DOI:
10.1039/D0RA05886F
(Paper)
RSC Adv., 2020,
10, 30848-30857
Synthesis of 1,3,4-oxadiazoles derivatives with antidepressant activity and their binding to the 5-HT1A receptor†
Received
6th July 2020
, Accepted 6th August 2020
First published on 20th August 2020
Abstract
In this study, two series of 1,3,4-oxadiazole derivatives were designed and synthesized using the forced swimming test (FST) model to test the antidepressant activity of the target compound in vivo. Five compounds with potential activity were selected from the FST model to test affinity with 5-HT1A receptor in vitro. The results of the FST experiment showed that compound N-(3-((5-((4-chlorobenzyl)thio)-1,3,4-oxadiazol-2-yl)methoxy)phenyl)acetamide (10g) showed the best antidepressant activity (DID = 58.93, percentage decrease in immobility duration in FST), similar to the activity of positive drug fluoxetine. Compound 10g also exhibited the most potent binding affinity to 5-HT1A receptors (Ki = 1.52 nM). The results of the in vivo 5-HT concentration estimation in mice showed that compound 10g may have an effect on the brain. The experimental results of exploratory activity in mice showed that compound 10g did not affect spontaneous activity in the open-field test model. Molecular docking was used to study the binding mode of compound 10g and the 5-HT1A receptor. Compound 10g showed significant interactions with residues at the active site on the 5-HT1A receptor. The physicochemical and pharmacokinetic properties of the target compounds were predicted using Discovery Studio 2019 and ChemBioDraw Ultra 14.
1. Introduction
Depression has become a major public health problem at present, with high prevalence, high suicide rate and significant societal costs.1,2 Currently, drug therapy is the main method of depression treatment. Some patients can be treated with drugs, but some patients still need to take drugs for a long time, but long-term use will have side effects, including affecting sleep quality, weight gain, gastrointestinal bleeding, etc. It also results in substantial healthcare costs.3,4 Therefore, there is an urgent need to search for new strategies for treatment of this disease.
Derivatives containing 1,3,4-oxadiazole structure are widely used in antidepressant drug research. For example, Singh et al., designed and synthesized a series of 1,3,4-oxadiazole derivatives, which showed good antidepressant activity.5 In addition, 3,4-dihydroquinolin-2(1H)-one also is a promising fragment in antidepressant drug research. For example, Deng et al., designed and synthesized a series of 3,4-dihydroquinolin-2(1H)-one derivatives, which showed good antidepressant activity (Fig. 1).6 In our previous studies, we designed and synthesized a series of 3,4-dihydroquinolin-2(1H)-one derivatives.7 Pharmacological experiment results show that this series of compounds showed good antidepressant activity in forced swimming test (FST) model. The preliminary mechanism of action indicates that it acts on the 5-hydroxy tryptamine 1A (5-HT1A) receptor and produces antidepressant activity. In this study, we adopted this series of compounds as lead compounds, combined structural fragments of 1,3,4-oxadiazole and 3,4-dihydroquinolin-2(1H)-one to improve their efficacy on depression, and a series of compounds 5a–i were designed and synthesized. To investigate the importance of the 3,4-dihydroquinolin-2(1H)-one fragment in the compound, a series of derivatives 10a–m were designed and synthesized by cutting at the 3 and 4 positions of 3,4-dihydroquinolin-2(1H)-one and by increasing the flexibility of the molecule to investigate the effect of ring opening on the compound activity (Fig. 2).
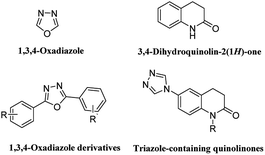 |
| Fig. 1 The chemical structure of 1,3,4-oxadiazole, 3,4-dihydroquinolin-2(1H)-one, and their derivatives. | |
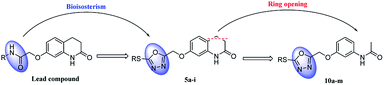 |
| Fig. 2 Structures of target compound 5a–i and 10a–m. | |
In this study, we used FST model, to test the antidepressant activity of the target compound in vivo. And 5-HT1A receptor binding assay was performed to determine the possible mechanism of action of the potent compound. The most potential compounds that showed effectiveness in FST, and the 5-HT concentration evaluation experiment was performed to test for their effect on 5-hydroxytryptamine (5-HT, serotonin) concentration in the mice brain, by using ELISA. The exploratory activity of the animals was also evaluated by the open-field test. The molecular docking module in the computer software Discovery Studio (DS) 2019 was used to analyze the possible modes of action of the potential compounds and 5-HT1A receptor active sites. Simultaneously, DS 2019 and ChemBioDraw Ultra 14.0 were used to predict the physicochemical and pharmacokinetic properties of the target compound. To confirm the structure of the target compound, we used high resolution mass spectrometry (HRMS) and 1H-nuclear/13C-nuclear magnetic resonance technology.
2. Results and discussion
2.1 Chemistry
The synthetic routes of target compounds 5a–i and 10a–m are shown in Schemes 1 and 2. First, intermediates 2 and 7 were synthesized under similar reaction conditions. Under reflux conditions, 7-hydroxy-3,4-dihydroquinolin-2(1H)-one/N-(3-hydroxyphenyl)acetamide was reacted with BrCH2CO2CH3 to obtain intermediates 2 and 7. Secondly, intermediates 2 and 7 were reacted with hydrazine hydrate using THF as a solvent, and intermediates 3 and 8 were obtained by reaction under reflux conditions. Next, intermediates 4 and 9 were generated via cyclization of intermediates 3 and 8 with CS2 under basic conditions. Following this process resulted in an incomplete reaction, and extra CS2 had to be added after 5–10 h to complete the reaction. The synthesis methods of intermediates 4 and 9 were synthesized according to the reported literature.8 Intermediates 4 and 9 were reacted with bromides to obtain target compounds 5a–i and 10a–m. The structures of all target compounds 5a–i and 10a–m were confirmed by 1H, 13C, and HRMS technology.
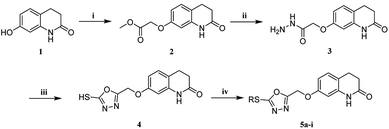 |
| Scheme 1 Synthesis of the target compounds 5a–i. Substituent R: 5a: n-C4H9; 5b: n-C5H11; 5c: n-C6H13; 5d: n-C7H15; 5e: CH2Ph; 5f: CH2Ph (p-F); 5g: CH2Ph (p-Cl); 5h: CH2Ph (p-CF3); 5i: CH2Ph (p-CH3). Reagents and conditions: (i) BrCH2CO2CH3, K2CO3, CH3CN; reflux, 6–8 h; (ii) NH2NH2.H2O, THF, reflux, 2 h; (iii) NaOH, CS2, C2H5OH, 80 °C, 19–21 h; (iv) RBr, K2CO3, acetone, 65 °C, 10 h. | |
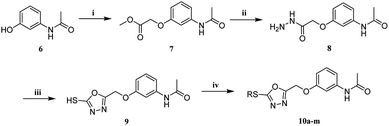 |
| Scheme 2 Synthesis of the target compounds 10a–m. Substituent R: 10a: CH2Ph; 10b: CH2Ph (o-F); 10c: CH2Ph (m-F); 10d: CH2Ph (p-F); 10e: CH2Ph (o-Cl); 10f: CH2Ph (m-Cl); 10g: CH2Ph (p-Cl); 10h: CH2Ph (o-CF3); 10i: CH2Ph (m-CF3); 10j: CH2Ph (p-CF3); 10k: CH2Ph (o-CH3); 10l: CH2Ph (m-CH3); 10m: CH2Ph (p-CH3). Reagents and conditions: (i) BrCH2CO2CH3, K2CO3, CH3CN; reflux, 6–8 h; (ii) NH2NH2.H2O, THF, reflux, 2 h; (iii) NaOH, CS2, C2H5OH, 80 °C, 19–21 h; (iv) RBr, K2CO3, acetone, 65 °C, 10 h. | |
2.2 Pharmacology
2.2.1. FST. The target compounds 5a–i and 10a–m were screened for antidepressant activity using FST model (dose: 40 mg kg; test time: 0.5 h). Fluoxetine was used as positive control drug in FST model. The results of the pharmacological experiments showed that most of the target compounds showed good antidepressant activity in the FST experimental model. The antidepressant activity of all target compounds are shown in Table 1.
Table 1 Antidepressant activities of compounds 5a–i and 10a–m in FST
Compounds |
R |
Antidepressant activitiesa |
Duration of immobility (s) (mean ± SEM)b |
DIDc (%) |
Target compounds and Fluoxetine were administered at 40 mg kg−1. Values represent the mean ± SEM (n = 8). %DID: percentage decrease in immobility duration. Significantly compared to control (0.01 < p < 0 .05). Very significantly compared to control (p < 0.01). |
5a |
C4H9 |
132.23 ± 13.12 |
15.62 |
5b |
C5H11 |
105.76 ± 18.45 |
32.51 |
5c |
C6H13 |
141.56 ± 20.38 |
9.67 |
5d |
C7H15 |
126.45 ± 21.77 |
19.31 |
5e |
CH2Ph |
128.83 ± 10.87 |
17.79 |
5f |
CH2Ph (p-F) |
99.87 ± 20.41d |
36.27 |
5g |
CH2Ph (p-Cl) |
67.24 ± 18.37e |
57.09 |
5h |
CH2Ph (p-CF3) |
75.04 ± 15.31e |
52.11 |
5i |
CH2Ph(p-CH3) |
101.84 ± 20.41 |
35.01 |
10a |
CH2Ph |
94.61 ± 18.87d |
39.63 |
10b |
CH2Ph (o-F) |
85.27 ± 21.19d |
45.59 |
10c |
CH2Ph (m-F) |
90.51 ± 15.69d |
42.24 |
10d |
CH2Ph (p-F) |
82.27 ± 17.29d |
47.50 |
10e |
CH2Ph (o-Cl) |
72.80 ± 16.12e |
53.54 |
10f |
CH2Ph (m-Cl) |
84.81 ± 20.39d |
45.88 |
10g |
CH2Ph (p-Cl) |
64.35 ± 17.48e |
58.93 |
10h |
CH2Ph (o-CF3) |
87.10 ± 14.67d |
44.42 |
10i |
CH2Ph (m-CF3) |
83.34 ± 18.70d |
46.82 |
10j |
CH2Ph (p-CF3) |
74.71 ± 18.88e |
52.32 |
10k |
CH2Ph (o-CH3) |
132.21 ± 22.20 |
15.63 |
10l |
CH2Ph (m-CH3) |
106.45 ± 19.96 |
32.07 |
10m |
CH2Ph (p-CH3) |
95.27 ± 16.61d |
39.21 |
Fluoxetine |
— |
67.31 ± 11.47e |
57.05 |
Control |
— |
156.72 ± 19.09 |
— |
The pharmacological data of target compounds 5a–i and 10a–m in FST model are shown in Table 1. As shown in Table 1. Most of compounds showed antidepressant activity at doses of 40 mg kg−1, with nine compounds, namely 5f, 10a–d, 10f, 10h–i, and 10m showing significant (p < 0.05, compared with the control group) antidepressant activity. Five compounds, namely 5g–h, 10e, 10g, and 10j showing high significant (p < 0.01, compared with the control group) antidepressant activity. Moreover, other compounds showed weak antidepressant activity. Especially, alkyl chain substituted compounds in the series of compounds 5a–i.
The pharmacological results in Table 1 show that in the FST model, compound 10g showed the best antidepressant activity, reduced the immobility time of the mice to 64.35 s, and its antidepressant activity was similar than that of fluoxetine (67.31 s). To explore the relationship between activity and compound dose, compounds 10g and fluoxetine were screened for antidepressant activity using three dose groups: 10, 20, and 40 mg kg−1, respectively. The pharmacological results show that within a certain dose range, as the dose increases, the antidepressant activity increases (Fig. 3).
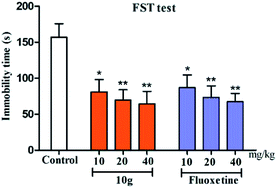 |
| Fig. 3 Antidepressant activities of compound 10g and fluoxetine in FST at different doses (40, 20, 10 mg kg−1). Values represent the mean ± SEM (n = 8). * Significantly compared to control (0.01 < p < 0.05). ** Very significantly compared to control (p < 0.01). | |
2.2.2. TST. The tail suspension test (TST) model is another effective way to screen antidepressants. In order to verify the effectiveness of compound 10g, we used the TST experimental model to further test the antidepressant activity of the compound. The pharmacological data of compound 10g in TST model is shown in Fig. 4. As shown in Fig. 4, compound 10g showing highly significant (p < 0.01, compared with the control group) antidepressant activity at 40 mg kg−1.
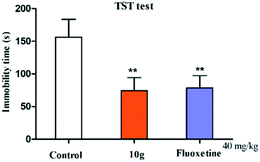 |
| Fig. 4 Antidepressant activities of compound 10g and fluoxetine in the TST test (40 mg kg−1). Values represent the mean ± SEM (n = 8). * Significantly compared to control (0.01 < p < 0.05). ** Very significantly compared to control (p < 0.01). | |
2.2.3. Determination of 5-HT concentration. The content and dysfunction of monoamine neurotransmitter 5-HT in the central nervous system may be related to the onset of various diseases such as psychosis, migraine, depression and so on. Studies have shown that 5-HT may be related to the neurobiochemical mechanism of depression. Pathological autopsy results of depression show that 5-HT levels in the brain stem and frontal lobe are reduced, and the total amount of 5-HT receptors in the hippocampus is reduced.9 In this study, 5-HT concentration is determined by enzyme-linked immunosorbent assay (ELISA) method. As shown in Fig. 5, at dose of 40 mg kg−1, compound 10g and fluoxetine significantly (p < 0.05) increased the 5-HT concentration in the mice compared with the control group. The results showed that compound 10g may have a certain effect on the 5-HT system, as it increased the 5-HT concentration in the brain of mice.
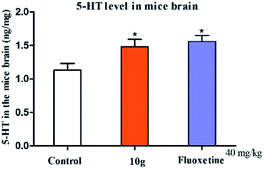 |
| Fig. 5 Effect of compound 10g and fluoxetine on brain 5-HT level in mice (40 mg kg−1). Compound 10g and fluoxetine were analyzed (the concentration of 5-HT in the mice brain) 1 h after oral administration. Represent the mean ± SEM (n = 8). * Significantly compared to control (0.01 < p < 0.05). | |
2.2.4. Open-field test. The open field test is a method for evaluating the behavior of experimental animals in a new environment, exploring behavior and tension. The frequency and duration of certain behaviors of the experimental animals in the novel environment reflect the autonomous behavior and inquiry behavior of the experimental animals in the strange environment.In the animal models of behavioral despair and depression, the shortening of animal immobility time is related to drug excitement sympathetic nerves.10,11 In this experiment, open field test was used to verify whether compound 10g can increase central excitability and affect the spontaneous activity of animals (this experiment examines spontaneous activities including: crossing, rearing, and grooming). The results of pharmacological showed that compound 10g had no significant difference (p > 0.5) compared to the control in the crossing, rearing, and grooming tests, and did not affect the spontaneous locomotor activity of mice (Fig. 6). The results of this experiment excluded that compound 10g is a false positive in mice due to excitatory central activity.
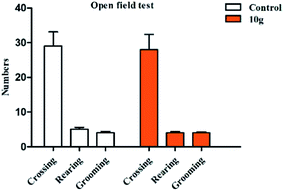 |
| Fig. 6 Exploratory activity (counts) in the open-field test. Compound 10g (40 mg kg−1) was administered 1 h before the test. Locomotion: number of line crossings; rearing: number of times seen standing on hind legs; grooming: number of modifications. The values represent the mean ± SEM (n = 8). | |
2.2.5. 5-HT1A receptor binding assay. The 5-HT1A receptor plays an important role in the pathogenesis of various mental and neurological diseases. Activation of postsynaptic 5-HT1A receptors is important for the favorable response to antidepressants.12,13 In this study, five potential compounds, 5g, 5h, 10e, 10g, and 10j were selected from FST model, and their affinities for the 5-HT1A receptor were determined to further study their possible mode of action. The Ki value of the compounds were determined using this experiment. The pharmacological results are shown in Table 2. As shown in Table 2, all compounds showed strong affinities for the 5-HT1A receptor, and compound 10g showed highest affinities for the 5-HT1A receptor, and its Ki value was 1.68 nM, similar to native neurotransmitter serotonin (Ki = 1.65 nM).
Table 2 5-HT1A receptor binding of selected compounds and reference serotonin
Compounds |
5-HT1A (Ki [nM] ± SEM)a |
Ki values were obtained from 8 concentrations of the compound, each in duplicate. |
5g |
1.77 ± 0.14 |
5h |
2.73 ± 0.22 |
10e |
2.01 ± 0.18 |
10g |
1.68 ± 0.13 |
10j |
4.14 ± 0.16 |
Serotonin |
1.65 ± 0.11 |
2.2.6. Structure–activity relationships. In this study, we used the previously synthesized 3,4-dihydroquinolin-2(1H)-one derivatives as lead compound, and combined structural fragment of 1,3,4-oxadiazole to improve their efficacy on depression, and a series of compounds 5a–i were designed and synthesized. To investigate the importance of the 3,4-dihydroquinolin-2(1H)-one fragment in the compound, a series of derivatives 10a–m were designed and synthesized by cutting at the 3 and 4 positions of 3,4-dihydroquinolin-2(1H)-one and by increasing the flexibility of the molecule to investigate the effect of ring opening on the compound activity. Screen the pharmacological activity of the target compound by FST model. The obtained compounds with better activity were further studied. Based on the pharmacological results obtained, the structure–activity relationships (SARs) of the target compounds were analyzed (Fig. 7).
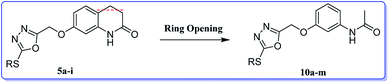 |
| Fig. 7 SARs of target compounds 5a–i and 10a–m. SARs for antidepressant activity of target compounds 5a–i and 10a–m at the R substituent: (1) benzyl-substituted derivatives are better than alkyl-substituted derivatives; (2) benzyl-substituted derivatives: p-F > o-F > m-F, p-Cl > o-Cl > m-Cl, p-CF3 > o-CF3 > m-CF3, p-CH3 > m-CH3 > o-CH3; (3) compounds substituted by Cl and CF3 groups on the benzene ring was superior to the compound substituted by F and CH3. | |
The pharmacological results obtained for compounds 5a–i showed that among the alkyl chain C4–C7-substituted compounds, all compounds showed weak antidepressant activity, compared with the control group, and it is not significant. For benzyl-substituted derivatives, the p-Cl substituted compound showed the best antidepressant activity than F-, CF3-, and CH3-substituted compounds. Overall, the antidepressant activity of the benzyl-substituted derivatives was superior to that of the alkyl chain-substituted derivatives. In view of the poor activity of alkyl chain substituted compounds in 5a–i, we only designed and synthesized benzyl substituted compounds in 10a–m. The pharmacological results of compounds 10a–m showed that among the benzyl-substituted derivatives, the activity of derivatives with different electronic group on the benzene ring was in the following order: p-F > o-F > m-F, p-Cl > o-Cl > m-Cl, p-CF3 > o-CF3 > m-CF3, p-CH3 > m-CH3 > o-CH3. The antidepressant activity of benzyl-substituted derivatives was superior to that of the alkyl chain-substituted derivatives.
By observing the pharmacological activities of 5a–i and 10a–m, it was found that the activity of compounds substituted by Cl and CF3 groups on the benzene ring was superior to the compounds substituted by F and CH3. It may be that the introduction of Cl and CF3 groups on the benzene ring has a greater influence on the charge distribution of the compound, which is conducive to the electrical binding of the compound and the receptor. Secondly, the introduction of Cl and CF3 groups on the benzene ring can increase the compound's liposolubility, and then make it easier to enter the brain tissue and play an antidepressant effect.
2.2.7. Docking study. In recent years, molecular docking, a theoretical simulation method that mainly studies intermolecular interactions and predicts the binding mode and affinity, has become an important technology in the field of computer-aided drug study. The most active compound 10g, native neurotransmitter serotonin, and the active site of the 5-HT1A receptor homology model were selected for molecular docking studies. The molecular docking module (Libdock) in the DS 2019 software was used for docking analysis. The results of molecular docking are shown in Fig. 8.
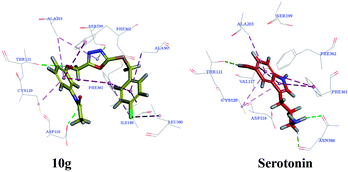 |
| Fig. 8 Compound 10g and serotonin show interactions with residues at the active site of 5-HT1A receptors. | |
According to the literature, the main amino acids around the active site are Ala93, Gln97, Phe112, Ile113, Asp116, Val117, Cys120, Thr121, Ile124, Ile189, Thr196, Ser199, Thr200, Ala203, Phe361, Phe362, Ala365, Thr379, Gly382, Ala383, and Asn386.14 The docking results of compound 10g (Libdock Score: 119.81) showed that it showed hydrogen bond with Asp116, Thr121, and Ser199; π–π stacking with Phe361 and Phe362; and π–alkyl interaction with Cys120, Ile189, Ala 203, and Ala365 (Fig. 8). The docking results of serotonin (Libdock Score: 88.84) showed that it showed hydrogen bond with Thr121, and Asn 386; pi–pi stacking with Phe361; and pi–alkyl interaction with Asp 116, Val 117, Cys120, and Ala 203 (Fig. 8). Results of compound 10g and serotonin in the active binding pocket of the 5-HT1A receptor are shown in Fig. 9. By analyzing the docking results of compound 10g, it can be speculated that the compound 10g can bind well with active site of 5-HT1A receptor, thereby exerting an antidepressant effect.
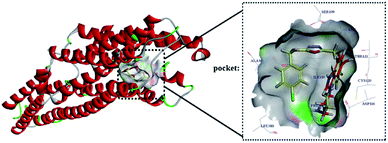 |
| Fig. 9 3D model of 5-HT1A receptor homology model, compound 10g (yellow) and serotonin (red) in the active binding pocket of 5-HT1A receptor. | |
2.2.8. Prediction of physicochemical and pharmacokinetic properties. Lipinski's “Rule of Five”, which is commonly used in drug design and screening, takes into consideration the following aspects: molecular weight (MW ≤ 500), number of hydrogen donors (nHBD ≤ 5), number of rotatable bonds (RotB ≤ 10), LogP ≤ 5, and the number of acceptors (nHBA ≤ 10). In fact, a compound that meets Lipinski's “Rule of Five” is more likely to be a suitable drug, even in the case of antidepressant drugs. We used DS 2019 to predict the physicochemical properties of the target compounds. As shown in Table 3, almost all target compounds met Lipinski's “Rule of Five”. Simultaneously, DS 2019 was used to predict the polar surface area (TPSA) of the target compounds, and the TPSA of all target compounds was shown to be less than 140 (TPSA is an indicator to examine the bioavailability of drugs; TPSA > 140 is considered to indicate low oral bioavailability).15
Table 3 Drug-likeness parameters of target compounds
Compds |
MWa |
C log P |
nHBD |
nHBA |
TPSA |
MW, molecular weight; C log P, calculated lipophilicity; nHBD, number of hydrogen bond donors; nHBA, number of hydrogen bond acceptors; TPSA, topological polar surface area; nRotB, number of rotatable bonds. |
5a |
333.11 |
2.00 |
1 |
5 |
72.28 |
5b |
347.13 |
2.53 |
1 |
5 |
72.28 |
5c |
361.15 |
3.06 |
1 |
5 |
72.28 |
5d |
375.16 |
3.59 |
1 |
5 |
72.28 |
5e |
367.10 |
1.98 |
1 |
5 |
72.28 |
5f |
385.09 |
2.13 |
1 |
5 |
72.28 |
5g |
401.06 |
2.70 |
1 |
5 |
72.28 |
5h |
435.09 |
2.87 |
1 |
5 |
72.28 |
5i |
381.11 |
2.48 |
1 |
5 |
72.28 |
10a |
355.10 |
1.85 |
1 |
5 |
72.28 |
10b |
373.09 |
1.99 |
1 |
5 |
72.28 |
10c |
373.09 |
1.99 |
1 |
5 |
72.28 |
10d |
373.09 |
1.99 |
1 |
5 |
72.28 |
10e |
389.06 |
2.56 |
1 |
5 |
72.28 |
10f |
389.06 |
2.56 |
1 |
5 |
72.28 |
10g |
389.06 |
2.56 |
1 |
5 |
72.28 |
10h |
423.09 |
2.73 |
1 |
5 |
72.28 |
10i |
423.09 |
2.73 |
1 |
5 |
72.28 |
10j |
423.09 |
2.73 |
1 |
5 |
72.28 |
10k |
369.11 |
2.35 |
1 |
5 |
72.28 |
10l |
369.11 |
2.35 |
1 |
5 |
72.28 |
10m |
369.11 |
2.35 |
1 |
5 |
72.28 |
In order to ensure that the compound has good pharmacokinetic properties, the ADME/T module in the DS 2019 software package is used to predict the pharmacokinetic properties of target compounds 5a–i and 10a–m (e.g. absorption, blood–brain barrier permeability) to avoid the compound's difficulty in penetrating the blood–brain barrier (BBB), low solubility and low oral bioavailability. The prediction results showed that all target compounds exhibited good absorption (ABS) and favorable BBB permeability (Fig. 10).
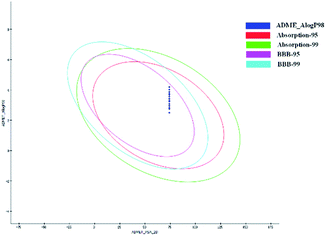 |
| Fig. 10 Target compounds 5a–i and 10a–m ADME/T value prediction. The blue dots in the four circular circles indicate that the compound has good pharmacokinetic properties, such as good ABS and favorable BBB permeability. | |
3. Experimental section
3.1 Chemistry
X-4 binocular microscope melting point apparatus was used to determine the melting point of the compound. The target compound was measured by dissolving in CDCl3 or DMSOd6, and tetramethylsilane was selected as an internal standard. 1H and 13C spectrum data were measured by a Bruker AV-300 nuclear magnetic resonance spectrometer. Abbreviations of spectral data are as follows: s, singlet; d, doublet; t, triplet; q, quartet; m, multiplet). Infrared (IR) is measured by an FT-IR1730 infrared spectrophotometer using the KBr tablet method. High-resolution mass spectra (HRMS) was determined by the mass spectrometer produced by Bruker Daltonik in the Germany. The solvent and reagent used were of analytical or chemical grade (Aladdin, China Reagent Network, Shenyang Chemical Reagent Factory, Sigma-Aldrich). The silica gel used for thin-layer plates and column chromatography was produced by Qingdao Ocean Chemical Factory.
3.1.1. Synthesis of intermediates 2 and 7. 7-Hydroxy-3,4-dihydroquinolin-2(1H)-one/N-(3-hydroxyphenyl)acetamide (10.0 mmol) and K2CO3 (11.0 mmol) were added to a 100 ml single-necked round bottom flask; to this, 30 ml CH3CN was added and the mixture was stirred at 70 °C for 0.5 h. After 0.5 h, methyl bromoacetate (10.5 mmol) was added to continue the reaction for 6 h. The reaction was followed by thin layer chromatography (TLC) detection. After the reaction was completed, the solvent was pumped off under reduced pressure in vacuo to obtain a brown solid. Next, 30 ml of water was added to the round bottom flask, and then the solution was stirred for 10 min, filtered with suction, washed three times with water, and dried to obtain intermediates 2 and 7.
3.1.2. Synthesis of intermediates 3 and 8. Intermediate 2/7 (10.0 mmol) and hydrazine hydrate (11.0 mmol) were added to a 100 ml single-necked round bottom flask, then 30 ml of THF was added, and the reaction was stirred at reflux for 2 h. TLC detection followed the reaction. After the reaction was completed, the excess THF was evaporated under vacuum to obtain the crude product. Add 10 ml CH3OH to the solid, stirred for 5 min, suction filtered, washed with cooled CH3OH, dried to obtain intermediates 3 and 8.
3.1.3. Synthesis of intermediates 4 and 9. Add intermediate 3/8 (10.0 mmol), NaOH (15.0 mmol) and CS2 (20.0 mmol) to a 100 ml single-necked round bottom flask, then add 30 ml EtOH, stir the reaction at 80 °C for 12 h, then add CS2 (5.0 mmol) continued to react for 7 h. TLC detection tracking reaction, after the completion of the reaction, add 10% HCl solution to adjust the pH to 4–5, until a large amount of white solid precipitated. Suction filtration, washed 3 times with water to obtain a white solid, and dried to obtain intermediates 4 and 9.
3.1.4. Synthesis of target compounds 5a–i and 10a–m. Intermediate 4/9 (10.0 mmol) and K2CO3 (11.0 mmol) were added to a 100 ml single-necked round bottom flask, then 30 ml of acetone was added, and the reaction was stirred at 65 °C for 1 h. Then bromine (10.5 mmol) was added to continue the reaction for 9 h. The reaction was tracked by TLC detection. After the reaction was completed, the solvent was vacuum dried under reduced pressure to obtain a white solid. To the white solid was added 30 ml of water, stirred for 10 min, filtered with suction, washed with water 3 times, dried, purified by column chromatography (CH3OH
:
CH2Cl2 = 1
:
100) to obtain the target compounds 5a–i and 10a–m.
7-((5-(Butylthio)-1,3,4-oxadiazol-2-yl)methoxy)-3,4-dihydroquinolin-2(1H)-one (5a)
Yield: 56%. Mp: 184–185 °C. 1H-NMR (CDCl3, 400 MHz, ppm) δ: 8.42 (s, 1H, CONH), 6.48–7.09 (m, 3H, Ar-H), 5.20 (s, 2H, OCH2), 3.26 (t, 2H, J = 8.0 Hz, SCH2), 2.91 (t, 2H, J = 8.0 Hz, COCH2), 2.62 (t, 2H, J = 8.0 Hz,
), 1.43–1.82 (m, 4H, CH2), 0.95 (t, 3H, J = 8.0 Hz, CH3). 13C NMR (100 MHz, CDCl3) δ: 171.7, 166.5, 162.9, 157.0, 138.4, 128.9, 117.48, 108.7, 102.8, 59.9, 32.2, 31.1, 30.8, 24.6, 21.7, 13.5. IR (KBr, cm−1): 1673 (C
O). ESI-HRMS calcd for C16H20N3O3S+ ([M + H]+): 334.1220; found: 334.1216.
7-((5-(Pentylthio)-1,3,4-oxadiazol-2-yl)methoxy)-3,4-dihydroquinolin-2(1H)-one (5b)
Yield: 47%. Mp: 191–192 °C. 1H-NMR (CDCl3, 400 MHz, ppm) δ: 7.94 (s, 1H, CONH), 6.44–7.09 (m, 3H, Ar-H), 5.19 (s, 2H, OCH2), 3.25 (t, 2H, J = 8.0 Hz, SCH2), 2.91 (t, 2H, J = 8.0 Hz, COCH2), 2.62 (t, 2H, J = 8.0 Hz,
), 1.32–1.84 (m, 6H, CH2), 0.91 (t, 3H, J = 8.0 Hz, CH3). 13C NMR (100 MHz, DMSOd6) δ: 170.7, 165.3, 164.0, 156.8, 139.8, 129.0, 117.5, 107.9, 102.8, 59.9, 32.4, 31.0, 30.4, 29.0, 24.4, 22.0, 14.2. IR (KBr, cm−1): 1677 (C
O). ESI-HRMS calcd for C17H22N3O3S+ ([M + H]+): 348.1376; found: 348.1372.
7-((5-(Hexylthio)-1,3,4-oxadiazol-2-yl)methoxy)-3,4-dihydroquinolin-2(1H)-one (5c)
Yield: 51%. Mp: 176–177 °C. 1H-NMR (CDCl3, 400 MHz, ppm) δ: 7.86 (s, 1H, CONH), 6.43–7.09 (m, 3H, Ar-H), 5.19 (s, 2H, OCH2), 3.26 (t, 2H, J = 8.0 Hz, SCH2), 2.91 (t, 2H, J = 8.0 Hz, COCH2), 2.62 (t, 2H, J = 8.0 Hz,
), 1.25–1.83 (m, 8H, CH2), 0.89 (t, 3H, J = 8.0 Hz, CH3). 13C NMR (100 MHz, DMSOd6) δ: 170.7, 165.3, 164.0, 156.9, 139.8, 129.0, 117.5, 107.9, 102.8, 59.9, 32.4, 31.1, 29.3, 27.9, 24.5, 22.4, 14.3. IR (KBr, cm−1): 1677 (C
O). ESI-HRMS calcd for C18H24N3O3S+ ([M + H]+): 362.1533; found: 362.1529.
7-((5-(Heptylthio)-1,3,4-oxadiazol-2-yl)methoxy)-3,4-dihydroquinolin-2(1H)-one (5d)
Yield: 44%. Mp: 182–183 °C. 1H-NMR (CDCl3, 400 MHz, ppm) δ: 8.08 (s, 1H, CONH), 6.45–7.09 (m, 3H, Ar-H), 5.19 (s, 2H, OCH2), 3.25 (t, 2H, J = 8.0 Hz, SCH2), 2.91 (t, 2H, J = 8.0 Hz, COCH2), 2.62 (t, 2H, J = 8.0 Hz,
), 1.27–1.84 (m, 10H, CH2), 0.88 (t, 3H, J = 8.0 Hz, CH3). 13C NMR (100 MHz, DMSOd6) δ: 170.7, 165.3, 164.0, 156.8, 139.8, 129.0, 117.5, 107.9, 102.8, 59.9, 32.4, 31.5, 31.0, 29.3, 28.5, 28.2, 24.4, 22.5, 14.4. IR (KBr, cm−1): 1674 (C
O). ESI-HRMS calcd for C19H26N3O3S+ ([M + H]+): 376.1689; found: 376.1686.
7-((5-(Benzylthio)-1,3,4-oxadiazol-2-yl)methoxy)-3,4-dihydroquinolin-2(1H)-one (5e)
Yield: 48%. Mp: 195–196 °C. 1H-NMR (CDCl3, 400 MHz, ppm) δ: 10.10 (s, 1H, CONH), 6.52–7.43 (m, 8H, Ar-H), 5.31 (s, 2H, OCH2), 4.51 (s, 2H, SCH2), 2.79 (t, 2H, J = 8.0 Hz, COCH2), 2.42 (t, 2H, J = 8.0 Hz,
). 13C NMR (100 MHz, CDCl3) δ: 170.7, 164.7, 164.2, 156.8, 139.8, 136.8, 129.4, 129.4, 129.0, 129.0, 128.2, 117.5, 107.9, 102.8, 59.9, 36.2, 31.0, 24.4. IR (KBr, cm−1): 1674 (C
O). ESI-HRMS calcd for C19H18N3O3S+ ([M + H]+): 368.1063; found: 368.1061.
7-((5-((4-Fluorobenzyl)thio)-1,3,4-oxadiazol-2-yl)methoxy)-3,4-dihydroquinolin-2(1H)-one (5f)
Yield: 42%. Mp: 171–172 °C. 1H-NMR (CDCl3, 400 MHz, ppm) δ: 10.09 (s, 1H, CONH), 7.08–7.48 (dd, 4H, J = 3.0 Hz, J = 3.0 Hz, Ar-H), 6.52–7.16 (m, 3H, Ar–H), 5.31 (s, 2H, OCH2), 4.51 (s, 2H, SCH2), 2.79 (t, 2H, J = 8.0 Hz, COCH2), 2.42 (t, 2H, J = 8.0 Hz,
). 13C NMR (100 MHz, CDCl3) δ: 170.7, 164.6, 164.2, 163.2 (d, 1Jc–f = 243.0 Hz), 156.8, 139.8, 133.2 (d, 3Jc–f = 3.0 Hz), 131.6, 131.6, 131.5, 131.5, 129.0, 117.5, 115.9 (d, 2Jc–f = 21.0 Hz), 107.9, 102.7, 59.9, 35.4, 31.0, 24.4. IR (KBr, cm−1): 1674 (C
O). ESI-HRMS calcd for C19H17FN3O3S+ ([M + H]+): 386.0969; found: 386.0967.
7-((5-((4-Chlorobenzyl)thio)-1,3,4-oxadiazol-2-yl)methoxy)-3,4-dihydroquinolin-2(1H)-one (5g)
Yield: 43%. Mp: 188–189 °C. 1H-NMR (CDCl3, 400 MHz, ppm) δ: 10.09 (s, 1H, CONH), 7.35–7.45 (dd, 4H, J = 4.0 Hz, J = 4.0 Hz, Ar-H), 6.51–6.62 (m, 3H, Ar-H), 5.31 (s, 2H, OCH2), 4.50 (s, 2H, SCH2), 2.79 (t, 2H, J = 8.0 Hz, COCH2), 2.42 (t, 2H, J = 8.0 Hz,
). 13C NMR (100 MHz, CDCl3) δ: 170.7, 164.5, 164.3, 156.8, 139.8, 136.1, 132.8, 131.3, 131.3, 129.0, 128.9, 128.9, 117.5, 107.9, 102.7, 59.9, 35.4, 31.0, 24.4. IR (KBr, cm−1): 1672 (C
O). ESI-HRMS calcd for C19H17ClN3O3S+ ([M + H]+): 402.0674; found: 402.0670.
7-((5-((4-Trifluoromethylbenzyl)thio)-1,3,4-oxadiazol-2-yl)methoxy)-3,4-dihydroquinolin-2(1H)-one (5h)
Yield: 37%. Mp: 199–201 °C. 1H-NMR (CDCl3, 400 MHz, ppm) δ: 7.97 (s, 1H, CONH), 7.54–7.59 (dd, 4H, J = 4.0 Hz, J = 4.0 Hz, Ar-H), 6.43–7.09 (m, 3H, Ar-H), 5.19 (s, 2H, OCH2), 4.50 (s, 2H, SCH2), 2.91 (t, 2H, J = 8.0 Hz, COCH2), 2.62 (t, 2H, J = 8.0 Hz,
). 13C NMR (100 MHz, CDCl3) δ: 170.7, 164.4, 156.8, 142.0 (d, 1Jc–f = 223.0 Hz), 130.2, 130.2, 130.2, 129.0, 125.8, 125.8, 123.2, 117.5, 107.9, 102.7, 59.9, 35.4, 31.0, 24.4. IR (KBr, cm−1): 1672 (C
O). ESI-HRMS calcd for C20H17F3N3O3S+ ([M + H]+): 436.0937; found: 436.0932.
7-((5-((4-Methylbenzyl)thio)-1,3,4-oxadiazol-2-yl)methoxy)-3,4-dihydroquinolin-2(1H)-one (5i)
Yield: 41%. Mp: 182–183 °C. 1H-NMR (CDCl3, 400 MHz, ppm) δ: 8.59 (s, 1H, CONH), 7.12–7.31 (dd, 4H, J = 4.0 Hz, J = 4.0 Hz, Ar-H), 6.49–7.08 (m, 3H, Ar–H), 5.18 (s, 2H, OCH2), 4.45 (s, 2H, SCH2), 2.90 (t, 2H, J = 8.0 Hz, COCH2), 2.62 (t, 2H, J = 8.0 Hz,
), 2.33 (s, 3H, CH3). 13C NMR (100 MHz, CDCl3) δ: 171.8, 165.8, 163.1, 157.0, 138.49, 138.0, 132.1, 129.5, 129.5, 129.0, 129.0, 128.9, 117.4, 108.7, 102.8, 99.9, 59.9, 36.5, 30.8, 24.6, 21.1. IR (KBr, cm−1): 1675 (C
O). ESI-HRMS calcd for C20H20N3O3S+ ([M + H]+): 382.1220; found: 382.1217.
N-(3-((5-(Benzylthio)-1,3,4-oxadiazol-2-yl)methoxy)phenyl)acetamide (10a)
Yield: 40%. Mp: 188–189 °C. 1H-NMR (CDCl3, 300 MHz, ppm) δ: 7.52 (s, 1H, CONH), 6.71–7.42 (m, 9H, Ar-H), 5.18 (s, 2H, OCH2), 4.47 (s, 2H, SCH2), 2.16 (s, 3H, COCH3). 13C NMR (75 MHz, CDCl3) δ: 168.6, 165.2, 163.4, 157.8, 139.4, 134.4, 131.8, 131.8, 130.7, 130.7, 129.9, 122.1, 113.4, 110.4, 106.5, 59.6, 35.9, 24.5. IR (KBr, cm−1): 1676 (C
O). ESI-HRMS calcd for C18H18N3O3S+ ([M + H]+): 356.1063; found: 356.1059.
N-(3-((5-((2-Fluorobenzyl)thio)-1,3,4-oxadiazol-2-yl)methoxy)phenyl)acetamide (10b)
Yield: 62%. Mp: 189–190 °C. 1H-NMR (CDCl3, 300 MHz, ppm) δ: 7.35 (s, 1H, CONH), 6.72–7.50 (m, 8H, Ar-H), 5.19 (s, 2H, OCH2), 4.49 (s, 2H, SCH2), 2.16 (s, 3H, COCH3). 13C NMR (75 MHz, CDCl3) δ: 168.5, 163.4, 162.5 (d,1Jc–f = 246.7 Hz), 157.8, 139.3, 131.2 (d, 3Jc–f = 3.0 Hz), 130.0 (d, 2Jc–f = 8.2 Hz), 129.8, 124.2 (d, 3Jc–f = 3.7 Hz), 122.6 (d, 2Jc–f = 14.2 Hz), 115.7, 115.4, 113.3, 110.4, 106.4, 59.6, 30.0, 24.5. IR (KBr, cm−1): 1671 (C
O). ESI-HRMS calcd for C18H17FN3O3S+ ([M + H]+): 374.0969; found: 374.0966.
N-(3-((5-((3-Fluorobenzyl)thio)-1,3,4-oxadiazol-2-yl)methoxy)phenyl)acetamide (10c)
Yield: 55%. Mp: 174–176 °C. 1H-NMR (CDCl3, 300 MHz, ppm) δ: 7.48 (s, 1H, CONH), 6.71–7.36 (m, 8H, Ar-H), 5.19 (s, 2H, OCH2), 4.44 (s, 2H, SCH2), 2.16 (s, 3H, COCH3). 13C NMR (75 MHz, CDCl3) δ: 168.6, 165.2, 164.3 (d, 1Jc–f = 245.2 Hz), 157.7, 139.3, 137.7 (d, 3Jc–f = 7.5 Hz), 130.3 (d, 2Jc–f = 8.2 Hz), 129.8, 124.7 (d, 3Jc–f = 3.0 Hz), 116.1 (d, 2Jc–f = 22.5 Hz), 115.2, 114.9, 113.4, 110.4, 106.4, 59.6, 36.0, 24.5. IR (KBr, cm−1): 1676 (C
O). ESI-HRMS calcd for C18H17FN3O3S+ ([M + H]+): 374.0969; found: 374.0965.
N-(3-((5-((4-Fluorobenzyl)thio)-1,3,4-oxadiazol-2-yl)methoxy)phenyl)acetamide (10d)
Yield: 52%. Mp: 182–183 °C. 1H-NMR (CDCl3, 300 MHz, ppm) δ: 7.52 (s, 1H, CONH), 6.71–7.40 (m, 8H, Ar-H), 5.18 (s, 2H, OCH2), 4.43 (s, 2H, SCH2), 2.16 (s, 3H, COCH3). 13C NMR (75 MHz, CDCl3) δ: 168.4, 164.0 (d, 1Jc–f = 246.0 Hz), 157.8, 139.3, 131.1 (d, 3Jc–f = 3.0 Hz), 130.8 (d, 2Jc–f = 8.2 Hz), 129.9, 129.9, 115.8, 115.8, 115.5, 113.3, 110.5, 106.4, 59.6, 35.9, 24.5, 17.2. IR (KBr, cm−1): 1674 (C
O). ESI-HRMS calcd for C18H17FN3O3S+ ([M + H]+): 374.0969; found: 374.0965.
N-(3-((5-((2-Chlorobenzyl)thio)-1,3,4-oxadiazol-2-yl)methoxy)phenyl)acetamide (10e)
Yield: 47%. Mp: 176–178 °C. 1H-NMR (CDCl3, 300 MHz, ppm) δ: 7.46 (s, 1H, CONH), 6.71–7.56 (m, 8H, Ar-H), 5.18 (s, 2H, OCH2), 4.57 (s, 2H, SCH2), 2.16 (s, 3H, COCH3). 13C NMR (75 MHz, CDCl3) δ: 168.7, 165.5, 163.4, 157.7, 139.4, 134.2, 133.2, 131.3, 129.8, 129.7, 129.6, 127.0, 113.4, 110.3, 106.4, 59.6, 34.4, 24.5. IR (KBr, cm−1): 1673 (C
O). ESI-HRMS calcd for C18H17ClN3O3S+ ([M + H]+): 390.0674; found: 390.0670.
N-(3-((5-((3-Chlorobenzyl)thio)-1,3,4-oxadiazol-2-yl)methoxy)phenyl)acetamide (10f)
Yield: 52%. Mp: 191–193 °C. 1H-NMR (CDCl3, 300 MHz, ppm) δ: 7.50 (s, 1H, CONH), 6.71–7.42 (m, 8H, Ar–H), 5.18 (s, 2H, OCH2), 4.42 (s, 2H, SCH2), 2.16 (s, 3H, COCH3). 13C NMR (75 MHz, CDCl3) δ: 168.6, 165.1, 163.4, 157.7, 139.3, 137.3, 134.4, 129.9, 129.8, 129.0, 128.3, 127.2, 113.4, 110.4, 106.4, 59.6, 35.9, 24.5. IR (KBr, cm−1): 1675 (C
O). ESI-HRMS calcd for C18H17ClN3O3S+ ([M + H]+): 390.0674; found: 390.0670.
N-(3-((5-((4-Chlorobenzyl)thio)-1,3,4-oxadiazol-2-yl)methoxy)phenyl)acetamide (10g)
Yield: 43%. Mp: 195–196 °C. 1H-NMR (CDCl3, 300 MHz, ppm) δ: 7.50 (s, 1H, CONH), 6.71–7.36 (m, 8H, Ar-H), 5.18 (s, 2H, OCH2), 4.41 (s, 2H, SCH2), 2.16 (s, 3H, COCH3). 13C NMR (75 MHz, CDCl3) δ: 168.6, 165.2, 163.3, 157.7, 139.4, 133.9, 133.8, 130.4, 130.4, 129.8, 128.8, 128.8, 113.4, 110.3, 106.4, 59.6, 35.8, 24.5. IR (KBr, cm−1): 1671 (C
O). ESI-HRMS calcd for C18H17ClN3O3S+ ([M + H]+): 390.0674; found: 390.0669.
N-(3-((5-((2-Trifluoromethylbenzyl)thio)-1,3,4-oxadiazol-2-yl)methoxy)phenyl)acetamide (10h)
Yield: 49%. Mp: 188–189 °C. 1H-NMR (CDCl3, 300 MHz, ppm) δ: 7.65 (s, 1H, CONH), 6.71–7.71 (m, 8H, Ar-H), 5.18 (s, 2H, OCH2), 4.66 (s, 2H, SCH2), 2.15 (s, 3H, COCH3). 13C NMR (75 MHz, CDCl3) δ: 168.5, 165.5, 163.5, 157.8, 139.3, 134.0, 132.3, 131.9, 129.8, 128.3, 126.4 (q, Jc–f = 5.5 Hz), 125.9, 122.2, 113.4, 110.5, 106.4, 59.6, 33.1, 24.5. IR (KBr, cm−1): 1675 (C
O). ESI-HRMS calcd for C19H17F3N3O3S+ ([M + H]+): 424.0937; found: 424.0933.
N-(3-((5-((3-Trifluoromethylbenzyl)thio)-1,3,4-oxadiazol-2-yl)methoxy)phenyl)acetamide (10i)
Yield: 50%. Mp: 190–192 °C. 1H-NMR (CDCl3, 300 MHz, ppm) δ: 7.69 (s, 1H, CONH), 6.72–7.65 (m, 8H, Ar-H), 5.19 (s, 2H, OCH2), 4.51 (s, 2H, SCH2), 2.17 (s, 3H, COCH3). 13C NMR (75 MHz, CDCl3) δ: 168.5, 165.0, 163.4, 157.8, 139.3, 136.4, 132.4, 131.3, 129.8, 129.2, 125.7 (q, Jc–f = 11.2 Hz), 125.5, 124.9 (q, Jc–f = 11.2 Hz), 113.4, 110.4, 106.4, 59.6, 35.9, 24.5. IR (KBr, cm−1): 1671 (C
O). ESI-HRMS calcd for C19H17F3N3O3S+ ([M + H]+): 424.0937; found: 424.0934.
N-(3-((5-((4-Trifluoromethylbenzyl)thio)-1,3,4-oxadiazol-2-yl)methoxy)phenyl)acetamide (10j)
Yield: 45%. Mp: 176–177 °C. 1H-NMR (CDCl3, 300 MHz, ppm) δ: 7.59 (s, 1H, CONH), 6.72–7.59 (m, 8H, Ar-H), 5.20 (s, 2H, OCH2), 4.49 (s, 2H, SCH2), 2.17 (s, 3H, COCH3). 13C NMR (75 MHz, CDCl3) δ: 168.5, 165.0, 163.4, 162.4 (d, 1Jc–f = 240.7 Hz), 157.8, 139.5 (d, 2Jc–f = 17.2 Hz), 129.9, 129.4, 129.4, 129.4, 125.7, 125.7 (d, 3Jc–f = 3.7 Hz), 122.0, 113.3, 110.4, 106.4, 59.6, 35.8, 24.6. IR (KBr, cm−1): 1677 (C
O). ESI-HRMS calcd for C19H17F3N3O3S+ ([M + H]+): 424.0937; found: 424.0933.
N-(3-((5-((2-Methylbenzyl)thio)-1,3,4-oxadiazol-2-yl)methoxy)pheenyl)acetamide (10k)
Yield: 62%. Mp: 176–178 °C. 1H-NMR (CDCl3, 300 MHz, ppm) δ: 7.46 (s, 1H, CONH), 6.73–7.37 (m, 8H, Ar-H), 5.20 (s, 2H, OCH2), 4.51 (s, 2H, SCH2), 2.42 (s, 3H, Ar-H), 2.17 (s, 3H, COCH3). 13C NMR (75 MHz, CDCl3) δ: 168.4, 167.7, 163.2, 157.9, 139.3, 137.0, 132.6, 130.7, 130.2, 129.9, 128.5, 126.3, 113.3, 110.5, 106.4, 59.7, 35.0, 24.6, 19.1. IR (KBr, cm−1): 1675 (C
O). ESI-HRMS calcd for C19H20N3O3S+ ([M + H]+): 370.1220; found: 370.1215.
N-(3-((5-((3-Methylbenzyl)thio)-1,3,4-oxadiazol-2-yl)methoxy)phenyl)acetamide (10l)
Yield: 65%. Mp: 160–162 °C. 1H-NMR (CDCl3, 300 MHz, ppm) δ: 7.54 (s, 1H, CONH), 6.72–7.35 (m, 8H, Ar-H), 5.18 (s, 2H, OCH2), 4.44 (s, 2H, SCH2), 2.33 (s, 3H, Ar-H), 2.16 (s, 3H, COCH3). 13C NMR (75 MHz, CDCl3) δ: 168.4, 165.7, 163.2, 157.9, 139.3, 138.5, 134.9, 129.9, 129.7, 128.9, 128.6, 126.0, 113.3, 110.5, 106.4, 59.7, 36.7, 24.6, 21.2. IR (KBr, cm−1): 1677 (C
O). ESI-HRMS calcd for C19H20N3O3S+ ([M + H]+): 370.1220; found: 370.1216.
N-(3-((5-((4-Methylbenzyl)thio)-1,3,4-oxadiazol-2-yl)methoxy)phenyl)acetamide (10m)
Yield: 48%. Mp: 169–170 °C. 1H-NMR (CDCl3, 300 MHz, ppm) δ: 7.34 (s, 1H, CONH), 6.73–7.31 (m, 8H, Ar-H), 5.20 (s, 2H, OCH2), 4.45 (s, 2H, SCH2), 2.33 (s, 3H, Ar-H), 2.17 (s, 3H, COCH3). 13C NMR (75 MHz, CDCl3) δ: 168.3, 165.7, 163.1, 157.9, 139.2, 137.9, 132.0, 129.9, 129.4, 129.4, 129.0, 129.0, 113.3, 110.5, 106.3, 59.7, 36.5, 24.6, 21.1, 13.6. IR (KBr, cm−1): 1673 (C
O). ESI-HRMS calcd for C19H20N3O3S+ ([M + H]+): 370.1220; found: 370.1215.
3.2 Pharmacology
3.2.1. Animals and treatment. Kunming mice (18–22 g) and Wistar rats (280–300 g) were used for the experiment. All animals were free to move, eat, and drink before the experiment. All animal procedures were performed in accordance with the Guidelines for Care and Use of Laboratory Animals of Liaocheng University and experiments were approved by the Animal Ethics Committee of Liaocheng University (no. 20190312). We dissolved the target compound in 0.5% methylcellulose or dimethyl sulfoxide, and the target compound was administered via the intraperitoneal or subcutaneous injection method. Fluoxetine and serotonin were used as the positive control. The antidepressant activity of the target compound was initially screened by FST method (in vivo), and several compounds with potential activity were selected, and the affinity was measured by 5-HT1A binding assay (Ki, in vitro). 5-HT ELISA kits test the effect of the most active compound (10g) in the two experiments on the concentration of 5-HT in the mice brain. The TST method was used to further verify the in vivo antidepressant activity of the best active compound. At the same time, the open-field test method was used to test the effect of the compound on the exploratory activity of experimental mice. DS 2019 or ChemBioDraw Ultra 14.0 was used to predict the physicochemical and pharmacokinetic properties of the target compound.
3.2.2. Antidepressant activity evaluation. Antidepressant activity test were conducted in accordance with previously reported methods: FST model16 was used for antidepressant activity testing, and the TST model17,18 was further used for antidepressant activity testing. Through preliminary antidepressant activity test, five compounds (5g-h, 10e, 10g, and 10j) with potential activity were selected, and their affinities were determined, which was performed according to previously reported methods (the binding affinity for 5-HT1A receptor was determined by competition binding studies with [3H] 8-OH-DPAT using racemic 8-OH-DPAT as the reference compound), with a slight modification (10 μg membrane protein, 1 nM [3H]8-OH-DPAT, buffer containing 50 mM Tris-HCl (pH 7.4), 10 mM MgSO4, 0.5 mM EDTA and 0.1% ascorbic acid).19 Among these compounds, compound 10g, which showed high activity, was assessed for its effect on the 5-HT concentration in the mice. The 5-HT content was measured according to previously reported method.7 In order to evaluate the exploratory activity of the animals, the spontaneous activity of compound 10g was tested in the open-field test.20
3.2.3. Molecular docking studies, physicochemical, and pharmacokinetic property prediction. In this study, we selected the FASTA sequence of four 5-HT1A receptors with high homology from RCSB-PDB (web site: https://www.rcsb.org/) (PDB: 4IAQ, 6G79, 4IAR, and 5V54). Then, 2019 DS software was used to construct a homology model of 5-HT1A receptor. The molecular docking module (CDOCKER) in the DS 2019 software was used for docking, and the highest score in the CDOCKER score was analyzed. DS 2019 and ChemBioDraw Ultra 14.0 were used to construct the target compound's structural formula and predict the physicochemical and pharmacokinetic properties, such as MW, RotB, CLogP, nHBD, nHBA, TPSA, ABS level, and BBB permeability level of the target compound.
3.2.4. Statistical analysis. Data are expressed as mean ± standard deviation. One-way analysis of variance (ANOVA) was performed using GraphPad Prism 5.0 statistical software; 0.01 < p < 0.05 indicated statistically significant differences, p < 0.01 indicated highly significant differences.
4. Conclusions
In this study, we designed and synthesized two series of 1,3,4-oxadiazole derivatives as new antidepressant compounds. Most of the compounds showed significant antidepressant effects at dose of 40 mg kg−1. Especially, compound N-(3-((5-((4-chlorobenzyl)thio)-1,3,4-oxadiazol-2-yl)methoxy)phenyl)acetamide (10g), showed the best antidepressant activity in FST model, similar to the activity of positive drug fluoxetine. The 5-HT1A receptor binding assay showed compound 10g as the most suitable compound, with an value of Ki = 1.52 nM and high binding to 5-HT1A receptors. The results of the in vivo 5-HT concentration estimation in mice showed that compound 10g may have an effect on the mice brain, it can increase the concentration of 5-HT in the brain of mice. In the open-field test, compound 10g did not affect spontaneous activity. Molecular docking showed that compound 10g had significant interaction with the amino acid residues around the active site of 5-HT1A receptors. Prediction of the physicochemical and pharmacokinetic properties of the target compounds using DS 2019 and CHEMDRAW 14.0 software showed that all compounds had good physicochemical properties, as well as good BBB permeability and bioavailability.
Conflicts of interest
There are no conflicts to declare.
Acknowledgements
This work was supported by the Natural Science Foundation of Shandong (No. ZR2017BH037); National Natural Science Foundation of China (No. 21675071, 31701827 and 81803360); Youth Innovative Science and Technology Program of Shandong Colleges and University (2019KJM012); The Open Project of Shandong Collaborative Innovation Center for Antibody Drugs (No. CIC-AD1817, CIC-AD1820, CIC-AD1825).
Notes and references
- J. Olesen, P. Sobscki, T. Truelsen, D. Sestoft and B. Jonsson, Nord. J. Psychiatry, 2008, 62, 114 CrossRef PubMed.
- P. Andlin-Sobocki, B. Jonsson, H. U. Wittchen and J. Olesen, Eur. Med. J., Neurol., 2005, 12, 1 CrossRef PubMed.
- J. M. Ferguson, Prim. Care Companion J. Clin. Psychiatry, 2001, 3, 22 CrossRef PubMed.
- H. M. Vasiliadis, E. Latimer, P. A. Dionne and M. Préville, Can. J. Psychiatry, 2013, 58, 201 CrossRef PubMed.
- P. Singh, P. K. Sharma, J. K. Sharma, A. Upadhyay and N. Kumar, Org. Med. Chem. Lett., 2012, 2, 8 CrossRef PubMed.
- X. Q. Deng, M. X. Song, Y. Zheng and Z. S. Quan, Eur. J. Med. Chem., 2014, 73, 217 CrossRef CAS PubMed.
- S. B. Wang, H. Liu, G. Y. Li, J. Li, X. J. Li, K. Lei, L. C. Wei, Z. S. Quan, X. K. Wang and R. M. Liu, Pharmacol. Rep., 2019, 71, 1244 CrossRef CAS PubMed.
- N. S. Goud, S. M. Ghouse, M. Arifuddin, M. Alvala, A. Angeli and C. T. Supuran, Bioorg. Chem., 2019, 87, 765 CrossRef PubMed.
- J. H. Meyer, S. McMain, S. H. Kennedy, L. Korman, G. M. Brown, J. N. DaSilva, A. A. Wilson, T. Blak, R. Eynan-Harvey, V. S. Goulding, S. Houle and P. Links, Am. J. Psychiatry, 2003, 160, 90 CrossRef PubMed.
- P. M. Galdino, M. V. Nascimento, B. L. Sampaio, R. N. Ferreira, J. R. Paula and E. A. Costa, J. Ethnopharmacol., 2009, 124, 581 CrossRef CAS PubMed.
- H. Sakakibara, K. Ishida, O. Grundmann, J. Nakajima, S. Seo, V. Butterweck, Y. Minami, S. Saito, Y. Kawai, Y. Nakaya and J. Terao, Biol. Pharm. Bull., 2006, 29, 1767 CrossRef CAS PubMed.
- K. Ostrowska, D. Grzeszczuk, M. Głuch-Lutwin, A. Grybos, A. Siwek, A. Lesniak, M. Sacharczuk and B. Trzaskowsk, Bioorg. Med. Chem., 2018, 26, 527 CrossRef CAS PubMed.
- P. Blier and N. M. Ward, Biol. Psychiatry, 2003, 53, 93 CrossRef.
- D. R. Sherin, C. K. Geethu, J. Prabhakaran, J. J. Mann, J. S. Dileep and T. K. Manojkumar, Comput. Biol. Chem., 2019, 78, 108 CrossRef CAS PubMed.
- N. L. Allinger, J. Am. Chem. Soc., 1977, 99, 8127 CrossRef CAS.
- R. D. Porsolt, A. Bertin and M. Jalfre, Arch. Int. Pharmacodyn. Ther., 1977, 229, 327 CAS.
- L. Stéru, R. Chermat, B. Thierry and P. Simon, Psychopharmacology, 1985, 85, 367 CrossRef PubMed.
- M. Chatterjee, P. Verma and G. Palit, Indian J. Exp. Biol., 2010, 48, 306 Search PubMed.
- Z. S. Gu, Y. Xiao, Q. W. Zhang and J. Q. Li, Bioorg. Med. Chem. Lett., 2017, 27, 5420 CrossRef CAS PubMed.
- P. J. Elliott, J. Chan, Y. M. Parker and C. B. Nemeroff, Brain Res., 1986, 381, 259 CrossRef CAS PubMed.
Footnote |
† Electronic supplementary information (ESI) available. See DOI: 10.1039/d0ra05886f |
|
This journal is © The Royal Society of Chemistry 2020 |