DOI:
10.1039/D0SC03073B
(Edge Article)
Chem. Sci., 2020,
11, 9421-9425
Total synthesis of endiandric acid J and beilcyclone A from cyclooctatetraene†
Received
1st June 2020
, Accepted 16th July 2020
First published on 29th July 2020
Abstract
The endiandric acids are classic targets in natural product synthesis. The spectacular 8π/6π-electrocylisation/intramolecular Diels–Alder (8π/6π/IMDA) reaction cascade at the heart of their biosynthesis has inspired practitioners and students of pericyclic chemistry for nearly forty years. All previous synthetic approaches have sought to prepare a linear tetraene and thereby initiate the cascade. In this communication we demonstrate the use of cyclooctatetraene to rapidly intercept the 8π/6π/IMDA cascade at the cyclooctatriene stage. Endiandric acid J and beilcyclone A are prepared for the first time in six and five steps, respectively. The strategy features a tactical overall anti-vicinal difunctionalisation of cyclooctatetraene through SN2′ alkylation of cyclooctatetraene oxide followed by an intriguing tandem Claisen rearrangement/6π-electrocyclisation from the corresponding vinyl ether. This rapidly constructs an advanced bicyclo[4.2.0]octadiene aldehyde intermediate. Olefinations and intramolecular Diels–Alder cycloadditions complete the syntheses. This establishes a short and efficient new path to the endiandric acid natural products. DFT modelling predicts thermal racemisation of bicyclo[4.2.0]octadiene intermediates, dashing hopes of enantioselective synthesis.
Introduction
The endiandric acids are the first of a family of natural products characterised by the structure or intermediacy of a bicyclo[4.2.0]octadiene. Black and co-workers originally isolated and structurally elucidated endiandric acids A–G and proposed their biosynthesis:2 that the formation of a linear tetraene intermediate would initiate an 8π/6π-electrocyclic cascade reaction,1 followed by an intramolecular Diels–Alder (IMDA) reaction in either of two distinct modes to give complex tetracyclic scaffolds. The Nicoloau group promptly proved this concept in their classic 1982 synthesis.3 This work has become perhaps the most iconic example of pericyclic cascade reactions in biomimetic total synthesis.
More than 80 structurally related natural products have been isolated from the Beilschmiedia and Endiandra genera of plants,4 and a wide range of potent biological activities established. Synthetic efforts in the area have focused on the bacterial SNF4435 natural products,5 the sea mollusc bicyclo[4.2.0]octadienes natural products,6 and the kingianins,4 with elegant syntheses reported by the Parker,7 Trauner,8 Baldwin and Moses,9 and Sherburn and Lawrence10 groups.
The common feature of all previous synthetic approaches is the construction of a linear tetraene intermediate (Fig. 1a). Nicolaou adopted the semi-hydrogenation of dienediyne intermediates, Parker, Baldwin/Moses, and Trauner all adopted cross-coupling reactions, whereas Lawrence/Sherburn demonstrated the four-fold semi-hydrogenation of a linear tetrayne to give a Z,Z,Z,Z-tetraene intermediate. All previous approaches ultimately forge the 4–5 carbon–carbon bond through some kind of coupling reaction as a key step. While this is an intuitive and successful disconnection, it requires the often challenging stereoselective synthesis of situatable coupling partners.
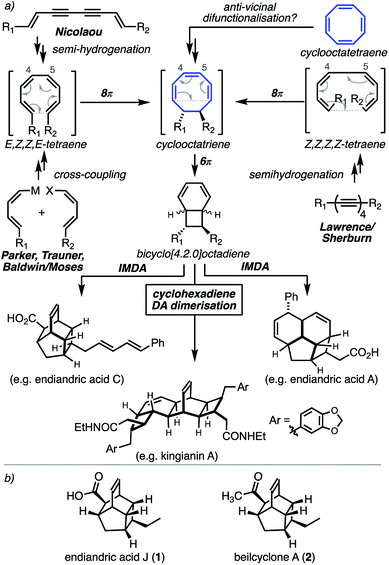 |
| Fig. 1 (a) Previous approaches to bicyclo[4.2.0]octadiene derived natural products. (b) Synthetic targets. | |
The use of π-rich hydrocarbons as key starting materials has been a powerful approach in natural product synthesis with reactive π-bonds providing the basis of dense functionalisation patterns.11 Annulenes have been used occasionally in this context. Examples include Snapper's use of cyclobutadiene in cycloaddition/cyclopropanation/rearrangement sequences in the synthesis of pleocarpenene and pleocarpenone.12 Sarlah recently reported a dearomative six-fold functionalisation of benzene in the synthesis of isocarbostyril alkaloids.13 Corey used cyclooctatetraene (COT) as the basis of his inspirational synthesis of the ladderane natural product pentacycloanammoxic acid.14
We realised that an anti-vicinal difunctionalisation of COT would intercept the 8π/6π cascade and potentially provide a rapid entry point to advanced bicyclo[4.2.0]octadiene intermediates (Fig. 1a). This concept was originally considered by the Nicolaou group, via a potential dialkylation of the bromination product of COT (Fig. 2b).15
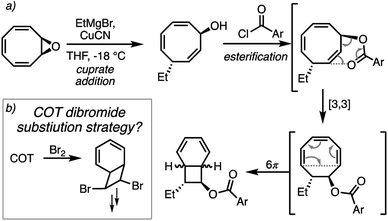 |
| Fig. 2 (a) Pineschi's alkylation of COT-oxide. (b) Addition of Br2 to COT. | |
Our recent work on the synthesis of bullvalenes16 prompted an appreciation of the hidden complexity and rich chemistry of COT.17 We were drawn to the work of Pineschi who established stereoselective and enantioselective anti-SN2′ addition ring-opening reactions of cyclooctatetraene oxide (Fig. 2a).18
They noted that esterification of the resultant cycloocta-2,4,7-trienol was occasioned by an unusual [3,3]-transposition of the ester, and subsequent 6π-electrocyclization to give the corresponding bicyclo[4.2.0]octadiene ester. By changing out the [3,3] ester transposition with a Claisen rearrangement, a new path to the 8π/6π natural products might be forged.
In this communication we demonstrate this concept through the synthesis of endiandric acid J 1 and beilcyclone A 2 (Fig. 1b). Isolated from the roots of Beilschmiedia erythrophloia, these structures differ only in the identity of the carbonyl function.19
Results and discussion
Our synthesis began with epoxidation of COT to give cyclooctatetraene oxide 3 in 71% yield (Scheme 1). This is followed by anti-SN2′ addition ring-opening of the epoxide using didecyl cuprate under Pineschi's conditions to give alcohol 4 in 96% yield, with complete regio- and stereo-selectivity. The allyl-pentadienyl-alcohol 4 is both acid sensitive and thermally unstable but could be purified by rapid flash chromatography on buffered silica gel.
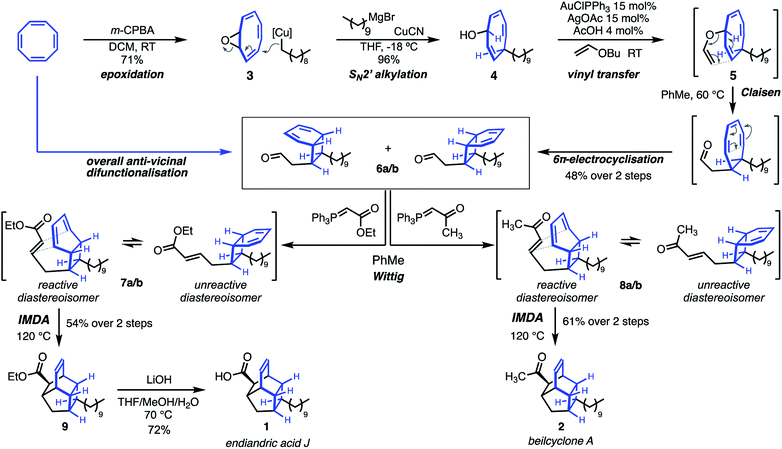 |
| Scheme 1 Synthesis of endiandric acid J and beilcyclone A. | |
The synthesis of vinyl ethers is a significant challenge in organic synthesis.20 The most widely used method employs mercury(II)-catalysed vinyl transfer, typically from an alkyl vinyl ether solvent.21 Reaction conditions generally require high catalyst loading, elevated temperatures, and extended reaction times. There are only several alternatives including palladium22 and iridium23 catalysed methods, both of which require elevated reaction temperatures.
In our hands, mercury(II)-catalysed vinyl transfer to alcohol 4 using either Hg(OAc)2 or Hg(TFA)2 was fraught with difficulty. Reactions suffered from low conversion, degradation, long reaction times, and poor reproducibility between runs.
Finally, modification of a gold(I)-catalysed protocol reported by Tokunaga24 using AuClPPh3 led to the successful synthesis of vinyl ether 5. Warming of the sensitive crude material in toluene at 60 °C prompted a Claisen/6π cascade to give aldehyde 6a/6b with complete regioselectivity and 1,2-anti stereospecificity as an inconsequential ∼2
:
1 mixture of diastereoisomers in 48% yield over 2 steps. This appears to be the first example of a tandem Claisen rearrangement/6π-electrocyclisation of a non-aromatic substrate.25 The transposition of the SN2′ addition into this cascade represents a tactical anti-vicinal difunctionalisation of COT, as well as setting the aldehyde as a key functional handle for endgame operations.
Wittig olefination of 6a/6b gave the α/β unsaturated ester 7a/7b and ketone 8a/8b, respectively as intermediates.26 Heating of the reaction mixtures at 120 °C promoted equilibration of the diastereoisomers through 6π-electrocyclic ring opening/closing and intramolecular Diels–Alder reaction to give beilcyclone A 2 in 61% yield, and tetracyclic ester 9 in 54% yield. Hydrolysis of the ester gave endiandric acid J 1 in 72% yield.
The 8π/6π natural products are all isolated as their racemates, or as mixtures of diastereoisomers with respect to bicyclo[4.2.0]octadienes fragments and another fixed element of stereogenicity. Despite this, the prospect of enantioselective chemical synthesis has remained an appealing open challenge. While there are a variety of good methods for enantioselective Nazarov reactions,27 as well as 6π-electrocyclic reactions,28 the question of enantioselective 8π-electrocyclic reactions is largely unsolved.29 Parker has reported a series of studies into stereoinduction of 8π/6π cascades using chiral auxiliaries, but diastereoisomer ratios were generally modest.30
By avoiding the interception of a linear tetraene, our strategy should in principle be applicable to enantioselectivity. Indeed, Pineschi's desymmetrisation of cyclooctatetraene oxide brings an enantioselective strategy into clear view. However, while perusing this goal, doubts grew as to the configurational stability of bicyclo[4.2.0]octadienes generally. While the dynamic relationship between endo and exo isomers through 6π-electrocyclic ring opening/closure is long known1,31 and would not destroy enantiopurity, transient 8π-electrocyclic ring opening to the corresponding linear tetraene certainly would. In a recent computational study,32 Houk predicted the 8π/6π transition state energies of the trans–trans-dimethyl-(E,Z,Z,E)-tetraene 8π/6π system at 91 kJ mol−1 and 95 kJ mol−1 relative to the cyclooctatriene, respectively. The finely balanced kinetics and thermodynamics of these 8π/6π systems warrants caution.
To anticipate the prospects for a successful enantioselective synthesis of 1 and/or 2 we conducted a computational study on truncated analogues of the Claisen/8π/6π cascade of vinyl ether 5, as well as the 8π/6π/IMDA cascade of involving esters 7a/b. The results of the latter analysis are presented in Fig. 3. The sequence was modelled using density functional theory calculations employing the M06-2X method and 6-311+G(d,p) basis set, which has been shown to give reliable thermochemistries for pericyclic reactions.33
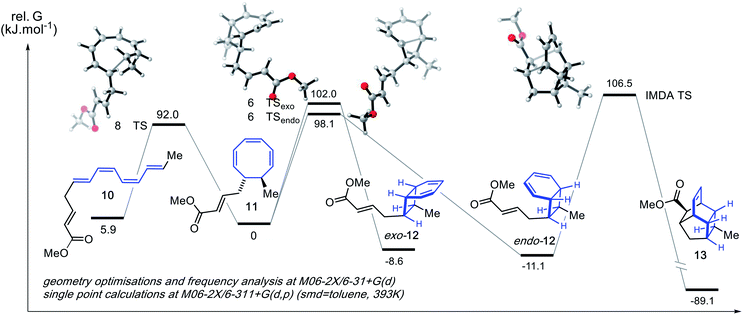 |
| Fig. 3 Computational analysis of the 8π/6π/IMDA cascade. | |
The predicted free energies of the 8π, 6πendo, 6πexo, and IMDA transition structures are predicted to be finely balanced, with the 8π electrocyclic reaction having the lowest barrier at 92.0 kJ mol−1. Unfortunately, we must conclude that under thermal reaction conditions, an enantiopure sample of the endo isomer of 16 (if it could be prepared) would almost entirely racemise prior to intramolecular Diels–Alder reaction. Conceivably a catalytic IMDA reaction might address this problem. However, the 8π/6π cascade of aldehyde 6a/b (not shown) is predicted to have a similar profile to that of 16 whereby an enantiopure sample of vinyl ether 5 would likely give rise to racemic 6a/b under the reaction conditions of its formation. This interpretation is aided by full kinetic modelling of these reaction sequences (see the ESI† for full details).
Conclusions
This study demonstrates a rapid new entry into the 8π/6π natural products through a distinctive synthetic strategy. This sets the stage for short and practical syntheses of other members of the family, as well as analogues. Computational analysis of the pericyclic cascades predicts the antipodal instability the bicyclo[4.2.0]octadiene intermediates and deters the pursuit of enantioselective synthesis in this case.
Conflicts of interest
There are no conflicts to declare.
Acknowledgements
We gratefully acknowledge the New Zealand Royal Society (Marsden Fund No. 15-MAU-154).
Notes and references
- R. Huisgen, A. Dahmen and H. Huber, J. Am. Chem. Soc., 1967, 89, 7130–7131 CrossRef CAS.
-
(a) W. M. Bandaranayake, J. E. Banfield and D. S. C. Black, J. Chem. Soc., Chem. Commun., 1980, 902–903 RSC;
(b) W. M. Bandaranayake, J. E. Banfield, D. S. C. Black, G. D. Fallon and B. M. Gatehouse, J. Chem. Soc., Chem. Commun., 1980, 4, 162–163 RSC;
(c) W. M. Bandaranayake, J. E. Banfield, D. S. C. Black, G. D. Fallon and B. M. Gatehouse, Aust. J. Chem., 1981, 34, 1655–1667 CrossRef CAS;
(d) W. M. Bandaranayake, J. E. Banfield and D. S. C. Black, Aust. J. Chem., 1982, 35, 557–565 CrossRef CAS;
(e) J. E. Banfield, D. S. C. Black, S. R. Johns and R. I. Willing, Aust. J. Chem., 1982, 35, 2247–2256 CrossRef CAS.
-
(a) K. C. Nicolaou, N. A. Petasis, R. E. Zipkin and J. Uenishi, J. Am. Chem. Soc., 1982, 104, 5555–5557 CrossRef CAS;
(b) K. C. Nicolaou, N. A. Petasis, J. Uenishi and R. E. Zipkin, J. Am. Chem. Soc., 1982, 104, 5557–5558 CrossRef CAS;
(c) K. C. Nicolaou, R. E. Zipkin and N. A. Petasis, J. Am. Chem. Soc., 1982, 104, 5558–5560 CrossRef CAS;
(d) K. C. Nicolaou, N. A. Petasis and R. E. Zipkin, J. Am. Chem. Soc., 1982, 104, 5560–5562 CrossRef CAS.
-
(a) B. N. Lenta, J. R. Chouna, P. A. Nkeng-Efouet and N. Sewald, Biomolecules, 2015, 5, 910–942 CrossRef CAS PubMed;
(b) A. Leverrier, M. E. T. H. Dau, P. Retailleau, K. Awang, F. Guéritte and M. Litaudon, Org. Lett., 2010, 12, 3638–3641 CrossRef CAS PubMed;
(c) M. N. Azmi, T. Péresse, C. Remeur, G. Chan, F. Roussi, M. Litaudon and K. Awang, Fitoterapia, 2016, 109, 190–195 CrossRef CAS PubMed.
-
(a) K. Kurosawa, K. Takahashi and E. Tsuda, J. Antibiot., 2001, 54, 541–547 CrossRef CAS PubMed;
(b) K. Takahashi, E. Tsuda and K. Kurosawa, J. Antibiot., 2001, 54, 548–553 CrossRef CAS PubMed.
-
(a) E. Manzo, M. L. Ciavatta, M. Gavagnin, E. Mollo, S. Wahidulla and G. Cimino, Tetrahedron Lett., 2005, 46, 465–468 CrossRef CAS;
(b) M. Cueto, L. D'Croz, J. L. Maté, A. San-Martín and J. Darias, Org. Lett., 2005, 7, 415–418 CrossRef CAS PubMed;
(c) H. Wei, T. Itoh, M. Kinoshita, N. Kotoku, S. Aoki and M. Kobayashi, Tetrahedron, 2005, 61, 8054–8058 CrossRef CAS;
(d) M. Kobayashi, H. Wei, T. Itoh and N. Kotoku, Heterocycles, 2006, 68, 111 CrossRef.
-
(a) K. A. Parker and Y.-H. Lim, J. Am. Chem. Soc., 2004, 126, 15968–15969 CrossRef CAS PubMed;
(b) H. N. Lim and K. A. Parker, Org. Lett., 2013, 15, 398–401 CrossRef CAS PubMed;
(c) For a recent review, see: K. A. Parker and H. N. Lim, Chapter 3 - The Mysterious Case of the Kingianins, in Strategies and Tactics in Organic Synthesis, ed. M. Harmata, Academic Press, 2014, vol. 10, pp. 51–78 Search PubMed.
-
(a) C. M. Beaudry and D. Trauner, Org. Lett., 2002, 4, 2221–2224 CrossRef CAS PubMed;
(b) C. M. Beaudry and D. Trauner, Org. Lett., 2005, 7, 4475–4477 CrossRef CAS PubMed;
(c) J. E. Barbarow, A. K. Miller and D. Trauner, Org. Lett., 2005, 7, 2901–2903 CrossRef CAS PubMed;
(d) V. Sofiyev, G. Navarro and D. Trauner, Org. Lett., 2008, 10, 149–152 CrossRef CAS PubMed;
(e) A. K. Miller and D. Trauner, Angew. Chem., 2005, 117, 4678–4682 (
Angew. Chem., Int. Ed.
, 2005
, 44
, 4602–4606
) CrossRef.
-
(a) J. E. Moses, J. E. Baldwin, R. Marquez and R. M. Adlington, Org. Lett., 2002, 4, 3731–3734 CrossRef CAS PubMed;
(b) M. F. Jacobsen, J. E. Moses, R. M. Adlington and J. E. Baldwin, Org. Lett., 2005, 7, 2473–2476 CrossRef CAS PubMed;
(c) R. Rodriguez, R. M. Adlington, S. J. Eade, M. W. Walter, J. E. Baldwin and J. E. Moses, Tetrahedron, 2007, 63, 4500–4509 CrossRef CAS;
(d) S. J. Eade, M. W. Walter, C. Byrne, B. Odell, R. Rodriguez, J. E. Baldwin, R. M. Adlington and J. E. Moses, J. Org. Chem., 2008, 73, 4830–4839 CrossRef CAS PubMed;
(e) J. C. Moore, E. S. Davies, D. A. Walsh, P. Sharma and J. E. Moses, Chem. Commun., 2014, 50, 12523–12525 RSC.
-
(a) S. L. Drew, A. L. Lawrence and M. S. Sherburn, Angew. Chem., Int. Ed., 2013, 52, 4221–4224 CrossRef CAS PubMed;
(b) S. L. Drew, A. L. Lawrence and M. S. Sherburn, Chem. Sci., 2015, 6, 3886–3890 RSC.
- For a recent example, see: C. G. Newton, S. L. Drew, A. L. Lawrence, A. C. Willis, M. N. Paddon-Row and M. S. Sherburn, Nat. Chem., 2015, 7, 82–86 CrossRef CAS PubMed.
- M. J. Williams, H. L. Deak and M. L. Snapper, J. Am. Chem. Soc., 2007, 129, 486–487 CrossRef CAS PubMed.
- T. W. Bingham, L. W. Hernandez, D. G. Olson, R. L. Svec, P. J. Hergenrother and D. Sarlah, J. Am. Chem. Soc., 2019, 141, 657–670 CrossRef CAS PubMed.
- V. Mascitti and E. J Corey, J. Am. Chem. Soc., 2004, 126, 15664–15665 CrossRef CAS PubMed.
-
K. C. Nicolaou and N. A. Petasis, Chapter 6 - Pericyclic Reactions in Organic Synthesis and Biosynthesis: Synthetic Adventures with Endiandric Acids A–G, in Strategies and Tactics in Organic Synthesis, ed. T. Lindberg, , Academic Press, 1984, vol. 1, pp. 155–173 Search PubMed.
-
(a)
G. I. Fray and R. G. Saxton, The Chemistry of Cyclo-octatetraene and its Derivatives, Cambridge University Press, New York, 1978 Search PubMed;
(b) L. A. Paquette, Tetrahedron, 1975, 31, 2855–2883 CrossRef CAS.
-
(a) O. Yahiaoui, L. F. Pašteka, B. Judeel and T. Fallon, Angew. Chem., Int. Ed., 2018, 57, 2570–2574 CrossRef CAS PubMed;
(b) O. Yahiaoui, L. P. Pašteka, C. J. Blake, C. G. Newton and T. Fallon, Org. Lett., 2019, 21, 9574–9578 CrossRef CAS PubMed.
-
(a) F. Del Moro, P. Crotti, V. Di Bussolo, F. Macchia and M. Pineschi, Org. Lett., 2003, 5, 1971–1974 CrossRef CAS PubMed;
(b) M. Pineschi, F. D. Moro, P. Crotti and F. Macchia, Eur. J. Org. Chem., 2004, 4614–4620 CrossRef CAS.
-
(a) P.-S. Yang, M.-J. Cheng, C.-F. Peng, J.-J. Chen and I.-S. Chen, J. Nat. Prod., 2009, 72, 53–58 CrossRef CAS PubMed;
(b) P.-S. Yang, M.-J. Cheng, J.-J. Chen and I.-S. Chen, Helv. Chim. Acta, 2008, 91, 2130–2138 CrossRef CAS.
- D. J. Winternheimer, R. E. Shade and C. A. Merlic, Synthesis, 2010, 2497–2511 CAS.
- W. H. Watanabe and L. E. Conlon, J. Am. Chem. Soc., 1957, 79, 2828–2833 CrossRef CAS.
- M. Bosch and M. Schlaf, J. Org. Chem., 2003, 68, 5225–5227 CrossRef CAS PubMed.
- Y. Okimoto, S. Sakaguchi and Y. Ishii, J. Am. Chem. Soc., 2002, 124, 1590–1591 CrossRef CAS PubMed.
-
(a) A. Nakamura and M. Tokunaga, Tetrahedron Lett., 2008, 49, 3729–3732 CrossRef CAS;
(b) The addition of a catalytic amount of acetic acid was found to significantly accelerate this reaction, see: S. S. Zalesskiy, V. N. Khrustalev, A. Y. Kostukovich and V. P. Ananikov, Organometallics, 2015, 34, 5214–5224 CrossRef CAS.
- For examples involving propargyl aryl ether substrates, see:
(a) R. Hesse, K. K. Gruner, O. Kataeva, A. W. Schmidt and H.-J. Knölker, Chem.-Eur J., 2013, 19, 14098–14111 CrossRef CAS PubMed;
(b) L. A. M. Murray, T. Fallon, C. J. Sumby and J. H. George, Org. Lett., 2019, 21, 8312–8315 CrossRef CAS PubMed.
- Intermediates 7a/b and 8a/b can be isolated and fully characterised. See the ESI† for full details.
- For recent reviews, see:
(a) M. G. Vinogradov, O. V. Turova and S. G. Zlotin, Org. Biomol. Chem., 2017, 15, 8245–8269 RSC;
(b) D. R. Wenz and J. R. de Alaniz, Eur. J. Org. Chem., 2015, 23–37 CrossRef CAS;
(c) T. Vaidya, R. Eisenberg and A. J. Frontier, ChemCatChem, 2011, 3, 1531–1548 CrossRef CAS.
- S. Thompson, A. G. Coyne, P. C. Knipe and M. D. Smith, Chem. Soc. Rev., 2011, 40, 4217–4231 RSC.
- Litaudon reported that two antipodes of kingianin A show striking differences in BCL-XL binding affinity assays, see: L. everrier, K. Awang, F. Guéritte and M. Litaudon, Phytochemistry, 2011, 72, 1443–1452 CrossRef PubMed.
-
(a) K. Kim, J. W. Lauher and K. A. Parker, Org. Lett., 2012, 14, 138–141 CrossRef CAS PubMed;
(b) K. A. Parker and Z. Wang, Org. Lett., 2006, 8, 3553–3556 CrossRef CAS PubMed.
-
(a) R. Huisgen, G. Boche, A. Dahmen and W. Hechtl, Tetrahedron Lett., 1968, 9, 5215–5219 CrossRef;
(b) A. C. Cope, A. C. Haven, F. L. Ramp and E. R. Trumbull, J. Am. Chem. Soc., 1952, 74, 4867–4871 CrossRef CAS.
- A. Patel and K. N. Houk, J. Org. Chem., 2014, 79, 11370–11377 CrossRef CAS PubMed.
-
(a) Y. Zhao, N. E. Schultz and D. G. Truhlar, J. Chem. Theory Comput., 2006, 2, 364–382 CrossRef PubMed;
(b) Y. Zhao and D. G. Truhlar, J. Phys. Chem. A, 2005, 109, 5656–5667 CrossRef CAS PubMed;
(c) Y. Zhao and D. G. Truhlar, J. Chem. Phys., 2006, 125, 194101 CrossRef PubMed;
(d) Y. Zhao and D. G. Truhlar, Theor. Chem. Acc., 2008, 120, 215–241 Search PubMed.
Footnote |
† Electronic supplementary information (ESI) available. See DOI: 10.1039/d0sc03073b |
|
This journal is © The Royal Society of Chemistry 2020 |
Click here to see how this site uses Cookies. View our privacy policy here.