DOI:
10.1039/D0RA05374K
(Paper)
RSC Adv., 2020,
10, 31662-31669
Biocompatible ionic liquid [Betaine][H2PO4] as a reusable catalyst for the substitution of xanthen-9-ol under solvent-free conditions†
Received
19th June 2020
, Accepted 8th August 2020
First published on 27th August 2020
Abstract
An ionic liquid, namely [Betaine][H2PO4], was found to be an efficient catalyst for the direct substitution reaction of xanthen-9-ol with different nucleophiles under solvent-free conditions. This catalytic system is easy to be operated and the following work-up procedure is simple, with the ionic liquid catalyst reusable for at least five cycles at a high catalytic activity level. In addition, the ionic liquid is easy to prepare and its raw materials are inexpensive and have good biocompatibility. Therefore, our study presents an intriguing and sustainable protocol for the direct substitution of alcohol.
Introduction
As novel functional materials, ionic liquids have been widely utilized in organic synthesis and catalysis,1–8 extraction and separation,9,10 biomass conversion11,12 and electrochemistry13,14 among others. In catalysis, the functions of ionic liquids include being a solvent, catalysts or co-catalysts, ligand for metal catalysts, and even separation assistants.15,16 The cations and anions of ionic liquids have shown significant synergetic effects in different organic syntheses,5,17,18 particularly the hydrogen bonds in functional ionic liquids, which have been proven to be able to simultaneously activate the electrophiles and nucleophiles in reactions and promote the related reactions under moderate conditions.2,19,20 Due to the excellent biocompatibility, easy preparation procedure, and low cost, choline and betaine-based ionic liquids have been utilized in Aldol reactions,21,22 Knoevenagel reactions,23,24 enzymatic reactions,25,26 and in the utilization of CO2.27–29 The inherent tunable properties of the ionic liquids such as acidity/basicity, solubility, and others make them intriguing in numerous kinds of reactions.
Alcohols are ideal substrates to construct C–C and C–X bonds as carbocation resources, which produce water as the sole by-product. However, alcohols traditionally have to be pre-converted to corresponding halides, sulfonates or related compounds with easy leaving groups due to the weak leaving ability of the hydroxyl group. This greatly increases the complexity of the overall processes and decreases the atom efficiency. Therefore, the development of the direct substitution of alcohols has fallen into the aspects of green chemistry.30 The direct substitutions of xanthen-9-ol with different nucleophiles have been realized via the catalysis of numerous Lewis acidic catalysts such as BF3·OEt2 in CH2Cl2,31 1,3-dichloro-tetra-n-butyl-distannoxane in CH2Cl2,32 and Ceric ammonium nitrate in anhydrous methanol.33 These reaction systems benefit from high reactivity, but due to their sensitivities to moisture, the anhydrous reaction conditions are compulsory. Indium Tris(dodecyl sulfonate), which is compatible with water can promote this reaction in aqueous solutions,34 still suffers from the potential metal residual in the product. The aqueous solution of Brønsted acids such as acetic acid,35 TFA,36 d odecylbenzenesulfonic acid37 and gluconid acid38 have also been utilized for this transformation. The limited number of reports on the reutilization of this type of catalysts revel that these systems suffer from catalytic activity decrease from 99% to 89% after five cycles. Alternatively, Cozzi et al.39,40 found an “on-water” catalyst-free nucleophilic substitution method, but excess nucleophilic reagents and long reaction times are necessary. Li et al.41 reported an ionic liquid-promoted substitution method for xanthen-9-ol with [Bmim][BF4] as solvent and catalyst. As they have stated in their manuscript, the ionic liquid [Bmim][BF4] is unstable in moisture and produces strongly acidic HF, which may be the main active species for the substitution reactions. Therefore, efficient catalysis systems for the direct substitution of xanthen-9-ol with mild and easy-prepared catalysts under moderate conditions are still needed.
As our ongoing work on the utilization of ionic liquids in organic syntheses,19,24,27,28,42–44 here, we investigated the catalytic effects of a series of functional ionic liquids on the direct substitution of xanthen-9-ol with different nucleophiles. The results showed that both of the cations and anions of the ionic liquids have significant effects on their catalytic activity for the target reactions, and the ionic liquid betainium dihydrogen phosphate([Betaine][H2PO4]) showed excellent catalytic activity under solvent-free conditions. Numerous nucleophiles such as indoles with different substituted groups, dicarbonyl compounds, malononitrile, pyrrole, pyridine-2-thiol, methimazole or azidotrimethylsilane were able to react with xanthen-9-ol under the catalysis of [Betaine][H2PO4] smoothly, leading to the target substitution products with high product yields. This catalytic system benefits from moderate and solvent-free reaction conditions, the simple operation and work-up procedures, and the reusability of the ionic liquid catalyst for at least five iterations. In addition, the preparation of the ionic liquid catalyst is very simple and its raw materials are biocompatible and inexpensive. All of these aforementioned properties make this catalytic system intriguing for the green chemistry applications.
Experimental section
The synthesis of ionic liquids
The protic ionic liquids were synthesized simply by the neutralization of corresponding amines with equal amount of acids in methanol. Typically, a certain amount of betaine was added into a round bottom flask using anhydrous methanol as the solvent. Under magnetic stirring, an equal amount of acid dissolved in anhydrous methanol was dropwise added to the mixture of betaine and methanol in an ice bath. The reaction was then stirred at 40 °C for 24 h. After the reaction was completed, the solvent was removed by rotary evaporation, and sufficiently dried in a vacuum oven at 40 °C to obtain betaine-based ionic liquids.
The typical procedure for the substitution reaction between xanthen-9-ol and nucleophiles
In a 10 mL round bottom flask, the required amount of xanthen-9-ol (0.5 mmol), indoles (0.5 mmol), and ionic liquid (0.05 mmol) were sequentially added. The reaction was conducted at 50 °C for corresponding time under solvent-free conditions. The whole reaction was monitored by TLC. Upon completion, the reaction system was cooled to room temperature, and then extracted with ethyl acetate and water. The product was dissolved in an organic phase and the ionic liquid was dissolved in an aqueous phase. The crude products could be obtained through the removal of ethyl acetate, and the column chromatography method was used to purify the products. The ionic liquid dissolved in water can be used for the next reaction run directly after it is fully dried in a vacuum drying oven.
Results and discussion
The catalytic performances of different ionic liquids
A series of ionic liquids with different cations and anions (their structures are illustrated in Scheme 1) were synthesized and their catalytic performances for the direct substitutions of xanthen-9-ol were investigated using the reaction between xanthen-9-ol and indole as the model reaction. The results shown in Table 1 suggest that both of the cations and the anions have significant effects on their catalytic activities for the substitution reactions, and when the ionic liquid [Betaine][H2PO4] was used as the catalyst, the target substitution product gave almost quantitative isolated yields under the solvent-free conditions within 4 h. Recently, it has been reported by Harper et al.45 that the increase of the hydrogen bond donor ability of the ionic liquids is beneficial to the direct substitution reactions of xanthen-9-ol. Accordingly, the highest catalytic activity of [Betaine][H2PO4] may be due to the presence of carboxyl group on the cation, which has the potential to increase the hydrogen bond donor ability of the ionic liquid.
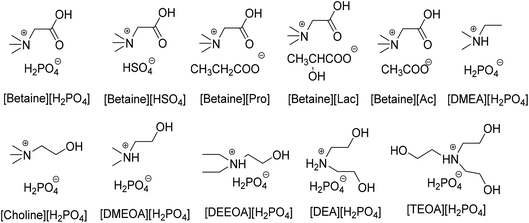 |
| Scheme 1 The structures of ionic liquids. | |
Table 1 Catalytic performances of different ionic liquids on the substitution reaction of xanthen-9-ol and indole
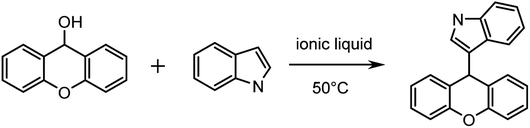
|
Entrya |
IL |
Yieldb (%) |
Entrya |
IL |
Yieldb (%) |
Reaction conditions: xanthen-9-ol (0.5 mmol), indoles (0.5 mmol), IL (0.1 eq.), 50 °C, 4 h. Isolated yields. |
1 |
[Betaine][Ac] |
90 |
7 |
[Choline][H2PO4] |
85 |
2 |
[Betaine][Pro] |
86 |
8 |
[DMEOA][H2PO4] |
88 |
3 |
[Betaine][Lac] |
88 |
9 |
[DEEOA][H2PO4] |
87 |
4 |
[Betaine][H2PO4] |
98 |
10 |
[DEA][H2PO4] |
86 |
5 |
[Betaine][HSO4] |
51 |
11 |
[TEOA][H2PO4] |
90 |
6 |
[DMEA][H2PO4] |
88 |
|
|
|
The influences of the dosage of ionic liquid and reaction temperature
Using the optimized ionic liquid, the influences of the dosage of ionic liquid and reaction temperature on the model reaction were investigated, and the results are shown in Table 2. It can be seen that the increase in the ionic liquid dosage from 0.01 to 0.1 equivalent is beneficial to the target reaction, but further increase in the dosage has no significant influence within 2 h (entries 1–5, Table 2), and the prolonged reaction time to 4 h leads to the completion of the reaction (entry 6, Table 2). The lowered reaction temperature is not beneficial to the reaction (entries 7–8, Table 2).
Table 2 The effect of the amount of ionic liquid on the reaction
Entrya |
Ratio |
Time (h) |
Yieldb (%) |
Reaction conditions: xanthen-9-ol (0.5 mmol), indoles (0.5 mmol), 50 °C. Isolated yields. The reaction under room temperature. The reaction at 40 °C. |
1 |
0.01 |
2 |
80 |
2 |
0.05 |
2 |
85 |
3 |
0.1 |
2 |
93 |
4 |
0.2 |
2 |
93 |
5 |
1.0 |
2 |
93 |
6 |
0.1 |
4 |
98 |
7c |
0.1 |
7 |
87 |
8d |
0.1 |
4 |
90 |
Direct substitution reactions of xanthen-9-ol with different nucleophiles
Using the optimized catalytic system, the direct substitution reactions of xanthen-9-ol with different nucleophiles were investigated and the results are summarized in Table 3. The results showed that indoles with various substitution groups at different positions (entries 2–11, Table 3) could all react with xanthen-9-ol smoothly to obtain the target compounds with excellent isolated yields, whether the substituents were electron-withdrawing or electron-donating groups. The other nucleophiles such as pyrrole, acetylacetone, malononitrile, pyridine-2-thiol, methimazole and azidotrimethylsilane (entries 12–17, Table 3) could also be easily converted to the target products under the optimized reaction conditions with excellent product yields. Owing to the potential biological activities of compounds bearing xanthen-9-yl groups,46–48 the current efficient catalytic methods for the preparation of variant xanthen-9-yl derivatives would be valuable.
Table 3 Extension of reaction substrate
The reutilization of ionic liquid
One of the most attractive features of ionic liquids in catalysis is its feasible recyclability and reusability. Therefore, the reutilization of recycled ionic liquid [Betaine][H2PO4] was also studied in this study using the reaction of xanthen-9-ol with indole as the model under the optimized reaction conditions. After the reaction was completed, the reaction mixture was washed using ethyl acetate and water. The ionic liquid dissolved in water was then rotary distillated to remove most of the water, and then sufficiently dried in a vacuum oven to obtain the recycled ionic liquid, which was then directly utilized for the next run. The results showed in Fig. 1 indicated that the catalytic activity of ionic liquid remained very high after five cycles. The further FT-IR spectra of the fresh and reutilized ionic liquid [Betaine][H2PO4] after five cycles shown in Fig. 2 suggested that there was no significant difference between the fresh and the recycled ionic liquid.
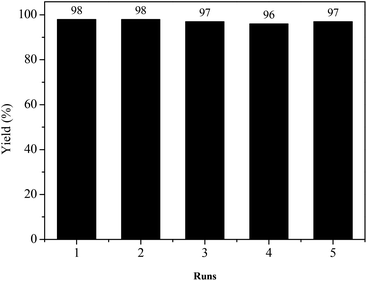 |
| Fig. 1 Recyclability of the IL catalyst. | |
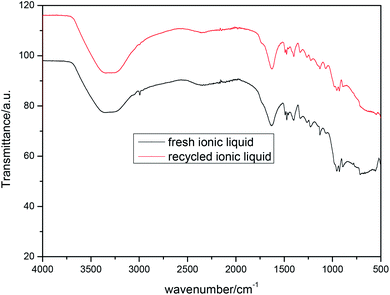 |
| Fig. 2 FT-IR spectra of the fresh and recycled ionic liquid [Betaine][H2PO4]. | |
Conclusion
In this study, we have found an inexpensive and easily prepared ionic liquid, namely [Betaine][H2PO4], which can efficiently catalyze the direct substitution reaction of xanthen-9-ol with different nucleophiles under solvent-free conditions, leading to the target products with excellent product yields. This catalytic system is easy to be operated and the following work-up procedure is very simple. The ionic liquid catalyst [Betaine][H2PO4] can be conveniently recycled and reutilized for at least five cycles without noticeable decrease in activity. The above-mentioned benefits, in addition to the inherent superiority of the direct substitution reactions of alcohol and the biocompatibility of raw materials for the preparation of the ionic liquid suggest that this reaction system is greatly sustainable and promising for the further utilization in the direct substitution of xanthen-9-ol with various nucleophiles. The finding that the presence of the carboxyl group on the Betaine cation is the main reason for the high catalytic activity of [Betaine][H2PO4] may give constructive indications for the further design of ionic liquid according to this type of reactions.
Conflicts of interest
There are no conflicts of interest to declare.
Acknowledgements
This work was supported by the National Natural Science Foundation of China (21373079 and 21733011) and S&T Research Foundation of Education Department of Henan Province (No. 20A150022).
References
- C. S. Ye, Z. Y. Qi, D. R. Cai and T. Qiu, Ind. Eng. Chem. Res., 2018, 58, 1123–1132 CrossRef.
- M. Y. Liu, Z. R. Zhang, H. Z. Liu, Z. B. Xie, Q. Q. Mei and B. X. Han, Sci. Adv., 2018, 4, 9319–9326 CrossRef PubMed.
- W. S. Miao and T. H. Chan, Acc. Chem. Res., 2006, 39, 897–908 CrossRef CAS PubMed.
- T. Hmissa, X. F. Zhang, N. R. Dhumal, G. J. McManus, X. Zhou, H. B. Nulwala and A. Mirjafari, Angew. Chem., Int. Ed., 2018, 57, 16005–16009 CrossRef CAS PubMed.
- A. K. Chakraborti and S. R. Roy, J. Am. Chem. Soc., 2009, 131, 6902–6903 CrossRef CAS PubMed.
- M. H. Li, F. T. Wu and Y. L. Gu, Chin. J. Catal., 2019, 40, 1135–1140 CrossRef CAS.
- L. Y. Xie, S. Peng, L. H. Lu, J. Hu, W. H. Bao, F. Zeng, Z. L. Tang, X. H. Xu and W. M. He, ACS Sustainable Chem. Eng., 2018, 6, 7989–7994 CrossRef CAS.
- C. Wu, L. H. Lu, A. Z. Peng, G. K. Jia, C. Peng, Z. Cao, Z. L. Tang, W. M. He and X. H. Xu, Green Chem., 2018, 20, 3683–3688 RSC.
- S. P. M. Ventura, F. A. E. Silva, M. V. Quental, D. Mondal, M. G. Freire and J. A. P. Coutinho, Chem. Rev., 2017, 117, 6984–7052 CrossRef CAS PubMed.
- F. L. Fan, Z. Qin, S. W. Cao, C. M. Tan, Q. G. Huang, D. S. Chen, J. R. Wang, X. J. Yin, C. Xu and X. G. Feng, Inorg. Chem., 2019, 58, 603–609 CrossRef CAS PubMed.
- L. L. Ni, J. Y. Xin, K. Jiang, L. Chen, D. X. Yan, X. M. Lu and S. J. Zhang, ACS Sustainable Chem. Eng., 2018, 6, 2541–2551 CrossRef CAS.
- Z. R. Zhang, J. L. Song and B. X. Han, Chem. Rev., 2017, 117, 6834–6880 CrossRef CAS PubMed.
- J. C. Varela, K. Sankar, A. Hino, X. R. Lin, W. S. Chang, D. Coker and M. Grinstaff, Chem. Commun., 2018, 54, 5590–5593 RSC.
- X. F. Sun, X. C. Kang, Q. G. Zhu, J. Ma, G. Y. Yang, Z. M. Liu and B. X. Han, Chem. Sci., 2016, 7, 2883–2887 RSC.
- S. G. Zhang, Q. H. Zhang, Y. Zhang, Z. J. Chen, M. Watanabe and Y. Q. Deng, Prog. Mater. Sci., 2016, 77, 80–124 CrossRef CAS.
- J. J. Wang, A. L. Zhu and L. J. Li, Sustainable catalysis systems based on ionic liquids in Sustainable catalytic processes, Elesvier B. V, 2015, ch. 3 Search PubMed.
- Y. Hu, J. L. Song, C. Xie, H. R. Wu, T. Jiang, G. Y. Yang and B. X. Han, ACS Sustainable Chem. Eng., 2019, 7, 5614–5619 CrossRef CAS.
- Z. Cao, Q. Zhu, Y. W. Lin and W. M. He, Chin. Chem. Lett., 2019, 30, 2132–2138 CrossRef CAS.
- A. L. Zhu, L. J. Li, C. Zhang, Y. T. Shen, M. J. Tang, L. L. Bai, C. Y. Du, S. J. Zhang and J. J. Wang, Green Chem., 2019, 21, 307–313 RSC.
- B. S. Wang, Z. J. Luo, E. H. M. Elageed, S. Wu, Y. Y. Zhang, X. P. Wu, F. Xia, G. R. Zhang and G. H. Gao, ChemCatChem, 2016, 8, 830–838 CrossRef CAS.
- S. Abello, F. Medina, X. Rodriguez, Y. Cesteros, P. Salagre, J. E. Sueiras, D. Tichit and B. Coq, Chem. Commun., 2004, 1096–1097 RSC.
- N. Fanjul-Mosteirin, C. Concellon and V. delAmo, Org. Lett., 2016, 18, 4266–4269 CrossRef CAS PubMed.
- P. Moriel, E. J. Garcia-Suarez, M. Martinez, A. B. Garcia, M. A. Montes-Moran, V. Calvino-Casilda and M. A. Banares, Tetrahedron Lett., 2010, 51, 4877–4881 CrossRef CAS.
- A. L. Zhu, R. X. Liu, L. J. Li, L. Y. Li, L. Wang and J. J. Wang, Catal. Today, 2013, 200, 17–23 CrossRef CAS.
- H. Zhao, C. Zhang and T. D. Crittle, J. Mol. Catal. B: Enzym., 2013, 85–86, 243–247 CrossRef CAS.
- J. Hoppe, R. Drozd, E. Byzia and M. Smiglak, Int. J. Biol. Macromol., 2019, 136, 296–304 CrossRef CAS PubMed.
- A. L. Zhu, M. J. Tang, Q. Z. Lv, L. J. Li, S. K. Bai, Q. Q. Li, W. L. Feng, Q. X. Li and J. J. Wang, J. CO2 Util., 2019, 34, 500–506 CrossRef CAS.
- A. L. Zhu, T. Jiang, B. X. Han, J. C. Zhang, Y. Xie and X. M. Ma, Green Chem., 2007, 9, 169–172 RSC.
- Y. X. Zhou, S. Q. Hu, X. M. Ma, S. G. Liang, T. Jiang and B. X. Han, J. Mol. Catal. A: Chem., 2008, 284, 52–57 CrossRef CAS.
- R. Kumar and E. V. Van der Eycken, Chem. Soc. Rev., 2013, 42, 1121–1146 RSC.
- L. D. Bratton, B. D. Roth, B. K. Trivedi and P. C. Unangst, J. Heterocycl. Chem., 2000, 37, 1103–1108 CrossRef CAS.
- L. Y. Liu, Y. Zhang, K. M. Huang, W. X. Chang and J. Li, Appl. Organomet. Chem., 2012, 26, 9–15 CrossRef CAS.
- S. Y. Wang and S. J. Ji, Synlett, 2007, 14, 2222–2226 Search PubMed.
- S. Y. Wang and S. J. Ji, Synth. Commun., 2008, 38, 465–472 CrossRef CAS.
- R. Ortiz, A. Koukouras, E. M.-L. pez and R. P. Herrera, Arabian J. Chem., 2020, 13, 1866–1873 CrossRef CAS.
- B. Hargittai and G. J. Barany, J. Pept. Res., 1999, 54, 468–479 CrossRef CAS PubMed.
- S. Shirakawa and S. Kobayashi, Org. Lett., 2007, 9, 311–314 CrossRef CAS PubMed.
- B. H. Zhou, J. Yang, M. H. Li and Y. L. Gu, Green Chem., 2011, 13, 2204–2211 RSC.
- P. G. Cozzi and L. Zoli, Green Chem., 2007, 9, 1292–1295 RSC.
- P. G. Cozzi and L. Zoli, Angew. Chem., Int. Ed., 2008, 47, 4162–4166 CrossRef CAS PubMed.
- L. Y. Liu, B. Wang, H. M. Yang, W. X. Chang and J. Li, Tetrahedron Lett., 2011, 52, 5636–5639 CrossRef CAS.
- A. L. Zhu, W. L. Feng, L. J. Li, Q. Q. Li and J. J. Wang, Catal. Lett., 2017, 147, 261–268 CrossRef CAS.
- A. L. Zhu, C. Y. Du, Y. Zhang and L. J. Li, J. Mol. Liq., 2019, 279, 289–293 CrossRef CAS.
- A. L. Zhu, Q. Q. Li, L. J. Li and J. J. Wang, Catal. Lett., 2013, 143, 463–468 CrossRef CAS.
- A. Gilbert, G. Bucher, R. S. Haines and J. B. Harper, Org. Biomol. Chem., 2019, 17, 9336–9342 RSC.
- C. W. Whitehead and C. A. Whitesitt, J. Med. Chem., 1974, 17, 1298–1304 CrossRef CAS PubMed.
- M. Aman and V. R. Rai, Biocontrol Sci. Technol., 2016, 26, 476–491 CrossRef.
- R. Chuprov-Netochin, Y. Neskorodov, E. Marusich, Y. Mishutkina, P. Volynchuk, S. Leonov, K. Skryabin, A. Ivashenko, K. Palme and A. Touraev, BMC Plant Biol., 2016, 16, 192–203 CrossRef PubMed.
Footnote |
† Electronic supplementary information (ESI) available. See DOI: 10.1039/d0ra05374k |
|
This journal is © The Royal Society of Chemistry 2020 |