DOI:
10.1039/D0RA07093A
(Paper)
RSC Adv., 2020,
10, 37202-37208
α-Hydroxy acid as an aldehyde surrogate: metal-free synthesis of pyrrolo[1,2-a]quinoxalines, quinazolinones, and other N-heterocycles via decarboxylative oxidative annulation reaction†
Received
18th August 2020
, Accepted 4th October 2020
First published on 7th October 2020
Abstract
A metal-free and efficient procedure for the synthesis of pyrrolo[1,2-a]quinoxalines, quinazolinones, and indolo[1,2-a]quinoxaline has been developed. The key features of our method include the in situ generation of aldehyde from α-hydroxy acid in the presence of TBHP (tert-butyl hydrogen peroxide), and further condensation with various amines, followed by intramolecular cyclization and subsequent oxidation to afford the corresponding quinoxalines, quinazolinones derivatives in moderate to high yields.
Introduction
Pyrrolo[1,2-a]quinoxaline motifs are shown in a large number of natural products as well as in several molecules under clinical trials (Fig. 1). For example, the pyrrolo[1,2-a]quinoxaline core system is extensively utilized in pharmaceuticals due to its potential biological activities, including anti-bacterial, anti-infective, anti-inflammatory, anti-HIV, anti-malarial, 5-HTR affinity etc.1 Furthermore, some of these derivatives have been utilized in electronic and optical fields.2 Due to their structural prevalence, significant efforts have been devoted, and several synthetic strategies have been developed for the construction of these compounds.3–5 Conventionally, 2-(1H-pyrrol-1-yl)aniline (1a) has been used as a starting material and is condensed with aldehydes4e,f,m,n,p–s or its equivalents to form the pyrrolo[1,2-a]quinoxaline derivatives.4
 |
| Fig. 1 Compounds containing pyrrolo[1,2-a]quinoxalines and quinazolinones. | |
However, the existing methods suffer from several limitations in that they require tedious synthetic procedures, expensive transition metal catalysts, stoichiometric reagents, elevated temperatures, toxic solvents, and catalyst, etc. In addition to that, aldehydes are prone to convert into acids by aerial oxidation, which may cause decarbonylation, to avoid formation of additional side products inert condition required to carry out the experiment (Scheme 1).6 Thus, it would be highly desirable to develop an environmentally benign method to synthesis of quinoxalines and its related derivatives.
 |
| Scheme 1 Previous and our approaches. | |
Similarly, quinazolinone plays a major role in drugs and exhibits potential biological activities.7 The substituted quinazolinones are associated with a range of biological and pharmacological activities such as an anti-inflammatory, anti-cancer, anti-fungal, anti-bacterial, etc. Additionally, numerous natural products contain the quinazolinone core unit, for example, L-Vasicinone, Rutaecarpine, Luotonin E, Bouchardatine, Tryptanthrin, and Methaqualone (Fig. 1).8 Vast number of diverse methodologies has been developed during the past decades for the synthesis of these compounds.9,10
Decarboxylative coupling reaction has recently emerged as a green method to construct C–C bonds, in various reactions such as Heck, cross coupling, oxidative arylation, allylations, etc.,11 However, most of the reactions utilized metal catalysts.12 Due to expensive and toxicity of metal catalyst, it is highly required to design an environmentally benign method. Few reports were utilized the α-hydroxy acids as an aldehyde equivalent.13 To the best of our knowledge there is no report related the direct synthesis of quinoxalines from α-hydroxy acids as an aldehyde surrogate. In our efforts to develop privileged heterocycles via metal-free approaches,14 herein we disclose a method to synthesize pyrrolo[1,2-a]quinoxalines and quinazolinones by utilizing α-hydroxy acids as aldehyde equivalent in the presence of TBHP.
Results and discussion
In our preliminary investigation, we selected 2-(1H-pyrrol-1-yl)aniline (1a, 1.0 equiv.) and lactic acid (2a, 5.0 equiv.) as model substrates to optimize the reaction condition, and the results are summarized in Table 1. By using FeCl3 (30 mol%) as the catalyst and tert-butyl hydrogen peroxide (TBHP, 5.0 equiv., 70% in H2O) as the oxidant in chloroform at 70 °C under the oxygen atmosphere, the corresponding pyrrolo[1,2-a]quinoxaline (3a) obtained in 23% yield (Table 1, entry 1).4l Replacing the catalyst to CuSO4 given the similar result (entry 2). Surprisingly, absence of a metal catalyst successfully generated the expected product 3a in 28% yield (entry 3). Furthermore, the product yield was increased to 52% when we, switched the solvent into dichloroethane (DCE, entry 4).
Table 1 Optimization of reaction conditionsa

|
S. no. |
Solvent |
Temp. (°C) |
Oxidant (5.0 equiv.) |
Time (h) |
Yieldb (%) |
Reaction conditions: 2-(1H-pyrrol-1-yl)aniline 1a (1.0 equiv.), lactic acid 2a (5.0 equiv.), solvent 2.0 mL, stirred at mentioned temperature. Isolated yield. 30 mol% FeCl3 used as a catalyst. 30 mol% CuSO4 used as a catalyst. Performed under oxygen atm. 4.0 equiv. of TBHP (tert-butyl hydrogen peroxide) used, DTBP: di-tert-butylperoxide, DCP: dicumyl peroxide, NR = no reaction, SR = side reaction. |
1c |
CHCl3 |
80 |
TBHP |
20 |
23e |
2d |
CHCl3 |
80 |
TBHP |
20 |
24e |
3 |
CHCl3 |
80 |
TBHP |
12 |
28e |
4 |
DCE |
70 |
TBHP |
12 |
52 |
5 |
DCE |
70 |
Oxone |
36 |
NR |
6 |
DCE |
70 |
Na2S2O8 |
36 |
NR |
7 |
DCE |
70 |
K2S2O8 |
36 |
NR |
8 |
DCE |
70 |
H2O2 |
24 |
48 |
9 |
DCE |
70 |
DTBP |
24 |
<10 |
10 |
DCE |
70 |
DCP |
24 |
37 |
11 |
DCE |
80 |
TBHP |
12 |
63 |
12 |
DCE |
80 |
TBHPf |
12 |
76 |
13 |
DMSO |
80 |
TBHPf |
24 |
<10 |
14 |
H2O |
80 |
TBHPf |
24 |
30 |
15 |
THF |
80 |
TBHPf |
24 |
NR |
16 |
Toluene |
80 |
TBHPf |
24 |
33 |
17 |
Acetone |
80 |
TBHPf |
12 |
SR |
18 |
DCE |
90 |
TBHPf |
10 |
71 |
These above results encouraged us to screen various types of oxidants such as Oxone, sodium persulfate, and potassium persulfate, which unfortunately failed to form the desired product (entries 5–7). Similarly, replacing TBHP with other peroxide oxidants led to catalyze the reaction, albeit with slightly lower yields (entries 8–10). Gratifyingly, decreasing the equivalence of TBHP to 4.0 equiv. and increasing the temperature to 80 °C, 3a was obtained in 76% yield (entry 12). To improve the yield of the product, the reaction was screened with various solvents such as DMSO, water, THF, toluene, and acetone (entries 13–17). But, unfortunately, other solvents failed to improve the yield of the reaction. Altering the temperature led to slightly lower the yield (entry 18). We concluded that the optimized conditions for the decarboxylative condensation followed by annulation reaction was 1a (1.0 equiv.), 2a (5.0 equiv.), and TBHP (4.0 equiv.) in DCE at 80 °C.
With the optimized reaction conditions in hand, we expanded the scope of the reaction and with various other hydroxyl acids and amine derivatives (Table 2). Different α-hydroxy acids were investigated with 1a and equivalent products were obtained in moderate to good yields. When we moved from aliphatic equivalent to aryl equivalent; (here the mandelic acid, equivalent of benzaldehyde) was tolerated and furnished the expected product (3b) in good yield (56%).3c Likewise, aliphatic substituted hydroxy acids afforded the pyrrolo[1,2-a]quinoxaline derivatives in good yields. 2-Methyl-2-hydroxypropionic acid (2-methyllactic acid) equivalent of acetone, 2-methyl-2-hydroxybutanoic acid equivalent of 2-butanone underwent the reaction smoothly and provided the expected products (3c & 3d) in moderate yields.5b,d
Table 2 Synthesis of pyrrolo[1,2-a]quinoxaline derivativesa
Reaction conditions: all the reactions were performed using SM (1.0 equiv.), α-hydroxy acid (5.0 equiv.), TBHP (4.0 equiv.), and DCE (2.0 mL) stirred at 80 °C. |
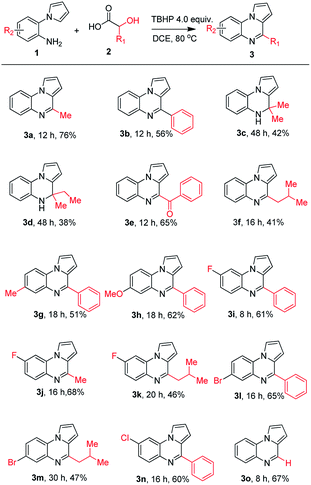 |
Interestingly, phenyllactic acid also produced the acetophenone derivative (3e, 65%) in good yield.4o This may be because benzylic protons are over-oxidized by TBHP. Moreover, the reaction of amine 1a with leucic acid is an equivalent of 3-methylbutanal, smoothly underwent the reaction generated the corresponding product 3f in moderate yield.4h
Later, we investigated various substituted aniline derivatives. Substrate containing methyl, and methoxy substituted aniline derivatives were compatible with this transformation and produced the respective products 3g, 3h in good yields.3c It was found that aniline containing the electron-withdrawing groups such as F, Br, and Cl could be amenable to the reaction, providing their equivalent products in good yields. Fluoro substituted amine was well tolerated under the present condition and afforded the corresponding quinoxalines in good yields. Both mandelic and lactic acid are furnishing the desired products 3i, 3j in good yields.3c,5a To synthesis products of 3a, and 3j acetaldehyde surrogate is required and acetaldehyde difficult to handle due to polymerization. Also, some of the reported methods are required either very high temperature, metal catalyst or strong acidic condition and so on.
Leucic acid also reacted smoothly and provided the final product 3k in moderate yield. Similarly, both Br and Cl substituents furnish the corresponding products (3l–n) in good yields under the standard reaction condition.5b In general, isovaleraldehyde is required to condense with 2-pyrrolyl aniline to obtain the products 3f, 3k, and 3m. But only one dihydro derivative was synthesized by using the above aldehyde.5d On the other hand, only product 3f was previously synthesized by using equivalent amino acid (leucine).4h Besides, glycolic acid, which was used as a one-carbon source, also proven to be appropriate candidate, reacted quite well under the optimal reaction condition, and provided pyrroloquinoxaline 3o in very good yield (67%).4a
Subsequently, we have expanded the scope of the decarboxylative condensation reaction with various anthranilamide derivatives and different α-hydroxy acids to synthesize quinazolinone derivatives. As shown in Table 3, we found that various functionalized anthranilamides successfully underwent this decarboxylative condensation to afford the expected products in good to excellent yields. For example, mandelic acid with anthranilamide produced the target product 5a in excellent yield (84%).10a Substrates bearing N-benzyl efficiently engaged and produced the products 5b–c in good yields.9z,10b A wide range 2-aminophenylbenzamides were synthesized which are compatible with standard conditions. Likewise, 2-amino-N-phenyl benzamide reacts with mandelic acid to generate the corresponding product 5d in 69% yield.10i
Table 3 Synthesis of quinazoline derivativesa
Reaction conditions: all the reactions were performed using SM (1.0 equiv.), α-hydroxy acid (5.0 equiv.), TBHP (4.0 equiv.), and DCE (2.0 mL) in stirred at 80 °C. |
 |
Further, similar results were obtained with phenyl ring containing methyl, methoxy, acetyl, dimethyl substituted anthranilamides which react with mandelic acid to afford expected quinazolinone derivatives (5e–i) in good yields.10e,i,j Also, N-p-tolyl-anthranilamide reacts with lactic acid to furnish the corresponding product 5i in high yield as 81%.10g Besides, cyclohexyl, ethyl cyclohexene, ethylphenyl, trifluoroethyl protected amides also provided expected products (5j–m) in good yields.10c,f,h Similarly, the chloro substituted anthranilamide ring also delivered the product 5n in moderate yield.10d Moreover, we applied the optimal condition to glycolic acid, which reacted with various 2-aminobenzamides to provide the expected quinazolinone products (5o–q) in good yields.14b To synthesis of substituted quinazoline derivatives such as 5o, 5p, and 5q, the reported methods requires harsh reaction conditions such as higher temperature, toxic solvent, longer reaction time, and so on.
After investigating the scope with various anthranilamides, we next tested the generality of the decarboxylative condensation reaction with various amine partners (Scheme 2). For example, the 2-aminobenzenesulphonamide with mandelic acid produced the target sulfonamide condensed product 6 with moderate yield.9x The reaction between 2-(1H-indol-1-yl)aniline and mandelic acid under the optimized reaction condition produced indolo[1,2-a]quinoxaline (7) albeit in lower yield.5b Besides, under the standard conditions, we synthesized one of the best-selling sedative-hypnotic drug Methaqualone (8), which was obtained by the reaction between suitable substituted anthranilamide with lactic acid, which acts as an acetaldehyde equivalent to provide the natural product Methaqualone 8 in 63% yield.14b
 |
| Scheme 2 Synthesis of other derivatives. | |
To gain more insight into the reaction mechanism, we have carried out the control experiments shown in Scheme 3. Mixing the reaction with the radical quencher TMEPO (15.0 equiv.) given the 5b in less than 10% yield. Other radical scavengers such as BHT (15.0 equiv.) & 1,1-diphenylethylene (15.0 equiv.) both are completely inhibiting the reaction and less than 5% product only obtained. The above radical scavenger experiments reveal that the reaction occurs via radical formation.
 |
| Scheme 3 Control experiments. | |
Based on the experimental results, the possible mechanism for the formation of quinazolinone is depicted in Scheme 4. Initially, TBHP is fragmented into a t-butoxide radical and a hydroxyl radical. Then, the hydroxyl radical reacts with α-hydroxy acids to form the aldehyde equivalent I by the elimination of both CO2 and H2O. Then, the amine 4 reacts with I to afford the imine intermediate II via condensation with t-butoxide radical and yields water as a side product. The dihydro aminal III is generated from II, through intramolecular cyclization and product 5 is formed by oxidation.
 |
| Scheme 4 Possible reaction mechanism. | |
Conclusion
We have developed a TBHP mediated, mild, and efficient approach to synthesis of various quinoxalines, quinazolinones, and indoloquinoxaline derivatives via decarboxylation followed by condensation using α-hydroxy acids as carbonyl source. The reaction proceeds in the absence of metal catalyst to afford the expected products in moderate to high yields. A series of substituted 2-amino benzamides are well suited in this process. Based on the control experimental results, a possible reaction mechanism suggests, the reaction occurs via radical mechanism. By utilizing this methodology, we have synthesized the bioactive natural product (Methaqualone) in good yield.
Experimental section
General information
1H NMR spectra were recorded on a Jeol RESONANCE ECZ 400S (400 MHz). Chemical shifts are reported in ppm from tetramethylsilane (TMS) with the solvent resonance resulting from incomplete deuteration as the internal reference (CDCl3: 7.26 ppm) or relative to TMS (δ 0.0). Data are reported as follows: chemical shift, multiplicity (s = singlet, d = doublet, t = triplet, q = quartet, br = broad, m = multiplet, dd = doublet of doublet, td = triplet of doublet), coupling constants (Hz), number of protons. 13C NMR spectra were recorded on a Jeol RESONANCE ECZ 400S (100 MHz) with complete proton decoupling. Chemical shifts are reported in ppm from tetramethylsilane with the solvent as the internal reference (CDCl3: 77.16 ppm). High-resolution mass spectrometry was performed with on LCQ Fleet-Thermo Scientifics. All reactant or reagent was purchased from Aldrich, TCI, Alfa Aesar and Acros, and were directly used without further purifications. Silica gel column chromatography was performed with Silica Gel of Kieselgel 60 F254 plate (Merck).
General procedure for the synthesis of heterocyclic compounds
A solution of 2-(1H-pyrrol-1-yl)anilines (1.0 equiv.) or 2-aminobenzamides (1.0 equiv.), α-hydroxy acids (5.0 equiv.), TBHP (70% in H2O, 4.0 equiv.) and DCE (2.0 mL) was stirred at 80 °C for particular times (see the individual substrates at the manuscript). The reaction progress was monitored by TLC. Then, 10 mL of NH4Cl was added to the reaction mixture and extracted with EtOAc (15 mL × 3). The combined organic fractions were dried over MgSO4, filtered, and concentrated under reduced pressure. The crude residue was then purified by flash column chromatography on silica gel by using hexanes/ethyl acetate as eluent to afford the pure product.
Conflicts of interest
There are no conflicts to declare.
Acknowledgements
This work was supported by the National Research Foundation of Korea grants funded by the Korea Government (MSIT) (MRC 2017R1A5A2015541, and 2020R1A2C1007346). This work was also supported by the Post-doctoral Fellowship program funded by the Ministry of Education of the Republic of Korea through the Chungbuk National University in 2018 (M. V.).
Notes and references
-
(a) J. Guillon, M. Le Borgne, C. Rimbault, S. Moreau, S. Savrimoutou, N. Pinaud, S. Baratin, M. Marchivie, S. Roche, A. Bollacke, A. Pecci, L. Alvarez, V. Desplat and J. Jose, Eur. J. Med. Chem., 2013, 65, 205–222 CrossRef CAS;
(b) J. Guillon, E. Mouray, S. Moreau, C. Mullié, I. Forfar, V. Desplat, S. Belisle-Fabre, N. Pinaud, F. Ravanello, A. Le-Naour, J.-M. Léger, G. Gosmann, C. Jarry, G. Déléris, P. Sonnet and P. Grellier, Eur. J. Med. Chem., 2011, 46, 2310–2326 CrossRef CAS;
(c) V. Desplat, S. Moreau, A. Gay, S. B. Fabre, D. Thiolat, S. Massip, G. Macky, F. Godde, D. Mossalayi, C. Jarry and J. Guillon, J. Enzyme Inhib. Med. Chem., 2010, 25, 204–215 CrossRef CAS;
(d) V. Desplat, A. Geneste, M.-A. Begorre, S. B. Fabre, S. Brajot, S. Massip, D. Thiolat, D. Mossalayi, C. Jarry and J. Guillon, J. Enzyme Inhib. Med. Chem., 2008, 23, 648–658 CrossRef CAS;
(e) G. Maga, S. Gemma, C. Fattorusso, G. A. Locatelli, S. Butini, M. Persico, G. Kukreja, M. P. Romano, L. Chiasserini, L. Savini, E. Novellino, V. Nacci, S. Spadari and G. Campiani, Biochemistry, 2005, 44, 9637–9644 CrossRef CAS;
(f) J. Guillon, P. Grellier, M. Labaied, P. Sonnet, J.-M. Léger, R. Déprez-Poulain, I. Forfar-Bares, P. Dallemagne, N. Lemaître, F. Péhourcq, J. Rochette, C. Sergheraert and C. Jarry, J. Med. Chem., 2004, 47, 1997–2009 CrossRef CAS.
-
(a) C. Tekuri, D. K. Singh and M. Nath, Dyes Pigm., 2016, 132, 194–203 CrossRef CAS;
(b) B. B. Carbas, A. Kivrak, M. Zora and A. M. Önal, Electroanal. Chem., 2012, 677–680, 9–14 CrossRef CAS;
(c) B. B. Çarbaş, A. Kivrak, M. Zora and A. M. Önal, React. Funct. Polym., 2011, 71, 579–587 CrossRef.
-
(a) E. Y. Zelina, T. A. Nevolina, L. N. Sorotskaja, D. A. Skvortsov, I. V. Trushkov and M. G. Uchuskin, Tetrahedron Lett., 2020, 61, 151532 CrossRef CAS;
(b) T. A. To, C. T. Nguyen, M. H. P. Tran, T. Q. Huynh, T. T. Nguyen, N. T. H. Le, A. D. Nguyen, P. D. Tran and N. T. S. Phan, J. Catal., 2019, 377, 163–173 CrossRef CAS;
(c) Q. Sun, L. Liu, Y. Yang, Z. Zha and Z. Wang, Chin. Chem. Lett., 2019, 30, 1379–1382 CrossRef CAS;
(d) A. A. Kalinin, L. N. Islamova and G. M. Fazleeva, Chem. Heterocycl. Compd., 2019, 55, 584–597 CrossRef CAS;
(e) A. Keivanloo, A. Soozani, M. Bakherad, M. Mirzaee, H. A. Rudbari and G. Bruno, Tetrahedron, 2017, 73, 1633–1639 CrossRef CAS;
(f) M. Wang, C. Liu and Y. Gu, Tetrahedron, 2016, 72, 6854–6865 CrossRef CAS;
(g) A. Keivanloo, S. S. Kazemi, H. Nasr-Isfahani and A. Bamoniri, Tetrahedron, 2016, 72, 6536–6542 CrossRef CAS;
(h) Z. Zhang, J. Li, G. Zhang, N. Ma, Q. Liu and T. Liu, J. Org. Chem., 2015, 80, 6875–6884 CrossRef CAS;
(i) H. Liu, T. Duan, Z. Zhang, C. Xie and C. Ma, Org. Lett., 2015, 17, 2932–2935 CrossRef CAS;
(j) M. De Fatima Pereira and V. Thiéry, Org. Lett., 2012, 14, 4754–4757 CrossRef;
(k) G. Liu, Y. Zhou, D. Lin, J. Wang, L. Zhang, H. Jiang and H. Liu, ACS Comb. Sci., 2011, 13, 209–213 CrossRef CAS;
(l) A. Huang, F. Liu, C. Zhan, Y. Liu and C. Ma, Org. Biomol. Chem., 2011, 9, 7351–7357 RSC.
-
(a) X. Wang, H. Liu, C. Xie, F. Zhou and C. Ma, New J. Chem., 2020, 44, 2465–2470 RSC;
(b) B. N. Patil, J. J. Lade, S. D. Pardeshi, P. Patil and A. C. Chaskar, ChemistrySelect, 2019, 4, 11362–11366 CrossRef CAS;
(c) S. D. Pardeshi, B. N. Patil, P. Patil and A. C. Chaskar, Tetrahedron Lett., 2019, 60, 151250 CrossRef;
(d) J. Ni, Y. Jiang, Z. Qi and R. Yan, Chem.–Asian J., 2019, 14, 2898–2902 CAS;
(e) T. Krishna, T. N. Reddy, E. Laxminarayana and D. Kalita, ChemistrySelect, 2019, 4, 250–253 CrossRef CAS;
(f) H.-R. Huo, X.-Y. Tang and Y.-f. Gong, Synthesis, 2018, 50, 2727–2740 CrossRef CAS;
(g) G. S. Mani, A. V. S. Rao, Y. Tangella, S. Sunkari, F. Sultana, H. K. Namballa, N. Shankaraiah and A. Kamal, New J. Chem., 2018, 42, 15820–15829 RSC;
(h) H. Liu, F. Zhou, W. Luo, Y. Chen, C. Zhang and C. Ma, Org. Biomol. Chem., 2017, 15, 7157–7164 RSC;
(i) J. J. Lade, B. N. Patil, M. V. Vhatkar, K. S. Vadagaonkar and A. C. Chaskar, Asian J. Org. Chem., 2017, 6, 1579–1583 CrossRef CAS;
(j) J. J. Lade, B. N. Patil, P. A. Sathe, K. S. Vadagaonkar, P. Chetti and A. C. Chaskar, ChemistrySelect, 2017, 2, 6811–6817 CrossRef CAS;
(k) C. Dai, S. Deng, Q. Zhu and X. Tang, RSC Adv., 2017, 7, 44132–44135 RSC;
(l) Z. An, L. Zhao, M. Wu, J. Ni, Z. Qi, G. Yu and R. Yan, Chem. Commun., 2017, 53, 11572–11575 RSC;
(m) Y. Wang, L. Cui, Y. Wang and Z. Zhou, Tetrahedron: Asymmetry, 2016, 27, 85–90 CrossRef CAS;
(n) C. Wang, Y. Li, J. Zhao, B. Cheng, H. Wang and H. Zhai, Tetrahedron Lett., 2016, 57, 3908–3911 CrossRef CAS;
(o) Z. Zhang, C. Xie, X. Tan, G. Song, L. Wen, H. Gao and C. Ma, Org. Chem. Front., 2015, 2, 942–946 RSC;
(p) A. Preetam and M. Nath, RSC Adv., 2015, 5, 21843–21853 RSC;
(q) A. Kamal, K. S. Babu, J. Kovvuri, V. Manasa, A. Ravikumar and A. Alarifi, Tetrahedron Lett., 2015, 56, 7012–7015 CrossRef CAS;
(r) P. N. M. Allan, M. I. Ostrowska and B. Patel, Synlett, 2019, 30, 2148–2152 CrossRef CAS;
(s) A. K. Verma, R. R. Jha, V. K. Sankar, T. Aggarwal, R. P. Singh and R. Chandra, Eur. J. Org. Chem., 2011, 2011, 6998–7010 CrossRef CAS.
-
(a) J. Li, J. Zhang, H. Yang, Z. Gao and G. Jiang, J. Org. Chem., 2017, 82, 765–769 CrossRef CAS;
(b) R. Rubio-Presa, M. a. R. Pedrosa, M. A. Fernández-Rodríguez, F. J. Arnáiz and R. Sanz, Org. Lett., 2017, 19, 5470–5473 CrossRef CAS;
(c) C. S. Yi and S. Y. Yun, J. Am. Chem. Soc., 2005, 127, 17000–17006 CrossRef CAS;
(d) D. Uraguchi, H. Sasaki, Y. Kimura, T. Ito and T. Ooi, J. Am. Chem. Soc., 2018, 140, 2765–2768 CrossRef CAS.
-
(a) G. V. R. Sharma and A. R. Robert, Res. Chem. Intermed., 2013, 39, 3251–3254 CrossRef CAS;
(b) A. Modak, A. Deb, T. Patra, S. Rana, S. Maity and D. Maiti, Chem. Commun., 2012, 48, 4253–4255 RSC;
(c) T. Iwai, T. Fujihara and Y. Tsuji, Chem. Commun., 2008, 6215–6217 RSC.
-
(a) D. S. Reddy and A. G. Kutateladze, Org. Lett., 2019, 21, 2855–2858 CrossRef CAS;
(b) Y. Li, J. Xiao, Q. Zhang, W. Yu, M. Liu, Y. Guo, J. He and Y. Liu, Bioorg. Med. Chem., 2019, 27, 568–577 CrossRef CAS;
(c) S. Gatadi, T. V. Lakshmi and S. Nanduri, Eur. J. Med. Chem., 2019, 170, 157–172 CrossRef CAS;
(d) A. A. El-Sayed, M. F. Ismail, A. E. G. E. Amr and A. M. Naglah, Molecules, 2019, 24, 3787 CrossRef;
(e) T. M. M. Maiden and J. P. A. Harrity, Org. Biomol. Chem., 2016, 14, 8014–8025 RSC;
(f) I. Khan, A. Ibrar, W. Ahmed and A. Saeed, Eur. J. Med. Chem., 2015, 90, 124–169 CrossRef CAS.
-
(a) Y. Zhang, T. Yan, D. Sun, C. Xie, T. Wang, X. Liu, J. Wang, Q. Wang, Y. Luo, P. Wang, T. Yagai, K. W. Krausz, X. Yang and F. J. Gonzalez, Free Radical Biol. Med., 2020, 148, 33–41 CrossRef CAS;
(b) Y. C. Tsai, C. L. Lee, H. R. Yen, Y. S. Chang, Y. P. Lin, S. H. Huang and C. W. Lin, Biomolecules, 2020, 10, 366 CrossRef CAS;
(c) J. Hesse-Macabata, B. Morgner, P. Elsner, U. C. Hipler and C. Wiegand, Sci.
Rep., 2020, 10, 1863 CrossRef CAS;
(d) J. Ma, L. Chen, J. Fan, W. Cao, G. Zeng, Y. Wang, Y. Li, Y. Zhou and X. Deng, Eur. J. Med. Chem., 2019, 168, 146–153 CrossRef CAS;
(e) I. Khan, S. Zaib, S. Batool, N. Abbas, Z. Ashraf, J. Iqbal and A. Saeed, Bioorg. Med. Chem., 2016, 24, 2361–2381 CrossRef CAS;
(f) U. A. Kshirsagar, Org. Biomol. Chem., 2015, 13, 9336–9352 RSC;
(g) H. Hammer, B. M. Bader, C. Ehnert, C. Bundgaard, L. Bunch, K. Hoestgaard-Jensen, O. H. U. Schroeder, J. F. Bastlund, A. Gramowski-Voß and A. A. Jensen, Mol. Pharmacol., 2015, 88, 401–420 CrossRef CAS;
(h) M. Viji and R. Nagarajan, J. Chem. Sci., 2014, 126, 1075–1080 CrossRef CAS;
(i) S. B. Mhaske and N. P. Argade, Tetrahedron, 2006, 62, 9787–9826 CrossRef CAS;
(j) C. Wattanapiromsakul, P. I. Forster and P. G. Waterman, Phytochemistry, 2003, 64, 609–615 CrossRef CAS.
-
(a) J. W. Collet, E. A. van der Nol, T. R. Roose, B. U. W. Maes, E. Ruijter and R. V. A. Orru, J. Org. Chem., 2020, 85, 7378–7385 CrossRef CAS;
(b) O. J. Turner, D. J. Hirst and J. A. Murphy, Chem.–Eur. J., 2020, 26, 3026–3029 CrossRef CAS;
(c) T. Song, P. Ren, Z. Ma, J. Xiao and Y. Yang, ACS Sustainable Chem. Eng., 2020, 8, 267–277 CrossRef CAS;
(d) L. Xie, C. Lu, D. Jing, X. Ou and K. Zheng, Eur. J. Org. Chem., 2019, 2019, 3649–3653 CrossRef CAS;
(e) Q. Xia, Z. Shi, J. Yuan, Q. Bian, Y. Xu, B. Liu, Y. Huang, X. Yang and H. Xu, Asian J. Org. Chem., 2019, 8, 1933–1941 CrossRef CAS;
(f) Q. Wang, M. Lv, J. Liu, Y. Li, Q. Xu, X. Zhang and H. Cao, ChemSusChem, 2019, 12, 3043–3048 CrossRef CAS;
(g) T. A. To, Y. H. Vo, H. T. T. Nguyen, P. T. M. Ha, S. H. Doan, T. L. H. Doan, S. Li, H. V. Le, T. N. Tu and N. T. S. Phan, J. Catal., 2019, 370, 11–20 CrossRef CAS;
(h) Q.-H. Teng, Y. Sun, Y. Yao, H.-T. Tang, J.-R. Li and Y.-M. Pan, ChemElectroChem, 2019, 6, 3120–3124 CrossRef CAS;
(i) F. A. Sofi, R. Sharma, A. K. Chakraborti and P. V. Bharatam, Eur. J. Org. Chem., 2019, 2019, 5887–5893 CrossRef CAS;
(j) M. Ramanathan, M.-T. Hsu and S.-T. Liu, Tetrahedron, 2019, 75, 791–796 CrossRef CAS;
(k) S. Ram, Shaifali, A. S. Chauhan, Sheetal, A. K. Sharma and P. Das, Chem.–Eur. J., 2019, 25, 14506–14511 CrossRef CAS;
(l) D.-Z. Lin, Y.-L. Lai and J.-M. Huang, ChemElectroChem, 2019, 6, 4188–4193 CrossRef CAS;
(m) Y. Liang, Z. Tan, H. Jiang, Z. Zhu and M. Zhang, Org. Lett., 2019, 21, 4725–4728 CrossRef CAS;
(n) P. T. Kirinde Arachchige and C. S. Yi, Org. Lett., 2019, 21, 3337–3341 CrossRef CAS;
(o) M. A. Iqbal, L. Lu, H. Mehmood, D. M. Khan and R. Hua, ACS Omega, 2019, 4, 8207–8213 CrossRef CAS;
(p) Y. Hu, S. Li, H. Li, Y. Li, J. Li, C. Duanmu and B. Li, Org. Chem. Front., 2019, 6, 2744–2748 RSC;
(q) A. Garia and N. Jain, J. Org. Chem., 2019, 84, 9661–9670 CrossRef CAS;
(r) N. Sayyad, Z. Cele, R. R. Aleti, M. Bera, S. Cherukupalli, B. Chandrasekaran, N. D. Kushwaha and R. Karpoormath, Eur. J. Org. Chem., 2018, 2018, 5382–5388 CrossRef CAS;
(s) J.-B. Peng, H.-Q. Geng, W. Wang, X. Qi, J. Ying and X.-F. Wu, J. Catal., 2018, 365, 10–13 CrossRef CAS;
(t) S. Mukhopadhyay, D. S. Barak and S. Batra, Eur. J. Org. Chem., 2018, 2018, 2784–2794 CrossRef CAS;
(u) W. Liu, G. Wu, W. Gao, J. Ding, X. Huang, M. Liu and H. Wu, Org. Chem. Front., 2018, 5, 2734–2738 RSC;
(v) Z.-Y. Liao, W.-H. Yeh, P.-Y. Liao, Y.-T. Liu, Y.-C. Chen, Y.-H. Chen, T.-H. Hsieh, C.-C. Lin, M.-H. Lu, Y.-S. Chen, M.-C. Hsu, T.-K. Li and T.-C. Chien, Org. Biomol. Chem., 2018, 16, 4482–4494 RSC;
(w) A. V. Dubey and A. V. Kumar, ACS Sustainable Chem. Eng., 2018, 6, 14283–14291 CrossRef CAS;
(x) L. Cao, H. Huo, H. Zeng, Y. Yu, D. Lu and Y. Gong, Adv. Synth. Catal., 2018, 360, 4764–4773 CrossRef CAS;
(y) K. Yamaguchi, S.-i. Kawaguchi, M. Sonoda, S. Tanimori and A. Ogawa, Tetrahedron Lett., 2017, 58, 4043–4047 CrossRef CAS;
(z) W. Phakhodee, S. Wangngae and M. Pattarawarapan, J. Org. Chem., 2017, 82, 8058–8066 CrossRef CAS;
(a
a) T. M. M. Maiden and J. P. A. Harrity, Org. Biomol. Chem., 2016, 14, 8014–8025 RSC.
-
(a) S. Das, S. Sinha, D. Samanta, R. Mondal, G. Chakraborty, P. Brandaõ and N. D. Paul, J. Org. Chem., 2019, 84, 10160–10171 CrossRef CAS;
(b) C. L. Lee, L. Wu, J.-S. Huang and C.-M. Che, Chem. Commun., 2019, 55, 3606–3609 RSC;
(c) S. Maiti, J. Kim, J.-H. Park, D. Nam, J. B. Lee, Y.-J. Kim, J.-M. Kee, J. K. Seo, K. Myung, J.-U. Rohde, W. Choe, O.-H. Kwon and S. Y. Hong, J. Org. Chem., 2019, 84, 6737–6751 CrossRef CAS;
(d) J. Sun, T. Tao, D. Xu, H. Cao, Q. Kong, X. Wang, Y. Liu, J. Zhao, Y. Wang and Y. Pan, Tetrahedron Lett., 2018, 59, 2099–2102 CrossRef CAS;
(e) H. M. Patel, M. N. Noolvi, A. A. Shirkhedkar, A. D. Kulkarni, C. V. Pardeshi and S. J. Surana, RSC Adv., 2016, 6, 44435–44455 RSC;
(f) R. Lingayya, M. Vellakkaran, K. Nagaiah and J. B. Nanubolu, Adv. Synth. Catal., 2016, 358, 81–89 CrossRef CAS;
(g) D. Kumar, P. S. Jadhavar, M. Nautiyal, H. Sharma, P. K. Meena, L. Adane, S. Pancholia and A. K. Chakraborti, RSC Adv., 2015, 5, 30819–30825 RSC;
(h) Y. Feng, Y. Li, G. Cheng, L. Wang and X. Cui, J. Org. Chem., 2015, 80, 7099–7107 CrossRef CAS;
(i) L. Xu, Y. Jiang and D. Ma, Org. Lett., 2012, 14, 1150–1153 CrossRef CAS;
(j) R. Giri, J. K. Lam and J.-Q. Yu, J. Am. Chem. Soc., 2010, 132, 686–693 CrossRef CAS.
-
(a) P. J. Moon and R. J. Lundgren, ACS Catal., 2020, 10, 1742–1753 CrossRef CAS;
(b) M.-C. Liu, W. Liu, H.-Y. Wu, Y.-B. Zhou, Q. Ding and Y. Peng, Org. Chem. Front., 2020, 7, 487–491 RSC;
(c) G. Wu, J. Wang, C. Liu, M. Sun, L. Zhang, Y. Ma, R. Cheng and J. Ye, Org. Chem. Front., 2019, 6, 2245–2249 RSC;
(d) G. Hong, J. Yuan, J. Fu, G. Pan, Z. Wang, L. Yang, Y. Xiao, P. Mao and X. Zhang, Org. Chem. Front., 2019, 6, 1173–1182 RSC;
(e) X. Zhang, X. Feng, C. Zhou, X. Yu, Y. Yamamoto and M. Bao, Org. Lett., 2018, 20, 7095–7099 CrossRef CAS;
(f) P. Liu, G. Zhang and P. Sun, Org. Biomol. Chem., 2016, 14, 10763–10777 RSC.
-
(a) W. Jiang, Y. Zhou, W. Sun and Y. Li, Appl. Organomet. Chem., 2020, 34, e5429 CrossRef CAS;
(b) H.-J. Zhang, Y.-C. Xie and L. Yin, Nat. Commun., 2019, 10, 1699 CrossRef;
(c) Y. Wang, H. Zhao, X. Xie, H. Jiang, H. Deng, J. Hao and W. Wan, Synth. Commun., 2019, 49, 2961–2970 CAS;
(d) L. Ren, M. Ran, J. He, D. Xiang, F. Chen, P. Liu, C. He and Q. Yao, Eur. J. Org. Chem., 2019, 2019, 5656–5661 CrossRef CAS;
(e) T. Pillaiyar, M. Uzair, S. Ullah, G. Schnakenburg and C. E. Müller, Adv. Synth. Catal., 2019, 361, 4286–4293 CrossRef CAS;
(f) Z.-Y. Mao, Y.-W. Liu, R.-J. Ma, J.-L. Ye, C.-M. Si, B.-G. Wei and G.-Q. Lin, Chem. Commun., 2019, 55, 14170–14173 RSC;
(g) J.-Y. Guo, T. Guan, J.-Y. Tao, K. Zhao and T.-P. Loh, Org. Lett., 2019, 21, 8395–8399 CrossRef CAS;
(h) R. A. Daley, E.-C. Liu and J. J. Topczewski, Org. Lett., 2019, 21, 4734–4738 CrossRef CAS;
(i) Q. Song, Q. Feng and M. Zhou, Org. Lett., 2013, 15, 5990–5993 CrossRef CAS;
(j) T. D. Montgomery, Y. Zhu, N. Kagawa and V. H. Rawal, Org. Lett., 2013, 15, 1140–1143 CrossRef CAS;
(k) J. D. Weaver, A. Recio, A. J. Grenning and J. A. Tunge, Chem. Rev., 2011, 111, 1846–1913 CrossRef CAS.
-
(a) A. Li, C. Huang, C.-W. Luo, L.-J. Li, W.-J. Yi, T.-W. Liu and Z.-S. Chao, Catal. Commun., 2017, 98, 13–16 CrossRef CAS;
(b) D. Yang, K. Yan, W. Wei, L. Tian, Y. Shuai, R. Li, J. You and H. Wang, Asian J. Org. Chem., 2014, 3, 969–973 CrossRef CAS;
(c) T. M. Shaikh and F.-E. Hong, Tetrahedron, 2013, 69, 8929–8935 CrossRef CAS.
-
(a) J. Sim, M. Viji, J. Rhee, H. Jo, S. J. Cho, Y. Park, S. Y. Seo, K. Y. Jung, H. Lee and J.-K. Jung, Adv. Synth. Catal., 2019, 361, 5458–5465 CrossRef CAS;
(b) S. Lee, J. Sim, H. Jo, M. Viji, L. Srinu, K. Lee, H. Lee, V. Manjunatha and J.-K. Jung, Org. Biomol. Chem., 2019, 17, 8067–8070 RSC;
(c) M. Viji, J. Sim, S. Li, H. Lee, K. Oh and J.-K. Jung, Adv. Synth. Catal., 2018, 360, 4464–4469 CrossRef CAS;
(d) J. Sim, H. Jo, M. Viji, M. Choi, J. A. Jung, H. Lee and J.-K. Jung, Adv. Synth. Catal., 2018, 360, 852–858 CrossRef CAS;
(e) M. Choi, M. Viji, D. Kim, Y. H. Lee, J. Sim, Y. S. Kwak, K. Lee, H. Lee and J.-K. Jung, Tetrahedron, 2018, 74, 4182–4187 CrossRef CAS.
Footnote |
† Electronic supplementary information (ESI) available. See DOI: 10.1039/d0ra07093a |
|
This journal is © The Royal Society of Chemistry 2020 |
Click here to see how this site uses Cookies. View our privacy policy here.