DOI:
10.1039/D0SC01932A
(Edge Article)
Chem. Sci., 2020,
11, 4948-4953
Palladium-catalysed 5-endo-trig allylic (hetero)arylation†
Received
4th April 2020
, Accepted 18th April 2020
First published on 30th April 2020
Abstract
A palladium-catalysed intramolecular allylic (hetero)arylation strategy for the synthesis of fused cyclopentenes incorporated with all-carbon quaternary and spiro centres is described. The method is straightforward, shows broad scope, proceeds in synthetically useful yields, and provides a rare means to construct complex cyclopentanoids. The reaction is believed to involve a kinetically unfavourable 5-endo-trig carbocyclisation of the tethered (π-allyl)palladium system. Further, this method was successfully applied as the key step in the total synthesis of diterpene natural products taiwaniaquinone H and dichroanone.
Introduction
Cyclopentannulated arenes and heteroarenes are the primary molecular architectures of many bioactive natural products and drug candidates; they also find potential applications in medicinal chemistry and in materials science.1 Among them are a sizeable number of bioactive molecules that encompass quaternary carbon atoms on one or more stereogenic centers.2 Some of the representative bioactive molecules possessing fused cyclopentane scaffolds and featuring at least one all-carbon quaternary/spiro centre are shown in Fig. 1.3
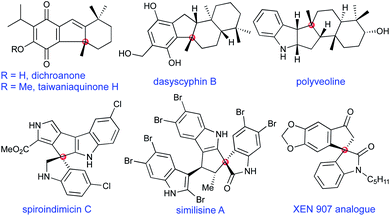 |
| Fig. 1 Some of the representative biologically active molecules under the purview of this work. | |
Among several methods for the synthesis of cyclopenta-fused arenes and heteroarenes, to our knowledge, a disconnection based on 5-endo-trig cyclisation mode is yet to be realised. Baldwin's rules describe 5-endo-trig cyclisation as a stereoelectronically disfavoured pathway owing to the geometric constraints of the reactive functional groups not being able to achieve the Burgi–Dunitz angle.4
Palladium-catalysed allylic alkylations have been the subject of intensive investigations subsequent to the seminal contributions of Tsuji and Trost, Scheme 1a.5 The construction of nearly all types of rings can be assumed by employing Tsuji–Trost chemistry.6 However, Pd-catalysed 5-endo-trig carbocyclisation leading to the formation of cyclopentanes is quite uncommon, Scheme 1b.7 Against this background, Malacria in 1998 reported an example depicting Pd-catalysed and silicon-directed 5-endo-trig cyclisation for the formation of a silylated cyclopentene.8 Recently, the Tius group demonstrated Pd-catalysed transformation of diketoesters to 2-hydroxycyclopentenones.9 While the reaction is considered to be an intramolecular allylic alkylation reaction,10 the possibility of Pd-catalysed anionic 5-endo-trig cyclisation may not be ruled out.7,11 A mechanistically distinct event on a similar substrate design was reported by Oestreich and co-workers, where palladium catalyses 5-endo-trig cyclisation of 1-(1H-indolyl)prop-2-enones for the formation of 3H-pyrrolo[1,2-a]indole-3-ones.12
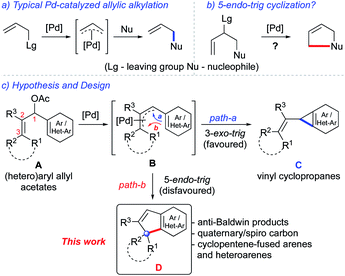 |
| Scheme 1 Pd-catalysed 5-endo-trig allylic arylation for fused cyclopentenes incorporated with a quaternary/spiro centre: this work. | |
Having developed several catalytic strategies for the synthesis of various types of cyclopropanoids and cyclopentanoids,13 we envisioned that appropriately substituted (hetero)aryl allyl acetates A under palladium catalysis will generate (π-allyl)palladium species B, which, by undergoing 3-exo-trig cyclisation will deliver vinyl cyclopropanes C (path-a), Scheme 1c.14 On the other hand, a possibility for the formation of fused cyclopentenes D may also arise if B undergoes rather unfavorable 5-endo-trig cyclisation (path-b). It is interesting to note that whenever competition arises, palladium catalysis usually favours the 3-exo-trig process over 5-endo-trig cyclisation; however, this fact may derive from kinetic preference.15
Here, we describe a general and straightforward Pd(II)-catalysed 5-endo-trig allylic (hetero)arylation of (hetero)aryl allyl acetates A that provides ready access to a wide range of indenes and cyclopentene-fused heteroarenes D incorporated with an all-carbon quaternary or a spiro centre, which, to our knowledge, have not been achieved thus far, as shown in Scheme 1c. At the beginning of the study, it was expected that the formation of C may outweigh the formation of D based on the considerations that the 5-endo-trig cyclisation mode is disfavoured (especially over 3-exo-trig process)14–16 and also that the steric encumbrance at C-3 (of B) may further discourage the formation of D.
Results and discussion
In order to verify the hypothesis presented in Scheme 1c, the oxindole-based allyl acetate 1a was prepared and the reaction was evaluated with respect to the solvent, temperature, and catalyst, Table 1. Initial screening with Pd(0) catalysts provided mixed results. For example, Pd(PPh3)4 showed no reaction while Pd2(dba)3 provided encouraging results by generating the desired product 2a, although in moderate yield (entries 1 and 2). The structure of 2a was readily deduced from the NMR data, and was eventually confirmed by the single-crystal X-ray diffraction analysis of 2a.17
Table 1 Optimisation of the reaction parametersa,b,c
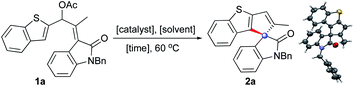
|
Entry |
Catalyst (10 mol%) |
Solvent |
Time (h) |
Yieldd (%) |
Reaction conditions: see the ESI for details.
Yield of the reaction at 30 °C (for 36 h) is 53%; yield at 45 °C (for 12 h) is 62%.
No reaction was observed with either Ni(cod)2 or [Ir(cod)Cl]2.
Isolated yield after column chromatography.
1a decomposed.
5 mol% PdCl2 was employed.
In the presence of 1 equiv. of 2,6-di-tert-butyl-4-methylpyridine (DTBMP).
In the presence of 1 equiv. of N,N-dimethylaminopyridine (DMAP).
|
1 |
Pd(PPh3)4 |
1,2-DCE |
48 |
— |
2 |
Pd2(dba)3 |
1,2-DCE |
48 |
48 |
3 |
Pd(PPh3)2Cl2 |
1,2-DCE |
48 |
— |
4 |
Pd(OAc)2 |
1,2-DCE |
48 |
30 |
5 |
PdCl2 |
1,2-DCE |
8 |
82 |
6 |
PdCl2 |
Toluene |
30 |
43 |
7e |
PdCl2 |
DMF |
10 |
— |
8 |
PdCl2 |
MeCN |
48 |
35 |
9 |
PdCl2 |
MeNO2 |
6 |
73 |
10 |
PdCl2 |
1,4-Dioxane |
36 |
63 |
11f |
PdCl2 |
1,2-DCE |
18 |
75 |
12 |
— |
1,2-DCE |
120 |
— |
13g |
PdCl2 |
1,2-DCE |
22 |
76 |
14h |
PdCl2 |
1,2-DCE |
16 |
74 |
Among Pd(II) catalysts, interestingly, Pd(OAc)2 provided 2a in poor yield, but the reaction catalysed by PdCl2 furnished 2a in good yield in a short reaction time (entries 3–5). As part of our efforts to improve the efficiency of the reaction, a brief solvent screening was undertaken, which did not provide any better result (entries 6–10). Lowering the catalyst loading to 5 mol% prolonged the reaction time and resulted in less yield of the product (entry 11). Further, no reaction was observed in the absence of any catalyst (entry 12) and the reactions which were performed in the presence of 2,6-di-tert-butyl-4-methylpyridine (DTBMP) or N,N-dimethylaminopyridine (DMAP), to rule out the possibility of any traces of HCl catalyzing the reaction, delivered 2a in good yields (entries 13 and 14).
Thus, an unusual Pd-catalysed 5-endo-trig spirannulation of oxindoles was established based on rational designing of the precursor. Unlike several palladium-catalysed reactions, intriguingly, this reaction does not require any external additives, oxidants, bases, or ligands.18
Under the optimised conditions, the generality of the method was evaluated. It is evident from Table 2 that a wide range of heteroarene-fused spirocyclopentene oxindoles possessing electronically diverse substituents can be obtained in good to excellent yields (2a–2k). The method can also be extended to the synthesis of indeno spiroxindoles such as 2l. In particular, the reaction works efficiently from both C-2 and C-3 of benzothiophenes (2a–2dvs.2e–2f), indoles (2g–2jvs.2k), and arenes (2l) indicating that the reaction may not be involving a C–H activation pathway.19
Table 2 Generality and scope: spiroxindolesa,b
Reaction conditions: see the ESI for details.
Isolated yield after column chromatography.
With the OBoc substrate instead of OAc.
|
|
Spirocyclopentane oxindoles are known to be privileged structures with regard to their structural complexity and their biological activity.20 In this context, it is worth highlighting that 2k represents part of the structure of natural products such as similisines and spiroindimicins (see Fig. 1) whereas 2e and 2f serve as their sulphur analogues.3 On the other hand, 2l represents a XEN 907 analogue.3
A critical role of the substituent (R1) was realised during the evaluation of the substrate scope, Table 2. For example, when 1m was treated under the optimised conditions, only the deacylated product 3m was realised.21
Having established an unprecedented approach for the synthesis of oxindoles bearing a spiro center, we intended to extend the concept for the creation of an all-carbon quaternary center, Table 3. It was expected that the substrate design 4 will provide cyclopentene-fused arenes and heteroarenes 5 having a quaternary carbon. Indeed, after brief optimisation of the reaction conditions, we could achieve the synthesis of natural product-like tri-, tetra- and pentacyclic indenes (5a–5d) and cyclopentannulated heteroarenes (5e–5i) in good to excellent yields. The structure of the representative compound (5e) was unambiguously confirmed by X-ray diffraction analysis.17 The reaction of 4j (where R1 = H) under the prototypical conditions generated a complex mixture, thereby signifying the role of the substituent (R1) in the outcome of the reaction. Nevertheless, some of the merits of this strategy are: (i) neutral conditions, (ii) easily accessible starting compounds, (iii) occurrence of numerous natural products and pharmaceutically relevant compounds with the kind of molecular architecture accessed herein.1,3
Table 3 Substrate scope: cyclopentene-fused arenes and heteroarenesa,b
Reaction conditions: see the ESI for details.
Chromatographic yields.
|
|
We then turned our attention to establish the mechanism. Based on the experimental evidence22 and related literature reports,18 a plausible mechanism is proposed in Scheme 2. The reaction begins with the formation of an (η3-allyl)palladium complex E,23 which reorganises itself into F owing to steric considerations. The intermediate F is now poised to undergo an ene-type 5-endo-trig cyclisation to generate another π-allylpalladium species G, which upon rearomatisation via deprotonation24 delivers 2 (or 5) and regenerates the active catalyst. The measurement of the kinetic isotope effect (KIE)25 strongly suggested that the new C–C bond-forming cyclisation event most likely involved an ene-type allylic arylation step rather than a C–H activation pathway. Otherwise, typical metal-catalysed C–H functionalisation processes involve C–H bond cleavage in the rate determining step.26
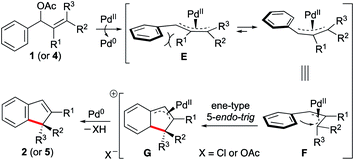 |
| Scheme 2 Plausible reaction mechanism. | |
Next, we decided to apply the current strategy in the total synthesis of taiwaniaquinone H 9 and dichroanone 10, Scheme 3.3 Taiwaniaquinoids are a family of diterpenoid natural products possessing a [6,5,6]-abeo-abietane skeleton with an all-carbon quaternary stereocenter at the pseudobenzylic position. Some of the members of taiwaniaquinoids exhibit potent cytotoxic activity against KB epidermoid carcinoma cancer cells, antitumor activity, aromatase inhibitory activity, to mention a few.27 Owing to their diverse bioactivity profiles and complex structural features, taiwaniaquinoids have attracted the attention of synthetic chemists.3b,28
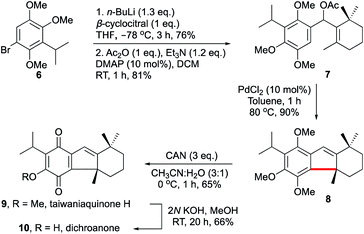 |
| Scheme 3 Acid-free synthesis of bioactive natural products taiwaniaquinone H and dichroanone. | |
Our strategy for the synthesis of 9 and 10 involved palladium-catalysed 5-endo-trig cyclisation of 7 to 8 as the key step, Scheme 3. Accordingly, the desired acetate 7 was synthesised by the addition of the lithiated 6 to β-cyclocitral followed by acylation of the intermediate alcohol.3b The reaction of 7 under the prototypical conditions described in Table 3 generated 8 in excellent yield. We then accomplished the total synthesis of taiwaniaquinone H 9 in 65% yield by performing ceric ammonium nitrate (CAN)-mediated oxidative transformation of 8. Subsequently, the total synthesis of dichroanone 10 was also achieved in 66% yield by subjecting 9 to methanolic KOH conditions. As such, the synthesis of 8 also represents the formal synthesis of taiwaniaquinol B.28 The synthetic methodology delineated herein is general and can pave the way for the acid-free synthesis of several other related taiwaniaquinoids as well.
Conclusions
An efficient Pd(II)-promoted 5-endo-trig cyclisation of (hetero)aryl allyl acetates to spirocyclopentene oxindoles, indenes and cyclopentene-fused heteroarenes is presented.29 A hallmark of this strategy is its ability to construct a quaternary or a spiro carbon center from easily accessible starting compounds under neutral conditions. Since the method represents one of the very few examples for highly efficient synthesis of complex cyclopentanoids under oxidant, base, additive or ligand-free conditions, it should find practical applications in the synthesis of natural products, pharmaceutically relevant compounds and materials. We are in the process of extending this work for the creation of new spiro structures, and applying the current strategy in the total synthesis of the spiroindimicin and polyveoline family of natural products. The results will be communicated in due course.
Conflicts of interest
There are no conflicts to declare.
Acknowledgements
IISER Mohali is thanked for funding, and for the NMR, mass and departmental X-ray facilities. S. S. V. R. thanks the DST for the Swarnajayanti fellowship (DST/SJF/CSA-01/2017-18), and the SERB for the Core Research Grant (CRG/2018/000016). B. S. thanks the UGC, and S. K. B., and K. K. thank IISER Mohali for the research fellowships.
Notes and references
-
(a) M. Somei and F. Yamada, Nat. Prod. Rep., 2005, 22, 73 RSC;
(b) S. Cacchi and G. Fabrizi, Chem. Rev., 2005, 105, 2873 CrossRef CAS PubMed;
(c) A. J. Kochanowska-Karamyan and M. T. Hamann, Chem. Rev., 2010, 110, 4489 CrossRef CAS PubMed;
(d) Y. Ie, K. Nishida, M. Karakawa, H. Tada and Y. Aso, J. Org. Chem., 2011, 76, 6604 CrossRef CAS PubMed;
(e) B. Gabriele, R. Mancuso and L. Veltri, Chem.–Eur. J., 2016, 22, 5056 CrossRef CAS PubMed;
(f) T. Chanda and M. S. Singh, Org. Biomol. Chem., 2016, 14, 8895 RSC;
(g) B. Satpathi, A. Mondal and S. S. V. Ramasastry, Chem.–Asian J., 2018, 13, 1642 CrossRef CAS PubMed;
(h) T. Vivekanand, B. Satpathi, S. K. Bankar and S. S. V. Ramasastry, RSC Adv., 2018, 8, 18576 RSC.
- The construction of quaternary/spiro carbon centres is challenging even in the context of modern organic synthesis, see:
(a) A. Y. Hong and B. M. Stoltz, Eur. J. Org. Chem., 2013, 14, 2745 CrossRef PubMed;
(b) K. W. Quasdorf and L. E. Overman, Nature, 2014, 516, 181 CrossRef CAS PubMed;
(c) E. V. Prusov, Angew. Chem., Int. Ed., 2017, 56, 14356 CrossRef CAS PubMed;
(d) P.-W. Xu, J.-S. Yu, C. Chen, Z.-Y. Cao, F. Zhou and J. Zhou, ACS Catal., 2019, 9, 1820 CrossRef CAS.
- For dichroanone and taiwaniaquinones, see:
(a) G. Majetich and J. M. Shimkus, J. Nat. Prod., 2010, 73, 284 CrossRef CAS PubMed;
(b) B. N. Kakde, A. Parida, P. Kumari and A. Bisai, Tetrahedron Lett., 2016, 57, 3179 CrossRef CAS . For dasyscyphins, see:;
(c) L. Zhang, X. Xie, J. Liu, J. Qi, D. Ma and X. She, Org. Lett., 2011, 13, 2956 CrossRef CAS PubMed . For polyveoline and similisines, see:;
(d) S. Dhiman and S. S. V. Ramasastry, Org. Lett., 2015, 17, 5116 CrossRef CAS PubMed . For spiroindimicins, see:;
(e) W. Zhang, Z. Liu, S. Li, T. Yang, Q. Zhang, L. Ma, X. Tian, H. Zhang, C. Huang, S. Zhang, J. Ju, Y. Shen and C. Zhang, Org. Lett., 2012, 14, 3364 CrossRef CAS PubMed . For XEN 907 and analogues, see:;
(f) S. Chowdhury, M. Chafeev, S. Liu, J. Sun, V. Raina, R. Chui, W. Young, R. Kwan, J. Fu and J. A. Cadieux, Bioorg. Med. Chem. Lett., 2011, 21, 3676 CrossRef CAS PubMed.
-
(a) J. E. Baldwin, J. Chem. Soc., Chem. Commun., 1976, 734 RSC;
(b) J. E. Baldwin, J. Cutting, W. Dupont, L. Kruse, L. Silberman and R. C. Thomas, J. Chem. Soc., Chem. Commun., 1976, 736 RSC;
(c) K. Gilmore, M. Manoharan, J. I. Wu, P. V. R. Schleyer and I. V. Alabugin, J. Am. Chem. Soc., 2012, 134, 10584 CrossRef CAS PubMed;
(d) I. V. Alabugin and K. Gilmore, Chem. Commun., 2013, 49, 11246 RSC.
-
(a) J. Tsuji, H. Takahashi and M. Morikawa, Tetrahedron Lett., 1965, 6, 4387 CrossRef;
(b) B. M. Trost and T. J. Fullerton, J. Am. Chem. Soc., 1973, 95, 292 CrossRef CAS.
-
(a) B. M. Trost, Acc. Chem. Res., 1996, 29, 355 CrossRef CAS;
(b) G. Jellerichs, J.-R. Kong and M. J. Krische, J. Am. Chem. Soc., 2003, 125, 7758 CrossRef PubMed;
(c) U. Kazmaier, Curr. Org. Chem., 2003, 7, 317 CrossRef CAS;
(d) B. M. Trost and M. L. Crawley, Chem. Rev., 2003, 103, 2921 CrossRef CAS PubMed;
(e) H. Miyabe and Y. Takemoto, Synlett, 2005, 11, 1641 Search PubMed;
(f) S.-L. You and L.-X. Dai, Angew. Chem., Int. Ed., 2006, 45, 5246 CrossRef CAS PubMed;
(g) M. Braun and T. Meier, Angew. Chem., Int. Ed., 2006, 45, 6952 CrossRef CAS PubMed;
(h) I. Ibrahem and A. Còrdova, Angew. Chem., Int. Ed., 2006, 45, 1952 CrossRef CAS PubMed;
(i) Z. Lu and S. Ma, Angew. Chem., Int. Ed., 2008, 47, 258 CrossRef CAS PubMed;
(j) T. Jensen and P. Fristrup, Chem.–Eur. J., 2009, 15, 9632 CrossRef CAS PubMed;
(k) J. D. Weaver, A. Recio III, A. J. Grenning and J. A. Tunge, Chem. Rev., 2011, 111, 1846 CrossRef CAS PubMed;
(l) M. J. Wanner, E. Claveau, J. H. van Maarseveen and H. Hiemstra, Chem.–Eur. J., 2011, 17, 13680 CrossRef CAS PubMed;
(m) I. D. G. Watsona and F. D. Toste, Chem. Sci., 2012, 3, 2899 RSC;
(n) K. C. Majumdar and B. Sinha, Synthesis, 2013, 45, 1271 CrossRef CAS;
(o) C. Kammerer, G. Prestat, D. Madec and G. Poli, Acc. Chem. Res., 2014, 47, 3439 CrossRef CAS PubMed;
(p) Y. Liu, S.-J. Han, W.-B. Liu and B. M. Stoltz, Acc. Chem. Res., 2015, 48, 740 CrossRef CAS PubMed;
(q) B. M. Trost, Tetrahedron, 2015, 71, 5708 CrossRef CAS PubMed;
(r) R. A. Fernandes and J. L. Nallasivam, Org. Biomol. Chem., 2019, 17, 8647 RSC.
-
Pd-catalyzed anionic rearrangement of vinyl cyclopropanes to cyclopentenes, see:
(a) Y. Morizawa, K. Oshima and H. Nozaki, Tetrahedron Lett., 1982, 2871 CrossRef CAS;
(b) M. Ahmar, B. Gazes and J. Gore, Tetrahedron, 1987, 43, 3453 CrossRef CAS;
(c) K. Hiroi and Y. Arinaga, Tetrahedron Lett., 1994, 35, 153 CrossRef CAS.
- For a single example of silicon-directed Pd-catalyzed 5-endo-trig cyclization, see: S. Thorimbert and M. Malacria, Tetrahedron Lett., 1998, 39, 9659 CrossRef CAS.
- For the Tius-Nazarov reaction, see:
(a) C. Bee, E. Leclerc and M. A. Tius, Org. Lett., 2003, 5, 4927 CrossRef CAS PubMed;
(b) Z. Cai and M. Harmata, Org. Lett., 2010, 12, 5668 CrossRef CAS PubMed;
(c) N. Shimada, C. Stewart, W. F. Bow, A. Jolit, K. Wong, Z. Zhou and M. A. Marcus, Angew. Chem., Int. Ed., 2012, 51, 5727 CrossRef CAS PubMed . For related Pd-catalyzed Nazarov-type reactions, see:;
(d) S. S. Subramanium, S. Handa, A. J. Miranda and L. M. Slaughter, ACS Catal., 2011, 1, 1371 CrossRef CAS;
(e) J. Shao, P. Hu, G. Hong, M. Fang, X. Li and X. Xu, Synlett, 2014, 25, 1009 CrossRef CAS.
- T. A. Atesin, G. M. Martinez and D. Flores, Organometallics, 2017, 36, 3589 CrossRef CAS.
- For formal anionic 5-endo-trig cyclizations, see:
(a) P. Beak and D. A. Burg, J. Org. Chem., 1989, 54, 1647 CrossRef CAS;
(b) W. A. Batson, D. Sethumadhavan and M. A. Tius, Org. Lett., 2005, 7, 2771 CrossRef CAS PubMed;
(c) C. P. Johnston, A. Kothari, T. Sergeieva, S. I. Okovytyy, K. E. Jackson, R. S. Paton and M. D. Smith, Nat. Chem., 2015, 7, 171 CrossRef CAS PubMed.
- S. R. Kandukuri, J. A. Schiffner and M. Oestreich, Angew. Chem., Int. Ed., 2012, 51, 1265 CrossRef CAS PubMed.
- Some selected references:
(a) S. Dhiman and S. S. V. Ramasastry, Chem. Commun., 2015, 51, 557 RSC;
(b) S. Dhiman and S. S. V. Ramasastry, Org. Lett., 2015, 17, 5116 CrossRef CAS PubMed;
(c) B. Satpathi and S. S. V. Ramasastry, Angew. Chem., Int. Ed., 2016, 55, 1777 CrossRef CAS PubMed;
(d) M. Raghu, J. Grover and S. S. V. Ramasastry, Chem.–Eur. J., 2016, 22, 18316 CrossRef CAS PubMed;
(e) S. K. Bankar, B. Singh, P. Tung and S. S. V. Ramasastry, Angew. Chem., Int. Ed., 2018, 57, 1678 CrossRef CAS PubMed;
(f) A. Mondal, R. Hazra, J. Grover, M. Raghu and S. S. V. Ramasastry, ACS Catal., 2018, 8, 2748 CrossRef CAS;
(g) U. K. Mishra, K. Patel and S. S. V. Ramasastry, Org. Lett., 2019, 21, 175 CrossRef CAS PubMed;
(h) B. Satpathi, L. Dutta and S. S. V. Ramasastry, Org. Lett., 2019, 21, 170 CrossRef CAS PubMed;
(i) K. Patel, U. K. Mishra, D. Mukhopadhyay and S. S. V. Ramasastry, Chem.–Asian J., 2019, 14, 4568 CrossRef CAS PubMed.
- 3-Exo-trig closures have extremely low activation barriers, see: B. Fiser, J. M. Cuerva and E. Gomez-Bengoa, Organometallics, 2018, 37, 390 CrossRef CAS.
- According to Trost, cyclopropanes are normally preferred with carbon nucleophiles, but this may be a kinetic preference and does not test the feasibility of five-membered ring formation via a 5-endo-trig cyclization, for details see: B. M. Trost and T. S. Scanlan, J. Am. Chem. Soc., 1989, 111, 4988 CrossRef CAS.
- For a recent example where the 3-exo-trig mode is preferred over 5-endo-trig, see: J. Braun, M. I. Ariëns, B. T. Matsuo, S. de Vries, E. D. H. van Wordragen, B. D. Ellenbroek, C. M. L. V. Velde, R. V. A. Orru and E. Ruijter, Org. Lett., 2018, 20, 6611 CrossRef CAS PubMed.
- X-ray crystallographic data of compounds 2a and 5e are provided in the ESI.† CCDC 1965370 (2a) and 1965374 (5e)..
-
(a) F.-Q. Yuan, L.-X. Gao and F.-S. Han, Chem. Commun., 2011, 47, 5289 RSC;
(b) F.-Q. Yuan, F.-Y. Sun and F.-S. Han, Tetrahedron, 2012, 68, 6837 CrossRef CAS.
- Considering the comparable efficiency with arenes and heteroarenes, the reaction can be better described as an ene-type process. Further, it is well-established that heteroarenes respond differently (from C-2 and C-3) under C–H activation conditions. For example, see:
(a) B. S. Lane and D. Sames, Org. Lett., 2004, 6, 2897 CrossRef CAS PubMed;
(b) N. P. Grimster, C. Gauntlett, C. R. A. Godfrey and M. J. Gaunt, Angew. Chem., Int. Ed., 2005, 44, 3125 CrossRef CAS PubMed;
(c) D. R. Stuart, E. Villemure and K. Fagnou, J. Am. Chem. Soc., 2007, 129, 12072 CrossRef CAS PubMed;
(d) X. C. Cambeiro, N. Ahlsten and I. Larrosa, J. Am. Chem. Soc., 2015, 137, 15636 CrossRef CAS PubMed;
(e) S. Zhang, Y.-H. Niu and X.-S. Ye, Org. Lett., 2017, 19, 3608 CrossRef CAS PubMed.
- Some
selected recent studies:
(a) J.-N. Desrosiers, L. Hie, S. Biswas, O. V. Zatolochnaya, S. Rodriguez, H. Lee, N. Grinberg, N. Haddad, N. K. Yee, N. K. Garg and C. H. Senanayake, Angew. Chem., Int. Ed., 2016, 55, 11921 CrossRef CAS PubMed;
(b) R. Samineni, J. Madapa, P. Srihari and G. Mehta, Org. Lett., 2017, 19, 3119 CrossRef CAS PubMed;
(c) L. Wang, S. Li, M. Blümel, R. Puttreddy, A. Peuronen, K. Rissanen and D. Enders, Angew. Chem., Int. Ed., 2017, 56, 8516 CrossRef CAS PubMed;
(d) C.-C. Wang, J. Huang, X.-H. Li, S. Kramer, G.-Q. Lin and X.-W. Sun, Org. Lett., 2018, 20, 2888 CrossRef CAS PubMed;
(e) P. D. Chaudhari, B.-C. Hong, C.-L. Wen and G.-H. Lee, ACS Omega, 2019, 4, 655 CrossRef CAS PubMed.
- Mass analysis of the crude reaction mixture also did not indicate the formation of the required product. See the ESI† for details.
- Experimental observations indicated the pronounced effect of the substitution at R1 (see Tables 2 and 3). We believe that R1 plays a dual role – electronic and steric. R1 stabilises cationic π-allylpalladium intermediates such as E and G. Especially, the carbon connected to R1 bears a formal positive charge in G; therefore the tertiary carbocation is stabilized. The steric role of R1 is also shown in Scheme 2. We believe that R1 is responsible in converting E to F. In order for this reaction to happen, the attainment of form F is necessary.
- The substrate itself may reduce Pd(II) to Pd(0) during the reaction (analogous to Wacker-type process), and consequently, the in situ formed Pd(0) serves as the active metal center to promote the subsequent allylation reaction. The formation of Pd black in our reactions is an indicative of this proposition. For a discussion on substrate-induced reduction of Pd(II), see: I. P. Beletskaya and A. V. Cheprakov, Chem. Rev., 2000, 100, 3009 CrossRef CAS PubMed and references cited therein.
- For deprotonation pathways in Pd-catalyzed reactions, see:
(a) D. Garcia-Cuadrado, P. de Mendoza, A. A. C. Braga, F. Maseras and A. M. Echavarren, J. Am. Chem. Soc., 2007, 129, 6880 CrossRef CAS PubMed;
(b) S. I. Gorelsky, D. Lapointe and K. Fagnou, J. Am. Chem. Soc., 2008, 130, 10848 CrossRef CAS PubMed;
(c) B. Liegault, I. Petrov, S. I. Gorelsky and K. Fagnou, J. Org. Chem., 2010, 75, 1047 CrossRef CAS PubMed.
- The kH/kD was found to be 0.51. See the ESI† for details.
- For the study of kinetic isotope effects in organometallic reactions, see:
(a) W. D. Jones, Acc. Chem. Res., 2003, 36, 140 CrossRef CAS PubMed;
(b) J.-J. Li, R. Giri and J.-Q. Yu, Tetrahedron, 2008, 64, 6979 CrossRef CAS;
(c) A. García-Rubia, B. Urones, R. G. Arrayas and J. C. Carretero, Chem.–Eur. J., 2010, 16, 9676 CrossRef PubMed;
(d) M. Gomez-Gallego and M. A. Sierra, Chem. Rev., 2011, 111, 4857 CrossRef CAS PubMed;
(e) E. M. Simmons and J. F. Hartwig, Angew. Chem., Int. Ed., 2012, 51, 3066 CrossRef CAS PubMed.
-
(a) T. Minami, M. Iwamoto, H. Ohtsu, H. Ohishi, R. Tanaka and A. Yoshitake, Planta Med., 2002, 68, 742 CrossRef CAS PubMed;
(b) C. I. Chang, J. Y. Chang, C. C. Kuo, W. Y. Pan and Y. H. Kuo, Planta Med., 2005, 71, 72 CrossRef CAS PubMed;
(c) T. Katoh, T. Akagi, C. Noguchi, T. Kajimoto, M. Node, R. Tanaka, M. Nishizawa, H. Ohtsu, N. Suzuki and K. Saito, Bioorg. Med. Chem., 2007, 15, 2736 CrossRef CAS PubMed.
- G. Majetich and J. M. Shimkus, Tetrahedron Lett., 2009, 50, 3311 CrossRef CAS and references cited therein.
- To rule out PdCl2 acting as a Lewis acid, the reaction of 1e was performed with Lewis acids such as MgBr2, InCl3, and ZnCl2. These reactions hardly gave any desired product. See the ESI† for details.
Footnotes |
† Electronic supplementary information (ESI) available. CCDC 1965370 and 1965374. For ESI and crystallographic data in CIF or other electronic format see DOI: 10.1039/d0sc01932a |
‡ These authors contributed equally to this work. |
|
This journal is © The Royal Society of Chemistry 2020 |