DOI:
10.1039/D0RA06048H
(Paper)
RSC Adv., 2020,
10, 31863-31873
Chemical composition, nutraceuticals characterization, NMR confirmation of squalene and antioxidant activities of Basella rubra L. seed oil†
Received
10th July 2020
, Accepted 18th August 2020
First published on 28th August 2020
Abstract
Basella rubra (Malabar spinach) is a commonly consumed green leafy vegetable in southern parts of India. The chemical composition, nutraceuticals characterization, squalene Nuclear Magnetic Resonance (NMR), in vitro antioxidant activities and cytotoxicity of B. rubra seed oil (33.08%) was investigated. Gas chromatography-mass spectrometry (GC/MS) analysis revealed the presence of palmitic (27.21 μmol%), oleic (33.83 μmol%) and linoleic acid (26.02 μmol%) with a total of 64.38 μmol% unsaturated fatty acids respectively. HPLC nutraceutical characterization showed a major constituent of gallic acid (11.23 mg%), γ-tocopherols (17.74 mg%), cycloartenylferulate (1.7 mg%), and squalene (1 g%). Squalene was further recovered (98%), purified (99.9%), and confirmed through 1H and 13C NMR. The in vitro antioxidant activities recorded by using 2,2-diphenyl-1-picrylhydrazyl (EC50 = 6 mg mL−1), ferric reducing antioxidant power (361.85 mM of Trolox Eq./100 g) and 2,2′-azino-bis-3-ethylbenzothiazoline-6-sulphonic acid (EC50 = 56.19 mg mL−1) scavenging activity. In vitro cytotoxicity assessed on 3T3-L1 showed good cell survival without any toxicity (upto 400 μg mL−1). B. rubra seed oil has proven nutraceuticals and antioxidant potentials with least toxicity which can be recommended for functional foods applications.
1. Introduction
Lipids are the major components of the human diet. Plants and plant seeds are the main sources of oils and fats in many regions of the world for human and industrial use.1 These plant derived oils are with a high concentration of polyunsaturated fatty acids, that prevents cancer, coronary heart disease, diabetes, and depression.2 The chemical nature of acylglycerols (tri, di, and mono) for vegetable oils is usually derived from fruits and seeds.3 Similarly, these vegetable oils are good sources of nutraceutical compounds such as phenolics, flavonoids, phytosterols, and tocopherols etc.1 The popular vegetable oils such as palm, coconut, peanut, and sunflower oils etc. constitute 75% of the world's consumed vegetable oil sources for frying, canning and preparations of emulsions and margarines.2 As there is growing evidence on all fats and oils are not equivalent, interest in polyunsaturated rich oil sources has been emerging.4 Henceforth, to meet this increasing demand for high oil yielding vegetable sources, new oil yielding Basella rubra seeds were taken for analysis.
Basella spp. (Basellaceae) includes two major varieties such as Basella alba (green stem and leaves) and Basella rubra (red-violet stem, leaves and fruits).5 Basella spp. foliage is a rich source of nutritionally and nutraceuticals important compounds.5 B. rubra L. commonly known as Malabar or Indian spinach is commonly consumed green leafy vegetable in southern parts of India.6 The structural aspects of phyto-chemical constituents of B. rubra and anti-angiogenic activity of stem extracts of Basella spp. have been reviewed and investigated recently.7,8 The nutritional and nutraceutical potentials of B. rubra fruit extracts on human cervical cancer cells (SiHa) were also been demonstrated.9 However, there is a limited knowledge on the importance of the B. rubra seeds for their folk medicinal uses for scientific knowledge. The biochemical characterization of B. alba seed oils pertaining to yield, specific gravity, colour and fatty acid composition were been recently reported.3 This report says that the B. alba seed oil can be promoted in cosmetic industries for skin care products and considered as a new source of non-conventional oil for pharmaceutical, cosmetic and food industries. In general, plant seed oils are good sources of phenolics and antioxidants that can scavenge the free radicals produced in the body by activating the endogenous defense systems and modulating the cellular signaling processes.1
Reactive oxygen and nitrogen species (ROS/RNS) are essential for detoxifications, immunity and chemical signaling.10 When the human being are stressed the production of ROS/RNS increases, that can be reduced by the consumption of foods rich in antioxidants such as phenolics, flavonoids and phytochemicals etc.11 The antioxidants in plant seed oils will form allylic free radicals and isomerize to the stable free radicals. The spice essential oils and vegetable oils are rich in natural antioxidants that delay the lipid peroxidation process and contribute to the consumer acceptance of the food products. The high level of flavonoid rich diets will reduce the risk of cancers such as colon, breast, and pancreatic cancers.12 Sterols exhibit antioxidant potentials by lowering serum cholesterol properties, which are important constituents of plant seed oils. The most common plant sterols are phytosterols (β-sitosterols, stigmasterols, campesterols and squalene) that are main reason behind their use as functional food ingredients.1
Squalene is an important component used in cosmetics for its photoprotective role and used as a lubricant.13 The decreased risk of colon cancer by the use of olive oil is attributed to the presence of squalene, that exhibits a chemopreventive.14 The available source of squalene is primarily from shark and whale which is under protection act of marine animals. Hence, there is need for the vegetable source wherein olive, wheat germ and rice bran contains only 0.1–0.7%.15 However, Amaranthus oil reported to contain high amount of squalene (3.6–6.1%) than any other vegetable oils with low total oil content of 5–8% respectively.16
The present aim of this investigation was to carry out the physicochemical composition, GC/MS characterization of individual fatty acids, nutraceutical characterizations by HPLC, NMR confirmation of squalene, in vitro antioxidant potentials and cytotoxicity of commonly consumed green leafy vegetable B. rubra seed oil.
2. Experimental
2.1. Chemicals
HPLC grade methanol, acetonitrile, ethanol, acetone, ascorbic acid, gallic acid, 2,2-diphenyl-1-picrylhydrazyl radical (DPPH), 2,2′-azino-bis-3-ethylbenzothiazoline-6-sulphonic acid (ABTS), glacial acetic acid, sodium carbonate, Folin–Ciocalteu's reagent, aluminum chloride, ammonium molybdate, potassium ferricyanide, ferric chloride were obtained from Sigma Aldrich and Hi-media Chemicals (Bangalore, India). All other chemicals used were of analytical grade. For HPLC analysis, degassed and 0.2 μm membrane filtered triple distilled water was used. All the chemicals used in Nuclear Magnetic Resonance (NMR) work purchased from Sigma Aldrich such as solvent deuterated chloroform (CDCl3) and TMS (tetramethyl silane)-as an internal NMR standard.
2.2. Source of the raw material
The seeds of B. rubra L. were collected from the local markets of Tirupati, Andhra Pradesh, India. The seeds were sown in micropots containing sand
:
soil
:
compost (1
:
1
:
1) for seed germination, maintained at controlled temperature (29–32 °C) and relative humidity (65%) under greenhouse conditions. The botanical confirmation of this plant was confirmed and herbarium specimen deposited at Herbarium deposition centre of Department of Botany, University of Mysore, Mysore (ref. no. 02.08.13.05). The image representing the B. rubra climber, inflorescence bearing fruiting bodies, collected de-pulped seeds, grinded seed powder, and extracted seed oil shown as ESI Fig. 1.† The fruits of B. rubra were collected and de-pulped manually with hand by crushing the fruits. The left over seeds were cleaned with running tap water to remove the residual pulp material and seeds were air-dried. The seed samples were made to coarse powder using mixer grinder (Make: Maharaja Whiteline Perfect W&R 500 Mixer Grinder) for 3 ± 0.2 min at high speed and stored in polythene air tight bags until further use.
2.3. Physicochemical composition of the oil
The toluene co-distillation method using Dean and Stark apparatus was used to estimate the final moisture content of the B. rubra seed powder before oil extraction in triplicates as described earlier.17 The co-distillation was done up to 4 h until the moisture is removed completely.
The oil was extracted from the above prepared seed powder sample with hexane in a Soxhlet extractor, wherein the samples were subjected to heating in the round bottom flask containing boiling chips on a water bath set at 60 ± 5 °C for about 8 h and the total oil yield was calculated gravimetrically.18
The colour of the extracted oil samples were determined using colour measurement instrument (Konica Minolta CM-5, VA, USA). The specific gravity at 25 °C, refractive index by using hand refractometer (Atago Co. RX-5000, Tokyo, Japan), free fatty acid value (FFA), peroxide value (PV), iodine value (IV), saponification value (SV), and unsaponifiable matter (USM) of oil was analyzed.18
The glyceride (triacyl glycerol-TAG, diacyl glycerol-DAG, and monoacyl glycerol-MAG) composition of the oil was estimated by using the standard column chromatographic method. TAG, DAG and MAG were eluted with the standard solvent system and the quantity of each fraction was determined gravimetrically after evaporating the solvent.18
The separation of the total lipid into individual lipid classes such as neutral (triglycerides, fatty acids, mono and diglycerides) and polar lipids (glyco and phospholipids) eluted with chloroform, acetone and methanol respectively.19
The oil sample was analyzed for the carbon, hydrogen, nitrogen and sulphur (CHNS) content using Elemental Analyser (Vario EL III) wherein sulphanilic acid was used as a standard compound. The percentage CHNS content was determined.20
2.4. Fatty acid analysis by GC/MS
The fatty acid methyl esters (FAME) were prepared for the extracted B. rubra seed oil (100 mg) sample by trans-esterification with heptadeconoic acid (C17:0) of 1 mg mL−1 was added as the internal standard (see ESI†).18 GC/MS analysis was performed by using an Agilent HP-7890B chromatograph connected directly to a 5977 inert mass spectrometer (Agilent Technologies, Milan, Italy), with GC column, DB-23 (60 m, 0.25 mm I.D. 0.25 mm film thickness) with inlet temperature was 250 °C (see ESI†).
2.5. Fourier-transform infrared spectroscopy (FTIR) analysis
The FTIR spectra of the oil extracted was recorded in triplicates (Tensor II, M/s. Bruker, Germany) from mid infrared range of 4000 to 400 cm−1 with a DLATGS (Deuterated L-Alanine Doped Triglycine Sulphate). The qualitative transmission molecular fingerprint was compiled with a PC based data acquisition with OPUS software version 7.5. The spectral data obtained were compared with the reference identification FTIR functional group interpretation. Prior to each sample the background spectra were collected by measuring an empty well on the silicon plate. The presented FTIR spectra are representative spectra of three spectra collected.
2.6. Determination of nutraceuticals composition and antioxidant activities
2.6.1. Preparation of methanolic extractives of oil. 1 g oil sample was extracted with 20 mL of 70% aqueous methanol containing 0.1% hydrochloric acid (HCl) and 80% aqueous methanol by shaking continuously for 1 h at room temperature.21 All the extractives were stored at −20 °C until further use.
2.6.2. Determination of total phenolics content (TPC). The soluble phenolic compounds in the 70% aqueous methanol containing 0.1% hydrochloric acid (HCl) and 80% aqueous methanol oil extractives were determined using the Folin–Ciocalteu's reagent as per the method described with slight modifications (see ESI†).22 The values were expressed as gallic acid equivalent (GAEq.), which is one of the most commonly, used standards in phenolic estimations.23
2.6.3. Determination of total flavonoid content (TFC). For the determination of TFC, 200 μL of above extractive was diluted with absolute ethanol to an appropriate concentration. 1 mL of diluted sample was mixed with 1 mL of 2% (w/v) methanolic solution of aluminum chloride (AlCl3).24 After incubation at room temperature for 15 min, the absorbance of the reaction mixture was read at 430 nm with a double beam spectrophotometer (UV-1800 A, Shimadzu Corporation, Kyoto, Japan). The TFC was expressed as rutin equivalent (REq.) in mg/100 g of oil.
2.6.4. Total phytosterols content. Total phytosterols content of the oil was determined by the Liebermann–Burchard method.25 Briefly, a known quantity of oil was diluted with chloroform and to this Liebermann–Burchard reagent was added and the colour developed was measured at 640 nm. A standard curve was also generated using cholesterol as a standard compound, and the results were expressed as total phytosterols as cholesterol equivalent.
2.6.5. Determination of oryzanol content. Oryzanol content was determined using double beam spectrophotometer (UV-160 A, Shimadzu Corporation, Kyoto, Japan) by measuring the absorbance of the extracted oil in hexane at 314 nm followed by calculation using the extinction coefficient of 358.9 and expressing the oryzanol content as g/100 g.26
2.6.6. Estimation of lignans content. The lignans present in the oil was determined using spectrophotometric method. 0.01 g of oil sample was dissolved in 10 mL of HPLC grade chloroform and hexane (7
:
3 v/v) and the absorbance was measured at 288 nm followed by calculation using E1 cm1% value of 230.27
2.6.7. Determination of carotenoid content. Carotenoids content of the extracted oil was determined by diluting 1 g of melted oil sample at 65 °C to 10 mL using hexane. From this solution, 1 mL aliquot was further diluted to 10 mL with hexane and the absorbance was measured at 446 nm using double beam spectrophotometer (UV-160 A, Shimadzu Corporation, Kyoto, Japan). The carotenoid content was calculated by using the diffusion coefficient of 383 and expressed as mg/100 g of oil.28
2.6.8. Determination of total antioxidant assay by phosphomolybdenum method. A 0.3 mL oil extractive was combined with 28 mM sodium phosphate, 4 mM ammonium molybdate and the reaction mixture was incubated at 95 °C for 90 min. After cooling to room temperature, the absorbance of the solution was measured at 695 nm using a double beam spectrophotometer (UV-160 A, Shimadzu Corporation, Kyoto, Japan) against blank.29 The total antioxidant activity was expressed as the number of gram equivalent of ascorbic acid (AAEq.) in g/100 g of oils.
2.6.9. 2,2-Diphenyl-1-picrylhydrazyl (DPPH) radical scavenging activity. DPPH free radical scavenging activity was determined according to the method reported (see ESI†).30 It is a most widely used method for the analysis of antioxidant activity of natural products and vegetable oils. The radical scavenging activity was expressed as effective concentration (EC50).5
DPPH radical scavenging activity (EC50) = (OD control − OD sample)/OD control × 100 |
2.6.10. Ferric reducing antioxidant power (FRAP). FRAP was performed in the oil extracts according to the method reported with Trolox as standard and the results are expressed as mM of Trolox/100 g of oil (see ESI†).31
2.6.11. 2,2′-Azino-bis-3-ethylbenzothiazoline-6-sulphonic acid (ABTS) scavenging activity assay. TEAC (Trolox equivalent antioxidant capacity) assay measures the ability of a compound to eliminate or scavenge radicals compared with Trolox as an antioxidant (see ESI†).32 The radical scavenging activity was represented by the percentage ABTS-scavenging activities of the oil extractives.
2.7. Separation of phenolics and flavonoids by HPLC
Phenolics and flavonoids were separated by reverse phase HPLC (Shimadzu LC 20A; Shimadzu Corp.) fitted with a C18 column (250 × 4.6, 5 μm, Waters Corporation, Milford, MA, USA) and UV detector (see ESI†).33
2.8. Separation of tocopherol by HPLC
The tocopherols in the oil dissolved in HPLC grade hexane was determined by HPLC (Shimadzu Corporation, Tokyo, Japan) with an isocratic elution of methanol/water (95
:
5) solvent system with C18 column (250 mm × 4.6 mm i.d.) with a flow rate of 1.5 mL min−1 with fluorescent detection with emission and excitation of 290 and 330 nm.34
2.9. Separation of oryzanol by HPLC
Oryzanol separation profile of oil was determined by HPLC (Shimadzu Corporation, Tokyo, Japan) with an isocratic elution of acetonitrile/methanol/isopropanol (10
:
9
:
1) solvent system as the mobile phase connected with C18 Shim-pack column (150 mm × 4.6 mm i.d.) with a flow rate of 1.0 mL min−1 and quantified by using oryzanol standard (Sigma Aldrich, Bangalore, India).35
2.10. Separation of phytosterols by HPLC
Separation of phytosterols and squalene was performed in an HPLC-UV (LC-20A, Shimadzu, Tokyo, Japan), with a 20 μL injection loop, and a UV detector monitored at 205 nm. The temperature was controlled at 30 ± 0.1 °C with a column heater. Separation was performed on a C18 – 5 μm column (250 × 4.6 mm i.d. YMC, USA), with 30
:
70 (v/v) methanol
:
acetonitrile as mobile phase with a flow rate of 1.2 mL min−1 and the detection wavelength is 205 nm.36
2.11. Column purification of squalene
The USM was further purified for squalene by column chromatography on a silica gel (24 g, 230–400 mesh, Sigma Co.) in glass column (see ESI†). Squalene eluted completely in the initial fractions (fraction 1 to fraction 8) with petroleum ether eluate.
2.12. HPLC, FTIR, and GC/MS confirmation of purified squalene
Squalene contents in the collected fractions were recorded by using HPLC-UV (LC-20A, Shimadzu, Tokyo, Japan), with a 20 μL injection loop, and a UV detector monitored at 205 nm. Squalene was identified by comparing the retention time to pure squalene (>99%) purchased from Sigma Aldrich, Bangalore, India. The collected fractions were analyzed for squalene functional finger printing with FTIR. Further, GC/MS confirmation was also performed by preparing the FAME with the collected fraction and standard (Sigma, Bangalore) as mentioned above.
2.13. 1H and 13C NMR for structural confirmation of squalene
All the solution-state NMR experiments were performed on 500 MHz Bruker AVANCE spectrometers, equipped with a double resonance probe. The sample temperature was regulated to 298 ± 2 K. All the spectra were reference to TMS as internal standard. Standard experiments such as 1D 1H and 13C{1H} were recorded. The purified samples were diluted with CDCl3, vortexed thoroughly for proper mixing and transferred to NMR sample tube and then used directly for NMR measurements.
2.14. Cell culture and MTT assay for cytotoxicity
3T3-L1 preadipocyte cells were obtained from NCCS, Pune, India. The obtained cells were grown in a humidified chamber with 5% CO2, 95% air and sub-cultured as monolayer in DMEM supplemented with heat inactive FCS (10 mL/100 mL DMEM). Cells were seeded in a 96-well microtiter plate at a concentration 5 × 103 cells per well in a final volume of 100 μL culture medium and left for 24 h at 37 °C. The preadipocyte cells and matured adipocytes were treated with various concentrations of oil for 24 h. The cell survival (%) was determined using standard MTT assay described. The percent cell survival was calculated using the formula.37
2.15. Statistical analysis
All the results are presented in the form of mean ± SD of three replicates. Data were subjected to one-way ANOVA followed by post hoc Duncan's Multiple Range Test (DMRT) using SPSS 17 (SPSS Inc., Chicago, IL, USA) for determining significance at p < 0.05. The EC50 values were calculated using the non-linear regression.
3. Results and discussion
3.1. Estimation of moisture content
The toluene distillation method is the most appropriate method for the determination of moisture content in seed samples. The moisture content of the B. rubra seed powder is 5.13% as shown in Table 1. The relatively less moisture content of the seeds will improve the time span of usability and with no cold preservation of the produce. Al-Sebaeai et al. revealed a comparative pattern in moisture content in two varieties of fenugreek seeds i.e. Yemeni (8.91%) and Saudi seeds (10.23%).38
Table 1 Physicochemical composition of the B. rubra seed oila
Parameters |
Content |
All the values are mean ± SD of three replicates. |
Moisture of seeds (%) |
5.13 ± 0.11 |
Total oil content (%) |
33.08 ± 0.78 |
Specific gravity at 25 °C |
0.89 ± 0.01 |
Refractive index at 25 °C |
1.456 ± 0.018 |
![[thin space (1/6-em)]](https://www.rsc.org/images/entities/char_2009.gif) |
Colour measurement |
L |
5.85 ± 0.27 |
a* |
3.04 ± 0.3 |
b* |
4.61 ± 0.18 |
Chroma |
5.53 ± 0.11 |
Hue |
0.99 ± 0.06 |
FFA (%) |
1.53 ± 0.2 |
PV (mEq. O2/100 g) |
1.23 ± 0.05 |
IV (I2/100 g) |
81.56 ± 0.59 |
SV (mg/100 g) |
182.73 ± 4.17 |
USM (%) |
4.55 ± 0.20 |
![[thin space (1/6-em)]](https://www.rsc.org/images/entities/char_2009.gif) |
Glyceride composition (%) |
TAG |
87.55 ± 1.94 |
DAG |
9.84 ± 0.29 |
MAG |
2.61 ± 1.64 |
![[thin space (1/6-em)]](https://www.rsc.org/images/entities/char_2009.gif) |
Lipid classes (%) |
Neutral lipid |
95 ± 1.28 |
Glycolipid |
2.64 ± 0.32 |
Phospholipid |
2.36 ± 0.24 |
![[thin space (1/6-em)]](https://www.rsc.org/images/entities/char_2009.gif) |
Elemental composition (%) |
Carbon |
77.55 ± 0.19 |
Hydrogen |
17.08 ± 0.23 |
Nitrogen |
0.45 ± 0.06 |
Sulphur |
0.03 ± 0.00 |
3.2. Determination of oil content
The oil content of B. rubra seeds was 33.08%, which is high contrasted with other oil seeds, for example, cotton (13%), soybean (14%), fenugreek seeds (5.69%) and palm fruit (20%). This shows the significance of B. rubra seeds as one of the richest source of vegetable oil (Table 1). A 25.46% of oil content in the B. alba seeds which is nearly less with that of B. rubra seeds was reported.3 Similarly, the oil content (23.2%) of B. rubra seeds has been reported.39
3.3. Physicochemical composition of the oil
The physicochemical composition of B. rubra seed oil is presented in Table 1. The extracted oil was subjected for the colour measurement by colour measurement spectroscopy. The L, a*, b*, chroma and hue values obtained are shown in Table 1. The B. rubra seed oil specific gravity (0.89) and refractive index (1.456) at 25 °C was in comparison with the reported vegetable oils such as coconut, sunflower, and rice bran oil etc. (0.92 and 1.45–1.47) and B. alba seed oil with 0.91 and 1.47 respectively at 20 °C.3,40 The FFA is one of the quality indices to monitor the quality of the oils. The FFA and PV of B. rubra seed oil were 1.53 and 1.23 mEq. O2/100 g respectively. The FFA value was comparatively less compared to the FFA value reported in B. alba seed oil.3 The PV is one of the generally utilized measures for the assurance of oxidative rancidity in oils and fats. The high PV of these oils was probably due to the presence of higher levels of unsaturated fatty acids which could be less liable to oxidative rancidity at ambient temperature.41 The IV (81.56 I2/100 g) represents degree of unsaturation of oils, higher the values, higher is the amount of double/triple bonds in the fatty acids composition (Table 1). The reported values were relatively less compared to the fenugreek seed oil (130.94 I2/100 g) and B. alba seed oil (107.16 I2/100 g). The SV represent average molecular weight and fatty acid chain length of the oils. The SV of the studied oil was 182.73 mg/100 g which is relatively in the range compared with the earlier reports in B. alba seed oil (195.42 mg/100 g) which can be used for soap and lather shaving creams due to its high SV.42 Similarly, Al-Sebaeai et al. reported a similar SV in the seeds of Trigonella foenum-graecum (180 mg/100 g).40
The USM represents the natural antioxidant compounds present in the extracted oil such as phytosterols, tocopherols etc., which vary according to the natural antioxidant composition of the oil (Table 1). The B. rubra seed oil showed an amount of 4.55% USM. The USM of rice bran oil varies from 2.6–4.5% as reported earlier.43 The refined oils possess lower USM levels than its crude oil form due to the loss of minor nutraceuticals during processing. Hence, crude oils with good quality are preferable to deliver maximum nutraceutical benefits to consumers. The total glyceride composition (TAG, DAG and MAG) analyzed by column purification percentage of B. rubra seed oil was shown in Table 1. The TAG was found to be highest with (87.55%) followed by DAG with 9.84% and lowest of MAG (2.61%). The DAG content is 77% higher than the commercial sunflower oil reported.44 Wiesman et al. reported that the TAG in vegetable oils is major (95–98%) and the high TAG is vital source of energy and nutrition for humans.45 Similarly, the DAG is also comparable with the vegetable oil with 6.75% as reported earlier.46 In the present study, the major lipid classes of neutral lipid (95%), glycolipid (2.64%) and phospholipid (2.36%) were determined in B. rubra seed oil respectively. The elemental composition also showed that carbon and hydrogen are found in high content (Table 1).
3.4. GC/MS analysis
GC/MS chromatographic analysis revealed that C18:1 cis-9 (33.83 μmol%), C16:0 (27.21 μmol%) and C18:2 (26.02 μmol%) was the major fatty acids quantified (Fig. 1a and b). The lowest was identified to be C14:0 (0.47 μmol%) in the B. rubra seed oil. The other compounds detected were C16:1 (0.82 μmol%), C18:0 (5.49 μmol%), C18:1 cis-12 (3.71 μmol%), and C20:0 (0.93 μmol%). The percentage of unsaturated fatty acid composition is more (64.38 μmol%) compared with saturated fatty acids (34.1 μmol%). The values of fatty acids were comparable with the fatty acid profiles of B. alba seed oil as reported earlier.3 Similar comparison study on the fatty acid profile and tocopherols were characterized in B. rubra seed oil.47 The higher unsaturated fatty acids of B. rubra are more compared to the other reported seed oils such as shea butter (6.9%), avocado (15.5%), and Canarium schweinfurthii (28.8%) respectively. This higher PUFA may contribute to the flexibility, fluidity and selective permeability of cellular membranes that will beneficially reduce cardiovascular diseases.48 The higher content of linoleic acid in B. rubra seed oil could be beneficial to promote this oil in cosmetic industries for dehydration of skin tissue and reduce scaly lesions that are deficient in essential fatty acids.49
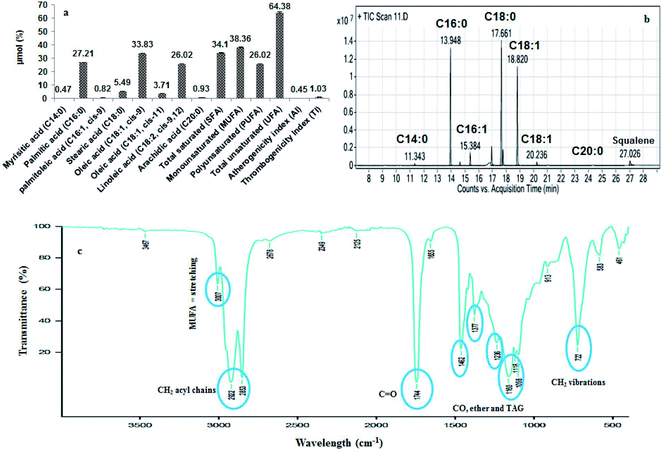 |
| Fig. 1 GC/MS quantification profile of individual fatty acids content (a), total ion chromatogram (b), and the FTIR finger printing showing the major functional groups identified (c) from B. rubra seed oil. | |
3.5. Fourier-transform infrared spectroscopy (FTIR) analysis
The extracted B. rubra seed oil was subjected to FTIR analysis to identify the functional groups present in the oil sample. The FTIR transmission spectrum of visible and near infrared finger print is shown in Fig. 1c. The spectrum showed peaks at 722 and 1098 interpretation of overlapping of the methylene rocking vibration and to the out of plane vibration of cis distribution olefins and stretching C–O–C of ether group respectively. Similarly, the peak at 1118 represent the TAG derived from secondary alcohol and 1744 showing C
O stretching mode of lipids. The 2853, 2922, and 3007 representing that asymmetric CH2 stretching mode of the methylene chains in membrane lipids, CH2 acyl chains, and –C
CH cis double bonds stretching that correlates with the MUFA content.
3.6. Determination of nutraceuticals composition and antioxidant activities
In general there are various methods for the determination of antioxidant capacities of oil samples. Each radical has its own limitation for the antioxidant compounds such as phenolics, flavonoids, tocopherols and phytosterols that will strongly exert antioxidant activity.
3.6.1. Total phenolics content (TPC) determination. The TPC in seed oils, play as an important indicator of oil quality and they protect lipids from peroxidation and used for the scavenging of free radicals. As shown in Table 2, the B. rubra seed oil of 70% aqueous methanol with 0.1% hydrochloric acid and 80% aqueous methanol extractives varied slightly depending on the solvent used. The content of TPC is 32.99 mg/100 g GAEq. in 80% aqueous methanol and 34.22 in 70% aqueous methanol with 0.1% hydrochloric acid of B. rubra seed oil respectively. The TPC plays an important role in overall antioxidant activity in the samples. The analyzed values of TPC were comparatively higher compared to other vegetable oils such as ground nut oil (3.09 mg), coconut oil (1.8 mg), rice bran oil (0.89 mg) and sunflower oil (0.49 mg) for 100 g GAEq. of oil sample.40 A similar trend of higher phenolic content was found in white mustard (150), coriander seeds (20) and caraway seeds (78) mg GAEq./100 g of oil.1 The difference in TPC may be due to the processing conditions of the refining and condition of extractions of vegetable oils.50
Table 2 In vitro nutraceutical compounds and antioxidant potentials of the B. rubra seed oil extracta
Parameter |
Content |
All the values are mean ± SD of three replicates. Values are 70% aqueous methanol with 0.1% hydrochloric acid results. |
Total phenols content (mg/100 g of oil gallic acid Eq.) |
32.99 ± 0.38 (34.22 ± 0.04)b |
Total flavonoid content (mg/100 g of oil rutin Eq.) |
557.88 ± 10.62 (560.66 ± 12.33)b |
Phytosterols (g/100 g) |
2.26 ± 1.10 |
Oryzanol (%) |
0.01 ± 0.002 |
Lignans (g/100 g of oil sesamol Eq.) |
0.02 ± 0.002 |
Carotenoids (mg/100 g of oil) |
30.5 ± 0.84 |
Total antioxidant assay by phosphomolybdate method (g/100 g of oil ascorbic acid Eq.) |
0.21 ± 0.1 (0.11 ± 0.06)b |
DPPH (mg EC50/mL) |
6.01 ± 0.2 (5.84 ± 0.11)b |
FRAP (mM of Trolox/100 g of oil) |
361.85 ± 23.45 (148.92 ± 9.06)b |
ABTS (mg EC50/mL) |
56.19 ± 2.38 (219.05 ± 14.80)b |
3.6.2. Determination of total flavonoid content (TFC). The flavonoids represents a large group of plant phenolics, for over half of the 8000 naturally occurring phenolic compounds.51 The TFC of B. rubra seed oil extractives showed higher flavonoid content compared to all other vegetable oils. Considerably high content of TFC in B. rubra seed oil was recorded in 80% aqueous methanol extractive (557.88 mg/100 g) and 70% aqueous methanol with 0.1% hydrochloric acid extractive (560.66 mg/100 g). Xuan et al. reported a very low levels of TFC in 14 types of vegetable oils studied with a highest TFC content in Inca Inchi oil (34 mg/100 g REq.) and lowest in safflower oils (3 mg/100 g REq.).52 The high content of TFC in B. rubra oil shows its important as a good source of antioxidant rich potentials of the seed oils and improving shelf-life of the oil.
3.6.3. Total phytosterols content. The result of total phytosterols showed that 2.26 g/100 g of B. rubra seed oil as shown in Table 2. The values are high compared with sesame oil with 0.44 g/100 g. Vegetable oils are the richest source of phytosterols in various levels that helps to enhance reduction of LDL cholesterol in blood and reduces the risk of cardiovascular diseases.53
3.6.4. Determination of oryzanol content. Table 2 shows the oryzanol content of B. rubra seed oils as 0.01%. Oryzanol is one of the important nutraceutical components present in oil extracted from rice brans and has several biological effects. Gopala Krishna et al. have reported the oryzanol content in different rice brans varieties.26
3.6.5. Estimation of lignans content. Table 2 shows the lignans content in B. rubra seed oil with 0.02 g/100 g. The content of lignans is comparatively low with sesame oil with 1.08 g/100 g.53 Lignans are group of compounds found in sesame oil and comprises of sesamin, sesamolin, sesaminol and sesamolinol. Sesame lignans are unique in bioactivity, functional, physiological and nutritional properties.
3.6.6. Determination of carotenoid content. The carotenoids content of B. rubra seed oil was 30.5 mg%. They are a distinct compounds found in palm oil (53.5 mg/100 g oil) as reported earlier.28 The carotenoid content was positively correlated with the red to green colour values (L, a* and b*) observed in the oils. Hence, we can avoid the use of synthetic colouring agents by the use of B. rubra seed oil in a various food formulations.
3.6.7. Determination of total antioxidant assay by phosphomolybdenum method. The antioxidants present in foods have a major role in maintaining the quality of oils by reducing the oxidative breakdown of lipids and nutritive value of oils.34 The antioxidant activity of the B. rubra seed oil extractives exhibited a varied activity with phosphomolybdate ions, compared with the standard ascorbic acid and results were expressed as AAEq. The 80% aqueous methanol extractive showed a higher activity (0.21) than 70% aqueous methanol with acid (0.11) g AAEq./100 g of oil which indicates the potential of oil as a good antioxidant rich source (Table 2).
3.6.8. DPPH radical scavenging activity. The radical scavenging activity of edible oil extracts tested using DPPH where the disappearance of the purple colour DPPH radicals by the test sample was calculated. The low EC50 value indicates the stronger DPPH radical scavenging activity which was shown in Table 2. The 80% aqueous methanol and 70% aqueous methanol acid extractive exhibited an EC50 value of 6 and 5.84 mg mL−1 oil sample respectively. The radical scavenging activity of oil will avoid/reduce the lipid peroxidation of tissues which intern reduces the risk of cancers in the tissues. The B. rubra seed oil is having higher or equivalent antioxidant capacity than sunflower oil and rice bran oil with EC50 values of 8 and 5 mg mL−1 respectively.34
3.6.9. Ferric reducing antioxidant power (FRAP). FRAP is one of the best method to find out the antioxidant activity of the extracted oils. The studied oils were subjected to FRAP analyses to find out the activity towards free radicals scavenging potentials. The 80% aqueous methanol and 70% aqueous methanol acid extractive showed 361.85 and 148.92 mM of Trolox/100 g of oil sample respectively (Table 2). In acid extractive, the degradation of ferric reducing antioxidant radical degradation was high than the 80% aqueous methanol. The reported values are in comparison with the commercial rapeseed oil acetone (339.6 μmol Fe/100 g) and methanol extracts (300.1 μmol Fe/100 g) respectively.54
3.6.10. ABTS scavenging activity assay. Another commonly used method for the determination of antioxidant potentials is ABTS. The ABTS free radical scavenging activity by B. rubra seed oil showed that 56.19 mg EC50/mL in 80% aqueous methanol which is significantly lower compared with the 70% aqueous methanol acid (Table 2). A less ABTS scavenging activity of vegetable oils with 4.56 mg EC50/mL for ground nut oil.40
3.7. Separation of polyphenols and flavonoids by HPLC
Fig. 2a represents the HPLC quantification profile of the B. rubra seed oil to find out the polyphenols and flavonoids. The identified polyphenols were gallic, protocatechuic, catechin, syringic acid, rutin and quercetin. Gallic acid was the highest (11.23 mg/100 g) in 70% aqueous methanol extractive and there was 6.2 folds reduction in 80% aqueous methanol with 0.1% HCl extractive (1.81 mg/100 g) of oil. Syringic acid was found in least quantity 0.25 mg/100 g in 70% aqueous methanol extractive.
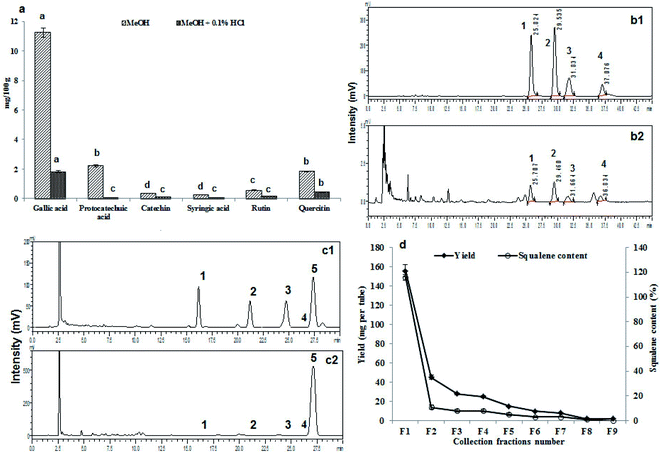 |
| Fig. 2 (a) HPLC quantification of individual phenolics and flavonoids of B. rubra seed oil, (b) HPLC separation profile of oryzanols standards (b1) and B. rubra seed oil (b2). Peaks 1 = cycloartanylferulate, 2 = 24-methylene cycloartenylferulate, 3 = campesterylferulate, 4 = β-sitosterylfrulate, (c) HPLC separation profile of phytosterols standards (c1) and B. rubra seed oil (c2). Peaks 1 = ergosterol, 2 = cholesterol, 3 = stigmasterol, 4 = β-sitosterol, 5 = squalene and (d) separation of USM for yield per tube and squalene content (%) by silica gel column. All the values are mean ± SD of triplicates and the significance was tested by Duncan multiple range test at p < 0.05, and the values with same superscript were found not significant difference from each other. | |
3.8. Separation of tocopherols by HPLC
Table 3 shows the tocopherols profiles of the B. rubra seed oil with gamma tocopherols as the major compound (17.74 mg/100 g). The beta and delta tocopherols were found to be least with 6.23 and 6.69 mg/100 g respectively. The content of gamma tocopherols was in concomitant with the 50% content in rape seed oil (34–37 mg/100 g), 3 folds high beta tocopherols than rice bran oil (2 mg/100 g) and 6 folds increase in delta tocopherols compared with rape seed oil (1 mg/100 g) as reported.54
Table 3 HPLC quantification profiles of phytosterols and tocopherol compoundsa
Compounds |
Content (mg/100 g of oil) |
All the values are mean ± SD of three replicates. ND = not detected. Significance was tested by Duncan multiple range test at p < 0.05, and values with different superscript for each parameter were found significant difference from each other. |
Tocopherols |
α |
8.23 ± 0.58b |
β |
6.23 ± 0.42c |
γ |
17.74 ± 1.37a |
δ |
6.69 ± 0.48c |
![[thin space (1/6-em)]](https://www.rsc.org/images/entities/char_2009.gif) |
Oryzanol |
Cycloartanylferulate |
1.70 ± 0.05b |
24-Methylene cycloartanylferulate |
2.30 ± 0.13a |
Campesterylferulate |
0.41 ± 0.01d |
β-Sitosterylfrulate |
0.67 ± 0.02c |
![[thin space (1/6-em)]](https://www.rsc.org/images/entities/char_2009.gif) |
Phytosterols |
Ergosterol |
23.47 ± 2.06d |
Cholesterol |
1.82 ± 0.47e |
Stigmasterol |
54.02 ± 1.36c |
β-Sitosterol |
121.25 ± 0.57b |
Squalene |
999.59 ± 14.26a |
3.9. Separation of oryzanol by HPLC
Oryzanol is a major compound present in rice bran oil which is a fore-fight for obesity and also acts as anti-aging and antidandruff agent. It inhibits the cholesterol oxidation, suppresses tumour growth, effective in the treatment of inflammatory disease and provides stability to food products. In B. rubra seed oil four types of ferulates were identified and quantified such as cycloartanyl ferulate, 24-methylene cycloartanyl ferulate, campesteryl ferulate and β-sitosteryl ferulate (Table 3 and Fig. 2b).
3.10. Separation of phytosterols and squalene by HPLC
Phytosterols constitute largest portion of the USM of the oils. They play an important role in cardiovascular disease by reduction of LDL levels in the blood. The ergosterol, fucosterol, stigmasterol and β-sitosterols were identified and quantified (Table 3 and Fig. 2c). Squalene was found to be highest (1%) in the oil which is an intermediate component of the cholesterol biosynthesis that acts as an antioxidant in most of the oils. It is an effective singlet oxygen quencher, prevent lipid peroxidation and protect skin from ultraviolet radiations.
3.11. Column purification of squalene
The squalene was column purified (99.9% purity) with 98% recovery using silica gel chromatography. The elution profile of the squalene and yield was shown in Fig. 2d. The initial fractions were found to be rich in squalene with good quantity, which was detected through TLC. Out of 45 fractions collected, after 9th fraction the squalene content and yield were found very less. A similar purification and fractionation with maximum recovery in first two fractions of squalene from Amaranthus grains have been reported.16
3.12. HPLC, FTIR, and GC/MS confirmation of squalene
The collected fractions were further analyzed by HPLC, FTIR, and GC/MS confirmations. The HPLC of squalene was performed with the same procedure as followed above in first nine fractions after complete evaporation and the chromatograms were presented in ESI Fig. 2a.† Similarly, the fractions obtained were analyzed for the molecular functional group finger printing with FTIR and compared with the standard squalene finger print and compared the peak interpretations. The three intense bands of C–H at 2958, 2918 and 2850 cm−1 and four intense skeletal vibrations modes at 1450, 1397, 1105, and 804 cm−1 were observed (ESI Fig. 2b†). The low intensity band at 1667 cm−1 due to C
C stretching was also of analytical importance. The GC/MS also confirmed by the two most abundant ions 69.1 and 81.1 (ESI Fig. 2c†). This FTIR interpretation data and GC/MS abundant ions were in correlation with the squalene FTIR spectral studies and GC/MS analysis in shark liver oils.55
3.13. 1H and 13C NMR for structural confirmation of squalene
The extracted and purified squalene was further confirmed by 1H and 13C NMR spectroscopy for the structure of the squalene. The fraction with highest squalene was used for the NMR study. The NMR spectrum, resulting data and expanded spectrums were shown in Fig. 3a, b, ESI Table 1 and Fig. 3† respectively. The obtained NMR data shows the purified compound is the squalene. The NMR results were also compared and correlated with standard squalene and available literature data (ESI Fig. 4†).16
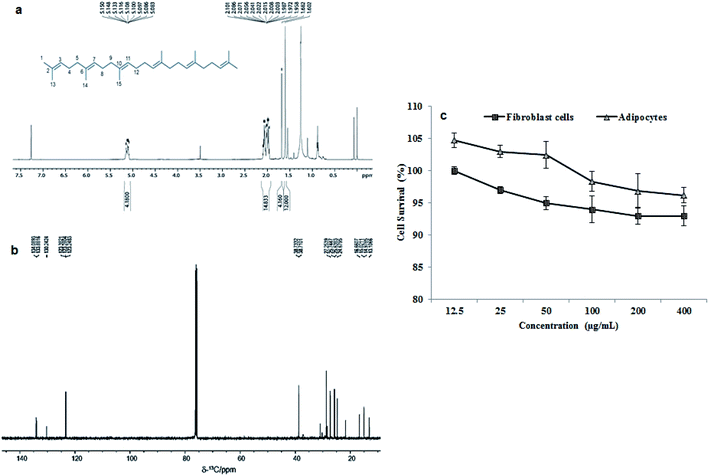 |
| Fig. 3 (a) One-dimensional 1H NMR spectrum along with the structure of squalene with proton and carbon numbering, (b) one-dimensional 13C NMR spectrum of purified squalene compound dissolved in CDCl3 recorded at 500 MHz field. The structure of squalene along with proton and carbon numbering also showed (please see ESI Table 1† for the assignments) and (c) cytotoxicity studies on 3T3-L1 immature fibroblast and matured adipocyte cell lines after exposing the cells for 24 h to B. rubra seed oil. Values are mean ± SD of five replicates (n = 5). | |
3.14. Cell culture and MTT assay for cytotoxicity
In vitro cytotoxicity results carried out through MTT assay after exposing to 3T3-L1 preadipocyte cells and matured adipocytes cells to B. rubra seed oil (Fig. 3c). No cytotoxicity was observed after 24 h exposure of the cells to seed oil up to 400 μg mL−1. A dose dependent decrease in cell viability was recorded with increasing the concentration in both the cell lines studied.
Overall, the B. rubra seed total oil content was 80.4% of that of sunflower oil which is commonly used vegetable oil. Similarly the IV, SV and TAG values of B. rubra seed oil were 79, 92 and 92% of sunflower seed oil respectively.44 It is quite interesting to note that there was 48%, 66%, 77.4% and 60% increase in the FFA, PV, DAG and MAG content respectively in B. rubra seed oil compared to sunflower seed oil. The total carotenoids content of B. rubra seed oil was pretty high (30.5 mg/100 g) compared to sunflower seed oil (0.31 mg/100 g).44 Very high content of total flavonoids content seems to be responsible for high free radical scavenging potential of B. rubra seed oil (6.01 mg EC50/mL) than sunflower seed oil (0.2 mg EC50/mL).52
4. Conclusion
The studied B. rubra seeds were having a good quantity of oil yield. Physicochemical parameters demonstrated the noteworthy great quality standards of B. rubra seed oil. The fatty acid composition by GC/MS indicated that palmitic, oleic and linoleic acids were the predominant fatty acids. Considering the bioactives of oil sample, it was found that oil extracted with 80% aqueous methanol was rich in phenolics and flavonoids especially the gallic, protocatechuic, catechin, syringic acid, rutin and quercetin as characterized by HPLC. Similarly, the superiority of the 80% aqueous methanol oil extracts with higher antioxidant activity was evident when assayed for total antioxidant activity, DPPH, FRAP, and ABTS. Interestingly, the seed oil can also be considered as a new rich source of squalene that established by HPLC, GC/MS, and NMR techniques which is a best molecule to fight against cancer and decreasing serum cholesterol levels. There was no toxicity of the oil when analyzed using 3T3-L1 cells up to 400 μg mL−1. Hence, the B. rubra seed oil has proven nutraceuticals (squalene) and antioxidant potentials with least toxicity which can be recommended as a vegetable oil by blending with the other commonly used cooking oils that can be recommended to use as functional foods applications.
Conflicts of interest
The authors have declared that there is no conflict of interest.
Acknowledgements
The author (SSK) is thankful to CSIR New Delhi for the award of Senior Research Fellowship and Department of Biotechnology (BT/PR1238/FNS/20/524/2011), Government of India, New Delhi for financial assistance. We greatly acknowledge the Director, CSIR-CFTRI for his kind support.
References
- M. Kozłowska, E. Gruczyńska, I. Ścibisz and M. Rudzińska, Food Chem., 2016, 213, 450–456 CrossRef PubMed.
- Y. P. Timilsena, J. Vongsvivut, R. Adhikari and B. Adhikari, Food Chem., 2017, 228, 394–402 CrossRef CAS PubMed.
- C. A. Diemeleou, L. T. Zoue and S. L. Niamke, Rom. Biotechnol. Lett., 2014, 19(1), 8966 CAS.
- L. L'homme, N. Esser, L. Riva, A. Scheen, N. Paquot, J. Piette and S. Legrand-Poels, J. Lipid Res., 2013, 54, 2998–3008 CrossRef PubMed.
- S. S. Kumar, P. Manoj and P. Giridhar, LWT--Food Sci. Technol., 2015, 64, 468–474 CrossRef CAS.
- A. Singh, P. K. Dubey, R. Chaurasiya, N. Mathur, G. Kumar, S. Bharati and P. C. Abhilash, Energy, Ecology and Environment, 2018, 3, 195–205 CrossRef.
- M. Singh, R. Kumari and M. Kotecha, Int. J. Basic Med. Sci. Pharm., 2016, 5, 206–223 CAS.
- B. R. Kumar, A. Anupam, P. Manchikanti, A. P. Rameshbabu, S. Dasgupta and S. Dhara, J. Food Sci. Technol., 2018, 55(5), 1675–1684 CrossRef CAS PubMed.
- S. S. Kumar, P. Manoj, P. Giridhar, R. Shrivastava and M. Bharadwaj, J. Funct. Foods, 2015, 15, 509–515 CrossRef CAS.
- B. Dimitrios, Trends Food Sci. Technol., 2006, 17, 505–512 CrossRef CAS.
- R. Baharfar, R. Azimi and M. Mohseni, J. Food Sci. Technol., 2015, 52, 6777–6783 CrossRef CAS PubMed.
- J. Safaei-Ghomi, A. H. Ebrahimabadi, Z. Djafari-Bidgoli and H. Batooli, Food Chem., 2009, 115, 1524–1528 CrossRef CAS.
- J. T. Budin, W. M. Breene and D. H. Putnam, J. Am. Oil Chem. Soc., 1996, 73(4), 475–481 CrossRef CAS.
- T. J. Smith, Expert Opin. Invest. Drugs, 2000, 9(8), 1841–1848 CrossRef CAS PubMed.
- K. A. Schnetzler and W. M. Breene, Food uses and amaranth product research: a comprehensive review, CRC Press, Boca Raton, Finland, 1994, pp. 155–184 Search PubMed.
- H. P. He, Y. Cai, M. Sun and H. Corke, J. Agric. Food Chem., 2002, 50(2), 368–372 CrossRef CAS PubMed.
- S. Ranganna, Hand book of analysis and quality control for fruits and vegetable products, Tata McGraw-Hill Publishing Company Limited, New Delhi, 2nd edn, 2001 Search PubMed.
- AOCS, Official methods and recommended practices of the American Oil Chemist's Society, Champaign, 2003 Search PubMed.
- W. W. Christie, The preparation of derivatives of lipids, in Lipid analysis, Pergamon Press Ltd., Oxford, 2nd edn, 1982, p. 51 Search PubMed.
- S. C. Sekhar, K. Karuppasamy, N. Vedaraman, A. E. Kabeel, R. Sathyamurthy, M. Elkelawy and H. A. E. Bastawissi, Energy Convers. Manage., 2018, 161, 141–154 CrossRef.
- I. Arcan and A. Yemenicioglu, J. Food Compos. Anal., 2009, 22, 184–188 CrossRef CAS.
- V. L. Singleton and J. A. Rossi, Am. J. Enol. Vitic., 1965, 16, 144–158 CAS.
- M. Claudine, W. Gary, M. Christine and S. Augustin, Am. J. Clin. Nutr., 2005, 81, 230–242 CrossRef PubMed.
- A. Djeridane, M. Yousfi, B. Nadjemi, D. Boutassouna, P. Stocker and N. Vidal, Food Chem., 2006, 97, 654–660 CrossRef CAS.
- S. M. Sabir, I. Hayat and S. D. A. Gardezi, Pak. J. Nutr., 2003, 2, 178–181 CrossRef.
- A. G. Gopala Krishna, K. H. Hemakumar and S. Khatoon, J. Am. Oil Chem. Soc., 2006, 83, 117–120 CrossRef.
- A. S. Bhatnagar, J. Hemavathy and A. G. Gopala Krishna, J. Food Sci. Technol., 2015, 52, 521–527 CrossRef CAS.
- K. Chandrasekaram, M. H. Ng, Y. M. Choo and C. H. Chuah, Am. J. Appl. Sci., 2009, 6, 529–533 CrossRef CAS.
- P. Prieto, M. Pineda and M. Agulilar, Anal. Biochem., 1999, 269, 337–341 CrossRef CAS PubMed.
- L. L. Yu, S. Haley, J. Perret, M. Harris, J. Wilson and M. Qian, J. Agric. Food Chem., 2002, 50, 1619–1624 CrossRef CAS PubMed.
- F. F. Iris Benzie and J. J. Strain, Methods Enzymol., 1999, 299, 15–27 Search PubMed.
- R. Re, N. Pellegrini, A. Proteggente, A. Pannala, M. Yang and C. Rice-Evans, Free Radical Biol. Med., 1999, 26, 1231–1237 CrossRef CAS PubMed.
- V. M. Burin, S. G. Arcari, A. M. T. Bordignon-Luiz and L. L. F. Costa, J. Chromatogr. Sci., 2011, 49(8), 647–651 CAS.
- S. Hemalatha, Food Chem., 2007, 105(3), 1076–1085 CrossRef CAS.
- E. J. Rogers, S. M. Rice, R. J. Nicolosi, D. R. Carpenter, C. A. McClelland and L. J. Romanczyk, J. Am. Oil Chem. Soc., 1993, 70, 301–307 CrossRef CAS.
- D. I. Sánchez-Machado, J. López-Hernández, P. Paseiro-Losada and J. López-Cervantes, Biomed. Chromatogr., 2004, 18(3), 183–190 CrossRef PubMed.
- T. Mosmann, J. Immunol. Methods, 1983, 65(1–2), 55–63 CrossRef CAS PubMed.
- M. A. Al-Sebaeai, M. Alfawaz, A. K. Chauhan, A. AL-Farga and S. Fatma, Int. J. Food Sci. Nutr., 2017, 6, 52–55 Search PubMed.
- B. Mathaus, K. Vosmann, L. Q. Pham and K. Aitzetmuller, J. Am. Oil Chem. Soc., 2003, 80, 1013–1020 CrossRef.
- C. Janu, D. S. Kumar, M. V. Reshma, P. Jayamurthy, A. Sundaresan and P. Nisha, J. Food Biochem., 2014, 38(1), 38–49 CrossRef CAS.
- J. M. deMan and C. K. Chow, Chemical and physical properties of fatty acids: fatty acids in foods and their health implications, New York, 1992, pp. 17–45 Search PubMed.
- O. U. Eka, J. Nutr. Sci., 1980, 1(1), 33–36 Search PubMed.
- A. G. Krishna, P. A. Prashanth, A. Pragasam, K. V. Raghavendra and S. Khatoon, J. Food Lipids, 2003, 10(4), 329–340 CrossRef CAS.
- R. Dhara and R. S. Singhal, J. Oleo Sci., 2014, 63(2), 169–176 CrossRef CAS PubMed.
- Z. Wiesman and B. P. Chapagain, Determination of fatty acid profiles and TAGs in vegetable oils by MALDI-TOF/MS fingerprinting, in Lipidomics, Humana Press, Totowa, NJ, 2009, pp. 315–336 Search PubMed.
- C. Pacheco, C. Palla, G. H. Crapiste and M. E. Carrín, Food Anal. Methods, 2014, 7(10), 2013–2022 CrossRef.
- R. Vimala and S. Keerthana, Int. J. Res. Dev. Pharm. Life Sci., 2014, 3, 1295–1299 Search PubMed.
- U. N. Das, Curr. Pharm. Biotechnol., 2006, 7(6), 467–482 CAS.
- T. Aburjai and F. M. Natsheh, Phytother. Res., 2003, 17(9), 987–1000 CrossRef PubMed.
- A. García, M. V. Ruiz-Méndez, C. Romero and M. Brenes, J. Am. Oil Chem. Soc., 2006, 83(2), 159–164 CrossRef.
- J. B. Harborne, H. Baxter and G. P. Moss, Handbook of bioactive compounds from plants, Taylor & Francis, London, 2nd edn, 1999 Search PubMed.
- T. D. Xuan, G. Gangqiang, T. N. Minh, T. N. Quy and T. D. Khanh, Foods, 2018, 7(2), 21 CrossRef PubMed.
- M. V. Reshma, C. Balachandran, C. Arumughan, A. Sunderasan, D. Sukumaran, S. Thomas and S. S. Saritha, Food Chem., 2010, 120(4), 1041–1046 CrossRef CAS.
- A. Szydłowska-Czerniak, C. Dianoczki, K. Recseg, G. Karlovits and E. Szłyk, Talanta, 2008, 76(4), 899–905 CrossRef PubMed.
- D. W. Hall, S. N. Marshall, K. C. Gordon and D. P. Killeen, Lipids, 2016, 51(1), 139–147 CrossRef CAS PubMed.
Footnote |
† Electronic supplementary information (ESI) available. See DOI: 10.1039/d0ra06048h |
|
This journal is © The Royal Society of Chemistry 2020 |
Click here to see how this site uses Cookies. View our privacy policy here.