DOI:
10.1039/D0RA06820A
(Paper)
RSC Adv., 2020,
10, 31101-31105
ortho-Naphthoquinone-catalyzed aerobic oxidation of amines to fused pyrimidin-4(3H)-ones: a convergent synthetic route to bouchardatine and sildenafil†
Received
7th August 2020
, Accepted 14th August 2020
First published on 21st August 2020
Abstract
A facile access to fused pyrimidin-4(3H)-one derivatives has been established by using the metal-free ortho-naphthoquinone-catalyzed aerobic cross-coupling reactions of amines. The utilization of two readily available amines allowed a direct coupling strategy to quinazolinone natural product, bouchardatine, as well as sildenafil (Viagra™) in a highly convergent manner.
N-Heterocyclic compounds with a pyrimidin-4(3H)-one core constitute a large number of natural products and biologically active molecules. For example, quinazolinone alkaloids possess a phenyl-fused pyrimidin-4(3H)-one structure and display a wide spectrum of pharmacological activities (Scheme 1a).1 Sildenafil (Viagra™), a potent and selective inhibitor of type 5 phosphodiesterases on smooth muscle cell, is based on the pyrazole-fused pyrimidin-4(3H)-one structure and marketed for erectile dysfunction.2 The synthetic approaches to the phenyl-fused pyrimidin-4(3H)-ones, quinazolinones, typically involve the condensation between anthranilamides and aldehydes to give aminal intermediates that in turn oxidized to quinazolinones under oxidation conditions (Scheme 1b). The oxidation catalysts include Cu,3 Fe,4 Ga,5 Ir,6 Mn,7 iodine,8 peroxide,9 however the aerobic oxidation of aminal intermediates is also known at 150 °C.10 The utilization of alcohols also effects the one-pot synthesis of quinazolinones through in situ oxidation to aldehydes in the presence of Fe,11 Ir,12 Mn,13 Ni,14 Pd,15 Ru,16 V,17 Zn,18 and iodine catalysts.19 Other precursors to aldehydes have been also identified using alkynes,20 benzoic acids,21 indoles,22 α-keto acid salts,23 β-keto esters,24 styrenes,25 sulfoxides,26 and toluenes.27 Non-aldehyde approaches to quinazolinones have been also demonstrated in the cross coupling of amidines,28 amines,29 benzamides,30 isocyanides,31 and nitriles.32 In 2013, the Nguyen group disclosed the synthesis of four quinazolinones, utilizing the autooxidation of benzylamines to imines that subsequently condensed with anthranilamides.33 While a closed system at 150 °C was necessary, the use of 40 mol% AcOH without solvent provided the quinazolinones in 46–75% yields. The Nguyen group also developed the FeCl3·6H2O-catalyzed condensation of 2-nitroanilines and benzylamines in the presence of 20 mol% of S8, where six quinazolinones were obtained in 68–75% yields.34 While the cross condensation of anthranilamides and benzylamines was accomplished, there exists a significant knowledge gap due to the limited substrate scope combined with less optimal reaction conditions (i.e. high reaction temperature, closed system in neat conditions and excess use of amines). In addition, it is not entirely clear if the cross condensation of amide-containing amines and benzylamines would work for other fused pyrimidin-4(3H)-one derivatives.35 To address such shortcomings in the cross condensation of amines, the ortho-naphthoquinone (o-NQ)-catalyzed aerobic cross amination strategy was investigated (Scheme 1c).36 Herein, we report a highly general approach to fused pyrimidin-4(3H)-one derivatives in the presence of o-NQ catalyst, culminating to the direct aerobic coupling of two amines to bouchardatine and sildenafil.
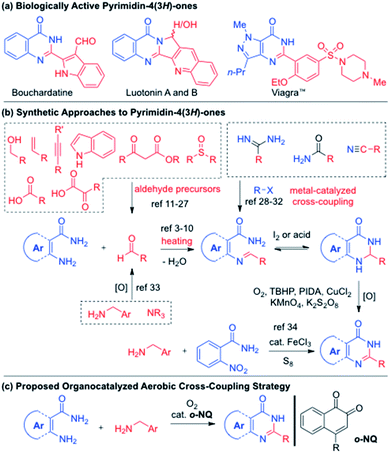 |
| Scheme 1 Biologically active fused pyrimidin-4(3H)-one derivatives and their synthetic methods. | |
Given that the o-NQ-catalyzed aerobic cross coupling of benzylamines and aniline derivatives such as o-phenylenediamines provided a facile approach to heterocyclic compounds including benzoimidazoles,37 the use of anthranilamide 1a and benzylamine 2a was examined as a model study (Table 1). The catalytic use of o-NQ1 smoothly converted benzylamine 2a to the corresponding homocoupled imine 2a′ under aerobic conditions. However, the subsequent in situ condensation reaction of 2a′ with anthranilamide 1a only provided the aminal product 3a in 11% yield (entry 1). To facilitate the cross coupling between the amine 2a and anthranilamide 1a, a catalytic amount of TFA was utilized where a significant improvement in yield was observed for 3a (entry 2). Faced with the inability to oxidize the aminal 3a to the corresponding product, quinazolinone 4a, other ortho-naphthoquinone catalysts were screened without much success (entries 3–5). To our delight, the examination of solvents revealed that the reaction temperature of 100 °C was needed for the formation of 4a (entries 6–11).38 The reaction in DMSO lowered down the ratio of 1a and 2a from 1.0
:
1.5 to 1.0
:
1.2 (entry 12) and the catalyst loading to 5 mol% without affecting the overall reaction efficiency (entry 13). The control experiments confirmed the critical roles of both o-NQ1 and TFA (entries 15–18), and the reaction utilized molecular oxygen as a terminal oxidant (entries 19 and 20). Piecing together the experimental data, the employment of 5 mol% o-NQ1 and 20 mol% TFA in DMSO at 100 °C was selected for further studies.
Table 1 Optimization of o-NQ-catalyzed aerobic cross-coupling of amines to quinazolinonea
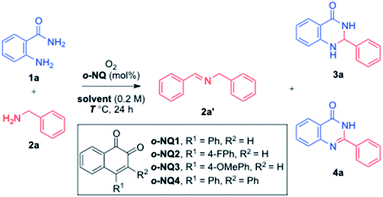
|
Entry |
Cat. (mol%) |
Solvent |
T (°C) |
Yieldb (%) |
Reaction using 1a (0.20 mmol), 2a (0.30 mmol), and o-NQ in solvent (0.2 M) under O2 balloon for 24 h. Yields based on internal standard and isolated yield in parentheses. Reaction for 6 h. Use of 2a (0.24 mmol, 1.2 equiv.). Reaction under air. Reaction under argon. NR = no reaction. |
1 |
o-NQ1 (10) |
CH3CN |
80 |
3a, 11 |
2 |
o-NQ1 (10)/TFA (20) |
CH3CN |
80 |
3a, 83 |
3 |
o-NQ2 (10)/TFA (20) |
CH3CN |
80 |
3a, 13 |
4 |
o-NQ3 (10)/TFA (20) |
CH3CN |
80 |
3a, 66 |
5 |
o-NQ4 (10)/TFA (20) |
CH3CN |
80 |
3a, 32 |
6 |
o-NQ1 (10)/TFA (20) |
MeOH |
65 |
3a, 5 |
7 |
o-NQ1 (10)/TFA (20) |
EtOH |
78 |
3a, 31 |
8 |
o-NQ1 (10)/TFA (20) |
DMF |
150 |
4a, >95 |
9c |
o-NQ1 (10)/TFA (20) |
DMSO |
150 |
4a, >95 |
10 |
o-NQ1 (10)/TFA (20) |
DMSO |
100 |
4a, >95 |
11 |
o-NQ1 (10)/TFA (20) |
DMSO |
80 |
3a, 30 |
12d |
o-NQ1 (10)/TFA (20) |
DMSO |
100 |
4a, >95 |
13d |
o-NQ1 (5)/TFA (20) |
DMSO |
100 |
4a, >95 (93) |
14 |
o-NQ1 (5)/TFA (10) |
DMSO |
100 |
3a/4a, 45/50 |
15 |
— |
DMSO |
100 |
3a, 10 |
16 |
o-NQ1 (10) |
DMSO |
100 |
3a/4a, 34/51 |
17 |
TFA (20) |
DMSO |
100 |
NR |
18 |
o-NQ1 (5)/AcOH (20) |
DMSO |
100 |
3a, 25 |
19e |
o-NQ1 (5)/TFA (20) |
DMSO |
100 |
3a, 33 |
20f |
o-NQ1 (5)/TFA (20) |
DMSO |
100 |
NR |
The optimized aerobic cross-coupling condition was applied to a variety of benzylamine derivatives (Scheme 2). In general, the electronic and steric characters of benzylamines did not significantly affect the formation of quinazolinones (4a–4m). However, the use of halogen-substituted and dimethoxy-substituted benzylamines led to the slightly lower yields of quinazolinones (4e, 4f and 4m) in 58–75% yields. In addition, the current aerobic cross-coupling reaction tolerated the furanyl and thiophenyl moieties, where the corresponding quinazolinones (4o and 4p) were obtained in 54% and 75% yields, respectively.
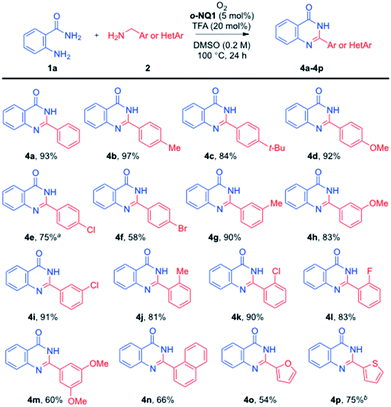 |
| Scheme 2 Substrate scope of aerobic oxidation to quinazolinones (areaction for 36 h, breaction for 12 h). | |
Further extension of the current aerobic cross-coupling reactions of amines is illustrated in Scheme 3. Thus, an array of substituted anthranilamides was readily employed to give the fused pyrimidin-4(3H)-one derivatives (4q–4x) in 61–84% yields. In particular, the N-substituted anthranilamides also participated in the current aerobic cross-coupling reaction in excellent yields (4y–4za). While the use of 3-amino-2-naphthamide led to the corresponding quinazolinone 4zb in 46% yield, the synthetic advantage of the current method was well demonstrated in the preparation of heteroaryl fused pyrimidin-4(3H)-one derivatives (4zc–4zh), where a variety of heterocyclic amines were successfully utilized in a tandem sequence of aerobic oxidation processes.
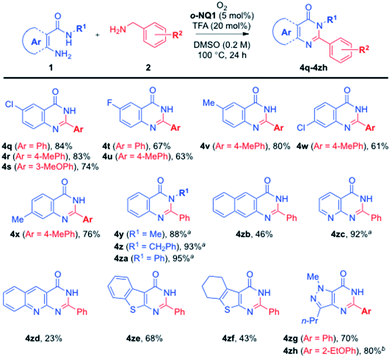 |
| Scheme 3 Further substrate scope for fused pyrimidin-4(3H)-one derivatives (areaction at 120 °C, breaction at 140 °C). | |
The mechanistic rationale of the aerobic cross-coupling reactions of amines is depicted in Scheme 4. Thus, the benzylamine 2a is condensed with the o-NQ1 catalyst to give the naphthol-imine species A.37a While the nucleophilic attack of 2a to the naphthol-imine A is favored due to the low nucleophilicity of the anthranilamide 1a, the presence of TFA promotes the cross-coupling between naphthol-imine A and anthranilamide 1a to give the naphthol-aminal B1. This process releases the hetero-coupled imine 3a′ and naphthol-amine C. The use of TFA promotes the intramolecular Mannich cyclization of imine 3a′, leading to the aminal 3a that in turn converts to the desired fused pyrimidin-4(3H)-one 4a with the help of o-NQ1 catalyst and molecular oxygen. Alternatively, the naphthol-imine A can produce the homocoupled imine 2a′ and the naphthol-amine C via the naphthol-aminal B2 through the nucleophilic attack of benzylamine 2a. The conversion of the naphthol-amine C to o-NQ1 catalyst is effected upon exposure to oxygen atmosphere.38 The homocoupled imine 2a′ undergoes hydrolysis at >80 °C to the benzaldehyde and benzylamine 2a that in turn re-enters the catalytic cycle.37b Our experimental observation of the homocoupled imine 2a′ by the 1H NMR and TLC analysis during the reaction supports the involvement of 2a′. However, the major pathway to the fused pyrimidin-4(3H)-one 4a appears to involve the naphthol-aminal B1 since the use of benzaldehyde instead of benzylamine 2a under the optimized conditions only led to the 80% conversion. The o-NQ1 catalyst without added TFA provided a mixture of aminal 3a and quinazolinone 4a in 34% and 51% yields, respectively (Table 1, entry 16). Thus, it is likely that the role of TFA is the catalyst for the cross-coupling of two amines to give the naphthol-aminal B1 and the cyclization of the imine 3a′ to aminal 3a. Our control experiments also revealed that TFA alone slowly oxidize 3a to 4a, but rapidly oxidized by the action of o-NQ1 within 10 h.39
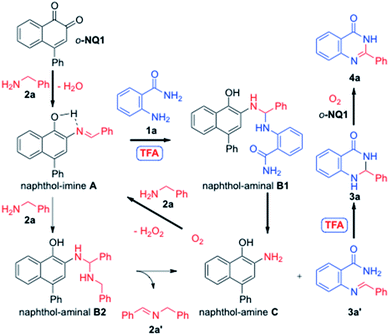 |
| Scheme 4 Mechanistic rationale for aerobic cross coupling reaction of amines. | |
The synthetic utility of the aerobic cross-coupling strategy is demonstrated in the synthesis of quinazolinone alkaloids and sildenafil (Scheme 5). The direct cross coupling of a commercially available pyrazole amine 1o and benzylamine 2r afforded a highly convergent synthetic approach to sildenafil.40 Likewise, the employment of anthranilamide 1a and 2-(aminomethyl)indole 2s provided the desired quinazolinone 5 in 70% yield, and the subsequent formylation under the Zeng's conditions41 paved a way to the total synthesis of bouchardatine. In addition, while the basicity of quinolin-2-ylmethanamine 2t required an excess of TFA, the corresponding quinazolinone 6 was obtained in 60% yield under the optimized conditions. The conversion of 6 to the luotonin natural products has been reported by the Argade group and others.42
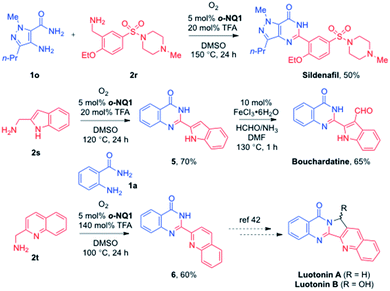 |
| Scheme 5 Synthetic utilization to quinazolinone alkaloids and sildenafil. | |
Conclusions
In summary, we have developed the aerobic cross-coupling reactions of amines to fused pyrimidin-4(3H)-one derivatives. This metal-free tandem aerobic oxidation sequence utilizes 5 mol% of o-NQ catalyst and 20 mol% of TFA as co-catalyst. The developed aerobic oxidation protocol allows a highly convergent approach to quinazolinone alkaloids and sildenafil. Given that the fused pyrimidin-4(3H)-one derivatives possess a diverse array of biological activities, the o-NQ-catalyzed tandem aerobic cross-coupling reactions should find their synthetic utility in the medicinal chemistry projects. We are current extending the o-NQ-catalyzed aerobic oxidation protocols to other heterocycles of medicinal interest, and our results will be reported in due course.
Conflicts of interest
There are no conflicts to declare.
Acknowledgements
This research was supported by the Chung-Ang University Research Scholarship Grants in 2019 and the National Research Foundation of Korea (NRF) grant funded by the Korean government (MSICT) (NRF-2015R1A5A1008958 and NRF-2019R1A2C2089953).
Notes and references
- For reviews, see:
(a) S. B. Mhaske and N. P. Argade, Tetrahedron, 2006, 62, 9787 CrossRef CAS;
(b) U. A. Kshirsagar, Org. Biomol. Chem., 2015, 13, 9336 RSC.
-
(a) M. Boolell, M. J. Allen, S. A. Ballard, S. Gepi-Attee, G. J. Muirhead, A. M. Naylor, I. H. Osterloh and C. Gingell, Int. J. Impotence Res., 1996, 8, 47 CAS;
(b) P. J. Dunn, Org. Process Res. Dev., 2005, 9, 88 CrossRef CAS;
(c) M. A. Gouda and W. S. Hamama, Synth. Commun., 2017, 47, 1269 CrossRef CAS.
-
(a) R. J. Abdel-Jalil, W. Voelter and M. Saeed, Tetrahedron Lett., 2004, 45, 3475 CrossRef CAS;
(b) D. Zhan, T. Li, H. Wei, W. Weng, K. Ghandi and Q. Zeng, RSC Adv., 2013, 3, 9325 RSC;
(c) S. Guo, Y. Li, L. Tao, W. Zhang and X. Fan, RSC Adv., 2014, 4, 59289 RSC;
(d) K. Upadhyaya, R. K. Thakur, S. K. Shukla and R. P. Tripathi, J. Org. Chem., 2016, 81, 5046 CrossRef CAS PubMed.
- G.-W. Wang, C.-B. Miao and H. Kang, Bull. Chem. Soc. Jpn., 2006, 9, 1426 CrossRef.
- J. Chen, D. Wu, F. He, M. Liu, H. Wu, J. Ding and W. Su, Tetrahedron Lett., 2008, 49, 3814 CrossRef CAS.
- F. Li, L. Lu and J. Ma, Org. Chem. Front., 2015, 2, 1589 RSC.
- M. Bakavoli, O. Sabzevari and M. Rahimizadeh, Chin. Chem. Lett., 2007, 18, 1466 CrossRef CAS.
-
(a) X.-S. Wang, K. Yang, M.-M. Zhang and C.-S. Yao, Synth. Commun., 2010, 40, 2633 CrossRef CAS;
(b) Y. Nagasawa, Y. Matsusaki, T. Nobuta, N. Tada, T. Miura and A. Itoh, RSC Adv., 2015, 5, 63952 RSC;
(c) R. Cheng, T. Guo, D. Zhang-Negrerie, Y. Du and K. Zhao, Synthesis, 2013, 45, 2998 CrossRef CAS.
- X.-F. Wu, S. Oschatz, A. Block, A. Spannenbergb and P. Langer, Org. Biomol. Chem., 2014, 12, 1865 RSC.
-
(a) Y.-F. Wang, F.-L. Zhang and S. Chiba, Org. Lett., 2013, 15, 2842 CrossRef CAS PubMed;
(b) N. Y. Kim and C.-H. Cheon, Tetrahedron Lett., 2014, 55, 2340 CrossRef CAS;
(c) B.-Q. Hu, J. Cui, L.-X. Wang, Y.-L. Tang and L. Yang, RSC Adv., 2016, 6, 43950 RSC;
(d) Z.-z. Wang and Y. Tang, Tetrahedron, 2016, 72, 1330 CrossRef CAS.
-
(a) D. Zhao, Y.-R. Zhou, Q. Shen and J.-X. Li, RSC Adv., 2014, 4, 6486 RSC;
(b) Y. Hu, L. Chen and B. Li, RSC Adv., 2016, 6, 65196 RSC.
-
(a) J. Zhou and J. Fang, J. Org. Chem., 2011, 76, 7730 CrossRef CAS PubMed;
(b) F. Li, L. Lu and P. Liu, Org. Lett., 2016, 18, 2580 CrossRef CAS PubMed.
- Z. Zhang, M. Wang, C. Zhang, Z. Zhang, J. Lu and F. Wang, Chem. Commun., 2015, 51, 9205 RSC.
- S. Parua, S. Das, R. Sikari, S. Sinha and N. D. Paul, J. Org. Chem., 2017, 82, 7165 CrossRef CAS PubMed.
- H. Hikawa, Y. Ino, H. Suzuki and Y. Yokoyama, J. Org. Chem., 2012, 77, 7046 CrossRef CAS PubMed.
- A. J. A. Watson, A. C. Maxwell and J. M. J. Williams, Org. Biomol. Chem., 2012, 10, 240 RSC.
- Z. Bie, G. Li, L. Wang, Y. Lv, J. Niu and S. Gao, Tetrahedron Lett., 2016, 57, 4935 CrossRef CAS.
- M. Sharif, J. Opalach, P. Langer, M. Beller and X.-F. Wu, RSC Adv., 2014, 4, 8 RSC.
- W. Ge, X. Zhu and Y. Wei, RSC Adv., 2013, 3, 10817 RSC.
-
(a) X. Yang, G. Cheong, J. Shen, C. Kuai and X. Cui, Org. Chem. Front., 2015, 2, 366 RSC;
(b) M. Abdullaha, S. Mohammed, M. Ali, A. Kumar, R. A. Vishwakarma and S. B. Bharate, J. Org. Chem., 2019, 84, 5129 CrossRef CAS PubMed.
- X. Chen, T. Chen, F. Ji, Y. Zhou and S.-F. Yin, Catal. Sci. Technol., 2015, 5, 2197 RSC.
-
(a) Y. Feng, Y. Li, G. Cheng, L. Wang and X. Cui, J. Org. Chem., 2015, 80, 7099 CrossRef CAS PubMed;
(b) F.-C. Jia, Z.-W. Zhou, C. Xu, Y.-D. Wu and A.-X. Wu, Org. Lett., 2016, 18, 2942 CrossRef CAS PubMed.
- J. K. Laha, K. V. Patel, K. S. S. Tummalapalli and N. Dayal, Chem. Commun., 2016, 52, 10245 RSC.
- Z. Li, J. Dong, X. Chen, Q. Li, Y. Zhou and S.-F. Yin, J. Org. Chem., 2015, 80, 9392 CrossRef CAS PubMed.
- W. Liu, W. Gao, J. Ding, X. Huang, M. Liu and H. Wu, Org. Chem. Front., 2018, 5, 2734 RSC.
- S. Lee, J. Sim, H. Jo, M. Viji, L. Srinu, K. Lee, H. Lee, V. Manjunatha and J. Jung, Org. Biomol. Chem., 2019, 17, 8067 RSC.
-
(a) D. Zhao, T. Wang and J.-X. Li, Chem. Commun., 2014, 50, 6471 RSC;
(b) S. Mohammed, R. A. Vishwakarma and S. B. Bharate, J. Org. Chem., 2015, 80, 6915 CrossRef CAS PubMed;
(c) Y. Jang, S. B. Lee, J. Hong, S. Chun, J. Lee and S. Hong, Org. Biomol. Chem., 2020, 18, 5435 RSC.
-
(a) C. Huang, Y. Fu, H. Fu, Y. Jiang and Y. Zhao, Chem. Commun., 2008, 6333 RSC;
(b) X. Liu, H. Fu, Y. Jiang and Y. Zhao, Angew. Chem., Int. Ed., 2009, 48, 348 CrossRef CAS PubMed;
(c) D. Yang, H. Fu, L. Hu, Y. Jiang and Y. Zhao, J. Comb. Chem., 2009, 11, 653 CrossRef CAS PubMed;
(d) X. Zhang, D. Ye, H. Sun, D. Guo, J. Wang, H. Huang, X. Zhang, H. Jiang and H. Liu, Green Chem., 2009, 11, 1881 RSC;
(e) B. Ma, Y. Wang, J. Peng and Q. Zhu, J. Org. Chem., 2011, 76, 6362 CrossRef CAS PubMed.
-
(a) W. Xu, Y. Jin, H. Liu, Y. Jiang and H. Fu, Org. Lett., 2011, 13, 1274 CrossRef CAS PubMed;
(b) W. Xu and H. Fu, J. Org. Chem., 2011, 76, 3846 CrossRef CAS PubMed;
(c) H. Wei, T. Li, Y. Zhou, L. Zhou and Q. Zeng, Synthesis, 2013, 45, 3349 CrossRef CAS;
(d) H. Li, L. He, H. Neumann, M. Beller and X.-F. Wu, Green Chem., 2014, 16, 1336 RSC;
(e) X.-F. Wu, S. Oschatz, M. Sharif, M. Beller and P. Langer, Tetrahedron, 2014, 70, 23 CrossRef CAS;
(f) M. Kumar, Richa, S. Sharma, V. Bhatt and N. Kumar, Adv. Synth. Catal., 2015, 357, 2862 CrossRef CAS.
- H. Chai, J. Li, L. Yang, H. Lu, Z. Qi and D. Shi, RSC Adv., 2014, 4, 44811 RSC.
- X. Jiang, T. Tang, J.-M. Wang, Z. Chen, Y.-M. Zhu and S.-J. Ji, J. Org. Chem., 2014, 79, 5082 CrossRef CAS PubMed.
- X. Yu, L. Gao, L. Jia, Y. Yamamoto and M. Bao, J. Org. Chem., 2018, 83, 10352 CrossRef CAS PubMed.
-
(a) T. B. Nguyen, L. Ermolenko and A. Al-Mourabit, Green Chem., 2013, 15, 2713 RSC. For the use of tertiary amines as aldehyde precursors, see:
(b) X. Chen, T. Chen, Y. Zhou, D. Han, L.-B. Han and S.-F. Yin, Org. Biomol. Chem., 2014, 12, 3802 RSC.
- T. B. Nguyen, J. L. Bescont, L. Ermolenko and A. Al-Mourabit, Org. Lett., 2013, 15, 6218 CrossRef CAS PubMed.
- For the use of 2 equiv. of K2S2O8, see: A. D. Hudwekar, G. L. Reddy, P. K. Verma, S. Gupta, R. A. Vishwakarma and S. D. Sawant, ChemistrySelect, 2017, 2, 4963 CrossRef CAS.
- For recent reviews on the biomimetic aerobic oxidation of amines by ortho-quinone catalyst-based oxidation protocols, see:
(a) B. Chen, L. Wang and S. Gao, ACS Catal., 2015, 5, 5851 CrossRef CAS;
(b) A. E. Wendlandt and S. S. Stahl, Angew. Chem., Int. Ed., 2015, 54, 14638 CrossRef CAS PubMed;
(c) M. Largeron, Org. Biomol. Chem., 2017, 15, 4722 RSC;
(d) R. Zhang and S. Luo, Chin. Chem. Lett., 2018, 29, 1193 CrossRef CAS;
(e) M. Largeron, Pure Appl. Chem., 2020, 92, 233 CAS. For the aerobic oxidation of benzylamines to pyrimidin-4(3H)-ones see:
(f) S. A. Pawar, A. N. Chand and A. V. Kumar, ACS Sustainable Chem. Eng., 2019, 7, 8274 CrossRef CAS (using non-catalytic ortho-quinone promoters);
(g) R. Sharma, M. Abdullaha and S. B. Bhatate, Asian J. Org. Chem., 2017, 6, 1370 CrossRef CAS (using ionic liquid).
- o-NQ catalysts are stable up to 150 °C, see:
(a) G. Golime, H. Y. Kim and K. Oh, Org. Lett., 2018, 20, 942 CrossRef CAS PubMed;
(b) Y. Goriya, H. Y. Kim and K. Oh, Org. Lett., 2016, 18, 5174 CrossRef CAS PubMed;
(c) G. Golime, G. Bogonda, H. Y. Kim and K. Oh, ACS Catal., 2018, 8, 4986 CrossRef CAS;
(d) K. Kim, H. Y. Kim and K. Oh, Org. Lett., 2019, 21, 6731 CrossRef CAS PubMed;
(e) T. Si, H. Y. Kim and K. Oh, ACS Catal., 2019, 9, 9216 CrossRef CAS.
- H. Y. Kim, S. Takizawa and K. Oh, Org. Biomol. Chem., 2016, 14, 7191 RSC.
- The conversion of aminal 3a to quinazolinone 4a was investigated by using 20 mol% TFA/O2 (86% conv. at 24 h), 5 mol% o-NQ1/O2 (92% conv. at 10 h), and O2 (81% conv. at 24 h) at 100 °C in DMSO (0.2 M).
- The commercial synthesis of sildenafil involves the amide coupling strategy, rather than the condensation of aldehyde and aminopyrazole route, possibly due to the two-step procedure (the formation of dihydrosildenafil by condensation and the following oxidation step to sildenafil), see: ref. 2b.
- Q.-D. Wang, B. Zhou, J.-M. Yang, D. Fang, J. Ren and B.-B. Zeng, Synlett, 2017, 28, 2670 CrossRef CAS.
-
(a) S. B. Mhaske and N. P. Argade, J. Org. Chem., 2004, 69, 4563 CrossRef CAS;
(b) K. R. Rao, R. Mekala, A. Raghunadh, S. B. Meruva, S. P. Kumar, D. Kalita, E. Laxminarayana, B. Prasad and M. Pal, RSC Adv., 2015, 5, 61575 RSC;
(c) R. Mekala, R. Kamaraju, S. Regati, N. Gudimalla, C. K. Bannoath and J. Sarva, Tetrahedron Lett., 2016, 57, 1418 CrossRef.
Footnote |
† Electronic supplementary information (ESI) available. See DOI: 10.1039/d0ra06820a |
|
This journal is © The Royal Society of Chemistry 2020 |
Click here to see how this site uses Cookies. View our privacy policy here.