DOI:
10.1039/C9SC05894J
(Edge Article)
Chem. Sci., 2020,
11, 2414-2419
Cross-dehydrogenative coupling enables enantioselective access to CF3-substituted all-carbon quaternary stereocenters†
Received
20th November 2019
, Accepted 9th January 2020
First published on 29th January 2020
Abstract
A cross-dehydrogenative coupling strategy for enantioselective access to acyclic CF3-substituted all-carbon quaternary stereocenters has been established. By using catalytic DDQ with MnO2 as an inexpensive terminal oxidant, asymmetric cross coupling of racemic δ-CF3-substituted phenols with indoles proceeded smoothly, providing CF3-bearing all-carbon quaternary stereocenters with excellent chemo- and enantioselectivities. The generality of the strategy is further demonstrated by efficient construction of all-carbon quaternary stereocenters bearing other polyfluoroalkyl and perfluoroalkyl groups such as CF2Cl, C2F5, and C3F7.
Introduction
Enantiopure molecules bearing a trifluoromethyl-containing stereogenic center often possess desirable properties.1 Therefore, practical and robust approaches for their enantioselective synthesis are highly attractive.2 On the other hand, the catalytic enantioselective construction of all-carbon quaternary stereocenters remains one of the great challenges in organic chemistry.3 In this context, enantioselective construction of acyclic CF3-substituted all-carbon quaternary centers is particularly daunting.4–7 Since the seminal work by Shibata, current strategies are restricted to 1,4-conjugate addition to β,β-disubstituted CF3-enones or nitroolefins (Scheme 1a),4 and two isolated methods including substitution of a propargyl electrophile (Scheme 1b),5 and hydrohydroxymethylation of CF3-bearing allenes (Scheme 1c).6 All of these methods rely on reactive functional groups, and extra steps are usually involved for their incorporation. Developing a strategically different C–H functionalization approach for enantioselective construction of CF3-bearing all-carbon quaternary centers is highly desirable.
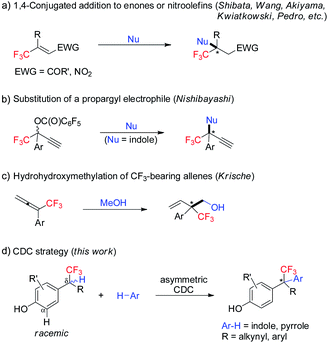 |
| Scheme 1 Overview of enantioselective access to acyclic CF3-substituted all-carbon quaternary stereocenters. | |
Enantioselective cross-dehydrogenative coupling (CDC) of two easily accessible C–H substrates represents a straightforward and economical approach in organic synthesis.8 Existing studies predominantly focused on C–H bonds adjacent to a heteroatom.9,10 But surprisingly, asymmetric CDC involving functionalization of acyclic benzylic C–H bonds has rarely been explored.11,12 Elegant works from the groups of Cozzi and Gong reported enantioselective CDC of 3-arylmethylindoles with aldehydes11a and malonates.11b In addition, CDC technology for enantioselective construction of all-carbon quaternary stereocenters has remained elusive.13 Recently, our group disclosed a chiral imidodiphosphoric acid catalyzed asymmetric CDC of 2,2-diarylacetonitriles with (hetero)arenes, furnishing triarylmethanes bearing all-carbon quaternary stereocenters with excellent enantioselectivity.13c Given the importance of optically active hetero-di- and hetero-triarylmethanes in chemistry, biology, material science, and medicine,14 we decided to explore the asymmetric CDC of racemic p-hydroxybenzyl CF3 moieties with heteroarenes for construction of these motifs containing CF3-substituted all-carbon quaternary stereocenters (Scheme 1d).
Three main challenges might obstruct the reaction design. First, regioselective oxidation of Cδ–H bond adjacent to strong electron-withdrawing CF3 group is difficult to achieve, which might be accompanied by competitive oxidation of Cα–H bond to 1,2-benzoquinones.15 Second, even if the expected oxidation proceeded smoothly, the CDC reaction might still be precluded by the potential incompatibility of electron-rich heteroarenes with strongly oxidative conditions. Third, the oxidized intermediate is expected to be highly unstable δ-CF3-substituted para-quinone methide (p-QM).16 Effective and enantioselective addition to highly congested CF3-substituted carbon of reactive p-QM intermediate under strongly oxidative conditions is substantially challenging.17–19
Results and discussion
Initially, chiral phosphoric acid catalyzed asymmetric CDC of p-hydroxybenzyl CF31a with indole 2a was selected as a model reaction for optimization (Table 1).20 To explore a suitable oxidation system, the reaction involving an initial oxidation of 1a followed by 3a catalyzed nucleophilic addition of 2a was conducted in a two-step, one-pot manner. As expected, efficient oxidation of 1a proved to be challenging. Common reagents for phenol oxidation, such as K3Fe(CN)6, (NH4)2S2O8, Ag2O, PhI(OAc)2, and MnO2, proved to be futile (entries 1 and 2, Table 1). Reaction with DDQ provided expected 4a in 10% yield with 26% ee, though the majority of 1a (83%) was recovered (entry 3, Table 1). Increasing the loading of DDQ did not improve oxidation conversion (entry 4, Table 1). We envisioned that oxidation of 1a with DDQ might be a reversible process, and adopting a DDQ-catalyzed oxidation system might be beneficial for breaking the equilibrium and driving the oxidation process. A screen of terminal oxidants towards metal oxides revealed that use of DDQ (25 mol%) as catalyst and MnO2 as stoichiometric oxidant furnished a complete and clean oxidation, and the CDC process afforded 4a in 70% yield with 55% ee (entries 5–7, Table 1).21 Reversal of the procedure by adding all the components prior to oxidation gave an inferior result (entry 8, Table 1). Optimization of chiral phosphoric acid catalysts identified 3c to be optimal (entries 7 and 9–12, Table 1). K2CO3 as a basic additive proved to be beneficial for improving the enantiocontrol (entry 13, Table 1). Increasing the loading of 2a was beneficial to the reaction by slowing down the nucleophilic addition process, and 4a was isolated in 86% yield with 93% ee (entry 14, Table 1).
Table 1 Optimization of the reaction conditionsa

|
Entry |
Oxidant |
Catalyst |
Yieldb (%) |
eec (%) |
Reaction conditions: 1a (0.1 mmol) and oxidant (0.12 mmol) in CH2Cl2 at 60 °C for 8 h, followed by 2a (0.1 mmol), 3 (5 mol%), 3 Å molecular sieves (20 mg) at −78 °C for 1 h.
Yield of isolated product.
Determined by chiral HPLC analysis.
K3Fe(CN)6, (NH4)2S2O8, Ag2O, and PhI(OAc)2 as oxidant.
2.0 equiv. of DDQ used.
25 mol% DDQ with 3.0 equiv. of terminal oxidant.
2a and 3a added before oxidation.
2.0 equiv. of K2CO3 as additive.
2a (0.3 mmol) used. n.d. = not determined.
|
1d |
Oxidant |
3a
|
<5 |
n.d. |
2 |
MnO2 |
3a
|
<5 |
n.d. |
3 |
DDQ |
3a
|
10 |
26 |
4e |
DDQ |
3a
|
12 |
21 |
5f |
DDQ/FeCl3 |
3a
|
<5 |
n.d. |
6f |
DDQ/Mn(OAc)3 |
3a
|
45 |
39 |
7f |
DDQ/MnO2 |
3a
|
70 |
55 |
8f,g |
DDQ/MnO2 |
3a
|
32 |
9 |
9f |
DDQ/MnO2 |
3b
|
<5 |
n.d. |
10f |
DDQ/MnO2 |
3c
|
78 |
85 |
11f |
DDQ/MnO2 |
3d
|
<5 |
n.d. |
12f |
DDQ/MnO2 |
3e
|
36 |
41 |
13f,h |
DDQ/MnO2 |
3c
|
83 |
88 |
14f,h,i |
DDQ/MnO2 |
3c
|
86 |
93 |
|
The scope of asymmetric CDC of δ-CF3-δ-alkynyl substituted 1 with 2a was investigated (Scheme 2). In general, substrates bearing a wide range of electronically varied aryl acetylenes with different substitution patterns were tolerated, affording respective hetero-diarylmethanes 4a–4g, 4j, and 4k in good yields with excellent ee. Polyarene naphthalene substituted acetylene 1h and thiophene substituted 1i proved to be competent coupling partners. δ-Alkyl acetylene-containing 1l–1p were also compatible with the asymmetric CDC process, furnishing corresponding 4l–4p in 66–73% yields with up to 93% ee. The process exhibited a good functional group tolerance, with common functionalities like halides (4d and 4e), primary alcohol (4n), propargyl chloride (4o), and silyl group (4p) well tolerated for further manipulation.
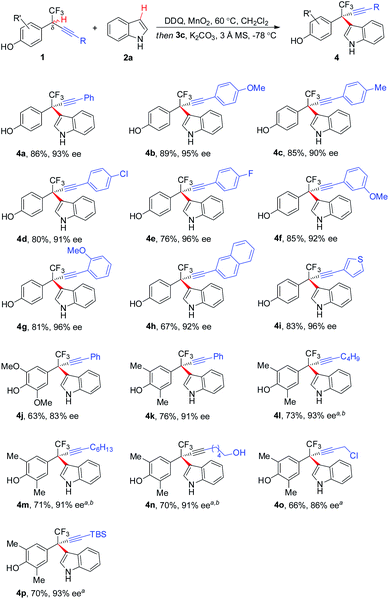 |
| Scheme 2 Scope of δ-alkynyl-substituted substrates for CF3-containing hetero-diarylmethanes. aReaction with 3b (5 mol%) and 2a (1.1 equiv.) without K2CO3 additive. bAsymmetric nucleophilic addition of 2a was performed at 0 °C. | |
Asymmetric CDC of a variety of δ-aryl substituted 5 with 2a proceeded smoothly, affording respective CF3-bearing hetero-triarylmethanes 6a–6f with 90–97% ee (Scheme 3). To our knowledge, this is the first example of direct and asymmetric construction of CF3-substituted tri-arylmethanes.5
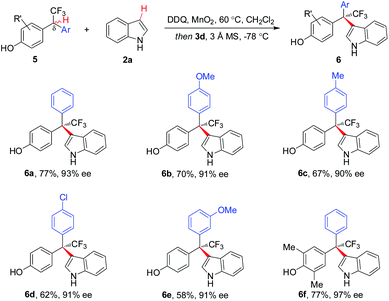 |
| Scheme 3 Scope of δ-aryl-substituted substrates for CF3-containing hetero-triarylmethanes. aReaction with 2a (1.1 equiv.). | |
The substituent effect of indoles was next evaluated (Scheme 4). A broad range of indoles 2 bearing either electron-donating or -withdrawing groups at different positions (C4, C5, C6, and C7) on aryl rings participated in the CDC process, furnishing corresponding 7a–7h in 75–88% yields with 90–96% ee. Additionally, C2-substituted indoles proved to be competent components, as demonstrated by the generation of 7i in 70% yield with 92% ee. Besides indole moieties, 2-substituted pyrroles were also identified to be suitable coupling partners in asymmetric CDC reaction, as illustrated by the formation of 9 in 65% yield with 93% ee (Scheme 5). While the scope of 2-substituted pyrroles was not exclusively explored, the result afforded a proof-of-concept for the modularity of the method for asymmetric preparation of diversely functionalized hetero-diarylmethanes bearing CF3-substituted all-carbon quaternary stereocenters.
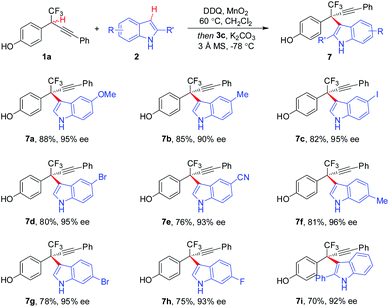 |
| Scheme 4 Scope of the indole components. | |
 |
| Scheme 5 Asymmetric CDC with 2-substituted pyrrole. a1.0 equiv. of 8 used. | |
The generality of the CDC approach is further demonstrated by enantioselective construction of all-carbon quaternary stereocenters bearing other polyfluoroalkyl or perfluoroalkyl groups, such as CF2Cl (11a), C2F5 (11b), and C3F7 (11c) (Scheme 6).
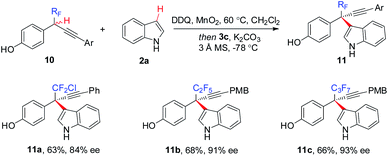 |
| Scheme 6 Enantioselective access to other polyfluoroalkyl-bearing all-carbon quaternary stereocenters. | |
The synthetic utilities of the method were next examined (Scheme 7). The phenolic hydroxyl group in 4a was removed through triflation followed by hydrogenation affording 12 in 86% yield (Scheme 7a). Phenol 4a can also undergo triflation followed by palladium-catalyzed cross-coupling reaction, furnishing biaryl 13 efficiently (Scheme 7b). Notably, the ee values of the products remain highly reserved in these processes.
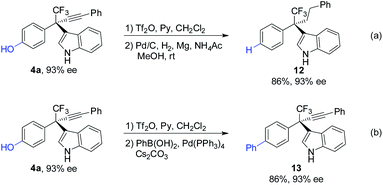 |
| Scheme 7 Representative product transformations. | |
Control experiments were performed to gain further insights into the mechanism (Scheme 8). Upon completion of oxidation of 1a, δ-CF3-δ-alkynyl-substituted p-QM 14 was isolated in 11% yield (Scheme 8a). The low yield might be ascribed to the poor stability of δ-CF3-substituted p-QM compound. Subjecting 14 to standard CDC conditions in the absence of oxidation elements furnished 4a with comparable yield and ee to those observed in the one-pot process, thus indicating the intermediacy of p-QM 14 (Scheme 8b). No invertible reaction was observed for 14 and 2,3-dichloro-4,5-dicyanohydroquinone (DDQH2), the reduction product of DDQ (Scheme 8c). The asymmetric 1,6-conjugate addition to 14 was not influenced by introducing stoichiometric amount of acidic DDQH2 (Scheme 8d). K2CO3 was found to be beneficial for improving the enantiocontrol (entry 13, Table 1). Accordingly, chiral potassium-organophosphate 3f was prepared in situ for real catalyst identification (Scheme 8e). No enantioselective catalytic reactivity was observed for 3f, implying that 3c but not 3f should be the real catalyst, and the hydroxyl group in chiral phosphoric acid is requisite. No reaction was observed for p-methoxybenzyl CF315, indicating the significance of the hydroxyl moiety in the in situ formation of p-QM intermediate (Scheme 8f). According to the above experiments, a plausible mechanism was recommended (Scheme 8g). Racemic p-hydroxybenzyl CF3 moiety 1a might be oxidized by catalytic amount of DDQ, giving p-QM 14 together with the generation of DDQH2. Stoichiometric MnO2 as terminal oxidant proved to be crucial to the complete oxidation of 1a to 14 by converting DDQH2 to DDQ for the catalytic cycle.21 Chiral phosphoric acid 3c catalyzed asymmetric 1,6-conjugate addition of indole 2a to 14 yielding expected 4a.22 Asymmetric CDC of 1a with N-methyl protected indole 19 provided inferior ee to unprotected 2a, implying that the N–H moiety might act as a hydrogen bond donor (Scheme 8h). A plausible transition state was proposed in Scheme 8i, in which chiral phosphoric acid acts as a bifunctional role for activation of both coupling partners and remote stereocontrol by hydrogen bonding. p-QM intermediates can be generated in situ through chiral phosphoric acid catalyzed dehydration of p-hydroxybenzyl alcohols.17 Accordingly, δ-CF3-substituted p-hydroxybenzyl alcohol 21 was subjected to the CDC condition without oxidation elements (Scheme 8j). However, no reaction was observed even at an elevated temperature, thus demonstrating the uniqueness of the oxidation strategy in generating unstable δ-CF3-substituted p-QM intermediates.
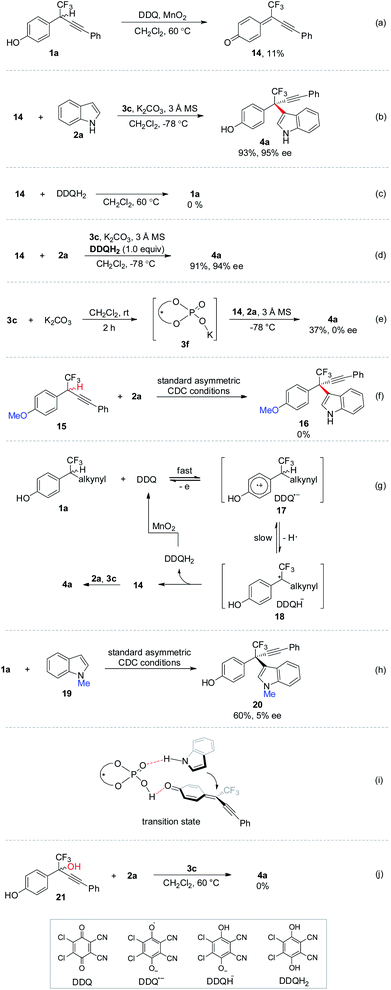 |
| Scheme 8 Control experiments and the proposed mechanism. | |
Conclusions
In summary, CDC strategy for enantioselective construction of CF3-substituted all-carbon quaternary stereocenters has been established for the first time. By using catalytic DDQ with MnO2 as an inexpensive terminal oxidant, asymmetric cross-coupling of racemic p-hydroxybenzyl CF3 moieties with indoles and pyrroles proceeded smoothly, providing acyclic CF3-bearing all-carbon quaternary centers with excellent chemo- and enantioselectivity. The generality of the strategy is further demonstrated by efficient formation of all-carbon quaternary centers bearing other polyfluoroalkyl and perfluoroalkyl groups such as CF2Cl, C2F5, and C3F7. We envisioned that the strategically different approach described here will provide an attractive platform for enantioselective access to all-carbon quaternary stereocenters bearing diverse perfluoroalkyl groups that are otherwise difficult to be prepared by existing methods.
Conflicts of interest
There are no conflicts to declare.
Acknowledgements
We gratefully acknowledge the National Science Foundation of China (21722204 and 21971148), Fok Ying Tung Education Foundation (151035), and Youth Interdiscipline Innovative Research Group of Shandong University (2020QNQT009).
Notes and references
-
(a)
Fluorine in Pharmaceutical and Medicinal Chemistry: From Biophysical Aspects to Clinical Applications, ed. V. Gouverneur and K. Müller, Imperial College Press, London, 2012 Search PubMed;
(b)
Fluorine in Medicinal Chemistry and Chemical Biology, ed. I. Ojima, Wiley, Chichester, U.K., 2009 Search PubMed;
(c) K. Müller, C. Faeh and F. Diederich, Science, 2007, 317, 1881 CrossRef PubMed;
(d) W. K. Hagmann, J. Med. Chem., 2008, 51, 4359 CrossRef CAS PubMed.
- For selected reviews, see:
(a) K. Mikami, Y. Itoh and M. Yamanaka, Chem. Rev., 2004, 104, 1 CrossRef CAS PubMed;
(b) N. Shibata, S. Mizuta and H. Kawai, Tetrahedron: Asymmetry, 2008, 19, 2633 CrossRef CAS;
(c) J. Nie, H.-C. Guo, D. Gahard and J.-A. Ma, Chem. Rev., 2011, 111, 455 CrossRef CAS PubMed;
(d) T. Liang, C. N. Neumann and T. Ritter, Angew. Chem., Int. Ed., 2013, 52, 8214 CrossRef CAS;
(e) X. Yang, T. Wu, R. J. Phipps and F. D. Toste, Chem. Rev., 2015, 115, 826 CrossRef CAS.
- For selected reviews of catalytic asymmetric construction of all-carbon quaternary stereocenters, see:
(a) K. Fuji, Chem. Rev., 1983, 93, 2037 CrossRef;
(b) E. J. Corey and A. Guzman-Perez, Angew. Chem., Int. Ed., 1998, 37, 388 CrossRef;
(c) I. Denissova and L. Barriault, Tetrahedron, 2003, 59, 10105 CrossRef CAS;
(d) B. M. Trost and C. Jiang, Synthesis, 2006, 369 CrossRef CAS;
(e) M. Bella and T. Gasperi, Synthesis, 2009, 2009, 1583 CrossRef;
(f) M. Shimizu, Angew. Chem., Int. Ed., 2011, 50, 5998 CrossRef CAS PubMed;
(g) J. Christoffers and A. Mann, Angew. Chem., Int. Ed., 2001, 40, 4591 CrossRef CAS;
(h) I. Marek, Y. Minko, M. Pasco, T. Mejuch, N. Gilboa, H. Chechik and J. P. Das, J. Am. Chem. Soc., 2014, 136, 2682 CrossRef CAS PubMed;
(i) K. W. Quasdorf and L. E. Overman, Nature, 2014, 516, 181 CrossRef CAS PubMed;
(j) Y. Liu, S.-J. Han, W.-B. Liu and B. M. Stoltz, Acc. Chem. Res., 2015, 48, 740 CrossRef CAS PubMed;
(k) X.-P. Zeng, Z.-Y. Cao, Y.-H. Wang, F. Zhou and J. Zhou, Chem. Rev., 2016, 116, 7330 CrossRef CAS PubMed.
- For asymmetric 1,4-conjugate addition to β,β-disubstituted CF3-enones or nitroolefins, see:
(a) H. Kawai, S. Okusu, E. Tokunaga, H. Sato, M. Shiro and N. Shibata, Angew. Chem., Int. Ed., 2012, 51, 4959 CrossRef CAS PubMed;
(b) H. Kawai, Z. Yuan, T. Kitayama, E. Tokunaga and N. Shibata, Angew. Chem., Int. Ed., 2013, 52, 5575 CrossRef CAS PubMed;
(c) Q. Chen, G. Wang, X. Jiang, Z. Xu, L. Lin and R. Wang, Org. Lett., 2014, 16, 1394 CrossRef CAS PubMed;
(d) P. Kwiatkowski, A. Cholewiak and A. Kasztelan, Org. Lett., 2014, 16, 5930 CrossRef CAS;
(e) A. Sanz-Marco, G. Blay, C. Vila and J. R. Pedro, Org. Lett., 2016, 18, 3538 CrossRef CAS;
(f) I. Ibαñez, M. Kaneko, Y. Kamei, R. Tsutsumi, M. Yamanaka and T. Akiyama, ACS Catal., 2019, 9, 6903 CrossRef.
- K. Tsuchida, Y. Senda, K. Nakajima and Y. Nishibayashi, Angew. Chem., Int. Ed., 2016, 55, 9728 CrossRef CAS PubMed.
- M. Holmes, K. D. Nguyen, L. A. Schwartz, T. Luong and M. J. Krische, J. Am. Chem. Soc., 2017, 139, 8114 CrossRef CAS PubMed.
- For enantioselective construction of cyclic CF3-containing all-carbon quaternary centers, see:
(a) J. R. Denton, D. Sukumaran and H. M. L. Davies, Org. Lett., 2007, 9, 2625 CrossRef CAS;
(b) Q.-H. Deng, H. Wadepohl and L. H. Gade, J. Am. Chem. Soc., 2012, 134, 10769 CrossRef CAS;
(c) L. Woźniak, J. J. Murphy and P. Melchiorre, J. Am. Chem. Soc., 2015, 137, 5678 CrossRef PubMed;
(d) Z.-M. Zhang, B. Xu, S. Xu, H.-H. Wu and J. Zhang, Angew. Chem., Int. Ed., 2016, 55, 6324 CrossRef CAS PubMed;
(e) R. Calvo, A. Comas-Vives, A. Togni and D. Katayev, Angew. Chem., Int. Ed., 2019, 58, 1447 CrossRef CAS PubMed;
(f) A. Saito, N. Kumagai and M. Shibasaki, Angew. Chem., Int. Ed., 2017, 56, 5551 CrossRef CAS;
(g) J. Zhang, H.-H. Wu and J. Zhang, Org. Lett., 2017, 19, 6080 CrossRef CAS;
(h) D. A. Nagib, M. E. Scott and D. W. C. MacMillan, J. Am. Chem. Soc., 2009, 131, 10875 CrossRef CAS PubMed;
(i) L. Yin, L. Brewitz, N. Kumagai and M. Shibasaki, J. Am. Chem. Soc., 2014, 136, 17958 CrossRef CAS PubMed.
- For reviews on CDC reactions, see:
(a) C.-J. Li, Acc. Chem. Res., 2009, 42, 335 CrossRef CAS PubMed;
(b) C. S. Yeung and V. M. Dong, Chem. Rev., 2011, 111, 1215 CrossRef CAS PubMed;
(c) C. Liu, H. Zhang, W. Shi and A. Lei, Chem. Rev., 2011, 111, 1780 CrossRef CAS;
(d) C. Zheng and S.-L. You, RSC Adv., 2014, 4, 6173 RSC;
(e) Y. Qin, J. Lv and S. Luo, Tetrahedron Lett., 2014, 55, 551 CrossRef CAS;
(f) L. Yang and H. Huang, Catal. Sci. Technol., 2012, 2, 1099 RSC.
- For C–H bond adjacent to nitrogen, see:
(a) Z. Li and C.-J. Li, Org. Lett., 2004, 6, 4997 CrossRef CAS PubMed;
(b) J. Zhang, B. Tiwari, C. Xing, X. Chen and Y. R. Chi, Angew. Chem., Int. Ed., 2012, 51, 3649 CrossRef CAS PubMed;
(c) D. A. DiRocco and T. Rovis, J. Am. Chem. Soc., 2012, 134, 8094 CrossRef CAS PubMed;
(d) G. Zhang, Y. Ma, S. Wang, Y. Zhang and R. Wang, J. Am. Chem. Soc., 2012, 134, 12334 CrossRef CAS PubMed;
(e) Y. Tan, W. Yuan, L. Gong and E. Meggers, Angew. Chem., Int. Ed., 2015, 54, 13045 CrossRef CAS PubMed;
(f) G. Wei, C. Zhang, F. Bureš, X. Ye, C.-H. Tan and Z. Jiang, ACS Catal., 2016, 6, 3708 CrossRef CAS;
(g) Q. Yang, L. Zhang, C. Ye, S. Luo, L.-Z. Wu and C.-H. Tung, Angew. Chem., Int. Ed., 2017, 56, 3694 CrossRef CAS PubMed.
- For C–H bond adjacent to oxygen, see:
(a) Z. Meng, S. Sun, H. Yuan, H. Lou and L. Liu, Angew. Chem., Int. Ed., 2014, 53, 543 CrossRef CAS PubMed;
(b) A. Lee, R. C. Betori, E. A. Crane and K. A. Scheidt, J. Am. Chem. Soc., 2018, 140, 6212 CrossRef CAS PubMed;
(c) G. Wang, X. Xin, Z. Wang, G. Lu, Y. Ma and L. Liu, Nat. Commun., 2019, 10, 559 CrossRef PubMed.
- Two isolated examples on asymmetric CDC of acyclic benzylic C–H bond, see:
(a) F. Benfatti, M. Guiteras Capdevila, L. Zoli, E. Benedetto and P. G. Cozzi, Chem. Commun., 2009, 45, 5919 RSC;
(b) C. Guo, J. Song, S. W. Luo and L. Z. Gong, Angew. Chem., Int. Ed., 2010, 49, 5558 CrossRef CAS.
- For asymmetric CDC of C–H bond in xanthenes, see:
(a) B. Zhang, S.-K. Xiang, L.-H. Zhang, Y. Cui and N. Jiao, Org. Lett., 2011, 13, 5212 CrossRef CAS PubMed;
(b) E. Larionov, M. M. Mastandrea and M. A. Pericàs, ACS Catal., 2017, 7, 7008 CrossRef CAS; for asymmetric cyanation and arylation of benzylic and allylic C–H bonds, see:
(c) W. Zhang, F. Wang, S. D. McCann, D. Wang, P. Chen, S. S. Stahl and G. Liu, Science, 2016, 353, 1014 CrossRef CAS;
(d) J. Li, Z. Zhang, L. Wu, W. Zhang, P. Chen, Z. Lin and G. Liu, Nature, 2019, 574, 516 CrossRef CAS;
(e) W. Zhang, L. Wu, P. Chen and G. Liu, Angew. Chem., Int. Ed., 2019, 58, 6425 CrossRef CAS; for benzylic C–H trifluoromethylation, see:
(f) H. Xiao, Z. Liu, H. Shen, B. Zhang, L. Zhu and C. Li, Chem, 2019, 5, 940 CrossRef CAS.
- Two isolated examples on asymmetric formation of all-carbon quaternary stereocenters via CDC strategy, see:
(a) H. Wu, Y.-P. He, L. Xu, D.-Y. Zhang and L.-Z. Gong, Angew. Chem., Int. Ed., 2014, 53, 12218 CrossRef PubMed;
(b) W. Cao, X. Liu, R. Peng, P. He, L. Lin and X. Feng, Chem. Commun., 2013, 49, 3470 RSC;
(c)
Z. Wang, Y. Zhu, X. Pan, G. Wang, L. Liu, Angew. Chem., Int. Ed., DOI:10.1002/anie.201912739.
-
(a) D. F. Duxbury, Chem. Rev., 1993, 93, 381 CrossRef CAS;
(b) V. Nair, S. Thomas, S. C. Mathew and K. G. Abhilash, Tetrahedron, 2006, 62, 6731 CrossRef CAS;
(c) M. Shiri, M. A. Zolfigol, H. G. Kruger and Z. Tanbakouchian, Chem. Rev., 2010, 110, 2250 CrossRef CAS;
(d) S. Mondal and G. Panda, RSC Adv., 2014, 4, 28317 RSC;
(e) M. Nambo and C. M. Crudden, ACS Catal., 2015, 5, 4734 CrossRef CAS.
-
(a) M. O. Ratnikov, L. E. Farkas and M. P. Doyle, J. Org. Chem., 2012, 77, 10294 CrossRef CAS PubMed;
(b) K. V. N. Esguerra, Y. Fall and J.-P. Lumb, Angew. Chem., Int. Ed., 2014, 53, 5877 CrossRef CAS PubMed.
- δ-CF3-substituted p-QMs without two α-alkyl substituents are highly unstable species, which might readily undergo polymerization and other undesired transformations, see:
(a) J. J. Murray, J. Org. Chem., 1968, 33, 3306 CrossRef CAS;
(b) J. A. Hyatt, J. Org. Chem., 1983, 48, 129 CrossRef CAS.
- One isolated report on asymmetric formation of all-carbon quaternary centers via 1,6-conjugate addition to in situ generated p-QMs, see: Z. Wang, Y. F. Wong and J. Sun, Angew. Chem., Int. Ed., 2015, 54, 13711 CrossRef CAS.
- Asymmetric 1,6-conjugate addition of p-QMs for tertiary stereocenter formation (C–C bond forging process), see:
(a) W.-D. Chu, L.-F. Zhang, X. Bao, X.-H. Zhao, C. Zeng, J.-Y. Du, G.-B. Zhang, F.-X. Wang, X.-Y. Ma and C.-A. Fan, Angew. Chem., Int. Ed., 2013, 52, 9229 CrossRef CAS;
(b) L. Caruana, F. Kniep, T. K. Johansen, P. H. Poulsen and K. A. Jørgensen, J. Am. Chem. Soc., 2014, 136, 15929 CrossRef CAS PubMed;
(c) Y. Lou, P. Cao, T. Jia, Y. Zhang, M. Wang and J. Liao, Angew. Chem., Int. Ed., 2015, 54, 12134 CrossRef CAS;
(d) K. Zhao, Y. Zhi, T. Shu, A. Valkonen, K. Rissanen and D. Enders, Angew. Chem., Int. Ed., 2016, 55, 12104 CrossRef CAS;
(e) S. Li, Y. Liu, B. Huang, T. Zhou, H. Tao, Y. Xiao, L. Liu and J. Zhang, ACS Catal., 2017, 7, 2805 CrossRef CAS;
(f) M. J. Smith, K. D. Reichl, R. A. Escobar, J. T. Heavey, D. F. Coker, S. E. Schaus and J. A. Porco Jr, J. Am. Chem. Soc., 2019, 141, 148 CrossRef CAS.
- Asymmetric reduction of p-QMs for tertiary stereocenter formation, see:
(a) M. Chen and J. Sun, Angew. Chem., Int. Ed., 2017, 56, 11966 CrossRef CAS;
(b) Z. Wang, F. Ai, Z. Wang, W. Zhao, G. Zhu, Z. Lin and J. Sun, J. Am. Chem. Soc., 2015, 137, 383 CrossRef CAS.
-
(a) T. Akiyama, Chem. Rev., 2007, 107, 5744 CrossRef CAS PubMed;
(b) M. Terada, Synthesis, 2010, 42, 1929 CrossRef;
(c) D. Parmar, E. Sugiono, S. Raja and M. Rueping, Chem. Rev., 2014, 114, 9047 CrossRef CAS PubMed.
- L. Liu and P. E. Floreancig, Org. Lett., 2010, 12, 4686 CrossRef CAS PubMed.
- Reviews on asymmetric 1,6-conjugate addition, see:
(a) A. G. Csákÿ, G. de La Herrán and M. C. Murcia, Chem. Soc. Rev., 2010, 39, 4080 RSC;
(b) A. T. Biju, ChemCatChem, 2011, 3, 1847 CrossRef CAS;
(c) E. M. P. Silva and A. M. S. Silva, Synthesis, 2012, 3109 CrossRef CAS.
Footnotes |
† Electronic supplementary information (ESI) available. See DOI: 10.1039/c9sc05894j |
‡ These authors contributed equally. |
|
This journal is © The Royal Society of Chemistry 2020 |
Click here to see how this site uses Cookies. View our privacy policy here.