DOI:
10.1039/D0RA07988J
(Paper)
RSC Adv., 2020,
10, 37369-37373
A study on the self-assembly mode and supramolecular framework of complexes of cucurbit[6]urils and 1-(4-methoxyphenyl)piperazine†
Received
18th September 2020
, Accepted 29th September 2020
First published on 8th October 2020
Abstract
Self-assembly between symmetrical dicyclohexyl-substituted cucurbit[6]uril (abbreviated as (CyH)2Q[6]) and cyclopentanocucurbit[6]uril (CyP6Q[6]) as hosts and 1-(4-methoxyphenyl)piperazine (MeOPP) as a guest molecule has been studied by means of single-crystal X-ray diffraction analysis, NMR, MALDI-TOF mass spectrometry, and other characterization methods. The experimental results showed that the self-assembly was driven by the formation of exclusion complexes by the cucurbit[n]uril and the guest, that is, supramolecular interaction between the negative charge of the cucurbit[n]uril portals and a coordination polymer guest. Complexes were formed between the positive charge of the cucurbit[n]uril outer wall and inorganic anions, thus generating self-assemblies with multi-dimensional and multi-level supramolecular frameworks.
1. Introduction
Porous materials, such as inorganic zeolites,1 metal–organic frameworks (MOFs),2,3 and covalent organic frameworks (COFs),4,5 have been widely used in heterogeneous catalysis, adsorption, and ion-exchange processes, etc.6–9 In this context, cucurbit[n]urils10–16 are expected to become basic construction units. According to studies of the electrical properties of the surface structure of cucurbit[n]urils, the following statements can be made17 (Scheme 1). (1) The regions around the carbonyl units of the portals bear a significant negative potential, which makes cucurbit[n]urils organic ligand molecules with multiple binding sites. Cucurbit[n]urils with different degrees of polymerization have portals of different sizes, which can provide coordination environments with different structural characteristics, giving rise to diverse complexes and supramolecular assemblies. This constitutes the coordination chemistry of cucurbit[n]urils.18–21 (2) The inner cavities of cucurbit[n]urils are electrically neutral. Cucurbit[n]urils with different degrees of polymerization also have cavities of different sizes, which can accommodate guest molecules of different sizes. This constitutes the host–guest chemistry of cucurbit[n]urils.22–24 (3) The outer surfaces of the cucurbit[n]urils bear positive electrostatic potentials. This has given rise a new research field25,26 based on interactions between the outer surface of Q[n]s (OSIQ) and electronegative species.
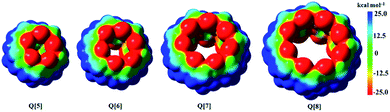 |
| Scheme 1 Electrostatic potential maps (ESPs) for Q[5], Q[6], Q[7], and Q[8], respectively. ESPs are mapped on electron density isosurfaces (0.001 e au−3) for cucurbit[n]urils at the B3LYP/6-311G (d, p) level of theory with Gaussian09. | |
For the present study, we selected 1-(4-methoxyphenyl)piperazine (MeOPP), an antihypertensive intermediate of phenylpiperazine, as the guest. As hosts, we selected cucurbit[n]urils substituted with cyclopentyl27 or cyclohexyl groups,28 because these modified cucurbit[n]urils (Scheme 2) have better water solubility than unsubstituted cucurbit[n]urils.
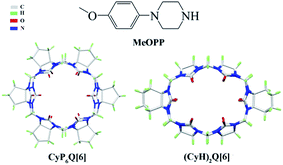 |
| Scheme 2 CyP6Q[6], (CyH)2Q[6], and the guest molecule used in this study. | |
Single crystal X-ray diffraction analysis, nuclear magnetic resonance (NMR), matrix-assisted laser desorption ionization time-of-flight mass spectrometry (MALDI-TOF), and other characterization methods have been used to study supramolecular self-assembly between the guest molecule and the two cucurbit[n]uril hosts. The results showed that the guest and the respective cucurbit[n]uril hosts self-assembled through the formation of exclusion complexes, specifically supramolecular interaction between the negative charge of the cucurbit[n]uril portal and a coordination polymer guest. Moreover, complexes formed between the positive charge of the outer wall of cucurbit[n]uril and the inorganic anions generated self-assemblies with multi-dimensional and multi-level supramolecular frameworks.
2. Experimental
2.1. Experimental equipment and reagents
Symmetrical dicyclohexyl-substituted cucurbit[6]uril ((CyH)2Q[6])28 and cyclopentanocucurbit[6]uril ((CyP)6Q[6])27 were prepared and purified in accordance to a literature method. All reagents were obtained in analytically pure form. Diffraction data for the complexes were collected at 273.15 K on a Bruker D8 VENTURE diffractometer or a Bruker SMART Apex-II CCD diffractometer. NMR spectra were recorded on a Varian INOVA 400M spectrometer. MALDI-TOF mass spectrometry was performed on an Agilent 6545 Q-TOF mass spectrometer.
2.2. Synthesis of the complexes
The (CyH)2Q[6] (15 mg, 14.6 mmol) was placed in a beaker and taken up in 6 M hydrochloric acid (10 mL). MeOPP (15.4 mmol) was taken up in distilled water (3 mL), which was heated to aid dissolution. The latter solution was then poured into the former. Finally, a small amount of the inducing agent CdCl2 was added, and the solution was heated and stirred for 10 min in a water bath at 30 °C, cooled to room temperature, and allowed to stand. After 3 weeks, colorless single crystals suitable for crystal structure determination had precipitated (43% yield). Complex 2 was synthesized in a similar manner as complex 1 in a yield of 39%.
2.3. Crystal measurement
A measurable (transparent and crack-free) crystal of the complex of appropriate size was selected, fixed on a glass fiber with vaseline, and introduced into the Bruker Smart Apex II single-crystal X-ray diffractometer to collect diffraction data. An Mo target was adopted; excitation voltage 20 kV; wavelength (λ(Mo-Kα)) 0.71073 Å. The SHELXT-14 program was used for structure analysis, and the SHELXL-14 program was used for data refinement by a full-matrix least-squares method. The SQUEEZE routine of PLATON was used to remove some solvent molecules from the crystal. CCDC-2021200 (1) and CCDC-2021162 (2) contain the crystal data for this paper. The main crystal structure parameters are shown in Table 1.
Table 1 Crystallographic data for the complexes
|
1 |
2 |
Conventional R on Fhkl: ∑‖Fo| − |Fc‖/∑|Fo|. Weighted R on |Fhkl|2: ∑[w(Fo2 − Fc2)2]/∑[w(Fo2)2]1/2. |
Empirical formula |
C55H64N26O13 |
C65H76N26O13 |
Formula weight |
1297.286 |
1429.492 |
Crystal system |
Triclinic |
Monoclinic |
Space group |
P![[1 with combining macron]](https://www.rsc.org/images/entities/char_0031_0304.gif) |
C12/c1 |
a [Å] |
14.944(3) |
29.984(8) |
b [Å] |
15.743(4) |
13.001(2) |
c [Å] |
19.200(4) |
25.823(7) |
α [°] |
90.039(8) |
90 |
β [°] |
90.099(8) |
111.150(14) |
γ [°] |
112.980(8) |
90 |
V [Å3] |
4158.6(15) |
9388(4) |
Z |
1 |
1 |
Dcalcd [g cm−3] |
2.820 |
0.034 |
T [K] |
273.15 |
273.15 |
μ [mm−1] |
5.269 |
0.063 |
Parameters |
1065 |
622 |
Rint |
0.0552 |
0.0699 |
R [I > 2σ(I)]a |
0.0538 |
0.0461 |
wR [I > 2σ(I)]b |
0.1498 |
0.1112 |
R (all data) |
0.0775 |
0.0755 |
wR (all data) |
0.1686 |
0.1291 |
GOF on F2 |
1.028 |
1.040 |
2.4. Determination by 1H NMR
A 2.0–2.5 × 10−3 mmol L−1 solution of the guest was prepared in D2O. It was then added dropwise to a solution of the cucurbituril (0.5–0.7 g) in D2O. The spectral changes were monitored on a Varian Inova 400M NMR spectrometer at 20 °C.
2.5. Determination by mass spectrometry
The sample was prepared as a 10 μg mL−1 solution in doubly-distilled water, which was filtered into a chromatographic sample bottle through an aqueous 0.22 μm filter membrane. Mass spectra were recorded on an Agilent 6545 Q-TOF mass spectrometer.
3. Results and discussion
3.1. Description of the crystal structures of complexes 1 and 2
3.1.1. Description of the crystal structure of complex 1. Fig. 1(f) shows the supramolecular self-assembly formed from (CyH)2Q[6], MeOPP, and [CdCl4]2−. In this self-assembly, the carbonyl oxygen atoms at the (CyH)2Q[6] portal interacted with the MeOPP molecule through hydrogen-bonding and ion–dipole interactions. Specifically, the proton (H26A) on the nitrogen of the MeOPP molecule interacted with the carbonyl oxygen atoms (O3, O4) at the (CyH)2Q[6] portal through ion–dipole interactions. The carbonyl oxygen atoms (C
O) at the portal of (CyH)2Q[6] were connected with the heterocycle of the MeOPP molecule through hydrogen-bonding with distances in the range 2.165–2.687 Å. There was a weak π⋯π interaction between the carbonyl oxygen atom of the (CyH)2Q[6] portal and the benzene ring of MeOPP (2.333 Å) [Fig. 1(a)]. Two adjacent MeOPP molecules formed an angle of 34.51° with the plane of the benzene ring, and were connected by a hydrogen-bonding dislocation interaction (2.477 Å) [Fig. 1(b)]. Two adjacent (CyH)2Q[6] molecules formed an angle of 58.94° with the plane of the terminal carbonyl group, which were connected by a dipole–dipole dislocation interaction [Fig. 1(c)]. The [CdCl4]2− anion interacted with the MeOPP molecule through ion–dipole interactions [Fig. 1(d and e)].
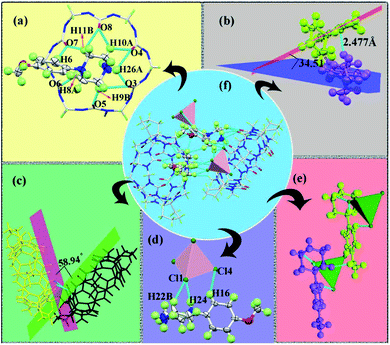 |
| Fig. 1 (a) Crystal diagram of complex 1; (b) the interaction of adjacent guest molecules; (c) the interaction of adjacent (CyH)2Q[6] molecules; (d and e) the interaction of MeOPP molecules with [CdCl4]2−; (f) supramolecular self-assembly of complex 1. | |
Fig. 2(b) shows the stacking diagram of complex 1. The supramolecular framework of (CyH)2Q[6]@MeOPP was formed by dipole–dipole interactions between molecules of (CyH)2Q[6] and weak interactions between the cucurbituril and the guest, assisted by surrounding [CdCl4]2− anions. In the c-direction, the adjacent cucurbituril molecules were arranged in a V-shape with MeOPP molecules through OSIQ induced by the anions [Fig. 2(a)]. Two-dimensional surfaces were formed between layers through self-induction and anion induction [Fig. 1(c)], whereby MeOPP molecules were connected by hydrogen bonds and coordinate with surrounding [CdCl4]2− anions through weak interactions [Fig. 2(d)]. A cucurbituril molecule interacted with four [CdCl4]2− anions through anion-induced OSIQ [Fig. 2(e)], while the cucurbituril portal and outer wall were connected to six guest molecules through weak interaction, among which four guest molecules interacted with the cucurbituril portals and the other two guest molecules were connected to methylene (–CH2) units on outer wall of the cucurbituril molecule through hydrogen-bonding [Fig. 2(f)].
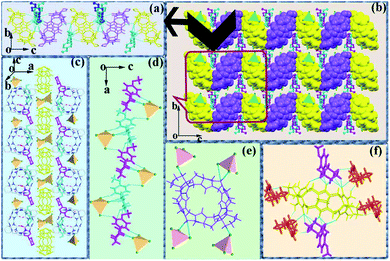 |
| Fig. 2 Crystal structure of complex 1: (a) 1D chain structure of the assembly 1. Hydrogen atoms are omitted for clarity. (b) Overview of supramolecular assembly in complex 1; (c) interaction between layers; (d) interaction between moieties; (e) Representative anion-induced OSIQ between a (CyH)2Q[6] molecule and neighbouring anions. Interaction between (CyH)2Q[6] and neighboring [CdCl4]2− anions; (f) interaction between (CyH)2Q[6] and the surrounding MeOPP molecules. | |
3.1.2. Description of the crystal structure of complex 2. Fig. 3(b) shows the supramolecular self-assembly formed by CyP6Q[6], MeOPP, and [CdCl4]2−. In this self-assembly, the interaction mode of the guest molecule was similar to that in complex 1. The protons (H1, H2A, H2B) on the N atoms of the MeOPP molecule and the carbonyl oxygen atoms (O4, O10, O11) at the cucurbituril portal were engaged in ion–dipole interactions (1.962–2.552 Å). The carbonyl oxygen atoms (C
O) at the portal of CyP6Q[6] were connected with the heterocycle of MeOPP by hydrogen-bonding, with distances in the range 2.164–2.522 Å. There was also a weak π⋯π interaction (2.632 Å) between a carbonyl oxygen atom at the portal of CyP6Q[6] and the benzene ring of MeOPP [Fig. 3(a and c)].
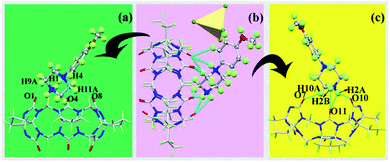 |
| Fig. 3 Crystal diagram of complex 2: (a and c) interactions between CyP6Q[6] and MeOPP; (b) supramolecular self-assembly of complex 2. | |
It can be observed from Fig. 4(a) that one cucurbituril molecule interacted with six [CdCl4]2− anions through anion-induced OSIQ. This was different from the situation in complex 1 in that [CdCl4]2− anions were engaged in ion–dipole interactions with bridging “waist” methylene (–CH2) units of CyP6Q[6]. A CyP6Q[6] molecule was surrounded by four MeOPP molecules through noncovalent bonds, whereby two guest molecules interacted with the cucurbituril portal through weak interactions, and the other two guest molecules interacted with the “waist” methylene (–CH2) units of the bridging CyP6Q[6] molecule through hydrogen bonds [Fig. 4(b)]. There was hydrogen-bonding between the carbonyl oxygen atoms at the portal of one cucurbituril molecule and the methylene (–CH2) units of another adjacent cucurbituril [Fig. 4(c)]. Fig. 4(d) shows the general situation of supramolecular assembly in complex 2, in which [CdCl4]2− anions surrounded each CyP6Q[6] molecule to form a hexagonal honeycomb framework [Fig. 4(e)]. Each unit in the framework was filled with coordination polymer based on CyP6Q[6]@MeOPP [Fig. 4(f)], and the cucurbituril was surrounded by guest molecules through weak interactions, thus forming a two-dimensional surface. Salient bond lengths in the complexes are shown in Table 2.
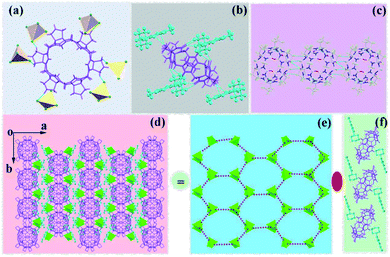 |
| Fig. 4 Crystal structure of complex 2: (a) representative anion-induced OSIQ between a CyP6Q[6] molecule and neighboring anions; (b) interactions between CyP6Q[6] and surrounding MeOPP molecules; (c) interaction between melon rings; (d) overview of supramolecular assembly in complex 2; (e) [CdCl4]2− hexagonal honeycomb framework; (f) 1D chain structure of the assembly 2. Hydrogen atoms are omitted for clarity. | |
Table 2 Partial bond length data for complexes 1 and 2
Complexes |
Bond |
Bond length/Å |
1 |
H9B–O3 |
2.165 |
H9B–O5 |
2.687 |
H8A–O6 |
2.382 |
H6–O7 |
2.333 |
H11B–O8 |
2.681 |
H10A–O8 |
2.519 |
H10A–O4 |
2.454 |
H26A–O4 |
2.277 |
H26A–O3 |
2.180 |
H38A–O3 |
2.556 |
H60A–O12 |
2.656 |
Cl1–H22B |
2.828 |
Cl1–H24 |
2.149 |
Cl4–H16 |
2.834 |
2 |
H1–O4 |
2.536 |
H2A–O11 |
2.363 |
H2B–O11 |
2.552 |
H2A–O11 |
1.962 |
H10A–O7 |
2.368 |
H9A–O1 |
2.503 |
H11A–O4 |
2.522 |
H11A–O8 |
2.164 |
H4–O4 |
2.632 |
3.2. 1H NMR and MALDI-TOF mass spectrometric analysis of cucurbiturils and guests
3.2.1. 1H NMR spectra of cucurbiturils and guests. NMR is one of the most commonly used and effective methods for studying host–guest chemistry. When the molar ratio of the guest to the host was 0.25 (Table 3), all of the proton signals of the guest shifted to lower field, indicating that the whole guest molecule was located within the portals of the respective cucurbiturils, and was thus de-shielded by the cucurbituril. Meanwhile, the chemical shifts of protons far away from the piperazine moiety showed little change.
Table 3 1H NMR data of interaction between cucurbiturils and guest
Complex |
Hα |
Hβ |
Hγ |
Hδ |
Hε |
MeOPP |
7.01 |
6.91 |
3.67 |
3.28 |
3.25 |
n(MeOPP)/n((CyH)2Q[6]) = 0.25 |
−0.40 |
−0.06 |
−0.12 |
−0.42 |
−0.39 |
n(MeOPP)/n(CyP6Q[6]) = 0.25 |
−0.21 |
−0.01 |
−0.02 |
−0.28 |
−0.24 |
Fig. 5 shows 1H NMR titration spectra obtained upon adding free guest to the cucurbiturils. In Fig. 5(A), (a) is the NMR spectrum of (CyH)2Q[6], (b–d) are the NMR spectra when n(MeOPP)/n((CyH)2Q[6]) is equal to 0.25, 0.75, and 1, and (e) is the NMR spectrum of the free guest. As the concentration of the guest was increased, the proton signals shifted towards lower field to varying degrees, indicating that MeOPP molecules interact with the outer walls of the cucurbiturils. This is consistent with the single-crystal X-ray diffraction characterization. The 1H NMR titration spectra of CyP6Q[6]@MeOPP are similar to those of (CyH)2Q[6]@MeOPP, and so are not described in detail.
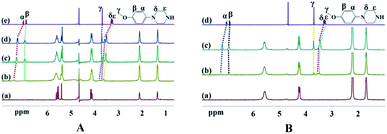 |
| Fig. 5 (A) (CyH)2Q[6]@MeOPP 1H NMR titration spectra: (a) 1H NMR spectrum of (CyH)2Q[6]; (b) n(MeOPP)/n((CyH)2Q[6]) = 0.25; (c) n(MeOPP)/n((CyH)2Q[6]) = 0.75; (d) n(MeOPP)/n((CyH)2Q[6]) = 1; (e) free guest MeOPP; (B) 1H NMR titration spectra of CyP6Q[6]@MeOPP: (a) 1H NMR spectrum of CyP6Q[6]; (b) n(MeOPP)/n(CyP6Q[6]) = 0.25; (c) n(MeOPP)/n(CyP6Q[6]) = 1; (d) free guest MeOPP. | |
3.2.2. Mass spectrometry. In order to further understand the interaction mode of the host and guest, we used matrix-assisted laser desorption/ionization time-of-flight mass spectrometry to characterize the structures of the complexes, as shown in Fig. 6. The (CyH)2Q[6]@MeOPP and CyP6Q[6]@MeOPP systems showed obvious molecular ion peaks at m/z 1297.5177 and 1429.6175, respectively. These molecular ion peaks could be attributed to [(CyH)2Q[6] + MeOPP + H]+ and [CyP6Q[6] + MeOPP + H]+ (theoretical calculated m/z values 1297.549 and 1429.6158, respectively). The results show that the host and guest interact in a 1
:
1 ratio, consistent with the results of the above analysis.
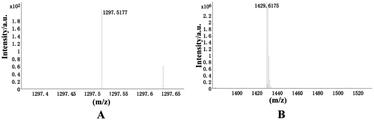 |
| Fig. 6 MALDI-TOF mass spectra of (CyH)2Q[6]@MeOPP and CyP6Q[6]@MeOPP. | |
4. Conclusions
In this article, it shows that 1-(4-methoxyphenyl)piperazine (MeOPP) interacted with symmetrical dicyclohexyl-substituted cucurbit[6]uril ((CyH)2Q[6]) and cyclopentanocucurbit[6]uril (CyP6Q[6]) under hydrothermal conditions in the presence of CdCl2 as an inducer to construct two different supramolecular self-assemblies. These two self-assembled structures have been characterized by single crystal XRD analysis, NMR, and MALDI-TOF mass spectrometry.
Our experiments have shown that, in the presence of CdCl2 as an inducer, the heterocycle of the MeOPP molecule acted like a “lid” connected to the carbonyl oxygen atoms of the cucurbituril portals. Moreover, the benzene ring and cucurbituril were also engaged in a weak π⋯π interaction, thereby making the guest molecule more firmly bound at the cucurbituril portal. The outer wall of melon rings interacted with multiple [CdCl4]2− anions through anion-induced OSIQ, and the rings were connected by hydrogen bonds to form a two-dimensional supramolecular self-assembly.
A difference was that there was a dislocation arrangement between adjacent cucurbituril moieties in complex 1, and there was also a dislocation arrangement between the guests; on the contrary, there was a parallel arrangement in complex 2.
Conflicts of interest
There are no conflicts to declare.
Acknowledgements
This work was financially supported by the National Natural Science Foundation of China (Grant No. 21762011) and Guizhou Science and Technology Planning Project (Guizhou Science and Technology Cooperation Platform Talent [2017]5788).
Notes and references
- M. Dusselier and M. E. Davis, Chem. Rev., 2018, 118, 5265–5329 CrossRef CAS.
- O. M. Yaghi, G. M. Li and H. L. Li, Nature, 1995, 378, 703–706 CrossRef CAS.
- A. Helal, Z. H. Yamani, K. E. Cordova and O. M. Yaghi, Natl. Sci. Rev., 2017, 4, 296–298 CrossRef CAS.
- H. Wang, Z. T. Zeng, P. Xu, L. S. Li, G. G. Zeng, R. Xiao, Z. Y. Tang, D. L. Huang, L. Tang, C. Lai, D. N. Jiang, Y. Liu, H. Yi, L. Qin, S. J. Ye, X. Y. Ren and W. W. Tang, Chem. Soc. Rev., 2019, 48, 488–516 RSC.
- P. J. Waller, F. Gandara and O. M. Yaghi, Acc. Chem. Res., 2015, 48, 3053–3063 CrossRef CAS.
- Y. H. Wen, J. Zhang, Q. Xu, X. T. Wu and Q. L. Zhu, Coord. Chem. Rev., 2018, 376, 248–276 CrossRef CAS.
- J. Y. Kim, H. Oh and H. R. Moon, Adv. Mater., 2019, 31, 1805293 CrossRef.
- S. S. Han, J. L. Mendoza-Cortes and W. A. Goddard III, Chem. Soc. Rev., 2009, 38, 1460–1476 RSC.
- G. Chedid and A. Yassin, Nanomaterials, 2018, 8(11), 916 CrossRef.
- D. Whang, J. Heo, J. H. Park and K. Kim, Angew. Chem., Int. Ed., 1998, 37, 78–80 CrossRef CAS.
- L. Isaacs, Chem. Commun., 2009, 619–629 RSC.
- A. I. Day, R. J. Blanch, A. P. Arnold, S. Lorenzo, G. R. Lewis and I. Dance, Angew. Chem., Int. Ed., 2002, 41, 275–277 CrossRef CAS.
- L. Isaacs, S. K. Park, S. M. Liu, Y. H. Ko, N. Selvapalam, Y. Kim, H. Kim, P. Y. Zavalij, G. H. Kim, H. S. Lee and K. Kim, J. Am. Chem. Soc., 2005, 127, 18000–18001 CrossRef CAS.
- X. Xiao, J. X. Liu, Z. F. Fan, K. Chen, Q. J. Zhu, S. F. Xue and Z. Tao, Chem. Commun., 2010, 46, 3741–3743 RSC.
- J. Kim, I. S. Jung, S. Y. Kim, E. Lee, J. K. Kang, S. Sakamoto, K. Yamaguchi and K. Kim, J. Am. Chem. Soc., 2000, 122, 540–541 CrossRef CAS.
- S. M. Liu, K. Kim and L. Isaacs, J. Org. Chem., 2007, 72, 6840–6847 CrossRef CAS.
- X. L. Ni, X. Xiao, H. Cong, Q. J. Zhu, S. F. Xue and Z. Tao, Acc. Chem. Res., 2014, 47, 1386–1395 CrossRef CAS.
- M. N. Sokolov, M. N. Dybtsev and V. P. Fedin, Russ. Chem. Bull., 2003, 52, 1041–1060 CrossRef CAS.
- O. A. Gerasko, M. N. Sokolov and V. P. Fedin, Pure Appl. Chem., 2004, 76, 1633–1646 CAS.
- V. P. Fedin, Russ. J. Coord. Chem., 2004, 30, 151–158 CrossRef CAS.
- X. L. Ni, X. Xiao, H. Cong, L. L. Liang, K. Chen, X. J. Cheng, N. N. Ji, Q. J. Zhu, S. F. Xue and Z. Tao, Chem. Soc. Rev., 2013, 42, 9480–9508 RSC.
- K. Kim, Chem. Soc. Rev., 2002, 31, 96–107 RSC.
- J. Lagona, P. Mukhopadhyay, S. Chakrabarti and L. Isaacs, Angew. Chem., Int. Ed., 2005, 44, 4844–4870 CrossRef CAS.
- E. Masson, X. Ling, R. Joseph, L. Kyeremeh-Mensah and X. Lu, RSC Adv., 2012, 2, 1213–1247 RSC.
- F. Zhang, T. Yajima, Y. Z. Li, G. Z. Xu, H. L. Chen, Q. T. Liu and O. Yamauchi, Angew. Chem., Int. Ed., 2005, 44, 3402–3407 CrossRef CAS.
- Y. Huang, R. Gao, M. Liu, L. Chen, X. Ni, X. Xiao, H. Cong, Q. Zhu, K. Chen and Z. Tao, Angew. Chem., Int. Ed., 2020 DOI:10.1002/anie.202002666.
- F. Wu, L. H. Wu, X. Xiao, Y. Q. Zhang, S. F. Xue, Z. Tao and A. I. Day, J. Org. Chem., 2012, 77, 606–611 CrossRef CAS.
- L. M. Zheng, J. N. Zhu, Y. Q. Zhang, Q. J. Zhu, S. F. Xue, Z. Tao, J. X. Zhang, Z. Xin, Z. B. Wei, L. S. Long and A. I. Day, Supramol. Chem., 2008, 20, 709–716 CrossRef CAS.
Footnote |
† Electronic supplementary information (ESI) available. See DOI: 10.1039/d0ra07988j |
|
This journal is © The Royal Society of Chemistry 2020 |