DOI:
10.1039/D0SC02816A
(Edge Article)
Chem. Sci., 2020,
11, 6830-6835
Palladium-catalyzed dearomative 1,4-difunctionalization of naphthalenes†
Received
18th May 2020
, Accepted 10th June 2020
First published on 10th June 2020
Abstract
A highly diastereoselective dearomatization of naphthalenes via a Pd-catalyzed 1,4-difunctionalization reaction is described. In the presence of a commercially available palladium precursor and ligand, intramolecular dearomative Heck-type insertion provides π-allylpalladium intermediates which are readily captured by a series of nucleophiles in excellent yields (up to 99%). This reaction features mild conditions, broad substrate scope, and useful transformations of the products.
Introduction
The difunctionalization of alkenes is widely recognized as a powerful approach to generate significant molecular complexity from simple chemical feedstock.1 In particular, Pd-catalyzed difunctionalization of 1,3-dienes, which introduces two functional groups across the conjugated C
C double bonds, has witnessed significant progress in the past decade.2 Mechanistically, Pd(II) complexes, usually generated from the oxidative addition of Pd(0) precursors, have been employed to achieve these reactions through Heck insertion3 to form a π-allylpalladium species. The subsequent regioselective nucleophilic attacks afford 1,2- or 1,4-addition-like products (Scheme 1a).2d However, the scope of these transformations was in general limited to structurally simple dienes4 and furans.5
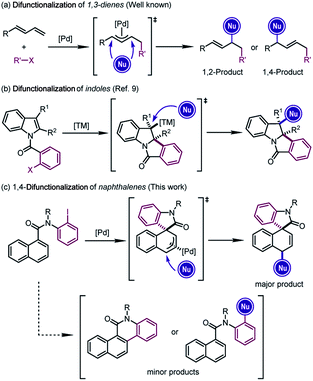 |
| Scheme 1 Difunctionalization of 1,3-dienes, indoles and naphthalenes. | |
In line with our continuous interest in catalytic dearomatization reactions,6 we envisioned that the formal “conjugated diene” structure of the phenyl ring might serve as the equivalent of 1,3-dienes. However, the dearomatization of electronically unbiased aromatic compounds such as naphthalenes and benzenes remained challenging,7 due to the generally higher aromatic stabilization energies of plain arenes compared with their heteroaromatic counterparts (36 kcal mol−1 for benzene and 22 kcal mol−1 for pyrrole).6r Therefore, the translation of Pd-catalyzed difunctionalization of 1,3-dienes to aromatic systems would open a new window for the dearomatization of non-activated arenes.
Inspired by the recent developments in Pd-catalyzed dearomative Heck reactions8 that are terminated by the nucleophilic attack on alkylpalladium intermediates (Scheme 1b),9 we realized that the π-allylpalladium intermediates formed from the Heck-type insertion into the naphthalene ring might also be captured using external nucleophiles, furnishing the dearomative 1,4-difunctionalization of naphthalenes (Scheme 1c).10 The successful execution of this reaction design relied on the judicious selection of the catalytic system which could overcome the thermodynamic disadvantage of the dearomatization process and at the same time avoid the competitive C–H activation or direct cross-coupling reactions. Herein we report our results from this study.
Results and discussion
We began our investigation by studying the 1,4-difunctionalization of N-(2-iodophenyl)-N-methyl-1-naphthamide (1a) with dimethyl malonate (2a) (Table 1). Firstly, we tested different ligands in the presence of PdCl2 (10 mol%), NaH (2.0 equiv.), and Ag3PO4 (1.0 equiv.) in DMA at 100 °C. When BINAP (L1), BINAP(O) (L2), and Feringa phosphoramidite (rac-L3) were used as the ligand respectively, the dearomatized product 3a was obtained as a single diastereomeric isomer in good yields (67–90%) (entries 1–3), whose structure and relative configuration were confirmed by X-ray crystallographic analysis. The relative configuration of 3a revealed that the in situ formed π-allylpalladium intermediate was attacked by the nucleophile via an outer sphere mechanism. On the other hand, the reaction with the PHOX ligand (L4) was sluggish (entry 4). Subsequently, different solvents were examined by using rac-L3 as the ligand (entries 5–8). DMA was found to be the optimal one among those tested. In particular, the desired reaction was prohibited significantly when tBuOH was employed (entry 6). Next, the effects of various bases were examined (entries 9–12). This revealed that 3a could be obtained in moderate yields by using K2CO3 or K3PO4, while the target product was not observed when 1,2,2,6,6-pentamethylpiperidine (PMP) or tBuOK was employed. Surprisingly, the judicious choice of silver salts was quite critical to this reaction. Among those tested, Ag3PO4 provided the optimal reaction outcomes. Other commonly used silver salts including AgOTf, AgNTf2, and AgBF4 were ineffective (entries 13–15). Finally, the optimal yield of 3a (95%) was obtained by using a pre-prepared sodium salt of 2a with lower catalyst loading (5 mol%) (entry 16).
Table 1 Optimization of the reaction conditionsa
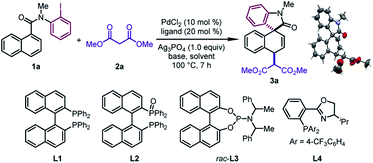
|
Entry |
Ligand |
Base |
Solvent |
1a
(%) |
3a
(%) |
Reaction conditions: 1a (0.2 mmol), 2a (0.4 mmol), PdCl2 (0.02 mmol), ligand (0.04 mmol), base (0.4 mmol), and Ag3PO4 (0.2 mmol) in solvent (1.0 mL) at 100 °C.
Yield determined by 1H NMR using CH2Br2 (0.2 mmol) as an internal standard.
Ligand (0.02 mmol).
rac-L3: (Ra,R,R + Sa,S,S) : (Sa,R,R + Ra,S,S) = 1 : 4.
Isolated yield.
PMP: 1,2,2,6,6-pentamethylpiperidine.
AgOTf as the silver salt.
AgNTf2 as the silver salt.
AgBF4 as the silver salt.
Sodium salt of 2a (pre-prepared by mixing NaH (0.4 mmol) and 2a (0.4 mmol) in DMA at room temperature for 0.5 h), PdCl2 (0.01 mmol), and rac-L3 (0.02 mmol).
|
1c |
L1
|
NaH |
DMA |
— |
79 |
2c |
L2
|
NaH |
DMA |
15 |
67 |
3 |
rac-L3d |
NaH |
DMA |
— |
90 (90e) |
4c |
L4
|
NaH |
DMA |
44 |
43 |
5 |
rac-L3d |
NaH |
Toluene |
69 |
22 |
6 |
rac-L3d |
NaH |
t
BuOH |
Quant. |
Trace |
7 |
rac-L3d |
NaH |
DCE |
63 |
36 |
8 |
rac-L3d |
NaH |
Dioxane |
52 |
51 |
9 |
rac-L3d |
K2CO3 |
DMA |
43 |
46 |
10 |
rac-L3d |
K3PO4 |
DMA |
51 |
29 |
11 |
rac-L3d |
PMPf |
DMA |
Quant. |
Trace |
12 |
rac-L3d |
t
BuOK |
DMA |
66 |
Trace |
13g |
rac-L3d |
NaH |
DMA |
66 |
27 |
14h |
rac-L3d |
NaH |
DMA |
84 |
8 |
15i |
rac-L3d |
NaH |
DMA |
77 |
5 |
16j |
rac-L3d |
NaH |
DMA |
— |
97 (95e) |
With the optimal conditions in hand, we surveyed the generality of this novel 1,4-difunctionalization by allowing various naphthalene derivatives 1 to react with sodium salts of dialkyl malonates 2 (Table 2). When the methyl group on the nitrogen tether of 1 was changed to isopropyl or benzyl groups, the desired products 3b and 3c were obtained in good yields (82–86%). The substrates bearing an electron-donating group (Me and OMe) or halide (F, Cl, and Br) at the para position of the phenyl ring led to 3d–3h in 75–98% yields. The good tolerance with halides would offer a handle on subsequent transformations. In contrast, when an electron-withdrawing group (CF3, CO2Me, and CN) was incorporated into the aryl iodide moiety, the corresponding products (3i, 3j, 3l) were formed in moderate yields (40–51%). Notably, an ortho substituent on the phenyl ring was well tolerated, affording the dearomatized product 3m in 80% yield. Naphthalene derivatives bearing a substituent at the C6 position furnished the products 3n–3p in reasonable yields (39–82%). It is worth noting that 3a could be obtained in 75% yield by using N-(2-bromophenyl)-N-methyl-1-naphthamide as the substrate and BINAP as the ligand. In addition, when diethyl malonate or dibenzyl malonate was utilized as the nucleophile, the desired products 3q and 3r were obtained with good yields (81–87%). The dearomatized product 3s bearing two all-carbon quaternary stereocenters could be afforded in 28% yield. The low yield probably resulted from the unfavorable steric hindrance in the second step.
Table 2 Substrate scope of naphthalenes and malonic diestersa
1 (0.2 mmol), 2 (0.4 mmol, pre-prepared from malonic ester with NaH), PdCl2 (0.01 mmol), rac-L3 (0.02 mmol), and Ag3PO4 (0.2 mmol) in DMA (1.0 mL) at 100 °C.
Pre-synthesized PdCl2–BINAP complex (0.01 mmol) was used.
N-(2-Bromophenyl)-N-methyl-1-naphthamide was used at 120 °C.
PdCl2 (0.02 mmol) and rac-L3 (0.04 mmol) at 120 °C.
|
|
Next, different 1,3-diketones were explored under slightly modified conditions (Table 3). When acetylacetone and 3,5-heptanedione were employed as the coupling partners, the corresponding products 4a and 4b were delivered in good yields (67–69%). Notably, cyclic β-diketones were also tolerated, leading to 4c and 4d in moderate yields (42–55%).
Table 3 Substrate scope of 1,3-diketonesa
1a (0.2 mmol), 2 (0.4 mmol), pre-synthesized PdCl2–BINAP complex (0.02 mmol), Ag3PO4 (0.2 mmol), and Na2HPO4·12H2O (0.4 mmol) in DMA (1.0 mL) at 120 °C.
|
|
The reaction design was successfully applied to the sodium salts of β-ketoesters (Table 4). The reactions of diverse alkyl substituted β-ketoesters furnished the desired products in good to excellent yields (5a–5f, 56–99%). Aryl β-ketoesters also participated smoothly in the reaction, regardless of the electronic properties of the aryl group, delivering 5g–5i in good yields (59–76%). Of particular note is that 2-thienyl and 2-furyl β-ketoesters could be converted to 5j and 5k in moderate to good yields (45–89%).
Table 4 Substrate scope of β-ketoestersa,b
1a (0.2 mmol), 2 (0.4 mmol, pre-prepared from β-ketoester with NaH), PdCl2 (0.01 mmol), rac-L3 (0.02 mmol), and Ag3PO4 (0.2 mmol) in DMA (1.0 mL) at 100 °C.
The diastereomeric selectivity originates from the reversal of the relative configuration at the position denoted with an asterisk.
PdCl2 (0.02 mmol), rac-L3 (0.04 mmol).
|
|
In addition to β-ketoesters, the esters bearing an electron-withdrawing group (NO2 and SO2Ph) at the α-position were proved to be viable participants, leading to 5l and 5m in moderate yields (24–51%) (Scheme 2). Although obtained as a mixture of a pair of diastereoisomers with poor dr values, the products could undergo decarboxylation to deliver pure dearomatized compounds (vide infra).
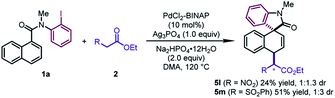 |
| Scheme 2 Substrate scope of esters. | |
To further demonstrate the generality of this reaction, we focused on developing the 1,4-difunctionalization with nitrogen-based nucleophiles (Table 5). Various benzyl amines were investigated as the nucleophiles. The corresponding products 6a and 6b could be obtained in excellent yields (97–99%). Moreover, furfurylamine and 2-thiophenemethylamine were also compatible, leading to dearomatized products 6c and 6d in good yields (76–98%). Cyclic secondary amines, such as pyrrolidine, piperidine and morpholine, reacted as the coupling partners to form the tertiary amines 6f–6h in excellent yields (98–99%). Diethylamine and cyclohexylamine also participated in the reaction smoothly, leading to 6e and 6i in excellent yields (88–95%). It is noteworthy that various anilines regardless of electronic properties were also viable reaction partners. The desired products 6k–6m could be delivered in high yields (80–99%). The structure of 6k was determined by X-ray crystallographic analysis. Notably, the reactions of tryptamine and allylamine led to 6j and 6n in low yields. When (R)-1-phenylethylamine was used, 6o was obtained in 96% yield with a 1
:
1 dr.
Table 5 Substrate scope of aminesa
1a (0.2 mmol), 2 (0.4 mmol), pre-synthesized PdCl2–BINAP complex (0.01 mmol), Ag3PO4 (0.2 mmol), and Na2HPO4·12H2O (0.4 mmol) in DMA (1.0 mL) at 120 °C.
|
|
Preliminary investigations on the enantioselective variants of the dearomative 1,4-difunctionalization reactions of naphthalene derivatives with dimethyl malonate or aniline were performed. Unfortunately, the utilization of a series of chiral ligands did not afford satisfactory asymmetric induction (see the ESI† for details).
To test the practicality of this method, a gram-scale dearomative 1,4-difunctionalization reaction of 1a (4.0 mmol) and aniline with a lower catalyst loading (2.5 mol%) was carried out (Scheme 3). The desired product 6k could be afforded in 96% yield (1.3 g). Some synthetic transformations of the dearomatized products have been examined. The mixture of two diastereoisomers of 5g (1
:
1 dr) could be decarboxylated to form 7 in 80% yield. The newly formed C–N bond of 6k could be cleaved by the hydrogenolysis reaction with Pd/C, leading to 8 in excellent yield. In addition, the β-diketone compound 4a could be condensed with hydroxylamine hydrochloride and hydrazine monohydrate, furnishing the corresponding isoxazole 9 and pyrazole 10 in good yields, respectively.
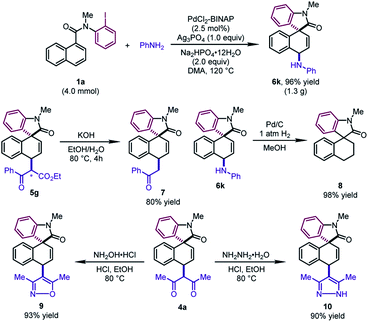 |
| Scheme 3 Gram-scale reaction and transformations of the products. | |
Conclusions
In summary, we have developed a Pd-catalyzed dearomative 1,4-difunctionalization of naphthalene derivatives by mimicking the reactivity of simple conjugated dienes in more challenging electronically unbiased aromatic systems. Diverse nucleophiles were found to be compatible with the reaction conditions. Various functionalized spirooxindoles could be obtained efficiently in good to excellent yields (up to 99%) with exclusive diastereoselectivity. Further exploration of the application of this methodology is currently underway in our laboratory.
Conflicts of interest
There are no conflicts to declare.
Acknowledgements
We thank the MOST (2016YFA0202900), NSFC (21821002 and 91856201) and Chinese Academy of Sciences (XDB20000000 and QYZDY-SSW-SLH012) for generous financial support. S.-L. Y. acknowledges the support from the Tencent Foundation through the XPLORER PRIZE.
Notes and references
- For selected reviews:
(a) K. H. Jensen and M. S. Sigman, Org. Biomol. Chem., 2008, 6, 4083–4088 RSC;
(b) R. I. McDonald, G. Liu and S. S. Stahl, Chem. Rev., 2011, 111, 2981–3019 CrossRef CAS PubMed;
(c) D. M. Schultz and J. P. Wolfe, Synthesis, 2012, 44, 351–361 CrossRef CAS PubMed;
(d) M. S. Sigman and E. W. Werner, Acc. Chem. Res., 2012, 45, 874–884 CrossRef CAS PubMed;
(e) G. Yin, X. Mu and G. Liu, Acc. Chem. Res., 2016, 49, 2413–2423 CrossRef CAS PubMed.
- For selected reviews:
(a) J. W. Han and T. Hayashi, Tetrahedron: Asymmetry, 2010, 21, 2193–2197 CrossRef CAS;
(b) Z. Wu and W. Zhang, Chin. J. Org. Chem., 2017, 37, 2250–2262 CrossRef CAS;
(c) Y. Xiong, Y. Sun and G. Zhang, Tetrahedron Lett., 2018, 59, 347–355 CrossRef CAS;
(d) X. Wu and L.-Z. Gong, Synthesis, 2019, 51, 122–134 CrossRef CAS;
(e) G. Li, X. Huo, X. Jiang and W. Zhang, Chem. Soc. Rev., 2020, 49, 2060–2118 RSC.
- For selected reviews:
(a) R. F. Heck, Acc. Chem. Res., 1979, 12, 146–151 CrossRef CAS;
(b) I. P. Beletskaya and A. V. Cheprakov, Chem. Rev., 2000, 100, 3009–3066 CrossRef CAS PubMed;
(c) A. B. Dounay and L. E. Overman, Chem. Rev., 2003, 103, 2945–2964 CrossRef CAS PubMed;
(d) M. Shibasaki, E. M. Vogl and T. Ohshima, Adv. Synth. Catal., 2004, 346, 1533–1552 CrossRef CAS;
(e) D. Mc Cartney and P. J. Guiry, Chem. Soc. Rev., 2011, 40, 5122–5150 RSC;
(f) H. Li, C. Ding, B. Xu and X. Hou, Acta Chim. Sin., 2014, 72, 765–770 CrossRef CAS;
(g) A. Biffis, P. Centomo, A. Del Zotto and M. Zecca, Chem. Rev., 2018, 118, 2249–2295 CrossRef CAS PubMed;
(h) Y. Ping, Y. Li, J. Zhu and W. Kong, Angew. Chem., Int. Ed., 2019, 58, 1562–1573 CrossRef CAS PubMed.
- For selected examples:
(a) K. Kagechika and M. Shibasaki, J. Org. Chem., 1991, 56, 4093–4094 CrossRef CAS;
(b) K. Kagechika, T. Ohshima and M. Shibasaki, Tetrahedron, 1993, 49, 1773–1782 CrossRef CAS;
(c) C. S. Nylund, J. M. Klopp and S. M. Weinreb, Tetrahedron Lett., 1994, 35, 4287–4290 CrossRef;
(d) T. Ohshima, K. Kagechika, M. Adachi, M. Sodeoka and M. Shibasaki, J. Am. Chem. Soc., 1996, 118, 7108–7116 CrossRef CAS;
(e) D. Flubacher and G. Helmchen, Tetrahedron Lett., 1999, 40, 3867–3868 CrossRef CAS;
(f) X. Wu, H.-C. Lin, M.-L. Li, L.-L. Li, Z.-Y. Han and L.-Z. Gong, J. Am. Chem. Soc., 2015, 137, 13476–13479 CrossRef CAS PubMed;
(g) Y. Liu, Y. Xie, H. Wang and H. Huang, J. Am. Chem. Soc., 2016, 138, 4314–4317 CrossRef CAS PubMed;
(h) Z.-L. Tao, A. Adili, H.-C. Shen, Z.-Y. Han and L.-Z. Gong, Angew. Chem., Int. Ed., 2016, 55, 4322–4326 CrossRef CAS PubMed;
(i) L. Luo, H. Zheng, J. Liu, H. Wang, Y. Wang and X. Luan, Org. Lett., 2016, 18, 2082–2085 CrossRef CAS PubMed;
(j) L. Xu, X. Zhang, M. S. McCammant, M. S. Sigman, Y.-D. Wu and O. Wiest, J. Org. Chem., 2016, 81, 7604–7611 CrossRef CAS PubMed;
(k) N. J. Adamson, E. Hull and S. J. Malcolmson, J. Am. Chem. Soc., 2017, 139, 7180–7183 CrossRef CAS PubMed;
(l) H.-C. Shen, P.-S. Wang, Z.-L. Tao, Z.-Y. Han and L.-Z. Gong, Adv. Synth. Catal., 2017, 359, 2383–2389 CrossRef CAS;
(m) N. J. Adamson, K. C. E. Wilbur and S. J. Malcolmson, J. Am. Chem. Soc., 2018, 140, 2761–2764 CrossRef CAS PubMed;
(n) S. Park and S. J. Malcolmson, ACS Catal., 2018, 8, 8468–8476 CrossRef CAS;
(o) X. Wu, S.-S. Chen, L. Zhang, H.-J. Wang and L.-Z. Gong, Chem. Commun., 2018, 54, 9595–9598 RSC;
(p) T. Zhang, H.-C. Shen, J.-C. Xu, T. Fan, Z.-Y. Han and L.-Z. Gong, Org. Lett., 2019, 21, 2048–2051 CrossRef CAS PubMed;
(q) Y. Zhang, H.-C. Shen, Y.-Y. Li, Y.-S. Huang, Z.-Y. Han and X. Wu, Chem. Commun., 2019, 55, 3769–3772 RSC;
(r) D. Zhu, Z. Jiao, Y. R. Chi, T. P. Gonçalves, K.-W. Huang and J. S. Zhou, Angew. Chem., Int. Ed., 2020, 59, 5341–5345 CrossRef CAS PubMed.
-
(a) J. Li, H. Peng, F. Wang, X. Wang, H. Jiang and B. Yin, Org. Lett., 2016, 18, 3226–3229 CrossRef CAS PubMed;
(b) J. Liu, X. Xu, J. Li, B. Liu, H. Jiang and B. Yin, Chem. Commun., 2016, 52, 9550–9553 RSC;
(c) Z. Wang, W. Luo, L. Lu and B. Yin, J. Org. Chem., 2018, 83, 10080–10088 CrossRef CAS PubMed.
- For recent reviews of dearomative reactions:
(a) A. R. Pape, K. P. Kaliappan and E. P. Kündig, Chem. Rev., 2000, 100, 2917–2940 CrossRef CAS PubMed;
(b) S. Quideau, L. Pouységu and D. Deffieux, Synlett, 2008, 467–495 CrossRef CAS;
(c) L. Pouységu, D. Deffieux and S. Quideau, Tetrahedron, 2010, 66, 2235–2261 CrossRef;
(d) L. Pouységu, T. Sylla, T. Garnier, L. B. Rojas, J. Charris, D. Deffieux and S. Quideau, Tetrahedron, 2010, 66, 5908–5917 CrossRef;
(e) S. P. Roche and J. A. Porco Jr, Angew. Chem., Int. Ed., 2011, 50, 4068–4093 CrossRef CAS PubMed;
(f) D.-S. Wang, Q.-A. Chen, S.-M. Lu and Y.-G. Zhou, Chem. Rev., 2012, 112, 2557–2590 CrossRef CAS PubMed;
(g) C.-X. Zhuo, W. Zhang and S.-L. You, Angew. Chem., Int. Ed., 2012, 51, 12662–12686 CrossRef PubMed;
(h) C.-X. Zhuo, C. Zheng and S.-L. You, Acc. Chem. Res., 2014, 47, 2558–2573 CrossRef CAS PubMed;
(i) Q. Ding, X. Zhou and R. Fan, Org. Biomol. Chem., 2014, 12, 4807–4815 RSC;
(j) S. P. Roche, J.-J. Youte Tendoung and B. Tréguier, Tetrahedron, 2015, 71, 3549–3591 CrossRef CAS;
(k) W.-T. Wu, L. Zhang and S.-L. You, Chem. Soc. Rev., 2016, 45, 1570–1580 RSC;
(l) C. Zheng and S.-L. You, Chem, 2016, 1, 830–857 CrossRef CAS;
(m) M. J. James, P. O'Brien, R. J. K. Taylor and W. P. Unsworth, Chem.–Eur. J., 2016, 22, 2856–2881 CrossRef CAS PubMed;
(n) W. Sun, G. Li, L. Hong and R. Wang, Org. Biomol. Chem., 2016, 14, 2164–2176 RSC;
(o) J.-B. Chen and Y.-X. Jia, Org. Biomol. Chem., 2017, 15, 3550–3567 RSC;
(p) W.-T. Wu, L. Zhang and S.-L. You, Acta Chim. Sin., 2017, 75, 419–438 CrossRef CAS;
(q) J. Bariwal, L. G. Voskressensky and E. V. Van der Eycken, Chem. Soc. Rev., 2018, 47, 3831–3848 RSC;
(r) W. C. Wertjes, E. H. Southgate and D. Sarlah, Chem. Soc. Rev., 2018, 47, 7996–8017 RSC;
(s) J. An and M. Bandini, Chimia, 2018, 72, 610–613 CrossRef CAS PubMed;
(t) Y.-Z. Cheng, X. Zhang and S.-L. You, Sci. Bull., 2018, 63, 809–811 CrossRef CAS;
(u) G. Huang and B. Yin, Adv. Synth. Catal., 2019, 361, 405–425 CrossRef CAS; for a book:
(v)
S.-L. You, Asymmetric Dearomatization Reactions, Wiley-VCH, Weinheim, 2016 CrossRef.
- For selected recent examples:
(a) M. Bao, H. Nakamura and Y. Yamamoto, J. Am. Chem. Soc., 2001, 123, 759–760 CrossRef CAS PubMed;
(b) B. Peng, X. Feng, X. Zhang, L. Ji and M. Bao, Tetrahedron, 2010, 66, 6013–6018 CrossRef CAS;
(c) B. Peng, S. Zhang, X. Yu, X. Feng and M. Bao, Org. Lett., 2011, 13, 5402–5405 CrossRef CAS PubMed;
(d) B. M. Trost, V. Ehmke, B. M. O'Keefe and D. A. Bringley, J. Am. Chem. Soc., 2014, 136, 8213–8216 CrossRef CAS PubMed;
(e) E. H. Southgate, J. Pospech, J. Fu, D. R. Holycross and D. Sarlah, Nat. Chem., 2016, 8, 922–928 CrossRef CAS PubMed;
(f) M. Okumura, S. M. Nakamata Huynh, J. Pospech and D. Sarlah, Angew. Chem., Int. Ed., 2016, 55, 15910–15914 CrossRef CAS PubMed;
(g) S. Zhang, J. Cai, Y. Yamamoto and M. Bao, J. Org. Chem., 2017, 82, 5974–5980 CrossRef CAS PubMed;
(h) S. Zhang, A. Ullah, Y. Yamamoto and M. Bao, Adv. Synth. Catal., 2017, 359, 2723–2728 CrossRef CAS;
(i) M. Okumura, A. S. Shved and D. Sarlah, J. Am. Chem. Soc., 2017, 139, 17787–17790 CrossRef CAS PubMed;
(j) L. W. Hernandez, U. Klöckner, J. Pospech, L. Hauss and D. Sarlah, J. Am. Chem. Soc., 2018, 140, 4503–4507 CrossRef CAS PubMed;
(k) M. Komatsuda, K. Muto and J. Yamaguchi, Org. Lett., 2018, 20, 4354–4357 CrossRef CAS PubMed;
(l) Z.-P. Yang, R. Jiang, Q.-F. Wu, L. Huang, C. Zheng and S.-L. You, Angew. Chem., Int. Ed., 2018, 57, 16190–16193 CrossRef CAS PubMed;
(m) W. C. Wertjes, M. Okumura and D. Sarlah, J. Am. Chem. Soc., 2019, 141, 163–167 CrossRef CAS PubMed;
(n) M. Komatsuda, H. Kato, K. Muto and J. Yamaguchi, ACS Catal., 2019, 9, 8991–8995 CrossRef CAS;
(o) C. Tang, M. Okumura, H. Deng and D. Sarlah, Angew. Chem., Int. Ed., 2019, 58, 15762–15766 CrossRef CAS PubMed;
(p) R.-Q. Xu, P. Yang, C. Zheng and S.-L. You, Chin. J. Chem., 2020, 38, 683–689 CrossRef CAS.
- For a recent review:
(a) N. Zeidan and M. Lautens, Synthesis, 2019, 51, 4137–4146 CrossRef CAS; for selected recent examples:
(b) P. Yang and S.-L. You, Org. Lett., 2018, 20, 7684–7688 CrossRef CAS PubMed;
(c) P. Yang, R.-Q. Xu, C. Zheng and S.-L. You, Chin. J. Chem., 2020, 38, 235–241 CrossRef CAS.
-
(a) C. Shen, R.-R. Liu, R.-J. Fan, Y.-L. Li, T.-F. Xu, J.-R. Gao and Y.-X. Jia, J. Am. Chem. Soc., 2015, 137, 4936–4939 CrossRef CAS PubMed;
(b) D. A. Petrone, A. Yen, N. Zeidan and M. Lautens, Org. Lett., 2015, 17, 4838–4841 CrossRef CAS PubMed;
(c) S. Chen, X.-X. Wu, J. Wang, X.-H. Hao, Y. Xia, Y. Shen, H. Jing and Y.-M. Liang, Org. Lett., 2016, 18, 4016–4019 CrossRef CAS PubMed;
(d) D. A. Petrone, M. Kondo, N. Zeidan and M. Lautens, Chem.–Eur. J., 2016, 22, 5684–5691 CrossRef CAS PubMed;
(e) R.-R. Liu, T.-F. Xu, Y.-G. Wang, B. Xiang, J.-R. Gao and Y.-X. Jia, Chem. Commun., 2016, 52, 13664–13667 RSC;
(f) R.-R. Liu, Y. Xu, R.-X. Liang, B. Xiang, H.-J. Xie, J.-R. Gao and Y.-X. Jia, Org. Biomol. Chem., 2017, 15, 2711–2715 RSC;
(g) R.-R. Liu, Y.-G. Wang, Y.-L. Li, B.-B. Huang, R.-X. Liang and Y.-X. Jia, Angew. Chem., Int. Ed., 2017, 56, 7475–7478 CrossRef CAS PubMed;
(h) N. Zeidan, T. Beisel, R. Ross and M. Lautens, Org. Lett., 2018, 20, 7332–7335 CrossRef CAS PubMed;
(i) R.-X. Liang, R.-Z. Yang, R.-R. Liu and Y.-X. Jia, Org. Chem. Front., 2018, 5, 1840–1843 RSC;
(j) A. D. Marchese, F. Lind, Á. E. Mahon, H. Yoon and M. Lautens, Angew. Chem., Int. Ed., 2019, 58, 5095–5099 CrossRef CAS PubMed;
(k) C. Shen, N. Zeidan, Q. Wu, C. B. J. Breuers, R.-R. Liu, Y.-X. Jia and M. Lautens, Chem. Sci., 2019, 10, 3118–3122 RSC;
(l) R.-X. Liang, K. Wang, Q. Wu, W.-J. Sheng and Y.-X. Jia, Organometallics, 2019, 38, 3927–3930 CrossRef CAS.
-
(a) Z. Zuo, H. Wang, Y. Diao, Y. Ge, J. Liu and X. Luan, ACS Catal., 2018, 8, 11029–11034 CrossRef CAS;
(b) X. Liao, D. Wang, Y. Huang, Y. Yang and J. You, Org. Lett., 2019, 21, 1152–1155 CrossRef CAS PubMed.
Footnote |
† Electronic supplementary information (ESI) available. CCDC 1982544-1982545. For ESI and crystallographic data in CIF or other electronic format see DOI: 10.1039/d0sc02816a |
|
This journal is © The Royal Society of Chemistry 2020 |
Click here to see how this site uses Cookies. View our privacy policy here.