DOI:
10.1039/D0RA07824G
(Paper)
RSC Adv., 2020,
10, 40690-40696
Use of pyrazoles as ligands greatly enhances the catalytic activity of titanium iso-propoxide for the ring-opening polymerization of L-lactide: a cooperation effect†
Received
12th September 2020
, Accepted 22nd October 2020
First published on 9th November 2020
1. Introduction
Petrochemical plastics are extensively used in modern society; however, widely discarded plastic waste pollutes the environment continually1–4 because bacteria cannot decompose it naturally within a short period. To accelerate the environmental degradation of polymers, biodegradable polylactide (PLA)5–7 has been developed for creating a sustainable society. PLA-based biomaterials are used in various fields8–18 because of their biocompatible19,20 and permeable21 physical properties. One of the methods of PLA synthesis is the Lewis acidic metal-catalyzed ring-opening polymerization (ROP) of cyclic esters.22–31
For biomaterials, metal residuals present in resulting PLA are a serious problem, and using catalysts with non-cytotoxic metals is a straightforward approach to solving this problem. Because of the non-cytotoxic property and strong Lewis acidity of titanium, Ti complexes22,23,30–33 are commonly used catalysts in LA ROP. Numerous Ti complexes bearing various ligands such as calix[4]arene,33,34 Schiff base,35–39 salen,40–42 salan,43–46 phenolate,47–50 aminophenolate,51,52 benzotriazole phenolate,53–55 phosphinophenolate,56 thiophenolate,57,58 bis-phenolate-N-heterocyclic carbene,45 pyridonate,59,60 and pyrrolide61 have been reported to exhibit considerable catalytic activity or controllability, which is contributed by ligands. However, for most studies, focusing on materials is inconvenient, because the synthesis and purification of Ti catalysts are time-consuming processes. An efficient method of fabricating PLA without time-consuming Ti catalyst-base synthesis and purification is necessary. Herein, commercially available Ti alkoxides was also used catalysts for cyclic esters polymerization.62–65 Recently, dinuclear Ti complexes38 bearing hydrazine-bridging Schiff base ligands (Fig. 1) were reported to exhibit a high catalytic activity of LA polymerization because of the cooperation between two Ti atoms. Based on this study, if pyrazole is added to LA polymerization with titanium iso-propoxide (TiOiPr4) as a catalyst, bringing two Ti atoms close together to enable dinuclear cooperation is possible. Following this strategy, several pyrazole derivatives (Fig. 2) were added to LA polymerization with a TiOiPr4 catalyst to investigate dinuclear cooperation relative to the mixture of TiOiPr4 and pyrazole.
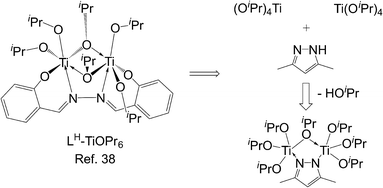 |
| Fig. 1 Strategy of dinuclear Ti complexes in LA polymerization inspired by the literature. | |
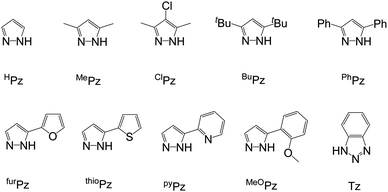 |
| Fig. 2 Pyrazole derivatives used in this study. | |
2. Experimental section
2.1 Chemicals
Standard Schlenk techniques and a N2-filled glovebox were used all over the isolation and treatment of all the compounds. Solvents, L-lactide (LA), and deuterated solvents were purified prior to use. LA, HPz, MePz, BuPz, PhPz, furPz, thioPz, Tz, pyPz, and MeOPz were purchased from Aldrich. ClPz66 were prepared following literature procedures. 1H and 13C NMR spectra were recorded on a Varian Gemini 2000-200 (200 MHz for 1H and 50 MHz for 13C) spectrometer. Chemical shifts (in ppm) of 1H NMR spectra were referenced to tetramethylsilane (δ = 0 ppm) in CDCl3 as an internal standard, and chemical shifts of 13C NMR spectra were reported in ppm referenced to the center line of a triplet at 77.0 ppm of CDCl3. Microanalyses were performed using a Heraeus CHN-O-RAPID instrument. The gel permeation chromatography (GPC) measurements were performed on a Jasco PU-2080 PLUS HPLC pump system equipped with a differential Jasco RI-2031 PLUS refractive index detector using THF (HPLC grade) as an eluent (flow rate 1.0 mL min−1, at 40 °C). The chromatographic column was JORDI Gel DVB 10−3 Å, and the calibration curve was made by primary polystyrene standards to calculate molar masses of PLA. Values of MnGPC were obtained through gel permeation chromatography (GPC) times 0.58.
2.2 Synthesis of MePz2Ti2OiPr7
A mixture of MePz (0.96 g, 10 mmol) and TiOiPr4 (2.84 g, 10 mmol) in toluene (20 mL), was stirred at room temperature for 24 h. Volatile materials were removed under vacuum to give light yellow mud, and then hexane was transferred to be the suspension. The light-yellow powder was obtained after filtering, and was recrystallized in toluene to form the crystal. Yield: 1.15 g (33%). 1H NMR spectrum (CDCl3, 200 MHz, Fig. S1†) was complex, but it could be assigned that two β-Hs were at 5.59 and 5.43 ppm, and the methine protons of isopropyl oxide were at 4.92–4.28 ppm, and dimethyl groups of MePz were at 2.39 and 2.14 ppm.
3. Results and discussion
3.1 Polymerization of LA
Table 1 presents the conditions for optimizing LA polymerization by using a mixture of TiOiPr4 and thioPz as the catalyst. Entries 1–5 in Table 1 reveal that the 1
:
1 ratio of TiOiPr4 and thioPz ([LA] = 1 M, [TiOiPr4] = 10 mM) exhibited the fastest polymerization rate. When [LA] was increased to 2 M with 40 mM of [TiOiPr4], after 10 min, the conversion became 87%. Under this condition ([LA] = 2 M, [LA]
:
[TiOiPr4]
:
[thioPz] = 50
:
1
:
1 in 5 mL toluene), various pyrazole derivatives were used to analyze LA polymerization, and all pyrazole derivatives improved the catalytic activity of TiOiPr4, except pyPz and Tz in the following order: furPz > BuPz > PhPz > MeOPz > HPz > MePz = thioPz > ClPz > pyPz > Tz. Although MePz improved the catalytic activity of TiOiPr4, it provided low controllability (dispersity, Đ = 1.68). To solve this problem, the TiOiPr4 concentration was decreased from 20 to 13.4 mM, and the Đ value was decreased to 1.10. LA polymerization using TiOiPr4 (13.4 mM) as a catalyst with MePz was systematically investigated with the [LA]/[TiOiPr4] ratio ranging from 50 to 300 (entries 16–20). The results revealed that LA polymerization was controllable, confirmed by the linear relationship between [LA]0/[TiOiPr4] and MnGPC (Fig. 3). However, the controllability in the [LA]/[TiOiPr4] ratio of 300 (Đ = 1.49, entry 20 in Table 1) was low, and it may be ascribed to transesterification67 because of the long polymerization time at 60 °C. Fig. 3 revealed that four isopropoxides of TiOiPr4 could be initiators to initiate LA. TiOiPr4 with thioPz exhibited a higher catalytic activity (3.5 folds) in LA polymerization than LH-TiOPr6 (entry 21)38 did.
Table 1 L-Lactide polymerization with the mixture of TiOiPr4 and pyrazole derivatives as catalysts in toluenea
Entry |
Ligand (TiOiPr4 : L) |
Time (min) |
Conv.b (%) |
MnGPCc (g mol−1) |
MnNMRb (g mol−1) |
Đc |
kobs (min−1) |
In general, the reaction was carried out in 5 mL toluene with [LA] = 2 M at 60 °C for LA polymerization ([LA] : [TiOiPr4] = 50 : 1). The data were determined using 1H NMR analysis. Values of MnGPC were corrected considering Mark–Houwink factor (0.58) from polystyrene standards in THF. [LA] = 1 M, in 5 mL toluene, [LA] : [TiOiPr4] = 100 : 1. [LA] : [TiOiPr4] : [MePz] = 50 : 1 : 1, [TiOiPr4] = 13.4 mM in toluene 15 mL. [LA] : [TiOiPr4] : [MePz] = 100 : 1 : 1, [TiOiPr4] = 13.4 mM in toluene 15 mL. [LA] : [TiOiPr4] : [MePz] = 150 : 1 : 1, [TiOiPr4] = 13.4 mM in toluene 15 mL. [LA] : [TiOiPr4] : [MePz] = 200 : 1 : 1, [TiOiPr4] = 13.4 mM in toluene 15 mL. [LA] : [TiOiPr4] : [MePz] = 300 : 1 : 1, [TiOiPr4] = 13.4 mM in toluene 15 mL. [LA] : [LH-TiOPr6] = 100 : 1, [LA] = 2.0 mM in toluene 5 mL at 60 °C. |
1d |
thioPz (1 : 0.5) |
50 |
85 |
3900 |
3300 |
1.30 |
0.035 |
2d |
thioPz (1 : 1) |
45 |
86 |
4700 |
4600 |
1.51 |
0.036 |
3d |
thioPz (1 : 2) |
125 |
84 |
7000 |
6000 |
2.10 |
0.008 |
4d |
thioPz (1 : 4) |
220 |
99 |
7400 |
6500 |
2.02 |
0.013 |
5 |
thioPz (1 : 1) |
10 |
87 |
2300 |
2200 |
1.27 |
0.232 |
6 |
L free |
22 |
92 |
2100 |
1600 |
1.75 |
0.161 |
7 |
HPz (1 : 1) |
8 |
88 |
2600 |
2100 |
1.31 |
0.285 |
8 |
MePz (1 : 1) |
13 |
94 |
7100 |
4400 |
1.68 |
0.233 |
9 |
ClPz (1 : 1) |
14 |
89 |
1900 |
1800 |
1.13 |
0.166 |
10 |
BuPz (1 : 1) |
9 |
95 |
2100 |
1700 |
1.23 |
0.394 |
11 |
PhPz (1 : 1) |
10 |
94 |
2500 |
2000 |
1.25 |
0.334 |
12 |
furPz (1 : 1) |
5 |
86 |
2200 |
2100 |
1.23 |
0.418 |
13 |
pyPz (1 : 1) |
15 |
88 |
2000 |
2200 |
1.18 |
0.152 |
14 |
MeOPz (1 : 1) |
10 |
92 |
6500 |
3000 |
1.38 |
0.288 |
15 |
Tz (1 : 1) |
20 |
87 |
1900 |
1800 |
1.12 |
0.120 |
16e |
MePz (1 : 1) |
16 |
88 |
1800 |
1700 |
1.10 |
— |
17f |
MePz (1 : 1) |
25 |
89 |
3900 |
3600 |
1.27 |
— |
18g |
MePz (1 : 1) |
24 |
80 |
5500 |
5200 |
1.27 |
— |
19h |
MePz (1 : 1) |
45 |
87 |
8500 |
7900 |
1.32 |
— |
20i |
MePz (1 : 1) |
95 |
90 |
13 100 |
12 500 |
1.49 |
— |
21j |
LH-TiOPr638 |
50 |
89 |
3100 |
— |
1.25 |
0.065 |
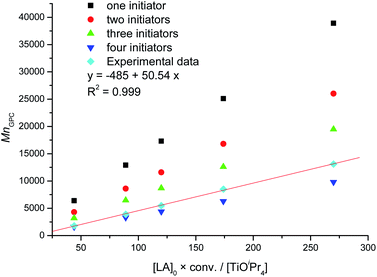 |
| Fig. 3 Linear plot of various Mncal. with the supposed initiators and MnGPC against [LA]0 × conv./[TiOiPr4] (Table 1, entries 16–20). | |
A survey of LA ROP using Ti complexes as catalysts revealed that few Ti catalysts could polymerize LA at room temperature. Therefore, LA polymerization was conducted at room temperature (Table 2) to determine whether the addition of pyrazole ligands can enhance the catalytic ability of TiOiPr4 at room temperature. In addition, reducing the polymerization temperature may improve the controllability of Ti catalysts.
Table 2 L-Lactide polymerization with the mixture of TiOiPr4 and pyrazole derivatives as catalysts in CH2Cl2 at room temperaturea
Entry |
Ligand (TiOiPr4 : L) |
Time (min) |
Conv.b (%) |
MnGPCc (g mol−1) |
MnNMRb (g mol−1) |
Đc |
kobs × 103 (min−1) |
In general, the reaction was carried out in 2.5 mL CH2Cl2 with [LA] = 2 M at room temperature for LA polymerization ([LA] : [TiOiPr4] = 25 : 1). The data were determined using 1H NMR analysis. Values of MnGPC were corrected considering Mark–Houwink factor (0.58) from polystyrene standards in THF. [LA] : [TiOiPr4] : [furPz] = 37.5 : 1 : 1, [TiOiPr4] = 0.08 M in 2.5 mL CH2Cl2. [LA] : [TiOiPr4] : [furPz] = 43.75 : 1 : 1, [TiOiPr4] = 0.08 M in 2.5 mL CH2Cl2. [LA] : [TiOiPr4] : [furPz] = 50 : 1 : 1, [TiOiPr4] = 0.08 M in 2.5 mL CH2Cl2. [LA] : [TiOiPr4] : [furPz] = 62.5 : 1 : 1, [TiOiPr4] = 0.08 M in 2.5 mL CH2Cl2. [LA] : [TiOiPr4] : [furPz] = 25 : 1 : 1, [TiOiPr4] = 0.08 M in 2.5 mL CDCl3. After the conversion of the reaction (entry 16) was 84%, LA (0.72 g) was transferred into the solution. After the conversion of the reaction (entry 17) was 92%, LA (0.72 g) was transferred into the solution. After the conversion of the reaction (entry 17) was 83%, LA (0.72 g) was transferred into the solution. [TiOiPr4] : [furPz] = 1000 : 1 : 1, melt reaction at 100 °C. [LA] : [TiOiPr4] = 1000 : 1, melt reaction at 100 °C. |
1 |
L free |
4290 |
85 |
1600 |
1700 |
1.25 |
0.3 |
2 |
HPz |
450 |
80 |
900 |
1000 |
1.12 |
3.0 |
3 |
MePz |
1670 |
85 |
1300 |
1200 |
1.08 |
0.9 |
4 |
ClPz |
720 |
85 |
1100 |
1200 |
1.08 |
2.0 |
5 |
BuPz |
370 |
88 |
1100 |
1000 |
1.07 |
5.0 |
6 |
PhPz |
1335 |
89 |
1400 |
1200 |
1.12 |
1.0 |
7 |
furPz |
540 |
90 |
1000 |
1100 |
1.08 |
4.0 |
8 |
thioPz |
660 |
84 |
1200 |
1200 |
1.08 |
2.0 |
9 |
pyPz |
660 |
87 |
1000 |
1100 |
1.08 |
2.4 |
10 |
MeOPz |
660 |
85 |
1200 |
1200 |
1.08 |
2.0 |
11 |
Tz |
1670 |
87 |
1400 |
1300 |
1.11 |
0.7 |
12d |
furPz |
315 |
95 |
1400 |
1200 |
1.08 |
— |
13e |
furPz |
310 |
88 |
1600 |
1500 |
1.09 |
— |
14f |
furPz |
450 |
88 |
1800 |
1800 |
1.11 |
|
15g |
furPz |
510 |
89 |
2100 |
2200 |
1.27 |
|
16h |
furPz |
390 |
84 |
700 |
800 |
1.13 |
— |
17i |
+100 LA |
895 |
92 |
1800 |
1900 |
1.09 |
— |
18j |
+100 LA |
1495 |
83 |
2300 |
2200 |
1.16 |
— |
19k |
+100 LA |
2000 |
72 |
2600 |
2600 |
1.14 |
— |
20l |
furPz |
240 |
93 |
51 100 |
— |
1.10 |
|
21m |
L free |
240 |
52 |
31 600 |
— |
1.26 |
|
All pyrazole derivatives improved the catalytic activity of TiOiPr4 in the following order: BuPz > furPz > HPz > pyPz > ClPz = thioPz = MeOPz > PhPz > MePz > Tz (Table 2). In the CH2Cl2 solution, the pyrazole ligand provided the benefit of considerable improvement of the catalytic reaction. For example, kobs of TiOiPr4 with BuPz was 17 times higher than that of TiOiPr4, and kobs of TiOiPr4 with furPz was 13 times higher than that of TiOiPr4. In addition, the controllability of TiOiPr4 with all pyrazole derivatives was improved (Đ = 1.07–1.12). Although TiOiPr4 with BuPz revealed the highest polymerization rate, BuPz is overly expensive. Therefore, furPz was used as a ligand with TiOiPr4 as a catalyst to polymerize LA with various ratios of [LA]/[TiOiPr4] (entries 12–19, Table 2). The [LA]/[TiOiPr4] ratio from 37.5 to 62.5 was investigated, and the molecular mass (MnGPC) of PLA increased from 1400 to 2100. According to the solubility of LA in CH2Cl2, the limit of the [LA]/[TiOiPr4] ratio is 62.5 (1.80 g of LA in 2.5 mL CH2Cl2), and PLA with high molecular mass PLA cannot be synthesized by increasing the [LA]/[TiOiPr4] ratio.
To investigate the living property68,69 of TiOiPr4 with the pyrazole ligand in LA polymerization, first, LA was polymerizated ([LA]
:
[TiOiPr4]
:
[furPz] = 100
:
4
:
4 in CDCl3, entry 16 in Table 2). After 390 min, the conversion was 84%, and 100 equivalents of LA were reloaded into the solution (entry 17 in Table 2). However, the polymerization time increased to 895 min with a 92% conversion. Subsequently, 100 equivalents of LA were reloaded into the solution (entry 18 in Table 2), and the polymerization rate decreased. After 1495 min, the conversion was 83%, and 100 equivalents of LA were reloaded into the solution (entry 19 in Table 2). The solution could not be stirred after 2000 min with a 72% conversion. The slower LA polymerization rate can be ascribed to the higher viscosity of the polymerizated solution. On the basis of the linear relationship between MnGPC and ([LA]0 × conv.)/[TiOiPr4] (entries 7, and 12–19 in Table 2 and Fig. 4), TiOiPr4 with the pyrazole ligand demonstrated a high controllability with narrow Đ for LA polymerization. To confirm that PLA with high molecular mass can be synthesized using TiOiPr4 with the pyrazole ligand, LA was polymerizated using TiOiPr4 with furPz ([LA]
:
[TiOiPr4]
:
[furPz] = 1000
:
1
:
1 at 100 °C without solvent, entry 20 in Table 2). After 240 min, the conversion was 93%, and PLA (MnGPC = 51
100, and Đ = 1.10) was obtained. Compared with LA polymerization using TiOiPr4 without furPz (240 min, conv. = 52%, MnGPC = 31
600, Đ = 1.26, entry 21 in Table 2), adding furPz to LA polymerization improved the polymerization rate and enhanced the controllability for producing PLA.
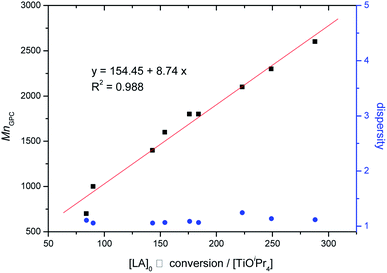 |
| Fig. 4 Linear plots of MnGPC versus ([LA]0 × conv.)/[TiOiPr4]. Blue solid dots indicate Đs. | |
The 1H nuclear magnetic resonance (NMR) spectrum of PLA (entry 2 in Table 2 and Fig. 5) confirmed the presence of one isopropyl oxide group (peak a) and a hydroxyl chain end (peak c′), suggesting that initiation occurred through insertion of an isopropyl oxide into LA. The matrix-assisted laser desorption/ionization time-of-flight (MALDI-TOF) mass spectrum of PLA (entry 5 in Table 2 and Fig. 6) revealed the presence of isopropyl oxide group at the end of the PLA chain.
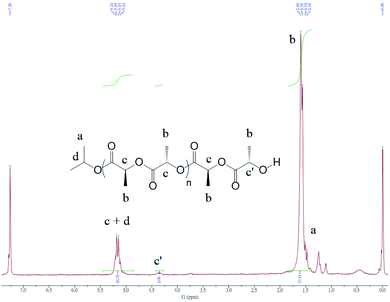 |
| Fig. 5 1H NMR spectrum of PLA (entry 13 in Table 2). | |
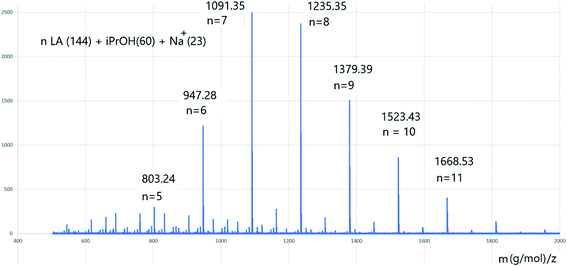 |
| Fig. 6 MALDI-TOF spectrum of PLA (entry 13 in Table 2). | |
3.2 Synthesis and characterization of MePz2Ti2OiPr7
To determine what was the real catalysis mechanism in the polymerization process, the reaction of MePz and TiOiPr4 (1
:
1) in toluene was investigated. Fig. 7 illustrates the crystal of MePz2Ti2OiPr7 (CCDC 1568213, Table S4†). However, the 1H NMR spectrum (Fig. S3†) revealed that MePz2Ti2OiPr7 was impure. The crystal data of MePz2Ti2OiPr7 indicated that the Ti–Ti distance was 3.2322(14) Å, which is slightly shorter than that of LBu-TiOPr638 distance (3.242 Å), and it implied the cooperative activation can occur in this system. To prove that MePz2Ti2OiPr7 is the real catalyst in LA polymerization, the crystal of impure MePz2Ti2OiPr7 was used as a catalyst in LA polymerization with the polymerization condition of entry 17 of Table 1 ([LA]
:
[MePz2Ti2OiPr7] = 100
:
0.5, [MePz2Ti2OiPr7] = 6.7 mM at 60 °C in 15 mL toluene). After 16 min, the conversion was 95% with MnGPC = 4900, Đ = 1.56, and kobs = 0.203 (min−1), and the results were similar to the results of entry 17 of Table 1 (conversion was 89% after 25 min).
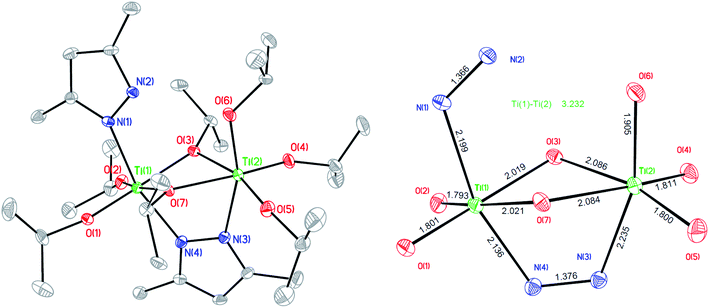 |
| Fig. 7 Molecular plot of MePz2Ti2OiPr7 with 20% probability ellipsoids (all hydrogen atoms were omitted for clarity). | |
4. Conclusions
Our strategy of using TiOiPr4 with pyrazole ligand for one-pot LA polymerization successfully improved the catalytic activity compared with using TiOiPr4 only. The crystal structure of MePz2Ti2OiPr7 revealed cooperative activation between two Ti atoms during LA polymerization. These results can provide a straightforward approach to synthesize PLA by using TiOiPr4 as a catalyst. In future, we intend to investigate the mechanism of LA polymerization.
Conflicts of interest
There are no conflicts to declare.
Acknowledgements
This study was supported by the Ministry of Science and Technology of Taiwan (Grant MOST 108-2113-M-037-017) and Kaohsiung Medical University (NSYSUKMU 107-P010 and KMU-DK109004). We thank the Centre for Research Resources and Development at Kaohsiung Medical University for instrumentation and equipment support.
Notes and references
- Y. Mato, T. Osobe, H. Takada, H. Kanehiro, C. Ohtake and T. Kaminuma, Environ. Sci. Technol., 2001, 35, 318–324 CrossRef CAS.
- D. S. Green, B. Boots, D. J. Blockley, C. Rocha and R. Thompson, Environ. Sci. Technol., 2015, 49, 5380–5389 CrossRef CAS.
- J. R. Jambeck, R. Geyer, C. Wilcox, T. R. Sieglar, M. Perryman, A. Andrady, R. Narayan and K. L. Law, Science, 2015, 347, 768–771 CrossRef CAS.
- J. Lamb, B. L. Willis, E. A. Foiorenza, C. S. Couch, R. Howard, D. N. Rader, J. D. True, L. A. Kelly, A. Ahmad, J. Jompa and C. D. Harvell, Science, 2018, 359, 460–462 CrossRef CAS.
- A. P. Gupta and V. Kumar, Eur. Polym. J., 2007, 43, 4053–4074 CrossRef CAS.
- M. Skotak, A. P. Leonov, G. Larsen, S. Noriega and A. Subramanian, Biomacromolecules, 2008, 9, 1902–1908 CrossRef CAS.
- Y. Zhu, C. Romain and C. K. Williams, Nature, 2016, 540, 354–362 CrossRef CAS.
- N. Kamaly, B. Yameen, J. Wu and O. C. Farokhzad, Chem. Rev., 2016, 116, 2602–2663 CrossRef CAS.
- K. Liu, X. Jiang and P. Hunziker, Nanoscale, 2016, 8, 16091–16156 RSC.
- C. G. Palivan, R. Goers, A. Najer, X. Zhang, A. Car and W. Meier, Chem. Soc. Rev., 2016, 45, 377–411 RSC.
- J. Y. Park, G. Gao, J. Jang and D.-W. Cho, J. Mater. Chem. B, 2016, 4, 7521–7539 RSC.
- S. K. Shukla, S. K. Shukla, P. P. Govender and N. G. Giri, RSC Adv., 2016, 6, 94325–94351 RSC.
- A. U. Birnin-Yauri, N. A. Ibrahim, N. Zainuddin, K. Abdan, Y. Y. Then and B. W. Chieng, Polymers, 2017, 9, 165 CrossRef.
- S. Medel, Z. Syrova, L. Kovacik, J. Hrdy, M. Hornacek, E. Jager, M. Hruby, R. Lund, D. Cmarko, P. Stepanek, I. Raska and B. Nyström, Eur. Polym. J., 2017, 93, 116–131 CrossRef CAS.
- A. Watts, N. Kurokawa and M. A. Hillmyer, Biomacromolecules, 2017, 18, 1845–1854 CrossRef CAS.
- C. Xiang and N. C. Acevedo, Polymers, 2017, 9, 179 CrossRef.
- S. Bhowmick, A. V. Thanusha, A. Kumar, D. Scharnweber, S. Rother and V. Koul, RSC Adv., 2018, 8, 16420–16432 RSC.
- B. Slater, S. O. Wong, A. Duckworth, A. J. P. White, M. R. Hill and B. P. Ladewig, Chem. Commun., 2019, 55, 7319–7322 RSC.
- M. A. Woodruff and D. W. Hutmacher, Prog. Polym. Sci., 2010, 35, 1217–1256 CrossRef CAS.
- Y. Ramot, M. Haim-Zada, A. J. Domb and A. Nyska, Adv. Drug Delivery Rev., 2016, 107, 153–162 CrossRef CAS.
- F. Carosio, S. Colonna, A. Fina, G. Rydzek, J. Hemmerlé, L. Jierry, P. Schaaf and F. Boulmedais, Chem. Mater., 2014, 26, 5459–5466 CrossRef CAS.
- B. H. Huang, C. Y. Tsai, C. T. Chen and B. T. Ko, Dalton Trans., 2016, 45, 17557–17580 RSC.
- C. Redshaw, Dalton Trans., 2016, 45, 9018–9030 RSC.
- Y. Wei, S. Wang and S. Zhou, Dalton Trans., 2016, 45, 4471–4485 RSC.
- Y. A. Chang and R. M. Waymouth, J. Polym. Sci., Part A: Polym. Chem., 2017, 55, 2892–2902 CrossRef CAS.
- T. Fuoco and D. Pappalardo, Catalysts, 2017, 7, 64 CrossRef.
- K. M. Osten and P. Mehrkhodavandi, Acc. Chem. Res., 2017, 50, 2861–2869 CrossRef CAS.
- C. Redshaw, Catalysts, 2017, 7, 165 CrossRef.
- R. Ligny, M. M. Hanninen, S. M. Guillaume and J. F. Carpentier, Chem. Commun., 2018, 54, 8024–8031 RSC.
- E. Stirling, Y. Champouret and M. Visseaux, Polym. Chem., 2018, 9, 2517–2531 RSC.
- A. B. Kremer and P. Mehrkhodavandi, Coord. Chem. Rev., 2019, 380, 35–57 CrossRef CAS.
- M. Chen and C. Chen, Chin. J. Chem., 2020, 38, 282–286 CrossRef CAS.
- O. Santoro and C. Redshaw, Catalysts, 2020, 10, 210 CrossRef CAS.
- Z. Sun, Y. Zhao, O. Santoro, M. R. J. Elsegood, E. V. Bedwell, K. Zahra, A. Walton and C. Redshaw, Catal. Sci. Technol., 2020, 10, 1619–1639 RSC.
- T. K. Saha, M. Mandal, D. Chakraborty and V. Ramkumar, New J. Chem., 2013, 37, 949 RSC.
- J.-X. He, Y.-L. Duan, X. Kou, Y.-Z. Zhang, W. Wang, Y. Yang and Y. Huang, Inorg. Chem. Commun., 2015, 61, 144–148 CrossRef CAS.
- D. Mandal, D. Chakraborty, V. Ramkumar and D. K. Chand, RSC Adv., 2016, 6, 21706–21718 RSC.
- H. C. Tseng, H. Y. Chen, Y. T. Huang, W. Y. Lu, Y. L. Chang, M. Y. Chiang, Y. C. Lai and H. Y. Chen, Inorg. Chem., 2016, 55, 1642–1650 CrossRef CAS.
- C. B. Durr and C. K. Williams, Inorg. Chem., 2018, 57, 14240–14248 CrossRef CAS.
- L. A. Brown, J. L. Rhinehart and B. K. Long, ACS Catal., 2015, 5, 6057–6060 CrossRef CAS.
- B. Gao, X. Li, R. Duan and X. Pang, New J. Chem., 2015, 39, 2404–2408 RSC.
- R. Duan, Z. Qu, X. Pang, Y. Zhang, Z. Sun, H. Zhang, X. Bian and X. Chen, Chin. J. Chem., 2017, 35, 640–644 CrossRef CAS.
- S. H. Kim, J. Lee, D. J. Kim, J. H. Moon, S. Yoon, H. J. Oh, Y. Do, Y. S. Ko, J.-H. Yim and Y. Kim, J. Organomet. Chem., 2009, 694, 3409–3417 CrossRef CAS.
- A. L. Zelikoff, J. Kopilov, I. Goldberg, G. W. Coates and M. Kol, Chem. Commun., 2009, 6804 RSC.
- C. Romain, L. Brelot, S. p. Bellemin-Laponnaz and S. Dagorne, Organometallics, 2010, 29, 1191–1198 CrossRef CAS.
- H.-W. Ou, H.-Y. Chen, H.-C. Tseng, M.-W. Hsiao, Y.-L. Chang, N.-Y. Jheng, Y.-C. Lai, T.-Y. Shih, Y.-T. Lin and H.-Y. Chen, J. Mol. Catal. A: Chem., 2014, 394, 97–104 CrossRef CAS.
- R. R. Gowda, D. Chakraborty and V. Ramkumar, Eur. J. Inorg. Chem., 2009, 2981–2993 CrossRef CAS.
- H. Y. Chen, M. Y. Liu, A. K. Sutar and C. C. Lin, Inorg. Chem., 2010, 49, 665–674 CrossRef CAS.
- R. R. Gowda, D. Chakraborty and V. Ramkumar, Inorg. Chem. Commun., 2011, 14, 1777–1782 CrossRef CAS.
- M. T. Jiang, S. R. Kosuru, Y. H. Lee, W. Y. Lu, J. K. Vandavasi, Y. C. Lai, M. Y. Chiang and H. Y. Chen, eXPRESS Polym. Lett., 2018, 12, 126–135 CrossRef CAS.
- S. L. Hancock, M. F. Mahon and M. D. Jones, Dalton Trans., 2011, 40, 2033–2037 RSC.
- L. C. Liang, S. T. Lin and C. C. Chien, Inorg. Chem., 2013, 52, 1780–1786 CrossRef CAS.
- M. Jeong Go, J. Min Lee, K. Mun Lee, C. Hwa Oh, K. H. Park, S. H. Kim, M. Kim, H.-R. Park, M. H. Park, Y. Kim and J. Lee, Polyhedron, 2014, 67, 286–294 CrossRef.
- D.-C. Liu, C.-Y. Li, P.-H. Lin, J.-D. Chen, C.-Y. Tsai, C.-H. Lin and B.-T. Ko, Inorg. Chem. Commun., 2018, 90, 1–7 CrossRef CAS.
- C.-Y. Li, C.-J. Yu and B.-T. Ko, Organometallics, 2012, 32, 172–180 CrossRef.
- L. C. Liang, Y. L. Hsu and S. T. Lin, Inorg. Chem., 2011, 50, 3363–3372 CrossRef CAS.
- M. Y. Lowe, S. Shu, S. M. Quan and P. L. Diaconescu, Inorg. Chem. Front., 2017, 4, 1798–1805 RSC.
- X. Xu, G. Luo, Z. Hou, P. L. Diaconescu and Y. Luo, Inorg. Chem. Front., 2020, 7, 961–971 RSC.
- R. L. Webster, N. Noroozi, S. G. Hatzikiriakos, J. A. Thomson and L. L. Schafer, Chem. Commun., 2013, 49, 57–59 RSC.
- D. J. Gilmour, R. L. Webster, M. R. Perry and L. L. Schafer, Dalton Trans., 2015, 44, 12411–12419 RSC.
- J. Liu, Y. Cao, L. Li, H. Pei, Y. Chen, J. Hu, Y. Qin, Y. Li, W. Li and W. Liu, RSC Adv., 2015, 5, 10318–10325 RSC.
- Y. Kim, G. K. Jnaneshwara and J. G. Verkade, Inorg. Chem., 2003, 42, 1437–1447 CrossRef CAS.
- C. J. Chuck, M. G. Davidson, M. D. Jones, G. Kociok-köhn, M. D. Lunn and S. Wu, Inorg. Chem., 2006, 45, 6595–6597 CrossRef CAS.
- J.-B. Zeng, M. Srinivansan, Y.-D. Li, R. Narayan and Y.-Z. Wang, J. Polym. Sci., Part A: Polym. Chem., 2010, 48, 5885–5890 CrossRef CAS.
- A. G. Amador, A. Watts, A. E. Neitzel and M. A. Hillmyer, Macromolecules, 2019, 52, 2371–2383 CrossRef CAS.
- F. Malbosc, V. Chauby, C. S.-L. Berre, M. Etienne, J.-C. Daran and P. Kalck, Eur. J. Inorg. Chem., 2001, 2689 CrossRef CAS.
- Y. Yu, E. J. Fischer, G. Storti and M. Morbidelli, Ind. Eng. Chem. Res., 2014, 53, 7333–7342 CrossRef CAS.
- O. Dechy-Cabaret, B. Martin-Vaca and D. Bourissou, Chem. Rev., 2004, 104, 6147–6176 CrossRef CAS.
- N. E. Kamber, W. Jeong, R. M. Waymouth, R. C. Pratt, B. G. G. Lohmeijer and J. L. Hedrick, Chem. Rev., 2007, 107, 5813–5840 CrossRef CAS.
Footnote |
† Electronic supplementary information (ESI) available. CCDC 1568213. For ESI and crystallographic data in CIF or other electronic format see DOI: 10.1039/d0ra07824g |
|
This journal is © The Royal Society of Chemistry 2020 |
Click here to see how this site uses Cookies. View our privacy policy here.