DOI:
10.1039/D0RA07344J
(Paper)
RSC Adv., 2020,
10, 44593-44600
Analysis of the effects of acoustic levitation to simulate the microgravity environment on the development of early zebrafish embryos†
Received
27th August 2020
, Accepted 25th November 2020
First published on 17th December 2020
Abstract
In this work, an acoustic standing wave field (ASWF) is used to simulate the space environment, which shows characteristics such as microgravity and the absence of containment and contact. Zebrafish embryos, used as the species under study in this work, were raised within the acoustic field by the authors, allowing the biological effects on such early zebrafish embryos, at each developmental stage and within the ASWF creating the acoustic levitation (AL) technology used, to be studied. In this way, the biological safety of thee specimens, simulating the space environment, could be carefully evaluated. Some important indexes of the process of zebrafish development, such as mortality, malformation rate, hatching rate, voluntary movement and heart rate were detected and analyzed. It has been found that the ASWF exerted considerable influence on the zebrafish embryos at the early development stage, influencing features such as the cleavage, blastula and gastrul stage, over the period 0–8 hour post fertilization (hpf). The zebrafish appear to show some features of teratogenesis, as well as lethal effects and a significant decrease of the hatching rate, after being treated by using the AL that was applied. Furthermore, it was observed that voluntary movements and the embryo heart rates apparently increased under these conditions. However, as the development of the embryo progressed into the bursa pharyngea stage (at 24–32 hpf), the influence of the ASWF creating the AL on zebrafish seemed almost to be insignificant, as there was no obvious difference between the characteristics of the experimental group and the control group. The experiment carried out has provided a scientific reference for the application of AL in this field, allowing the biological safety aspects of such zebrafish embryo development within a space environment to be evaluated.
Introduction
In recent years, the development of space technology has promoted consideration of the potential wider benefits of the utilization of the advantages of the space environment while, at the same time, the demand for undertaking a variety of scientific experiments within such a space environment is also increasing. However, it is well known that experiments carried out within the space environment are not only limited in scope, but also expensive to execute. Therefore, finding an experimental environment within which to simulate microgravity and create a containerless and non-contact space environment is an urgent problem to be tackled. Acoustic Standing Wave Field (ASWF) Levitation offers one such solution:1–4 however, there are few reports on the evaluation of key issues surrounding biological safety in that space environment, when using such an ASWF to create the levitation needed.
Given its successes seen in other fields such as materials preparation, acoustic levitation (AL) technology has shown a considerable potential for applications in life sciences and biology.5 Utilizing its characteristics of being contactless and allowing materials transportation,6–13 this technology can provide a wall-less, non-contact platform to allow the assembly of small parts, without negative effects from the container wall or sample holder to be experienced. Practical applications of this approach, such as in drug-loading, diagnosis and artificial insemination have been performed successfully.14–16 AL technology has also been employed to manipulate living cells, including murine embryonic stem cells, blood cells and small animals, including the ladybug, ant and adult fish, in biological studies reported. However, to date there are few reports on the evaluation of the actual safety of the AL technique on biological samples where, compared to other contactless levitation technologies, AL technology is usually considered to have negligible adverse biological effects on biological samples. Numerical analysis has been used to estimate that most of the energy of the acoustic wave is reflected away from the surface of such samples and it has been found that the organisms can still retain a high biological activity after being treated using AL,17 but try to escape from the trap of the acoustic standing wave field (ASWF). The zebrafish is important to study as its genome has 87% homology with the human genome and zebrafish are small in size, fast in development and transparent in the development stage, making them an ideal biological model in which to study embryonic developmental toxicity.18 In recent work, Sundvik et al. have reported on the biological effects of ASWF used in AL on zebrafishes,5 where they found that the development of both the otolith and trunk in zebrafish were not affected by the presence of the ASWF. However, in their work they did mention that the death rate increases for early zebrafish embryos after being treated for periods of 1000 or 2000 seconds by using AL. To date, to our knowledge, there are no other reports on the biological effects of AL on zebrafishes, a subject which to date has thus not been well studied and which is important.
In this paper, a study has been undertaken systematically to evaluate the biological safety aspects under these circumstances, by using zebrafish as model. The zebrafish embryo, at different development stages (that is critical time-points for embryo development) has been studied under treatment with AL. In order to understand more fully the effects of AL on the embryos studied, data were collected on key aspects: their death rate, hatchability, malformation rate, voluntary movement and heart rate. Further, their biological safety has also been evaluated, based on the effects of AL on the development of the nervous system, circulatory system, the brain and balance system within the zebrafish. Work undertaken has found that the biological effects of AL on the zebrafish embryo studied are closely related to the developmental stages of the embryo itself. The use of AL has had an apparent influence on the early embryonic development of the zebrafish (at 0 to 8 hours post fertilization (hpf)), which has resulted in the appearance of malformations, an increase of death rates and a noticeable decrease in the hatching rate. Additionally, voluntary movement and the heart rate of the embryos show an increasing trend, with the developments of brain and otolith being delayed. However, the influence of AL on the zebrafish is seen gradually to reduce in the later stages of embryo development, where there are no obvious differences seen between the experimental groups and the control group, after a 24 hpf development.
Materials and methods
AL technology
The AL technology used in this experiment is described below. The specific experimental setup here uses a monopodium type suspension device, illustrated in Fig. 1, in which the emitter was an ultrasonic transducer of 30 mm diameter and 35 mm ball radius, which was excited at a frequency of 21
061 Hz, with electrical characteristics of 17.3 V and 0.21 A. The reflector uses stainless steel as the material, being 35 mm in diameter and with a 33 mm ball cell radius. The distance between the emitter and the reflector was set to be 17.4 mm. Water droplets of 4 mm diameter, as well as eggs, can be levitated together in the air when the system is working. The AL technology used allows the formation of an acoustic field in the medium, using the ultrasonic transducer to generate a high frequency vibration, placing a reflector in the propagation path of the sound wave and adjusting the distance between the emitter and the reflector to an approximately integral multiple of ultrasonic half-wavelengths. As a result, a high-intensity standing wave is formed between the emitter and the reflector, which then can be used to levitate the object at the acoustic pressure node. The standing wave field also is applied along its radial direction, creating a restoring force on the levitated object, so that when this levitated object is disturbed slightly, it does not easily fall down. The acoustic levitation principle is shown diagrammatically in Fig. 1.
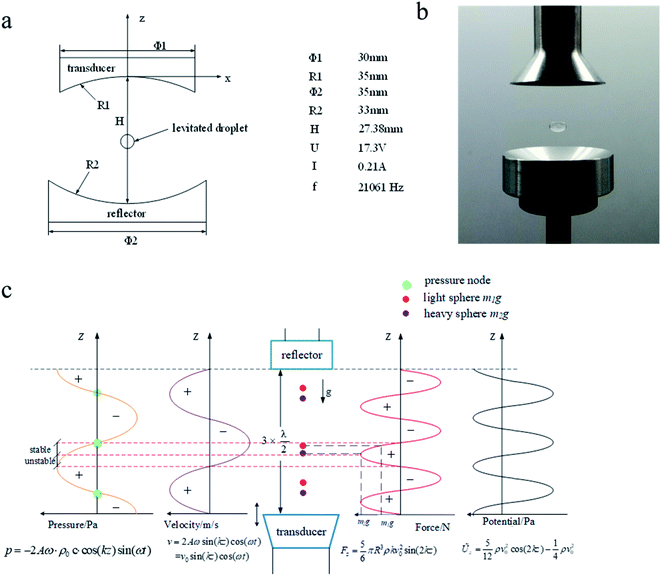 |
| Fig. 1 Acoustic levitation device (a). Principle model pattern of ultrasonic standing wave suspension device (b). Object graph of ultrasonic standing wave suspension device (c). | |
Live subject statement. All animal procedures were performed in accordance with the Guidelines for Care and Use of Laboratory Animals of Harbin Institute of Technology and approved by the Animal Ethics Committee of Harbin Institute of Technology.
Zebrafish
The zebrafish is a kind of spinal creature with 87% homology to the human genome. It is an ideal model in which to study embryonic developmental toxicity, owing to their small volume, fast development and embryonic transparency in the developmental stage. In the experiments planned, the AB zebrafish strain used was purchased from the Wuhan Institute of Aquatic Organisms of China. Zebrafish were bred in an automatic optical circulating water system (AAE-022-AA-A, Taiwan), at a room temperature of 28 °C, with a controlled circadian rhythm for 14 h/10 h, and fed brine shrimp eggs twice every day. In the afternoon, the female and male fishes (in a ratio 1
:
2) are put in a mating box with a transparent panel in the middle of the box to separate them. The next morning (at 9:00 am) and after removing the middle plate, the male and female fish began to mate. The fertilized eggs produced were collected and put in an E3 medium (comprising 5 mM NaCl, 0.17 mM KCl, 0.17 mM CaCl2·2H2O, 0.33 mM MgSO4·7H2O in ultrapure water). The healthy eggs of normal shape and the same developmental period were selected using a vertical microscope (type SMZ-168, Motic, China). This housing facility is an ordinary housing facility, and it has in keeping with national standard Laboratory Animal—Requirements of Environment and Housing Facilities (GB 14925-2001). The care of laboratory animal and the animal experimental operation have conforming to Beijing Administration Rule of Laboratory Animal, etc.
Treatment method
The normal development, fertilized eggs at different periods were randomly placed into culture plates, which have six holes filled with 4 mL of E3 medium. Each hole was filled with 15 eggs, kept in an incubator at a constant temperature of 28 °C. As shown in Fig. 1(b), the embryos of the experimental groups at developmental stages of 1, 4, 5, 6, 7, 8, 24, 27, 28, 29, 30, 32 hpf, respectively, are kept in a droplet of E3 medium and levitated for 30 minutes by use of AL. After that, they were moved back again into the incubator, and Fig. 2 shows in detail the time points in the experimental procedure for the AL treatments carried out. In order to eliminate the any accidental influences, these experiments were repeated three times. Every 24 hours, the nutrient solution was renewed and the dead embryos were picked out and discarded. The statistics on their voluntary movements were determined within 1 minute and the condition of the otolith and the brain beam development were checked, for the 24-phf zebrafish embryos. The statistics on the heartbeat frequencies in 15 s and any malformations were recorded, after the embryo developed for 48 hours. From 48 phf and beyond, their hatchability was checked every hour, until the control group hatched completely, at 54 hpf. Finally, the mortalities and malformations of the zebrafish embryos were recorded, at 96 hpf. The mortality rate, the hatching rate, voluntary movements and the heart rate data were obtained within the time stated and all data collected were analyzed by using the one-WayANOVA method with GraphPad Prism5 (GraphPad Software, San Diego, USA).
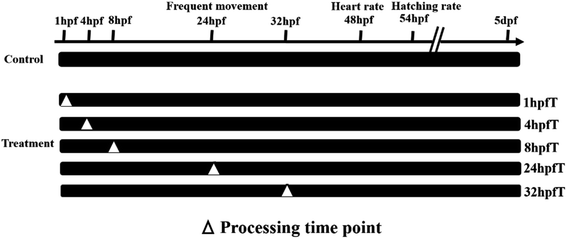 |
| Fig. 2 Treatment time point of ultrasonic standing wave suspended. | |
Results
Toxic effects of ASWF for AL on early zebrafish embryos
In order to study the potential for toxicity effects arising from the use of ASWF to create AL on each development period of the early zebrafish embryos studied, their mortalities first were studied. Fig. 3 shows data collected on the dependences of the mortality and the hatching rate on the developmental time for the zebrafish embryos exposed to AL. As shown in Fig. 3(A), the use of the AL treatment has led to an apparently significant lethality rate of the early zebrafish embryos, but fortunately with development progresses of embryos, the death rate decreased. For 1 phf embryos, the mortality was 82.3% and this reduced to 34.2% in the 4 hpf group. After that, their mortality rates fell rapidly until there was no difference between them and the control group. Fig. 3(B) shows the statistical results obtained for embryo hatchability in their different development stages. Compared with the data from the control group, the hatching rate of the zebrafish embryos decreased. After being treated with AL, at different times in the zebrafish embryo development, the incubation period has been seen to be significantly extended with respect to the control group. As indicated in Fig. 3(B), the control group had already fully hatched (100%) by 54 hpf, but for the experimental groups exposed to AL, these values were decreased to 50%, 37.5%, 46.7% and 53.3%, respectively.
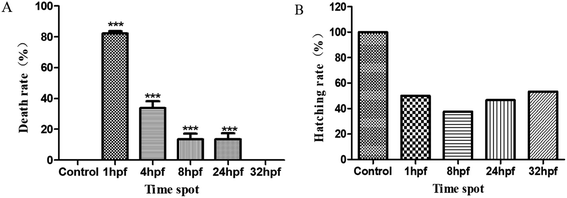 |
| Fig. 3 Influence of ultrasonic standing wave suspended processing on mortality and hatching rate of zebrafish. (A) The 96 hpf mortality of zebrafish embryo treated with ultrasonic standing wave suspension in each developmental period. (B) The 54 hpf hatchability of zebrafish embryo treated with ultrasonic standing wave suspension in each developmental period. | |
Teratogenic effects of AL on early zebrafish embryo
Fig. 4 shows the data on the deformities seen in the zebrafish, which are in development from the group of embryos treated by using AL. It was also found that the use of the AL technique had resulted in the slower development of zebrafish embryos and the deformities seen as a result mainly included spinal curvature, pericardial edema, yolk sac edema and atrophy of swim bladder. These malformations mainly occurred in the experimental group treated with AL, at the 4 hpf time point. The statistics on these malformations of the zebrafish studied are listed in Table 1. It should be noted that the mortality rate was 82.3% for 1 hpf experimental group, but there were no malformations in the fish which survived.
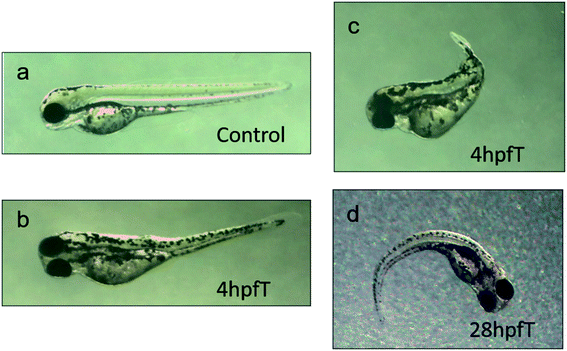 |
| Fig. 4 Teratogenic effect of ultrasonic standing wave suspended field on zebrafish embryo. (a) The control group (the group that without be processed by ultrasonic standing wave suspended field). (b) Bending and accompanied by cardiac edema. (c) Bending and aplasia. (d) Bending. | |
Table 1 The teratogenic effect of ultrasonic standing wave treatment on zebrafish embryo
Developmental time of zebra fish |
Processing time point in ultrasonic field |
Death rate of ultrasonic processing |
Malformation rate of ultrasonic processing |
Death/totality |
Malformation/survival |
Zygophase 0 hpf |
1 hpfT |
23/28 (82.14%) |
0/5 (0%) |
Blastula stage 2.25 hpf |
4 hpfT |
9/27 (33.33%) |
4/18 (22.22%) |
Gastrul stage 5.25 hpf |
8 hpfT |
3/23 (13.04%) |
1/20 (5.00%) |
Bursa pharyngea stage |
24 hpfT |
4/29 (13.79%) |
3/25 (12.00%) |
24 hpf |
32 hpfT |
0/30 (0%) |
0/30 (0%) |
Influence of AL on the circulatory system of the early zebrafish embryos
The circulatory system is one of the most sensitive systems to external physical stimulation. Zebrafish embryos began to hatch at 48 hpf. Within this stage, the development of cartilage became active and melanin began to accumulate and the development of circulation system has basically been completed.19 Fig. 5 shows the statistical results on the heart rates and degree of malformation of heart development for the zebrafish treated with AL, at these different development stages. As indicated in Fig. 5(A), the data have shown that the heart rates increased to 26.4%, 39.2%, 48.3% and 31.2%, respectively for the zebrafish treated by AL, at the following timepoints: 1, 8, 24 and 32 hpf. There are significant differences (P < 0.001) in what was observed between the experimental groups and the control group. Additionally, as shown in Fig. 5(B), the heart deformity of the pericardial edema in the 4 phf experimental group of zebrafishes was observed, where this kind of deformity accounted for 11.1% of all treated zebrafishes and for 50.0% of all abnormal fish in the experimental groups.
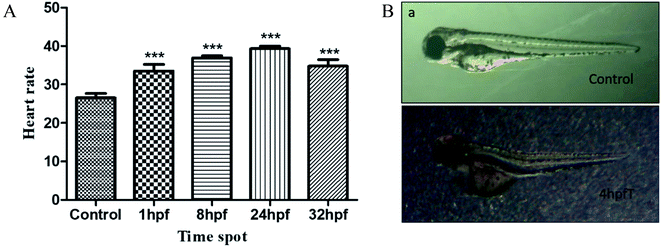 |
| Fig. 5 The effect of standing wave suspension processing in ultrasonic field on the heart development of zebrafish embryos. (A) The heart rate of zebrafish treated with standing wave suspension in ultrasonic field in 48 hpf, with 15 second in different development period. (B) The pericardium edema of zebrafish embryo be treated with standing wave suspension in ultrasonic field in 4 hpf. | |
Influence of AL on brain and balance system of the early zebrafish embryos
Fig. 6 shows the dependence of the development of zebrafish systema nervosum on the AL treatments used. The left-hand side of Fig. 6 shows the images of the brain beam and otolith development of the embryos treated with AL, at different hpf time points. It has been found that the development of the otolith and the brain beam of the embryo was delayed after being treated at 1, 4 and 8 hpf time points, when using AL. The brain beam profile under normal development is clear, and the morphology was completed in the control group at 24 hpf. However, the abnormal brain beam profile is vague and morphology is not completed. The otolith volume is larger and the edge is clear in the normal development, but it is smaller and even shows no development in the groups that have had the AL treatment. The right-hand side of Fig. 6 shows the dependence of voluntary movement, which is an index for the evaluation of brain nerve system function on the different AL-treated phf time points of the zebrafish embryos. Compared with the control group, the voluntary movements of zebrafish embryos have increased, after treatment with AL for 30 minutes.
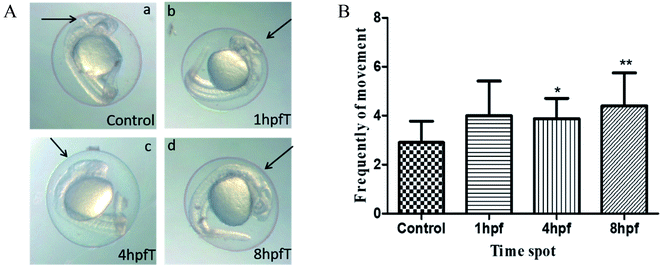 |
| Fig. 6 Effect of standing wave suspension processing in ultrasonic field on the development of cranial never system of zebrafish embryos. The effects of ultrasonic suspension on brain beam and otolith of zebrafish embryos in different developmental stage. | |
Discussion
For many reasons which include potential applications in a wide variety of areas, the zebrafish serves as an excellent model organism and has become one of the most important experimental systems that can be applied to in vivo studies, drug toxicology, environmental monitoring, embryonic development and disease treatment.20
Taking into account data on the influence of AL on important characteristics such as mortality rate, deformity ratio, hatch rate, heart and brain developments, the biological safety of AL on the zebrafish was evaluated in this study. Mortality is a main index used for the evaluation of acute toxicity which, as was found in this study, is closely dependent on the development stages of the zebrafish embryos which were treated using AL, at different time points. For the zebrafish embryos experiencing AL, at the 1 phf time point, the death rates are high, and rises to 80%. However, as time progresses and the embryo develops (such as was seen with AL treatment at time 8, 24 and 32 hpf), the death rates were seen gradually to reduce. It is, however, important to note that the mortality rate is zero for the group of 32 hpf, meaning that the influence of the AL exposure on the embryo development disappeared at this later development stage. The reason for that is that the early embryo is weak and very sensitive to external environmental stimuli. As a result, the development of early embryos showed an apparent influence from the use of AL and even has led to the termination of embryonic development.
At 54 phf, data were collected and a statistical analysis carried out into the hatching rates, as shown in Fig. 3(B), for zebrafish embryos treated with the use of AL at times 1, 8, 24 and 32 hpf. The hatching rates were seen to decrease after treatment with AL. It is suggested that the use of AL has led to dysplasia of the muscle tissue and motor nerves in the early embryos. As a result, the embryo cannot create enough power to break through the chorion.21 However, most of them will be hatched in the embryo anaphase, and only a few samples eventually died. Additionally, using the AL has postponed the hatching processes and as the embryo could not get sufficient oxygen from the environment, this has resulted in them not hatching and ultimately, their death.
Monitoring the heart rate is an important index for evaluating the function of the heart and thus the development of the circulation system in the zebrafish. In general, the heart rate tends to be stable when the zebrafish embryo has developed up to 48 phf. It has been found that the heart rate of the zebrafish embryo at 48 phf increased when samples were treated by use of AL, at 1, 8, 24 and 32 hpf, compared to the control groups. This difference was noted as being statistically significant (P < 0.001). It showed that the early developmental embryos were more sensitive to the effects of the AL treatment. From the toxicology point of view, the decline of the heart rate can be used as the evaluation index for the toxicological effects on the zebrafish embryos. The increase of the heart rate has resulted from an irritability response to the AL stimulation. Although the AL has no toxic effects on the zebrafish embryos, the heart development of the early embryos is more sensitive to the influence of the AL, resulting in heart cystica edema and an abnormal heart rate.
Autonomous movement (AM) within 1 min is used as an evaluation of the nervous system development of early zebrafish embryos at 24 hpf.22 Compared to the control group, the AM frequency of the AL treated embryo has increased. Looking in particular at the AL treated groups at times 4 and 8 hpf, the embryos showed a statistically significant difference (P < 0.05), which could be attributed to sensitive irritability of the nervous system to environmental stimuli, for the early zebrafish embryos.23 The frequency of the voluntary movement did not obviously decline but indicated that the function of the zebrafish nervous system was not influenced significantly by use of the AL treatment.
It was noted that deformities occurred in embryos when treated with AL at their early development stages (1, 4, 8 hpf) in the study carried out here. The malformations observed occurred slowly, mainly including the otolith, brain beam (epencephalon primordium), bending development and heart cyst edema. In the experimental groups observed, which were treated with AL in their later embryo stages, there is no apparent teratogenicity (observing at 24, 32 hpf). Compared with typical vertebrates, zebrafish have no outer and middle ear, but have a similar structure of the inner ear, which is comprised of three semicircular canals and otolith organs. The otolith has the function of being a sound sensor.24
It has been demonstrated that the sound exerts an apparent influence on the movement ability of the fish. Neo et al. found recently (in 2015) that even sound at a moderate sound level (112 dB re 1 μPa) can affect the swimming behavior of the fish studied.25 In the experiments, it was found that using the AL treatment at an early stage had a negative influence on the development of the otolith, resulting in a delay in the otolith development. It should be noted that in addition to experiencing the sound, the otolith also experiences the effects of gravity. It is noticeable that in the presence of the AL treatment, not only is there a change due to the presence of the sound, but also due to the gravity field present, as the embryo experiences a microgravity situation. The otolith is sensitive both to sound and gravity fields in their developmental process and it has been found that otolith fusion in zebrafishes was induced through the increase of the gravity field.26 When the zebrafish embryos are in the sound field provided by the AL, the otolith cannot feel the normal sound coming from the environment. Simultaneously, because of their being in a suspended situation, the embryos are in microgravity, which means that they do not perceive the influence of the Earth's gravity. As a result, the otolith can neither develop the sense of hearing normally, nor lose their ability to perceive the gravity field – therefore, the otolith development of these embryos appear slow or indeed abnormal. Beside leading to the slowness of the otolith development, the ASWF of the AL also postpones the development of the cerebellar primordium in the early zebrafish embryo. In the control group, observed at 24 phf, the development of the cerebellum primordium has been completed and the edge of the tissue is clear and it is possible to distinguish between the midbrain and hindbrain in the control group. However, after treatment at 1, 4 and 8 hpf, the edge of cerebellum primordium appears as a blur, even showing no growth and the midbrain and hindbrain did not separate completely. Thus, the development of the nervous system is affected, which results in the frequency of autonomous motion being abnormal and this has indicated that the ASWF of the AL applied creates a certain neurotoxin to early zebrafish embryo.27,28 Additionally, the ASWF of the AL leads to the bending of the zebrafish bodies, as the bone and articulate matter are sensitive to gravity, in the normal processes of embryo development. What is seen here can be associated with the absence of gravity in the processes which are undertaken under the presence of the AL treatment.29
The effects of ASWF of the AL approach on the development of zebrafish embryos can be understood according to an analysis of the naturally stages of the growth progress. After fertilization, the zebrafish eggs enter the blastula stage (t ≤ 5.25 phf). During this period, the development is much more sensitive to any stimulation from the environment and any disturbance or influence from the outside world can affect the growth of embryo considerably.30,31 The acute toxicity, caused by the use of AL, is much greater in the experimental groups at 1 and 4 hpf (compared to the control group), resulting in a high mortality. With the progress of their development, zebrafish embryos enter the gastrula stage at 5.25–10 hpf. In this period, the epiboly motions produce the original layer and the hypocotyl; connection between the cells occurs and the interaction of the cells appears; cell metabolism changes and the cells began to move; eventually tissues and organs are formed. At this time, the embryo is also sensitive to environmental stimuli. The mortality of the embryos in the 8 hpf groups is reduced drastically compared to that of the 1 and 4 hpf groups, while the hatching rate is still low. When the time reaches 10–24 hpf, the somite of the zebrafish embryo appears and organ primordium, tail bud, cerebellum primordial and brain beam are also observed. Two otoliths have appeared clearly in the ear and the development of the nervous system is essentially completed and the random, voluntary movement arises.
Conclusions
In summary, utilizing zebrafish as a model, the influence of the acoustic standing wave field (ASWF) creating acoustic levitation (AL), on each development stage of early zebrafish embryos has been studied successfully. The work has shown that the ASWF creating the AL results in a slight toxicity which influences the development of the early period of the zebrafish embryos, but that this effect is reduced in the later development period. An important observation is that the work has shown that this technology has high level of biocompatibility.
Conflicts of interest
There are no conflicts to declare.
Acknowledgements
This work was supported by the National Natural Science Foundation of China (31701296, 51675140), Open Project of State Key Laboratory of Urban Water Resources and Water Environment, Harbin Institute of Technology (No. ES201914).
Notes and references
- N. Pérez, M. A. B. Andrade and R. Canetti, et al., Experimental determination of the dynamics of an acoustically levitated sphere, J. Appl. Phys., 2014, 116(18), 184903 Search PubMed.
- J. Weber, C. Rey and J. Neuefeind, et al., Acoustic levitator for structure measurements on low temperature liquid droplets, Rev. Sci. Instrum., 2009, 80(8), 083904 Search PubMed.
- Z. Hong, W. Xie and B. Wei, Acoustic levitation with self-adaptive flexible reflectors, Rev. Sci. Instrum., 2011, 82(7), 074904 Search PubMed.
- E. Trinh and R. E. Apfel, Sound velocity of supercooled water down to −33 °C using acoustic levitation, Chem. Phys., 1980, 72(12), 6731–6735 Search PubMed.
- M. Sundvik, H. Nieminen, A. Salmi, P. Panula and E. Haeggstrom, Effects of acoustic levitation on the development of zebrafish, Danio rerio, embryos, Sci. Rep., 2015, 5, 13596 Search PubMed.
- E. Trinh, J. Robey and N. Jacobi, et al., Dual-temperature acoustic levitation and sample transport apparatus, J. Acoust. Soc. Am., 1986, 79(3), 604–612 Search PubMed.
- T. Kozuka, K. Yasui and T. Tuziuti, et al., Noncontact acoustic manipulation in air, Jpn. J. Appl. Phys., 2007, 46(7S), 4948 Search PubMed.
- H. Dong, L. Jia, Y. Guan, et al., Experiments and simulations of the standing wave acoustic field produced by two transducers mounted in contraposition, Proceedings of Meetings on Acoustics ICU, 2017, p. 065002 Search PubMed.
- J.-K. Park and P. I. Ro, Noncontact manipulation of light objects based on parameter modulations of acoustic pressure nodes, J. Vib. Acoust., 2013, 135(3), 031011 Search PubMed.
- D. Foresti, M. Nabavi and M. Klingauf, et al., Acoustophoretic contactless transport and handling of matter in air, Proc. Natl. Acad. Sci. U. S. A., 2013, 110(31), 12549–12554 Search PubMed.
- T. Hoshi, Y. Ochiai and J. Rekimoto, Three-dimensional noncontact manipulation by opposite ultrasonic phased arrays, Jpn. J. Appl. Phys., 2014, 53(7S), 07KE07 Search PubMed.
- Y. Ito, D. Koyama and K. Nakamura, High-speed noncontact ultrasonic transport of small objects using acoustic traveling wave field, Acoust. Sci. Technol., 2010, 31(6), 420–422 Search PubMed.
- M. Ding, D. Koyama and K. Nakamura, Noncontact ultrasonic transport of liquid using a flexural vibration plate, Appl. Phys. Express, 2012, 5(9), 097301 Search PubMed.
- A. Dazzi, R. Prazeres and F. Glotin, et al., Local infrared microspectroscopy with subwavelength spatial resolution with an atomic force microscope tip used as a photothermal sensor, Opt. Lett., 2005, 30(18), 2388–2390 Search PubMed.
- J. A. Baird, B. Van Eerdebrugh and L. S. Taylor, A classification system to assess the crystallization tendency of organic molecules from undercooled melts, J. Pharm. Sci., 2010, 99(9), 3787–3806 Search PubMed.
- C. J. Benmore and J. K. R. Weber, Amorphization of molecular liquids of pharmaceutical drugs by acoustic levitation, Phys. Rev. X, 2011, 1(1), 011004 Search PubMed.
- Z. Hong, C. Cao, B. Wei, W. Xie and Y. Lü, Acoustic method for levitation of small living animals, Appl. Phys. Lett., 2006, 89(21), 214102 Search PubMed.
- J. Kanungo, E. Cuevas, S. F. Ali and M. G. Paule, Zebrafish Model in Drug Safety Assessment, Curr. Pharm. Des., 2014, 20(34), 5416–5429 Search PubMed.
- H. Bai, W. Kong, C. Shao, Y. Li, Y. Liu and M. Liu, et al., Zebrafish Embryo Toxicity Microscale Model for Ichthyotoxicity Evaluation of Marine Natural Products, Mar. Biotechnol., 2016, 18(2), 264–270 Search PubMed.
- R. A. Marshall and D. P. Osborn, Zebrafish: a vertebrate tool for studying basal body biogenesis, structure, and function, Cilia, 2016, 5(1), 16 Search PubMed.
- J. He, J. Gao, C. Huang and C. Li, Zebrafish models for assessing developmental and reproductive toxicity, Neurotoxicol. Teratol., 2014, 42, 35–42 Search PubMed.
- Y. Nishimura, S. Murakami and Y. Ashikawa, et al., Zebrafish as a systems toxicology model for developmental neurotoxicity testing, Congenital Anomalies, 2015, 55(1), 1 Search PubMed.
- X. Guo, M. Dumasa and B. L. Robinsona, et al., Acetyl L-carnitine targets adenosine triphosphate synthase in protecting zebrafish embryos from toxicities induced byverapamil and ketamine: An in vivo assessment, J. Appl. Toxicol., 2017, 37(2), 192–200 Search PubMed.
- Y. W. Lundberg, Y. Xu, K. D. Thiessen and K. L. Kramer, Mechanisms of otoconia and otolith development, Dev. Dyn., 2015, 244(3), 239–253 Search PubMed.
- Y. Neo, L. Parie and F. Bakker, et al., Behavioral changes in response to sound exposure and no spatial avoidance of noisy conditions in captive zebrafish, Front. Behav. Neurosci., 2015, 9, 28 Search PubMed.
- P. Pais-Roldán, A. P. Singh, H. Schulz and X. Yu, High magnetic field induced otolith fusion in the zebrafish larvae, Sci. Rep., 2016, 6, 24151 Search PubMed.
- M. Hashimoto and M. Hibi, Development and evolution of cerebellar neural circuits, Dev., Growth Differ., 2012, 54(3), 373–389 Search PubMed.
- T. Butts, M. S. Modrell, C. V. H. Baker and R. J. T. Wingate, The evolution of the vertebrate cerebellum: absence of a proliferative external granule layer in a non-teleost ray-finned fish: Vertebratecerebellum evolution, Evol. Dev., 2014, 16(2), 92–100 Search PubMed.
- S. Edsall and T. Franz-Odendaal, An Assessment of the Long-Term Effects of Simulated Microgravity on Cranial Neural Crest Cells in Zebrafish Embryos with a Focus on the Adult Skeleton, PLoS One, 2014, 9(2), e89296 Search PubMed.
- Z. Xiao, D. Yu, X. Zhang, S. Xu, D. Ma and J. Li, The biological study on early development of streak star plaice—morphology and ecology of fertilized eggs and growth characteristics of eggs and embryo, Mar. Sci., 2008, 32(2), 17–21 Search PubMed.
- C. B. Kimmel, W. W. Ballard, S. R. Kimmel, B. Ullmann and T. F. Schilling, Stages of embryonic development of the zebrafish, Dev. Dyn., 1995, 203(3), 253–310 Search PubMed.
Footnote |
† Electronic supplementary information (ESI) available. See DOI: 10.1039/d0ra07344j |
|
This journal is © The Royal Society of Chemistry 2020 |
Click here to see how this site uses Cookies. View our privacy policy here.