DOI:
10.1039/D0SC02439B
(Edge Article)
Chem. Sci., 2020,
11, 6070-6074
Silanol: a bifunctional group for peptide synthesis and late-stage functionalization†
Received
30th April 2020
, Accepted 24th May 2020
First published on 3rd June 2020
Abstract
Chemical modification of a specific amino acid residue on peptides represents an efficient strategy to improve their pharmacokinetics and facilitates the potential to achieve post-synthetic diversification of peptides. Herein, we reported the first Pd-catalyzed late-stage ortho-olefination of Tyr residues on peptides with high chemo- and site-selectivity, by employing the easily attached and removable silanol as a bifunctional protecting group and directing group. Up to hexapeptides with variation on amino acid sequences or locations of the Tyr residue and different olefins were compatible with this protocol, which enriched the chemical toolbox for late-stage modification via C(sp2)–H functionalization. Furthermore, the orthogonal protection strategies of Tyr were also developed and could be applied to SPPS.
Introduction
Emerged as a class of new therapeutic targets, the study of protein–protein interaction (PPI) is driving the rapid growth of synthetic peptides or peptidomimetics.1 However, the instability of peptides and analogues in vivo greatly depresses their bioactivity and pharmacokinetics.2 The incorporation of unnatural amino acids into peptides represents one of the most efficient strategies to improve their in vivo stability, which makes the development of new methods that could site-specifically modify the amino acid residues in peptides valuable.3 Successful efforts4 have been made to decorate alanine (Ala)5 and its homologues,6 tryptophan (Trp),7 phenylalanine (Phe)8 and histidine (His).9 We notice that the directed functionalization of the phenol motif has been achieved on small molecules,10 whereas very few examples related to tyrosine (Tyr)11 (Fig. 1a) have been reported. Particularly, site-selective modification of Tyr residues in polypeptide via C–C bond formation is hitherto undisclosed.
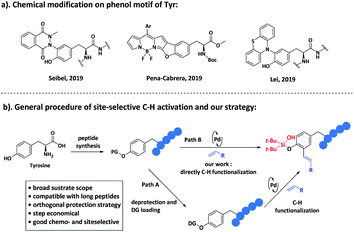 |
| Fig. 1 (a). Previous work about modification of tyrosine residues. (b). Traditional strategy for late-stage modification and our strategy. | |
The most commonly applied approach to address the site-selectivity in C–H functionalization relies on the installation of a directing group (DG) at a specific position,12 which is an extra step and might be problematic. Late-stage functionalization directed by the peptide backbone8b,c is an excellent strategy to avoid the installation of additional directing groups, but it is limited to few peptide sequences. We focus on step-economical chemistry and envisioned whether the protecting group (PG) for solid phase peptide synthesis (SPPS) on the phenol motif of Tyr could also act as the directing group (Fig. 1b), which could be directly employed for late-stage modification after peptide synthesis. A silicon tether seems ideal, as it can easily be attached or removed. Gevorgyan's group developed several silicon-tethered strategies13 for C–H functionalization at the ortho-position of the phenol motif14 for small molecules. However, site-selective functionalization4 of Tyr residues on peptides is predicted to be more challenging due to the multiple functional groups within the peptide sequence, and the existence of peptide backbones is expected to interfere with the interaction between the catalyst and DG. Herein, we reported the first Pd-catalyzed late-stage ortho-olefination of Tyr residues with high chemo- and site-selectivity on peptides, by employing bifunctional silanol on the phenol motif which served as a PG and DG. Silanol could also be applied in orthogonal protection strategies and solid phase peptide synthesis (SPPS).
Results and discussion
To test the feasibility of our hypothesis, we initiated our study by utilizing Tyr derived Boc-Y(Sil)-OMe (1a) as the model substrate and acrylate tert-butyl ester (2a) as the olefination reagent. Several C–H functionalization conditions (Table 1, entries 1–4)14a,15 that have been successfully applied to modify small molecules were tested. Perhaps unsurprisingly, we could not detect the formation of 3aa, probably due to the inefficient oxidation of Pd(0). Luckily, the desired product 3aa was obtained in 12% yield by using Ag2CO3 (Table 1, entry 5). Several other oxidants were screened (ESI, Table S1†); we were excited to find that PhI(OAc)2 could improve the reaction yield to 47% (Table 1, entry 6). Further investigation of the base showed that Li3PO4 was optimal and gave 3aa in 58% yield (Table 1, entry10). Continuous efforts on screening of additives (ESI, Table S4†) showed that addition of benzoquinone (BQ) exhibited the best reactivity and delivered 3aa in 76% yield after 24 h, which might have resulted from the addition of BQ that could suppress the formation of palladium black.16 Remarkably, a mono-olefination product was solely isolated from the reaction, no di-olefination product was detected. We envisioned that this might be because the two bulky tert-butyl groups on silicon blocked the rotation of silanol,14a thus preventing the second olefination. NMR analysis of 3aa confirmed that the olefination occurred at the ortho-position of the phenol motif, and the double bond was determined to be in E-configuration (ESI, Fig. S5 and S6†). The optimal reaction conditions for site-selective olefination are as follows: amino acid or peptide (1.0 eq.) and olefination reagent (4.0 eq.), with 10% mol of Pd(OAc)2, 3.0 eq. of PhI(OAc)2, 2.0 eq. of Li3PO4, and 0.2 eq. of BQ in DCE and agitation for 24 h at 90 °C.
Table 1 Optimization of the reaction conditionsa

|
Entry |
Base |
Oxidant |
Solvent |
Additive |
Yieldb [%] |
Reaction conditions: 1a (0.1 mmol, 1.0 eq.), 2a (0.4 mmol, 4.0 eq.), Pd(OAc)2 (0.01 mmol, 0.1 eq.), additive (0.02 mmol, 0.2 eq.), oxidant (0.3 mmol, 3.0 eq.), and base (0.2 mmol, 2.0 eq.) in solvent (1.0 mL) at 90 °C for 24 h.
1H NMR yield using 1,3,5-trimethoxybenzene as the internal standard.
L1 = (+)-menthyl(O2C)-Leu-OH.
t-AmOH = tertiary amyl alcohol.
L2 = Ac-Gly-OH.
Benzoquinone.
Isolated yield.
|
1 |
Li2CO3 |
AgOAc |
DCE |
L1c |
n.d |
2 |
KHCO3 |
O2 |
t-AmOHd |
— |
n.d |
3 |
Li2CO3 |
AgOAc |
DCE |
— |
n.d |
4 |
— |
Ag2CO3 |
HFIP |
L2e |
n.d |
5 |
Li2CO3 |
Ag2CO3 |
DCE |
— |
12 |
6 |
Li2CO3 |
PhI(OAc)2 |
DCE |
— |
47 |
7 |
NaHCO3 |
PhI(OAc)2 |
DCE |
— |
22 |
8 |
LiOAc |
PhI(OAc)2 |
DCE |
— |
<10 |
9 |
LiH2PO4 |
PhI(OAc)2 |
DCE |
— |
38 |
10 |
Li3PO4 |
PhI(OAc)2 |
DCE |
— |
58 |
11 |
Li3PO4 |
PhI(OAc)2 |
DCE |
L1 |
51 |
12 |
Li3PO4 |
PhI(OAc)2 |
DCE |
BQf |
76(73g) |
With the optimized reaction conditions in hand, we started to investigate the scope of olefin coupling partners 2 (ESI, Table S5†). To our delight, a wide range of alkenes could be successfully incorporated at the ortho-position of the phenol motif in Tyr residues with moderate to good yield (Scheme 1a), affording the acrylic ester products 3aa, 3ab and 3ac in 66–76% yields. Interestingly, vinyl phosphonate product 3ad, vinylethylene carbonate product 3ae, vinyl sulfonated product 3af and vinyl sulfonamide product 3ag could also be prepared by this strategy with 55–80% yields, indicating the broad substrate scope of this strategy. To our delight, alkenes 2h and 2i, derived from proline (Pro) and Thr, were also efficient coupling partners to give 3ah and 3ai in 69% and 61% yields, respectively, suggesting the potential of using this strategy to perform macrocyclization of peptides. The silanol group could be efficiently removed from 3aa by simply treating with TBAF to get 3b in 91% yield (ESI, Fig. S1†), suggesting the compatibility of the silanol group with other protecting groups. To further explore the utility of the bifunctional silanol group, we synthesized amino acid building blocks 1b–1e with the purpose of developing orthogonal protection strategies (ESI, Fig. S2†) for peptide synthesis and the subsequent functionalization. The attachment and removal of traditional protecting groups including N-Boc, N-Fmoc, N-Alloc and methyl esters were all compatible with the silanol group (Scheme 1b).
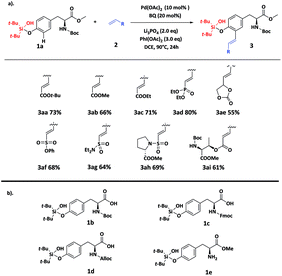 |
| Scheme 1 Scope of coupling partnersa and orthogonal protection strategies.a Reaction conditions: 1a (0.2 mmol, 1.0 eq.), 2 (0.8 mmol, 4.0 eq.), Pd(OAc)2 (0.02 mmol, 0.1eq.), BQ (0.04 mmol, 0.2 eq.), PhI(OAc)2 (0.6 mmol, 3.0 eq.), and Li3PO4 (0.4 mmol, 2.0 eq.) in DCE (2.0 mL) at 90 °C for 24 h. | |
Next, we turned our attention to explore the compatibility of this method. Pleasingly, most of the dipeptides 4b–4o (ESI, Fig. S3†) gave the desired mono-ortho-olefination products with good results. As shown, dipeptides with alkyl side chain amino acids at the C-terminus could be well tolerated to give the products 5ba–5ha in 42–66% yields (Scheme 2a). In general, peptides with side chain functional groups were difficult to modify. Gratefully, our catalytic system seemed to be quite compatible; dipeptides 4i–4k bearing aspartic acid (Asp), threonine (Thr) and lysine (Lys) residues at the C-terminus could also be decorated efficiently, affording the corresponding products 5ia, 5ja and 5kc in 48–56% yields. Interestingly, dipeptide 4l with Phe residues at the C-terminus gave the desired product 5la in 36% yield, with no functionalization of Phe residues detected. The promising results indicated that this strategy could achieve unique chemo-selective Tyr modification and discriminate the subtle differences between Phe and Tyr residues, suggesting that the directing ability of silanol is superior to that of the peptide backbone in this catalytic system. In addition, olefination of dipeptides 4m–4o bearing Tyr residues on the C-terminus could also be achieved, and delivered the modified products 5ma, 5na and 5oa in 48–57% yields (Scheme 2b). Compared to the Tyr monomer, the incomplete conversion of dipeptides made the yields slightly decreased. We considered that this might be caused by the chelation between the amides on the peptide backbone8b and the catalyst.
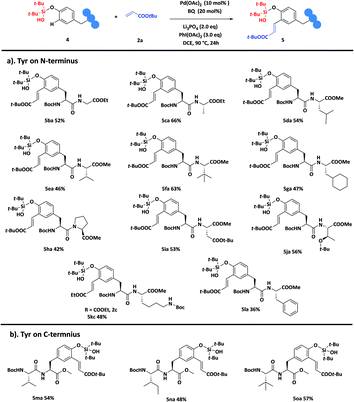 |
| Scheme 2
a
Scope of dipeptide substrates.aReaction conditions: 4 (0.2 mmol, 1.0 eq.), 2a (0.8 mmol, 4.0 eq.), Pd(OAc)2 (0.02 mmol, 0.1 eq.), BQ (0.04 mmol, 0.2 eq.), PhI(OAc)2 (0.6 mmol, 3.0 eq.), and Li3PO4 (0.4 mmol, 2.0 eq.) in DCE (2.0 mL) at 90 °C for 24 h. | |
To further expand the application of this strategy, polypeptides 6a–6n were synthesized by using 1b or 1c as building blocks according to the standard SPPS. The bifunctional silanol group seemed quite compatible and delivered polypeptide precursors (ESI, Fig. S4†) in good yields compared with the commercially available amino acid building blocks. As shown in scheme 3a, tripeptides (6a–6h) bearing Tyr residues on the N-terminus could be well decorated with 2a, afforded the corresponding products 7aa–7fa in 41–50% yields. Moreover, tripeptides 6g and 6h bearing Tyr residues on the C-terminus could also be well functionalized to give the products 7ga and 7ha in 45% and 43% yields respectively. We were excited to find that the olefination of longer peptides 6i–6n was also successful by employing this protocol (Scheme 3b), affording the modified tetrapeptides 7ia–7ka in 28–33% yields and the modified pentapeptides 7la and 7ma in 23% and 22% yields respectively. In addition, the modification of long peptides might be difficult and thus was rarely reported.4 We noticed that the solution of polypeptide substrates became dark quickly while adding Pd(OAc)2, which indicated the strong chelation of the peptide backbone and resulted in reduced conversion. Surprisingly, the olefinated hexapeptide 7na was eventually obtained in 21% yield by employing the strategy. We further explored the utilities of our newly developed methods by decorating tetrapeptide 6k with different alkenes, and delivered the corresponding acrylic ester product 7kb in 31% yield, vinyl phosphonate product 7kd in 26% yield, and vinyl sulfonamide product 7kg in 23% yield.
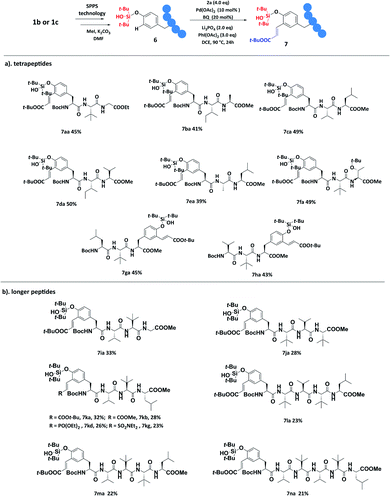 |
| Scheme 3 Applicationa and late-stage peptide modification.b aFor peptide synthesis, see the ESI.†b Reaction conditions: 6 (0.2 mmol, 1.0 eq.), 2a (0.8 mmol, 4.0 eq.), Pd(OAc)2 (0.02 mmol, 0.1 eq.), BQ (0.04 mmol, 0.2 eq.), PhI(OAc)2 (0.6 mmol, 3.0 eq.), and Li3PO4 (0.4 mmol, 2.0 eq.) in DCE (2.0 mL) at 90 °C for 24 h. | |
To our delight, the peptide backbone directed meta-olefination product was not detected even in these long chain peptides, which further confirmed that the directing ability of silanol was better than that of the peptide backbone. The possible mechanism might be related to the formation of a kinetically less-favored six-membered palladacycle with silanol rather than the peptide backbone, and BQ could work as a special ligand.16a Further mechanistic studies and modification of longer polypeptides are currently underway.
Conclusions
In summary, we have developed the first efficient Pd-catalyzed ortho-olefination of Tyr residues by employing the traditional protecting group silanol as the directing group to achieve high chemo- and site-selectivity, which avoided the conventional directing group loading step. A broad range of substrates could be well applied in this protocol, which is tolerant to various amino acids and peptide lengths up to hexamers. Furthermore, the novel orthogonal protection strategies involving silanol on the phenol motif of Tyr are also successfully applied to prepare polypeptides via SPPS. Moreover, both N-terminal and C-terminal elongated peptides are compatible with this protocol, suggesting the potential applications of this strategy in medicinal chemistry and drug discovery.
Conflicts of interest
The authors declare no conflict of interest.
Acknowledgements
The authors acknowledge financial support by the Guangdong Natural Science Funds for Distinguished Young Scholar (no. 2018B030306017), Guangdong Province Universities and Colleges Pearl River Scholar Funded Scheme (2018), National Natural Science Foundation of China (no. 81602972), Fundamental Research Funds for the Central Universities (20ykzd15) and Guangdong Provincial Key Laboratory of Chiral Molecule and Drug Discovery (2019B030301005).
Notes and references
-
(a) J. L. Lau and M. K. Dunn, Bioorg. Med. Chem., 2018, 26, 2700 CrossRef CAS PubMed;
(b) K. Fosgerau and T. Hoffmann, Drug Discov. Today, 2015, 20, 122 CrossRef CAS PubMed;
(c) P. G. Dougherty, A. Sahni and D. Pei, Chem. Rev., 2019, 119, 10241 CrossRef CAS PubMed.
-
(a) D. J. Drucker, Nat. Rev. Drug Discov., 2020, 19, 277 CrossRef CAS PubMed;
(b) H. Malhaire, J. C. Gimel, E. Roger, J. P. Benoit and F. Lagarce, Adv. Drug Deliv. Rev., 2016, 106, 320 CrossRef CAS PubMed;
(c) M. Goldberg and I. Gomez-Orellana, Nat. Rev. Drug Discov., 2003, 2, 289 CrossRef CAS PubMed;
(d) A. F. B. Rader, M. Weinmuller, F. Reichart, A. Schumacher-Klinger, S. Merzbach, C. Gilon, A. Hoffman and H. Kessler, Angew. Chem., Int. Ed., 2018, 57, 14414 CrossRef PubMed.
-
(a) J. N. deGruyter, L. R. Malins and P. S. Baran, Biochemistry, 2017, 56, 3863 CrossRef CAS PubMed;
(b) Y. Yu, C. Hu, L. Xia and J.-Y. Wang, ACS Catal., 2018, 8, 1851 CrossRef CAS;
(c) E. Remond, C. Martin, J. Martinez and F. Cavelier, Chem. Rev., 2016, 116, 11654 CrossRef CAS PubMed.
- W. Wang, M. M. Lorion, J. Shah, A. R. Kapdi and L. Ackermann, Angew. Chem., Int. Ed., 2018, 57, 14700 CrossRef CAS PubMed.
-
(a) B. V. S. Reddy, L. R. Reddy and E. J. Corey, Org. Lett., 2006, 8, 3391 CrossRef CAS PubMed;
(b) L. D. Tran and O. Daugulis, Angew. Chem., Int. Ed., 2012, 51, 5188 CrossRef CAS PubMed;
(c) B. Wang, C. Lu, S.-Y. Zhang, G. He, W. A. Nack and G. Chen, Org. Lett., 2014, 16, 6260 CrossRef CAS PubMed;
(d) Y.-J. Liu, Y.-H. Liu, Z.-Z. Zhang, S.-Y. Yan, K. Chen and B.-F. Shi, Angew. Chem., Int. Ed., 2016, 55, 13859 CrossRef CAS PubMed;
(e) T. Liu, J. X. Qiao, M. A. Poss and J.-Q. Yu, Angew. Chem., Int. Ed., 2017, 56, 10924 CrossRef CAS PubMed;
(f) M. Bauer, W. Wang, M. M. Lorion, C. Dong and L. Ackermann, Angew. Chem., Int. Ed., 2018, 57, 203 CrossRef CAS PubMed.
-
(a) G. He, Y. Zhao, S. Zhang, C. Lu and G. Chen, J. Am. Chem. Soc., 2012, 134, 3 CrossRef CAS PubMed;
(b) G. He, S.-Y. Zhang, W. A. Nack, R. Pearson, J. Rabb-Lynch and G. Chen, Org. Lett., 2014, 16, 6488 CrossRef CAS PubMed;
(c) G. He, B. Wang, W. A. Nack and G. Chen, Acc. Chem. Res., 2016, 49, 635 CrossRef CAS PubMed;
(d) D. Mu, F. Gao, G. Chen and G. He, ACS Catal., 2017, 7, 1880 CrossRef CAS;
(e) X. Zhang, G. Lu, M. Sun, M. Mahankali, Y. Ma, M. Zhang, W. Hua, Y. Hu, Q. Wang, J. Chen, G. He, X. Qi, W. Shen, P. Liu and G. Chen, Nat. Chem., 2018, 10, 540 CrossRef CAS PubMed;
(f) L. Liu, Y.-H. Liu and B.-F. Shi, Chem. Sci., 2020, 11, 290 RSC;
(g) B.-B. Zhan, Y. Li, J.-W. Xu, X.-L. Nie, J. Fan, L. Jin and B.-F. Shi, Angew. Chem., Int. Ed., 2018, 57, 5858 CrossRef CAS PubMed;
(h) B.-B. Zhan, J. Fan, L. Jin and B.-F. Shi, ACS Catal., 2019, 9, 3298 CrossRef CAS.
-
(a) Y. Yu, L.-K. Zhang, A. V. Buevich, G. Li, H. Tang, P. Vachal, S. L. Colletti and Z.-C. Shi, J. Am. Chem. Soc., 2018, 140, 6797 CrossRef CAS PubMed;
(b) M. J. Terrey, A. Holmes, C. C. Perry and W. B. Cross, Org. Lett., 2019, 21, 7902 CrossRef CAS PubMed;
(c) A. Schischko, H. Ren, N. Kaplaneris and L. Ackermann, Angew. Chem., Int. Ed., 2017, 56, 1576 CrossRef CAS PubMed;
(d) H. Zhang, H.-Y. Wang, Y. Luo, C. Chen, Y. Cao, P. Chen, Y.-L. Guo, Y. Lan and G. Liu, ACS Catal., 2018, 8, 2173 CrossRef CAS;
(e) N. Kaplaneris, T. Rogge, R. Yin, H. Wang, G. Sirvinskaite and L. Ackermann, Angew. Chem. Int. Ed., 2019, 58, 3476 CrossRef CAS PubMed;
(f) M. M. Lorion, N. Kaplaneris, J. Son, R. Kuniyil and L. Ackermann, Angew. Chem., Int. Ed., 2019, 58, 1684 CrossRef CAS PubMed;
(g) Z. Ruan, N. Sauermann, E. Manoni and L. Ackermann, Angew. Chem., Int. Ed., 2017, 56, 3172 CrossRef CAS PubMed;
(h) W. Wang, M. M. Lorion, O. Martinazzoli and L. Ackermann, Angew. Chem., Int. Ed., 2018, 57, 10554 CrossRef CAS PubMed;
(i) A. Schischko, N. Kaplaneris, T. Rogge, G. Sirvinskaite, J. Son and L. Ackermann, Nat. Commun., 2019, 10, 3553 CrossRef PubMed.
-
(a) J. Tang, Y. He, H. Chen, W. Sheng and H. Wang, Chem. Sci., 2017, 8, 4565 RSC;
(b) Z.-B. Bai, C.-X. Cai, Z.-L. Yu and H. Wang, Angew. Chem., Int. Ed., 2018, 57, 13912 CrossRef CAS PubMed;
(c) M. J. Terrey, C. C. Perry and W. B. Cross, Org. Lett., 2019, 21, 104 CrossRef PubMed;
(d) M. San Segundo and A. Correa, Chem. Sci., 2019, 10, 8872 RSC.
-
(a) X. Chen, F. Ye, X. Luo, X. Liu, J. Zhao, S. Wang, Q. Zhou, G. Chen and P. Wang, J. Am. Chem. Soc., 2019, 141, 18230 CrossRef CAS PubMed;
(b) A. F. M. Noisier, M. J. Johansson, L. Knerr, M. A. Hayes, W. J. Drury III, E. Valeur, L. R. Malins and R. Gopalakrishnan, Angew. Chem., Int. Ed., 2019, 58, 19096 CrossRef CAS PubMed.
-
(a) J. Xu, J. Chen, F. Gao, S. Xie, X. Xu, Z. Jin and J.-Q. Yu, J. Am. Chem. Soc., 2019, 141, 1903 CrossRef CAS PubMed;
(b) L. Y. Liu, J. X. Qiao, K. S. Yeung, W. R. Ewing and J.-Q. Yu, J. Am. Chem. Soc., 2019, 141, 14870 CrossRef CAS PubMed;
(c) Z. Huang and J.-P. Lumb, ACS Catal., 2018, 9, 521 CrossRef;
(d) J.-L. Dai, N.-Q. Shao, J. Zhang, R.-P. Jia and D.-H. Wang, J. Am. Chem. Soc., 2017, 139, 12390 CrossRef CAS PubMed;
(e) Q. Bu, T. Rogge, V. Kotek and L. Ackermann, Angew. Chem., Int. Ed., 2018, 57, 765 CrossRef CAS PubMed;
(f) Q.-Q. Wang, S. An, Z.-Q. Deng, W.-J. Zhu, Z.-Y. Huang, G. He and G. Chen, Nat. Catal., 2019, 2, 793 CrossRef CAS.
-
(a) J. Ertl, M. E. Ortiz-Soto, T.-A. Le, J. Bechold, J. Shan, J. Tessmar, B. Engels and J. Seibel, Chem.–Eur. J., 2019, 25, 6533 CrossRef CAS PubMed;
(b) J. L. Belmonte-Vazquez, E. Avellanal-Zaballa, E. Enriquez-Palacios, L. Cerdan, I. Esnal, J. Banuelos, C. Villegas-Gomez, I. Lopez Arbeloa and E. Pena-Cabrera, J. Org. Chem., 2019, 84, 2523 CrossRef CAS PubMed;
(c) C.-L. Song, K. Liu, Z.-J. Wang, B. Ding, S.-C. Wang, Y. Weng, C. W. Chiang and A.-W. Lei, Chem. Sci., 2019, 10, 7982 RSC.
-
(a) G. Li, L. Wan, G. Zhang, D. Leow, J. Spangler and J.-Q. Yu, J. Am. Chem. Soc., 2015, 137, 4391 CrossRef CAS PubMed;
(b) S. Lee, H. Lee and K.-L. Tan, J. Am. Chem. Soc., 2013, 135, 18778 CrossRef CAS PubMed;
(c) K. Hong, H. Park and J.-Q. Yu, ACS Catal., 2017, 7, 6938 CrossRef CAS PubMed;
(d) B. E. Haines and D. G. Musaev, ACS Catal., 2015, 5, 830 CrossRef CAS;
(e) F.-L. Zhang, K. Hong, T.-J. Li, H. Park and J.-Q. Yu, Science, 2016, 351, 252 CrossRef CAS PubMed;
(f) Q.-F. Wu, P.-X. Shen, J. He, X.-B. Wang, F. Zhang, Q. Shao, R.-Y. Zhu, C. Mapelli, J. X. Qiao, M. A. Poss and J.-Q. Yu, Science, 2017, 355, 499 CrossRef CAS PubMed;
(g) R.-Y. Zhu, Z.-Q. Li, H. S. Park, C. H. Senanayake and J.-Q. Yu, J. Am. Chem. Soc., 2018, 140, 3564 CrossRef CAS PubMed;
(h) M. Shang, K. S. Feu, J. C. Vantourout, L. M. Barton, H. L. Osswald, N. Kato, K. Gagaring, C. W. McNamara, G. Chen, L. Hu, S. Ni, P. Fernandez-Canelas, M. Chen, R. R. Merchant, T. Qin, S. L. Schreiber, B. Melillo, J. Q. Yu and P. S. Baran, Proc. Natl. Acad. Sci., 2019, 116, 8721 CrossRef CAS PubMed;
(i) P. Gandeepan and L. Ackermann, Chem, 2018, 4, 199 CrossRef CAS.
-
(a) M. Parasram and V. Gevorgyan, Acc. Chem. Res., 2017, 50, 2038 CrossRef CAS PubMed;
(b) Y. Wang and V. Gevorgyan, Angew. Chem., Int. Ed., 2017, 56, 3191 CrossRef CAS PubMed;
(c) D. Sarkar, A. V. Gulevich, F. S. Melkonyan and V. Gevorgyan, ACS Catal., 2015, 5, 6792 CrossRef CAS;
(d) D. Sarkar, F. S. Melkonyan, A. V. Gulevich and V. Gevorgyan, Angew. Chem., Int. Ed., 2013, 52, 10800 CrossRef CAS PubMed;
(e) A. V. Gulevich, F. S. Melkonyan, D. Sarkar and V. Gevorgyan, J. Am. Chem. Soc., 2012, 134, 5528 CrossRef CAS PubMed;
(f) M. Parasram, P. Chuentragool, D. Sarkar and V. Gevorgyan, J. Am. Chem. Soc., 2016, 138, 6340 CrossRef CAS PubMed.
-
(a) C. Huang, B. Chattopadhyay and V. Gevorgyan, J. Am. Chem. Soc., 2011, 133, 12406 CrossRef CAS PubMed;
(b) C. Huang, N. Ghavtadze, B. Chattopadhyay and V. Gevorgyan, J. Am. Chem. Soc., 2011, 133, 17630 CrossRef CAS PubMed;
(c) Y. Wang and V. Gevorgyan, Angew. Chem., Int. Ed., 2015, 54, 2255 CrossRef CAS PubMed.
-
(a) P. S. Thuy-Boun, G. Villa, D. Dang, P. Richardson, S. Su and J.-Q. Yu, J. Am. Chem. Soc., 2013, 135, 17508 CrossRef CAS PubMed;
(b) L. Liu, H. Yuan, T.-T. Fu, T. Wang, X. Gao, Z.-P. Zeng, J. Zhu and Y.-F. Zhao, J. Org. Chem., 2014, 79, 80 CrossRef CAS PubMed;
(c) Y. Lu, D. S. Leow, X.-S. Wang, K. M. Engle and J.-Q. Yu, Chem. Sci., 2011, 2, 967 RSC;
(d) R. Jazzar, J. Hitce, A. Renaudat, J. Sofack-Kreutzer and O. Baudoin, Chem. - Eur. J., 2010, 16, 2654 CrossRef CAS PubMed.
-
(a) C.-G. Jia, W.-J. Lu, T. Kitamura and Y. Fujiwara, Org. Lett., 1999, 1, 2097 CrossRef CAS;
(b) K. Hull and M. S. Sanford, J. Am. Chem. Soc., 2009, 131, 9651 CrossRef CAS PubMed;
(c) B. Milani, A. Anzilutti, L. Vicentini, A. S. Santi, E. Zangrando, S. Geremia and G. Mestroni, Organometallics, 1997, 16, 5064 CrossRef CAS.
Footnote |
† Electronic supplementary information (ESI) available. See DOI: 10.1039/d0sc02439b |
|
This journal is © The Royal Society of Chemistry 2020 |
Click here to see how this site uses Cookies. View our privacy policy here.