DOI:
10.1039/D0RA06635D
(Paper)
RSC Adv., 2020,
10, 31819-31823
Regioselective C–H sulfenylation of N-sulfonyl protected 7-azaindoles promoted by TBAI: a rapid synthesis of 3-thio-7-azaindoles†
Received
31st July 2020
, Accepted 21st August 2020
First published on 27th August 2020
Abstract
This paper describes the regioselective C-3 sulfenylation of N-sulfonyl protected 7-azaindoles with sulfonyl chlorides. In this transformation, dual roles of TBAI serving as both promoter and desulfonylation reagent have been demonstrated. The reaction proceeded smoothly under simple conditions to afford 3-thio-7-azaindoles in moderate to good yields with broad substrate scopes. This protocol refrains from using transition-metal catalysts, strong oxidants or bases, and shows its practical synthetic value in organic synthesis.
Introduction
Indole core structures are the most important nitrogen-containing aromatic heterocycles, which are widely distributed in organic synthesis,1 medicinal chemistry,2 natural products,3 pharmaceutical agents,4 and others.5 Among them, 7-azaindoles and their synthetic analogues which possess the same [4.3]-bicyclic indene architecture as indoles (Fig. 1), have become one of the most widely studied organic templates, in part probably because of their prevalence in many bio-active structures6 and functional molecules7 (Fig. 1a–c).
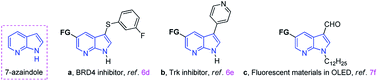 |
| Fig. 1 Biological activity and material applications of 7-azaindole derivatives. | |
Due to the significance of such sub-structures in various fields, chemists are showing an increased interest in developing effective methods to form 7-azaindole derivatives.8 Traditionally, 7-azaindole derivatives are synthesized starting from aminopyridines through the construction of pyrrole ring.8a,b,9 However, these methodologies suffer from some drawbacks such as toxic and foul-smelling reagents, prolonged reaction steps and low atom efficiency, which limit their wide applications. With the aim to functionalize the 7-azaindoles in a mild and atom-economical manner, transition-metal catalyzed C–H bonds activation has been described as an attractive strategy (Scheme 1a).10 For example, Sames,11 Fagnou,12 DeBoef,13 Das,14 Cao,15 and Laha16 reported independently the palladium-catalyzed C-2 arylation of 7-azaindole by using aryl iodide or benzene as coupling partners under different conditions. In addition, the N-oxide-assisted palladium-catalyzed C6–H arylation of 7-azaindoles has also been achieved by Fagnou and co-workers.12 Das's group17 realized the oxidative C3–H alkenylation of 7-azaindoles under palladium catalysis. However, the use of transition-metals may cause potential contamination of the products, which is particularly significant in the pharmaceutical industry and advanced functional materials. Among others, the C–H bonds activation reaction under transition-metal-free conditions has emerged as promising protocols because of their environmental friendliness. For instance, Liu18 and co-workers developed a regioselective deoxygenative C–H thiolation of 7-azaindole N-oxides with I2/PEG as the efficient and reusable catalytic system (Scheme 1b). Some other specific examples on C-3 sulfenylation of free 7-azaindole have also been achieved by Zhang,19 Wang,20 Liu21 and Sinha.22 Despite this progress, direct C-3 sulfenylation of N-sulfonyl protected 7-azaindoles using TBAI (tetrabutylammonium iodide) both as the promoter and as the desulfonylation reagent has not yet been documented. Based on our ongoing interest in the formation of C–S bond,23 herein, we want to disclose the regioselective C–H bond sulfenylation of N-sulfonyl protected 7-azaindoles promoted by TBAI (Scheme 1c).
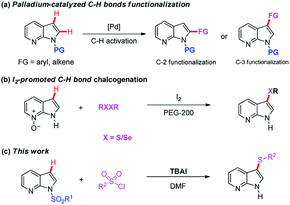 |
| Scheme 1 Regioseletive C–H functionalization of 7-azaindoles. | |
Results and discussion
At the outset of this investigation, we commenced our study on the model reaction of N-Ts protected 7-azaindole (1a) with tosyl chloride (2a) to optimize various reaction parameters. The results were summarized in Table 1. Initially, C-3 sulfenylation took place in the presence of TBAI (3 equiv.) in DMF under air, affording product 3a in 35% yield (entry 1, Table 1). The molecular structure of 3a was confirmed by NMR and HRMS spectra. Inspired by this result, various additives such as NaI, KI, I2 and TBAB were screened (entries 2–5, Table 1), however, no better results were observed with these experiments. The effect of solvent was also examined, and the results showed that DMAc gave lower yield of 3a (entry 6 vs. entry 1, Table 1) while no desired product was observed with 1,4-dioxane, acetonitrile, toluene and DCE (entries 7–10, Table 1). Gratifyingly, increasing the reaction temperature resulted in a significant improving of the product yield (entries 11–13, Table 1) and the highest yield (79%) product 3a was observed when the reaction was conducted at 120 °C for 18 h. The reaction time was also examined (entries 14 and 15, Table 1), and 6 h was found to be the best choice. The nitrogen protected reaction was also carried out, and a similar result was obtained in this reaction compared with the reaction in air (entry 17 vs. entry 15, 83% vs. 86%, Table 1). Based on the detailed investigations, we confirmed that the optimal conditions: TBAI (3.0 equiv.) as the additive in DMF at 120 °C under air atmosphere for 6 h (entry 15, Table 1).
Table 1 Optimization of the reaction conditionsa
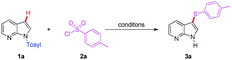
|
Entry |
Additive |
Solvent |
Yieldb (%) |
Reaction conditions: 1a (0.15 mmol), 2a (0.45 mmol), additive (3.0 equiv.) and solvent (1 mL), 80 °C, 18 h. Isolated yields. Run at 100 °C. Run at 120 °C. Run at 140 °C. Run for 12 h. Run for 6 h. Run for 3 h. Run under N2 atmosphere. N.R. = no reaction. |
1 |
TBAI |
DMF |
35 |
2 |
NaI |
DMF |
17 |
3 |
KI |
DMF |
14 |
4 |
I2 |
DMF |
N.R. |
5 |
TBAB |
DMF |
N.R. |
6 |
TBAI |
DMAc |
23 |
7 |
TBAI |
1,4-Dioxane |
N.R. |
8 |
TBAI |
Acetonitrile |
N.R. |
9 |
TBAI |
Toluene |
N.R. |
10 |
TBAI |
DCE |
N.R. |
11c |
TBAI |
DMF |
72 |
12d |
TBAI |
DMF |
79 |
13e |
TBAI |
DMF |
82 |
14d,f |
TBAI |
DMF |
84 |
15d,g |
TBAI |
DMF |
86 |
16d,h |
TBAI |
DMF |
80 |
17d,g,i |
TBAI |
DMF |
83 |
Based on the optimized conditions presented above, we subsequently focused on examining the generality and limitations of this protocol (Tables 2 and 3). Firstly, various protecting groups were surveyed for this transformation, and the results were summarized in Table 2. Both aryl sulfonyl and alkyl sulfonyl protected 7-azaindole underwent the reaction smoothly to provide the corresponding products in moderate to good yields (3a – 1–6, 54–86%, Table 2). Generally, different type of protecting groups have some appreciable influence on the outcome of the reaction, and tosyl group was confirmed as the best one. Next, a wide range of substituted aryl sulfonyl chlorides was subjected to the reaction with N-Ts protected 7-azaindole (1a) to produce the corresponding product 3b–3n in moderate to good yields (65–96%). Notably, benzenesulfonyl chloride and the monosubstituted (Me, OMe, t-Bu) benzenesulfonyl chlorides have proven to be suitable substrates for the reaction to provide the corresponding products (3b–c, 3g and 3j) in synthetic acceptable yields (73–91%). Substrates bearing electron-withdrawing substituents also resulted in good yields. Halides such as F, Cl, and Br afforded the desired products in 80–96% yields (3d–e, 3h–i, 3k–l), even strong electron-withdrawing groups (CF3) gave quite good yield of 3f (93%). Aryl sulfonyl chlorides containing a sterically hindered groups, bicyclic moiety naphthalene and substituted pyridine ring showed good compatibility (3m–p, 62–87%). Ethanesulfonyl chloride and cyclopropanesulfonyl chloride reacted as well to give the desired products 3q and 3r with yields of 52% and 46%, respectively. These results greatly expanded the substrate scope of this reaction.
Table 2 Substrate scope of protecting groups and sulfonyl chlorides for the sulfenylation reactionsa,b,c
Reaction conditions: 1 (0.15 mmol), 2 (0.45 mmol), TBAI (3 equiv.), DMF 1 mL, 120 °C, 6 h, under air. Isolated yields. R1 = p-tolyl for products 3b–3r. |
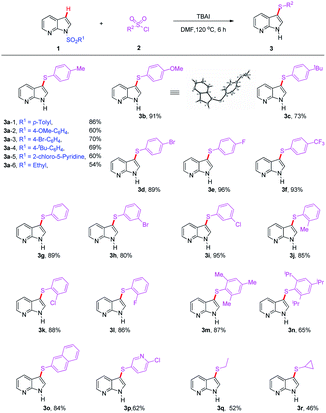 |
Table 3 Substrate scope of N-Ts protected 7-azaindoles for the sulfenylation reactionsa,b
Reaction conditions: 1 (0.15 mmol), 2a (0.45 mmol), TBAI (3 equiv.), DMF 1 mL, 120 °C, 6 h, under air. Isolated yields. |
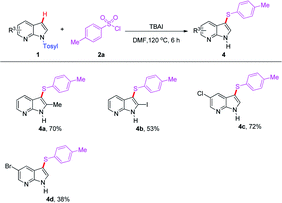 |
The compatibility of 7-azaindole derivatives was subsequently evaluated in this transformation (Table 3). Not surprisingly, the reaction of substrate 2a with several substituted N-Ts protected 7-azaindoles furnished the corresponding products (4a–d) in moderate yields. It is noteworthy that although relatively low yields were obtained when the halogenated 7-azaindoles were subjected to the reaction, it may provide a significant opportunity for their further transformation by transition-metal-catalyzed coupling reactions, especially in pharmacological demand.
Considering the experimental results and previously reports,24 a plausible mechanism was proposed and illustrated in Scheme 2. We envisioned that the formation of sulfonyl iodide A by anion exchange of sulfonyl chloride and TBAI would be the initial step of this transformation. Then, sulfonyl iodide A is continuously reduced into the intermediates B,21,25 which undergoes homolytic cleavage to produce the active sulfur radical C.24a Subsequently, the addition of sulfur radical to 7-azaindole occurs chemoselectively and generates a key intermediate D, which captured by the iodine radical from intermediates B affording intermediate E. Next, HI elimination takes place to provide 3-thio-7-azaindole F, which readily undergoes N-desulfonylation16a,26 to provide the desired products 3.
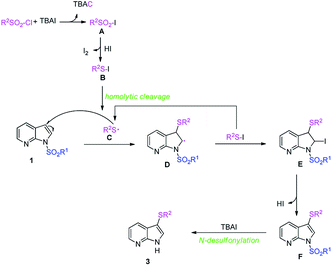 |
| Scheme 2 Plausible reaction mechanism. | |
Conclusions
In summary, we have described an efficient approach for the production of 3-thio-7-azaindoles via C–H bond activation under transition-metal-free conditions. In this protocol, dual roles of TBAI serving as both promoter and desulfonylation reagent have been demonstrated, and a series of 3-thio-7-azaindoles were obtained in moderate to good yields with high regioselectivity and good functional group tolerance. Further studies on extending the substrate scope and the application of the obtained products are underway, and the results will be forthcoming soon.
Conflicts of interest
There are no conflicts to declare.
Acknowledgements
The authors greatly acknowledge the financial support by The Education Department of Henan Province (20A210023), Henan Agricultural University (30500567, 30500701) and Key Science and the Technology Program of Science and Technology Department of Henan Province (152102210058, 122102210129).
Notes and references
-
(a) C. M. So, C. C. Yeung, C. P. Lau and F. Y. Kwong, A new family of tunable indolylphosphine ligands by one-pot assembly and their applications in suzuki-miyaura coupling of aryl chlorides, J. Org. Chem., 2008, 73, 7803–7806 CrossRef CAS PubMed;
(b) L. Chen and Y. Zou, Recent progress in the synthesis of phosphorus-containing indole derivatives, Org. Biomol. Chem., 2018, 16, 7544–7556 RSC.
-
(a) M. Zhang, Q. Chen and G. Yang, A review on recent developments of indole-containing antiviral agents, Eur. J. Med. Chem., 2015, 89, 421–441 CrossRef CAS;
(b) T. P. Singh and O. M. Singh, Recent progress in biological activities of indole and indole alkaloids, Mini-Rev. Med. Chem., 2018, 18, 9–25 CrossRef CAS PubMed;
(c) S. Suzen, Recent studies and biological aspects of substantial indole derivatives with anti-cancer activity, Curr. Org. Chem., 2017, 21, 2068–2076 CAS;
(d) S. M. Li, Prenylated indole derivatives from fungi: structure diversity, biological activities, biosynthesis and chemoenzymatic synthesis, Nat. Prod. Rep., 2010, 27, 57–78 RSC.
-
(a) S. R. Walker, E. J. Carter, B. C. Huff and J. C. Morris, Variolins and related alkaloids, Chem. Rev., 2009, 109, 3080–3098 CrossRef CAS PubMed;
(b) A. J. Kochanowska-Karamyan and M. T. Hamann, Marine indole alkaloids: potential new drug leads for the control of depression and anxiety, Chem. Rev., 2010, 110, 4489–4497 CrossRef CAS PubMed;
(c) R. J. Melander, M. L. Minvielle and C. Melander, Controlling bacterial behavior with indole-containing natural products and derivatives, Tetrahedron, 2014, 70, 6363–6372 CrossRef CAS PubMed;
(d) Y. Ma, X. Liang, Y. Kong and B. Jia, Structural diversity and biological activities of indole diketopiperazine alkaloids from fungi, J. Agric. Food Chem., 2016, 64, 6659–6671 CrossRef CAS PubMed.
-
(a) C. Gerhauer, M. You, J. Liu, R. M. Moriarty, M. Hawthorne, R. G. Mehta, R. C. Moon and J. M. Pezzuto, Cancer chemopreventive potential of sulforamate, a novel analogue of sulforaphane that induces phase II drug-metabolizing enzymes, Cancer Res., 1997, 57, 272–278 Search PubMed;
(b) C. Sherer and T. J. Snape, Heterocyclic Scaffolds as promising anticancer agents against tumours of the central nervous system: exploring the scope of indole and carbazole derivatives, Eur. J. Med. Chem., 2015, 97, 552–560 CrossRef CAS PubMed;
(c) F. R. de Sa Alves, E. J. Barreiro and C. A. M. Fraga, From nature to drug discovery: the indole scaffold as a ‘privileged structure’, Mini-Rev. Med. Chem., 2009, 9, 782–793 CrossRef PubMed.
-
(a) Z. R. Owczarczyk, W. A. Braunecker, A. Garcia, R. Larsen, A. M. Nardes, N. Kopidakis, D. S. Ginley and D. C. Olson, 5,10-Dihydroindolo[3,2-b]indole-based copolymers with alternating donor and acceptor moieties for organic photovoltaics, Macromolecules, 2013, 46, 1350–1360 CrossRef CAS;
(b) G. Nie, Z. Bai, W. Yu and L. Zhang, Electrochemiluminescence biosensor for ramos cells based on a nanostructured conducting polymer composite material (PICA-MWNTs), J. Polym. Sci., Part A: Polym. Chem., 2013, 51, 2385–2392 CrossRef CAS;
(c) M. Manickam, P. Iqbal, M. Belloni, S. Kumar and J. A. Preece, A brief review of carbazole-based photorefractive liquid crystalline materials, Isr. J. Chem., 2012, 52, 917–934 CrossRef CAS.
-
(a) A. A. Prokopov and L. N. Yakhontov, Chemistry of the azaindoles, Pharm. Chem. J., 1994, 28, 471–506 CrossRef;
(b) T. Irie and M. Sawa, 7-Azaindole: a versatile scaffold for developing kinase inhibitors, Chem. Pharm. Bull., 2018, 66, 29–36 CrossRef CAS PubMed;
(c) X. Chen, Z. Jin, Y. Gong, N. Zhao, X. Wang, Y. Ran, Y. Zhang, L. Zhang and Y. Li, 5-HT6 receptor agonist and memory-enhancing properties of hypidone hydrochloride (YL-0919), a novel 5-HT1A receptor partial agonist and SSRI, Neuropharmacology, 2018, 138, 1–9 CrossRef CAS PubMed;
(d) J. Zhang, J. Buell, K. Chan, P. N. Ibrahim, J. Lin, P. Pham, S. Shi, W. Spevak, G. Wu and J. Wu, Preparation of heterocyclic compounds as BRD4 inhibitors and uses thereof PCT, Int. Appl., WO2014145051A120140918, 2014;
(e) T.-T. Jaana, P. Rahel, J. Kaur, L. Kristi, D. A. Dobchev, D. Kananovich, A. Noole, M. Mandel, A. Kaasik, M. Lopp, T. Timmusk and M. Karelson, Indole-like trk receptor antagonists, Euro. J. Med. Chem., 2016, 121, 541–552 CrossRef PubMed;
(f) S. Hong, J. Kim, J. H. Seo, K. H. Jung, S. Hong and S. Hong, Design, synthesis, and evaluation of 3, 5-disubstituted 7-azaindoles as trk inhibitors with anticancer and antiangiogenic activities, J. Med. Chem., 2012, 55, 5337–5349 CrossRef CAS PubMed.
- For reviews, see:
(a) Y. Wu, H. Huang, J. Shen, H. Tseng, J. Ho, Y. Chen and P. Chou, Water-catalyzed excited-state proton-transfer reactions in 7-azaindole and its analogues, J. Phys. Chem. B, 2015, 119, 2302–2309 CrossRef CAS PubMed;
(b) T. Tu, Y. Chen, J. Shen, T. Lin and P. Chou, Excited-state proton transfer in 3-cyano-7-azaindole: from aqueous solution to ice, J. Phys. Chem. A, 2018, 122, 2479–2484 CrossRef CAS PubMed;
(c) S. Zhao and S. Wang, Luminescence and reactivity of 7-azaindole derivatives and complexes, Chem. Soc. Rev., 2010, 39, 3142–3156 RSC. For selected examples, see:
(d) K. Kaur, S. Chaudhary, S. Singh and S. K. Mehta, An azaindole–hydrazine imine moiety as sensitive dual cation chemosensor depending on surface plasmon resonance and emission properties, Sens. Actuators, 2016, 222, 397–406 CrossRef CAS;
(e) C. Martín, K. Kennes, M. Van der Auweraer, J. Hofkens, G. de Miguel and E. M. García-Frutos, Self−assembling azaindole organogel for organic light−emitting devices (OLEDs), Adv. Funct. Mater., 2017, 27, 1702176 CrossRef;
(f) C. Martin, C. Borreguero, K. Kennes, M. Van der Auweraer, J. Hofkens, G. de Miguel and E. M. Garcıá-Frutos, Simple donor–acceptor luminogen based on an azaindole derivative as solid-state emitter for organic light-emitting devices, ACS Energy Lett., 2017, 2, 2653–2658 CrossRef CAS.
-
(a) J. J. Song, J. T. Reeves, F. Gallou, Z. Tan, N. K. Yee and C. H. Senanayake, Organometallic methods for the synthesis and functionalization of azaindoles, Chem. Soc. Rev., 2007, 36, 1120–1132 RSC;
(b) A. Sofia Santos, A. C. Mortinho and M. M. B. Marques, Metal-catalyzed cross-coupling reactions on azaindole synthesis and functionalization, metal-catalyzed cross-coupling reactions on azaindole synthesis and functionalization, Molecules, 2018, 23, 2673 CrossRef PubMed;
(c) W.-H. Zhong, Y. Zhong, H. Mao and X. Chao, Recent progress on the synthesis of (aza)indoles through oxidative alkyne annulation reactions, Synlett, 2017, 15, 1867–1872 Search PubMed.
-
(a) M. J. D. Pires, D. L. Poeira and M. M. B. Marques, Metal-catalyzed cross-coupling reactions of aminopyridines, Eur. J. Org. Chem., 2015, 2015, 7197–7234 CrossRef;
(b) J. Merour, S. Routier, F. Suzenet and B. Joseph, Recent advances in the synthesis and properties of 4-, 5-, 6-or 7-azaindoles, Tetrahedron, 2013, 69, 4767–4834 CrossRef CAS.
-
(a) J. M. Lopchuk, Five-Membered Ring Systems: Pyrroles and Benzo Analogs, in Progress in Heterocyclic Chemistry, ed. G. Gribble and J. J. Joule, Elsevier, Oxford, 2017, vol. 29, pp. 183–238 Search PubMed;
(b) L. Ouyang and W. Wu, Recent advancements in palladium-catalyzed reactions involving molecular oxygen, Curr. Opin. Green Sustain. Chem., 2017, 7, 46–55 CrossRef;
(c) M. Petrini, Regioselective direct C-alkenylation of indoles, Chem.–Eur. J., 2017, 64, 16115–16151 CrossRef PubMed.
-
(a) B. S. Lane and D. Sames, Direct C−H bond arylation:
selective palladium-catalyzed c2-arylation of N-substituted indoles, Org. Lett., 2004, 6, 2897–2900 CrossRef CAS PubMed;
(b) B. S. Lane, M. A. Brown and D. Sames, Direct palladium-catalyzed C-2 and C-3 arylation of indoles: a mechanistic rationale for regioselectivity, J. Am. Chem. Soc., 2005, 127, 8050–8057 CrossRef CAS PubMed. - M. P. Huestis and K. Fagnou, Site-selective azaindole arylation at the azine and azole rings via n-oxide activation, Org. Lett., 2009, 11, 1357–1360 CrossRef CAS PubMed.
- S. Potavathri, K. C. Preira, S. I. Gorelsky, A. Pike, A. P. Lebris and B. DeBoef, Regioselective oxidative arylation of indoles bearing n-alkyl protecting groups: dual c−h functionalization via a concerted metalation−deprotonation mechanism, J. Am. Chem. Soc., 2010, 132, 14676–14681 CrossRef CAS PubMed.
- P. Kannaboina, K. Anilkumar, S. Aravinda, R. A. Vishwakarma and P. Das, Direct C-2 arylation of 7-azaindoles: chemoselective access to multiarylated derivatives, Org. Lett., 2013, 15, 5718–5721 CrossRef CAS PubMed.
-
(a) Y. Huang, Z. Lin and R. Cao, Palladium nanoparticles encapsulated in a metal–organic framework as efficient heterogeneous catalysts for direct c2 arylation of indoles, Chem.–Eur. J., 2011, 17, 12706–12712 CrossRef CAS PubMed;
(b) Y. Huang, T. Ma, P. Huang, D. Wu, Z. Lin and R. Cao, Direct C-H bond arylation of indoles with aryl boronic acids catalyzed by palladium nanoparticles encapsulated in mesoporous metal–organic framework, ChemCatChem, 2013, 5, 1877–1883 CrossRef CAS.
-
(a) J. K. Laha, R. A. Bhimpuria, D. Prajapati, N. Dayal and S. Sharma, Palladium-catalyzed regioselective c-2 arylation of 7-azaindoles, indoles, and pyrroles with arenes, Chem. Commun., 2016, 52, 4329–4332 RSC;
(b) J. K. Laha, R. A. Bhimpuria and M. K. Hunjan, Intramolecular oxidative arylations in 7-azaindoles and pyrroles: revamping the synthesis of fused N-heterocycle tethered fluorenes, Chem.–Eur. J., 2017, 23, 2044–2050 CrossRef CAS PubMed.
- P. Kannaboina, K. A. Kumar and P. Das, Site-selective intermolecular oxidative c-3 alkenylation of 7-azaindoles at room temperature, Org. Lett., 2016, 18, 900 CrossRef CAS PubMed.
- S. Liu, H. Yang, L. Jiao, J. Zhang, C. Zhao, Y. Ma and X. Yang, Regioselective Deoxygenative Chalcogenation of 7-Azaindole N-Oxides Promoted by I2/PEG-200, Org. Biomol. Chem., 2019, 17, 10073 RSC.
- P. Sang, Z. Chen, J. Zou and Y. Zhang, K2CO3 Promoted Direct Sulfenylation of Indoles: a Facile Approach towards 3-Sulfenylindoles, Green Chem., 2013, 15, 2096–2100 RSC.
- H. Zhang, X. Bao, Y. Song, J. Qu and B. Wang, Iodine-catalysed versatile sulfenylation of indoles with thiophenols: controllable synthesis of mono- and bis-arylthioindoles, Tetrahedron, 2015, 71, 8885–8891 CrossRef CAS.
- L. Chen, Y. Wei, Z. Yang, P. Liu, J. Zhang and B. Dai, NH4I/1,10-Phenanthroline catalyzed direct sulfenylation of N-heteroarenes with ethyl arylsulfinates, Tetrahedron, 2015, 71, 8885–8891 CrossRef.
- D. Equbal, R. Singh, Saima, A. G. Lavekar and A. K. Sinha, Synergistic dual role of [hmim]Br-ArSO2Cl in cascade sulfenylation−halogenation of indole: mechanistic insight into regioselective C−S and C−S/C−X (X = Cl and Br) bond formation in one pot, J. Org. Chem., 2019, 84, 2660–2675 CrossRef CAS PubMed.
-
(a) Z. Wu, H. Song, X. Cui, C. Pi, W. Du and Y. Wu, Sulfonylation of Quinoline N-Oxides with aryl sulfonyl chlorides via copper-catalyzed C–H bonds activation, Org. Lett., 2013, 15, 1270–1273 CrossRef CAS PubMed;
(b) M. Lai, K. Zhai, C. Cheng, Z. Wu and M. Zhao, Direct thiolation of Aza-Heteroaromatic N-oxides with disulfides via copper-catalyzed regioselective C–H bond activation, Org. Chem. Front., 2018, 5, 2986–2991 RSC;
(c) Z. Wu, M. Lai, S. Zhang, X. Zhong, H. Song and M. Zhao, An efficient synthesis of benzyl dithiocarbamates by base-promoted cross-coupling reactions of benzyl chlorides with tetraalkylthiuram disulfides at room temperature, Eur. J. Org. Chem., 2018, 2018, 7033–7036 CrossRef CAS;
(d) M. Lai, Z. Wu, Y. Wang, Y. Zheng and M. Zhao, Selective synthesis of aryl thioamides and aryl-α-ketoamides from α-oxocarboxylic acids and tetraalkylthiuram disulfides: an unexpected chemoselectivity from aryl sulfonyl chlorides, Org. Chem. Front., 2019, 6, 506–511 RSC;
(e) C. Cheng, M. Zhao, M. Lai, K. Zhai, B. Shi, S. Wang, R. Luo, L. Zhang and Z. Wu, Synthesis of aza-heteroaromatic dithiocarbamates via cross-coupling reactions of aza-heteroaromatic bromides with tetraalkylthiuram disulfides, Eur. J. Org. Chem., 2019, 2019, 2941–2949 CrossRef CAS.
-
(a) P. Katrun, C. Mueangkaew, M. Pohmakotr, V. Reutrakul, T. Jaipetch, D. Soorukram and C. Kuhakarn, Regioselective C2 sulfonylation of indoles mediated by molecular iodine, J. Org. Chem., 2014, 79, 1778–1785 CrossRef CAS PubMed;
(b) W. Fu, K. Sun, C. Qu, X. Chen, L. Qu, W. Bi and Y. Zhao, Iodine-mediated sulfonylation of quinoline n-oxides: a mild and metal-free on-pot synthesis of 2-sulfonyl quinolines, Asian J. Org. Chem., 2017, 6, 492–495 CrossRef CAS;
(c) F. Xiao, H. Chen, H. Xie, S. Chen, L. Yang and G. Deng, Iodine-catalyzed regioselective 2-sulfonylation of indoles with sodium sulfinates, Org. Lett., 2014, 16, 50–53 CrossRef CAS PubMed;
(d) J. Zhang, Z. Wang, L. Chen, Y. Liu, P. Liu and B. Dai, The fast and efficient KI/H2O2 mediated 2-sulfonylation of indoles and N-methylpyrrole in water, RSC Adv., 2018, 8, 41651–41656 RSC.
- S. Oae and H. Togo, Reduction of organic sulfonic acids, sodium sulfonates, and sulfonic esters to the corresponding disulfides with polyphosphoric acid derivatives, potassium iodide and the tetrabutylammonium iodids system, Bull. Chem. Soc. Jpn., 1983, 56, 3813–3817 CrossRef CAS.
-
(a) A. Yasuhara and T. Sakamoto, Deprotection of N-sulfonyl nitrogen-heteroaromatics with tetrabutylammonium fluoride, Tetrahedron Lett., 1998, 39, 595–596 CrossRef CAS;
(b) A. Yasuhara, M. Kameda and T. Sakamoto, Selective monodesulfonylation of N, N-disulfonylarylamines with tetrabutylammonium fluoride, Chem. Pharm. Bull., 1999, 47, 809–812 CrossRef CAS;
(c) Y. Liu, L. Shen, M. Prashad, J. Tibbatts, O. Repic and T. J. Blacklock, A green N-detosylation of indoles and related heterocycles using phase transfer catalysis, Org. Process Res. Dev., 2008, 12, 778–780 CrossRef CAS.
Footnote |
† Electronic supplementary information (ESI) available. CCDC 2018442. For ESI and crystallographic data in CIF or other electronic format see DOI: 10.1039/d0ra06635d |
|
This journal is © The Royal Society of Chemistry 2020 |
Click here to see how this site uses Cookies. View our privacy policy here.