see the ESI,† Table S2 for detailed information).
Table 2 Commercial instruments (Uritest-500B automatic urine chemistry analyzer) and aerogel microsensor to measure the glucose value in real urine samples of patients
Sample |
Detected by our method (mM) |
Detected by hospital (mM) |
Photo |
1 |
0.39 |
− |
|
2 |
3.91 |
− |
|
3 |
11.08 |
+ |
|
4 |
15.88 |
++ |
|
5 |
17.81 |
++++ |
|
Conclusions
In this study, a visual detection of urine glucose using a C/CdTe QD-GOx aerogel microsensor was developed. A ratiometric fluorescence response is realized through the quenching of the red fluorescence by H2O2 produced from the glucose/GOx reaction, while the green fluorescence intensity stays constant. A small change in the ratio of the two fluorescence intensities leads to a clear change in the fluorescence colour of the sensor, which can be easily observed under a UV lamp. The optimal response time is 5 min, the detection range of glucose concentration is 0 mM to 13 mM, and the detection limit is 0.223 mM. All of which match the requirements for diabetes mellitus screening in clinical practice. We also found that this glucose sensor has excellent selectivity, specificity and stability. The as-prepared microsensor can be stably stored for 30 days under −20 °C. The C/CdTe QD-GOx aerogel based microfluidic assay sensor provides a new approach for the optical and quantitative detection of glucose, which enables daily urine glucose monitoring for the diabetic patients in a convenient way.
Conflicts of interest
There are no conflicts to declare.
Acknowledgements
This work was supported by the National Key Scientific Instrument and Equipment Development Project (Grant No. 51627808), the National Natural Science Foundation of China (Grant No. 51605088, 51505083), the National Key R&D Program of China (Grant No. 2016YFC1305700) and the Fundamental Re-search Funds for the Central Universities (2242017K3DN20).
Notes and references
- K. Ogurtsova, J. D. Da Rocha Fernandes, Y. Huang, U. Linnenkamp, L. Guariguata, N. H. Cho, D. Cavan, J. E. Shaw and L. E. Makaroff, Diabetes Res. Clin. Pract., 2017, 128, 40–50 CrossRef CAS PubMed.
- N. H. Cho, J. E. Shaw, S. Karuranga, Y. Huang, J. D. Da Rocha Fernandes, A. W. Ohlrogge and B. Malanda, Diabetes Res. Clin. Pract., 2018, 138, 271–281 CrossRef CAS PubMed.
- A. Daleen, American journal of critical care: an official publication, American Association of Critical-Care Nurses, 2006, 15, 370–377 CrossRef.
- T. Correa, F. P. D. Almeida, A. B. Cavalcanti, A. J. Pereira and E. Silva, Crit. Care, 2009, 13, P122 CrossRef.
- Y. Li, Y. Y. Song, C. Yang and X. H. Xia, Electrochem. Commun., 2007, 9, 981–988 CrossRef CAS.
- M. Ling, J. Juan, Y. Gaixiu, L. Tianhong, Z. Hui and C. Chenxin, Anal. Chem., 2009, 81, 7271 CrossRef.
- P. N. Bartlett and D. J. Caruana, Analyst, 1992, 117, 1287–1292 RSC.
- S. Sattayasamitsathit, P. Thavarungkul, C. Thammakhet, W. Limbut and P. Kanatharana, Electroanal, 2010, 21, 2371–2377 CrossRef.
- I. T. Bae, E. Yeager, X. Xing and C. C. Liu, J. Electroanal. Chem. Interfacial Electrochem., 1991, 309, 131–145 CrossRef CAS.
- X. A. Wei and P. B. Shen, Chin. J. Chem. Phys., 2003, 16, 395–400 CAS.
- E. L. Hull, N. I. Matter, B. P. Olson, M. N. Ediger, A. J. Magee, J. F. Way, K. E. Vugrin and J. D. Maynard, J. Clin. Transl. Endocrinol., 2014, 1, 92–99 CAS.
- J. Chao, Z. Yu, H. Shen and C. Liu, ChemistrySelect, 2017, 2, 9181–9185 CrossRef.
- H. Zheng, R. Su, Z. Gao, W. Qi, R. Huang, L. Wang and Z. He, Anal Methods, 2014, 6, 6352–6357 RSC.
- J. Wei, Q. Li, J. Ren, X. Ren and X. Meng, Anal Methods, 2014, 6, 1922–1927 RSC.
- Y. Zhou, X. Huang, C. Liu, R. Zhang, X. Gu, G. Guan, C. Jiang, L. Zhang, S. Du, B. Liu, M. Han and Z. Zhang, Anal. Chem., 2016, 88, 6105–6109 CrossRef CAS PubMed.
- R. Rashidi, J. Alenezi, J. Czechowski, J. Niver and S. Mohammad, Chem. Pap., 2019, 73, 2845–2855 CrossRef CAS.
- Y. Wang, C. Zhang, X. Chen, B. Yang, L. Yang, C. Jiang and Z. Zhang, Nanoscale, 2016, 8, 5977–5984 RSC.
- Y. Wang, Y. Zhu, S. Yu and C. Jiang, RSC Adv., 2017, 7, 40973–40989 RSC.
- H. Tao, S. Wen, C. Ke, G. Hongxi, N. Zhonghua and L. Shaoqin, Mater. Chem. Front., 2019, 3, 193–198 RSC.
- Y. E. Jiaming, J. Shao, P. Yang, Z. Fan, H. Feng, B. Chen and D. U. Hangen, Food Sci., 2017, 38, 292–297 Search PubMed.
- N. Xie, Z. Hao, L. Bo, J. Wu and T. Han, J. Opt., 2017, 19 Search PubMed.
- J. L. Li, D. Day and M. Gu, Lab Chip, 2010, 10, 3054–3057 RSC.
- T. Hu, J. Xu, Y. Ye, Y. Han, X. Li, Z. Wang, D. Sun, Y. Zhou and Z. Ni, Biosens. Bioelectron., 2019, 136, 112–117 CrossRef CAS PubMed.
- T. Hu, Y. Ye, K. Chen, F. Long, W. Sang, Y. Zhou, D. Sun and Z. Ni, Anal Methods, 2018, 10, 5749–5754 RSC.
- X. Chen, D. Cui and L. Zhang, Chin. Sci. Bull., 2009, 54, 324–327 CAS.
- J. Ducrée, Procedia Chem., 2009, 1, 517–520 CrossRef.
- T. Hu, B. P. Isaacoff, J. H. Bahng, C. Hao, Y. Zhou, J. Zhu, X. Li, Z. Wang, S. Liu, C. Xu, J. S. Biteen and N. A. Kotov, Nano Lett., 2014, 14, 6799–6810 CrossRef CAS PubMed.
- S. Paulo, E. Palomares and E. Martinezferrero, Nanomaterials, 2016, 6, 157 CrossRef PubMed.
Footnote |
† Electronic supplementary information (ESI) available. See DOI: 10.1039/d0tb00328j |
|
This journal is © The Royal Society of Chemistry 2020 |