DOI:
10.1039/D0RA05105E
(Paper)
RSC Adv., 2020,
10, 29109-29113
Water-triggered union of multi-component reactions towards the synthesis of a 4H-chromene hybrid scaffold†
Received
9th June 2020
, Accepted 13th July 2020
First published on 6th August 2020
Abstract
An unprecedented union of multi-component reactions to construct pyrazole- and pyranopyrazole-adorned 4H-chromene from simple reactants in water at ambient temperature is reported. This innovative tactic has integrated two distinct four-component reactions (4CRs) that occur transiently to form four new heterocycles via ten covalent bonds in a single step.
Synthetic organic chemists have reached a level of intricacy, allowing access to any molecule with desired functionalities and stereochemistry. Nevertheless, there is a paucity of transformations for the efficient construction of small biomolecules and their analogs. Multi-component reactions1 (MCRs) are economical tools for the achievement of this goal, as they inherently involve the formation of several covalent bonds in one operation. MCR proficiency can be further refined by unifying two or more MCRs. In recent decades, the use of higher-order MCRs to construct structurally diverse and complex skeletons has become attractive in organic synthesis and medicinal chemistry.2
The union of multi-component reactions (union of MCRs) is an elegant tactic for performing higher-order MCRs and accessing diverse and complex molecular architectures. This approach was first coined by Ugi.3 The union of MCRs is generally achieved by tandem MCRs4 or by installing suitable building blocks with orthogonal functionality.5 The focus of union of MCRs is the orthogonal reactivity of any one of the reactants, which can be combined by two different MCRs without functional-group protection. Nurturing MCRs in water6 to attain important scaffolds from simple reactants is viewed as a greener approach to populate the chemical space.7 Diversity-oriented synthesis8 (DOS), a fascinating area of research in organic synthesis with its sub-disciplines of privileged sub-structure-based diversity-oriented synthesis9 (pDOS) and biology-oriented synthesis10 (BIOS), has provided several guiding principles to access biologically relevant small molecules.11 However, unification of the guiding principles of DOS with union of MCRs in water to access biologically relevant complex scaffold remains a challenge. Very few reports on union of MCRs have focused on the eminent Ugi-4-component reaction (U-4CR) permutation with other MCRs. Indeed, to the best of our knowledge, union of MCRs without the involvement of U-4CRs has not been accomplished so far. The development of new unions of MCR is limited by the compatibility of reactant and reactions in a given solvent.4i Herein, we report an innovative strategy that combines two different known 4CRs, which displays solvent compatibilities and produces multiple functional groups in one pot to achieve higher-order MCRs, leading to 4H-chromene scaffolds.
At the outset, we established a pyranopyrazole four-component reaction (PP4CR) in water to access a multi-functionalized pyranopyrazole scaffold.12 To create skeletal diversity, we installed a single-reactant-replacement13 (SRR) strategy into our PP4CR by orthogonal functionality on the oxo component. Consequently, skeletally diverse and medicinally important 4H-chromene14 and 4H-thiochromene15 derivatives were achieved. Encouraged by these results, we planned to combine these two recent 4CRs in water. As a pDOS approach, we rationally employed 2-hydroxy-5-methylisophthalaldehyde as an oxo component into our PP4CR and performed a pseudo-eight-component reaction to unite these two distinct 4CRs to obtain a complex structure with multi-functionality in a single core possessing the pyranopyrazole and 4H-chromene skeletons. Thus, we are the first to describe pseudo-eight-component reactions (8CRs) in water, with unification of two disparate 4CRs to access an unprecedented bioactive pyranopyrazole-substituted 4H-chromene scaffold (Scheme 1).
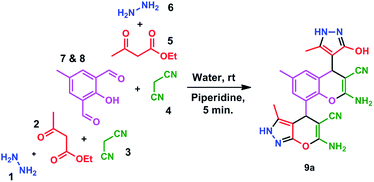 |
| Scheme 1 Pseudo-eight-component reaction in water. | |
The pseudo-8CR performed in water using two equivalents of hydrazine hydrate 96% (1), ethyl acetoacetate (2), malononitrile (3), and one equivalent of 2-hydroxy-5-methylisophthalaldehyde (7 and 8) in the presence of piperidine 10% base at ambient temperature resulted in the formation of product 9a within 5 min. The product was identified by nuclear magnetic resonance (NMR) as a complex heterocyclic hybrid architecture of 4H-chromene adorned with a pyrazole and pyranopyrazole substructure. To verify that this union of MCRs occurred either in tandem or in a one-pot manner, we performed an MCR using equimolar hydrazine hydrate 96% (1), ethyl acetoacetate (2), malononitrile (3), and 2-hydroxy-5-methylisophthalaldehyde (7 and 8) in water. The reaction yielded 9a only, which was confirmed by 1H NMR. The result suggests that both 4CRs might occur simultaneously. Thus, the reaction could not be controlled to obtain either a 4H-chromene or pyranopyrazole scaffold of a single 4CR while retaining the aldehyde functionality, which can be exploited in other MCRs. This reaction represents an unprecedented example of the true union of MCRs. The reaction occurred according to the mechanism shown in Scheme 2.
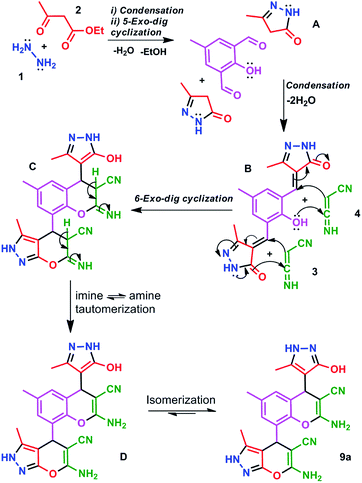 |
| Scheme 2 Plausible mechanism. | |
Initially, component 1 and 2 are involved in the condensation followed by 5-exo-dig azacyclization to yield 3-methyl pyrazolin-5-one (A) instantly. A undergoes a Knoevenagel condensation reaction with 2-hydroxy-5-methylisopthalaldehyde to form the intermediate B. The malononitrile (3) exists in nitrile–ketenimine tauomerism16 in water medium. The intermediate B is involved 6-exo-dig-oxocyclization with component 3. Cumulatively, four heterocycles are formed by 10 covalent bonds, such as C–C, C–N, and C–O. At the final stage, isomerization occurs to obtain the 1H-isomer of the pyrazole ring. A deshielding for
and consequent shielding for
of pyranopyrazole were observed in comparison with the corresponding H4 and C4 chemical shifts of chromene, indicating the proximity of
to the oxygen of the chromene moiety. The chromene derivatives are adorned with diverse carbo- and heterocyclic motifs, which are ubiquitous in natural products as well as synthetic bioactive molecules, and exhibit vital biological and pharmacological activities.17
The reaction showed similar selectivity with good-to-excellent yield when different 2-hydroxyisophthalaldehydes were used as oxo building blocks. The products were created in the course of reactions having two chiral centers. Only two diastereomers of the four possible stereoisomers were distinguishable in 1H and 13C NMR, and were inseparable by column chromatography. The diastereomer ratio was calculated from 1H NMR (Fig. 1).
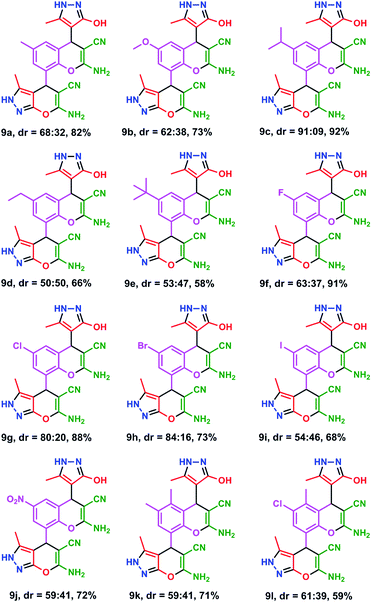 |
| Fig. 1 Library of pyrazole- and pyranopyrazole-adorned 4H-chromene scaffolds. | |
We subsequently investigated the use of unsymmetrical disubstituted isopthalaldehydes as an oxo component to this pseudo-8CR. The reaction occurred regioselectively, and the possible regio isomers of 9l are shown in Fig. 2.
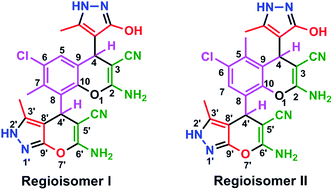 |
| Fig. 2 Possible regio isomers of pyranopyrazole-adorned 4H-chromen (9l). | |
The regio-isomer of 9l was characterized by heteronuclear multiple bond correlation spectroscopy (HMBC) (Fig. 3). In the 1H NMR of 9l, the signals at (4.59 + 4.58) and (5.19 + 5.18) ppm were assignable C4 and
protons, respectively. These proton signals were considered as starting points for analyzing the HMBC correlation spectrum to identify the isomer formed in this reaction.
 |
| Fig. 3 HMBC correlations of compound 9l. | |
In HMBC, the
proton correlates with only two carbon
signals at (97.06 + 96.85) and 55.24 ppm. The C4 proton correlates to four carbon signals at the chromene moiety and three carbon signals at the pyrazole ring C3 (56.15 + 55.15), C5 (132.59 + 132.44), C9 123.57, C10 159.22,
(135.59 + 135.53),
(103.87 + 103.59), and
145.46 ppm, respectively. The signals at (2.11 + 2.07) ppm, which are assignable to methyl protons attached to aromatic ring, correlate with three carbon signals assignable to the ipso carbon C5 (132.59 + 132.44), two ortho carbons C6 (128.66), and C9 (123.57 ppm) of the aromatic ring. The common correlations for C4 and aromatic methyl protons confirm that the methyl group is adjacent to C4. The structure of the regio-isomer II was assigned unambiguously by HMBC experiments. On cross-matching, the C4 proton correlates with the aromatic methyl protons correlations two carbon signals at (132.59 + 132.44) and 123.57 ppm are common in both correlations.
Gratifyingly, our union of MCRs is an ideal platform for the rapid construction of privileged scaffolds, introducing both diversity and complexity in a single-step manner with orthogonal functionality.
Conclusions
We have developed a true union of MCRs from simple reactants in water via a single step at ambient temperature. This elegant strategy involves the spontaneous formation of ten covalent bonds to access complex and diverse drug-like molecules. The products of union of MCRs also bear orthogonal functionality that may be selectively exploited to create novel compounds having been identified in leads and drugs. Further studies of synthesized scaffolds are in progress in our laboratory.
Experimental
To a stirred aqueous (25 mL) mixture of hydrazine hydrate 96% 1 (107 mg, 2 mmol), ethyl acetoacetate 2 (260 mg, 2 mmol), and malononitrile 3 (132 mg, 2 mmol), 2-hydroxyisophthalaldehyde 4 (1 mmol) and 5 mol% of piperidine catalyst were added successively at room temperature under an open atmosphere with vigorous stirring for 5–10 min. The solid produced from the reaction mixture was filtered, and washed with water and then with ethyl acetate and/or cold ethanol. The products obtained were pure on thin layer chromatography (TLC) and NMR spectra.
Conflicts of interest
There are no conflicts to declare.
Acknowledgements
G. V. and K. K thank CSIR (01(2500)/11/EMR-II) and DST (SR/S5/GC-22/2007), the Government of India, for financial support, the Central Instrumentation Facility (CIF), Pondicherry University, for the high-resolution NMR spectroscopy measurements, and the Department of Chemistry (DST-FIST), Pondicherry University, for the FT-IR spectroscopy measurements.
Notes and references
-
(a) R. W. Armstrong, A. P. Combs, P. A. Tempest, S. D. Brown and T. A. Keating, Multiple-Component Condensation Strategies for Combinatorial Library Synthesis, Acc. Chem. Res., 1996, 29, 123–132 CrossRef CAS;
(b) M. A. Mironov, Design of Multi-Component Reactions: From Libraries of Compounds to Libraries of Reactions, QSAR Comb. Sci., 2006, 25, 423–431 CrossRef CAS;
(c) W. Zhu, M. Mena, N. Sun, E. Jnoff, P. Pasau and L. Ghosez, Multicomponent Reactions for the Synthesis of Complex Piperidine Scaffolds, Angew. Chem., Int. Ed., 2009, 48, 1–5 CrossRef;
(d) G. R. Boyce and J. S. Johnson, Three-Component Coupling Reactions of Silyl Glyoxylates, Vinyl Grignard Reagent, and Nitroalkenes: An Efficient, Highly Diastereoselective Approach to Nitrocyclopentanols, Angew. Chem., Int. Ed., 2010, 49, 1–5 CrossRef;
(e) Multicomponent Reactions: Concepts and Applications for design and Synthesis, ed. R. P. Herrera and E. Marqués López, John Wiley & Sons, Inc., 2015 Search PubMed;
(f) T. Zarganes-Tzitzikas, A. L. Chandgude and A. Dömling, Multicomponent Reactions, Union of MCRs and Beyond, Chem. Rec., 2015, 15, 981–996 CrossRef CAS.
-
(a) M. E. Welsch, S. A. Snyder and B. R. Stockwell, Privileged Scaffolds for Library Design and Drug Discovery, Curr. Opin. Chem. Biol., 2010, 14, 347–361 CrossRef CAS;
(b) I. Monfardini, J. W. Huang, B. Beck, J. F. Cellitti, M. Pellecchia and A. Dömling, Screening Multicomponent Reactions for X-Linked Inhibitor of Apoptosis-Baculoviral Inhibitor of Apoptosis Protein Repeats Domain Binder, J. Med. Chem., 2011, 54, 890–900 CrossRef CAS;
(c) D. M. Beal and L. H. Jones, Molecular Scaffolds Using Multiple Orthogonal Conjugations: Applications in Chemical Biology and Drug Discovery, Angew. Chem., Int. Ed., 2012, 51, 6320–6326 CrossRef CAS.
-
(a) A. Dömling and I. Ugi, The Seven-Component Reaction, Angew. Chem., Int. Ed. Engl., 1993, 32, 563–564 CrossRef;
(b) A. Dömling, E. Herdtweck and I. Ugi, MCR V:1 Seven-Component Recation, Acta Chem. Scand., 1998, 52, 107–113 CrossRef.
-
(a) I. Ugi, A. Demharter, W. Hörl and T. Schmid, Ugi Reactions with trifunctionai α-Amino Acids, Aldehydes, Isocyanides and Alcohols, Tetrahedron, 1996, 52, 11657–11664 CrossRef CAS;
(b) F. Constabel and I. Ugi, Repetitive Ugi Reactions, Tetrahedron, 2001, 57, 5785–5789 CrossRef CAS;
(c) I. K. Ugi, B. Ebert and W. Hörl, Formation of 1,10-iminodicarboxylic acid derivatives, 2,6-diketo-piperazine and dibenzodiazocine-2,6-dione by variations of multicomponent reactions, Chemosphere, 2001, 43, 75–81 CrossRef CAS;
(d) D. E. Portlock, R. Ostaszewski, D. Naskar and L. West, A tandem Petasis–Ugi multi component condensation reaction: solution phase synthesis of six dimensional libraries, Tetrahedron Lett., 2003, 59, 603–605 CrossRef;
(e) D. E. Portlock, D. Naskar, L. West, R. Ostaszewski and J. J. Chen, Solid-phase synthesis of five-dimensional libraries via a tandem Petasis–Ugi multi-component condensation reaction, Tetrahedron Lett., 2003, 44, 5121–5124 CrossRef CAS;
(f) D. Naskar, A. Roy, W. L. Seibel, L. West and D. E. Portlock, The synthesis of aza-β-lactams via tandem Petasis–Ugi multi-component condensation and 1,3-diisopropylcarbodiimide (DIC) condensation reaction, Tetrahedron Lett., 2003, 44, 6297–6300 CrossRef CAS;
(g) M. Paravidino, R. Scheffelaar, R. F. Schmitz, F. J. J. de Kanter, M. B. Groen, E. Ruijter and R. V. A. Orru, A Flexible Six-Component Reaction To Access Constrained Depsipeptides Based on a Dihydropyridinone Core, J. Org. Chem., 2007, 72, 10239–10242 CrossRef CAS;
(h) N. Elders, D. van der Born, L. J. D. Hendrickx, B. J. J. Timmer, A. Krause, E. Janssen, F. J. J. de Kanter, E. Ruijter and R. V. A. Orru, The Efficient One-Pot Reaction of up to Eight Components by the Union of Multicomponent Reactions, Angew. Chem., Int. Ed., 2009, 48, 5856–5859 CrossRef CAS;
(i) T. H. Al-Tel, R. A. Al-Qawasmeh and W. Voelter, Rapid Assembly of Polyfunctional Structures Using a One-Pot Five- and Six-Component Sequential Groebke–Blackburn/Ugi/Passerini Process, Eur. J. Org. Chem., 2010, 5586–5593 CrossRef CAS;
(j) T. Niu, L. Gu, W. Yi and C. Cai, Copper-free Route to Triazole-Modified Peptidomimetic by the Combination of Two Multicomponent Reactions in One Pot, ACS Comb. Sci., 2012, 14, 309–315 CrossRef CAS.
-
(a) H. Bienaymé, Reagent Explosion: an Efficient Method to Increase Library Size and Diversity, Tetrahedron Lett., 1998, 39, 4255–4258 CrossRef;
(b) A. Dömling, The discovery of new isocyanide-based multi-component reactions, Curr. Opin. Chem. Biol., 2000, 4, 318–323 CrossRef;
(c) S. Brauch, L. Gabriel and B. Westermann, Seven-component reactions by sequential chemoselective Ugi–Mumm/Ugi–Smiles reactions, Chem. Commun., 2010, 46, 3387–3389 RSC.
-
(a) C. J. Li and L. Chen, Organic chemistry in water, Chem. Soc. Rev., 2006, 35, 68–82 RSC;
(b) K. Kumaravel and G. Vasuki, Multi-Component Reactions in Water, Curr. Org. Chem., 2009, 13, 1820–1841 CrossRef CAS.
-
(a) H. Waldmann, et. al., Bioactivity-guided mapping and navigation of chemical space, Nat. Chem. Biol., 2009, 5, 585–592 CrossRef;
(b) R. S. Bon and H. Waldmann, Bioactivity-Guided Navigation of Chemical Space, Acc. Chem. Res., 2010, 43, 1103–1114 CrossRef CAS;
(c) M. E. Welsch, S. A. Snyder and B. R. Stockwell, Privileged scaffolds for library design and drug discovery, Curr. Opin. Chem. Biol., 2010, 14, 347–361 CrossRef CAS;
(d) H. Lachance, S. Wetzel, K. Kumar and H. Waldmann, Charting, Navigating, and Populating Natural Product Chemical Space for Drug Discovery, J. Med. Chem., 2012, 55, 5989–6001 CrossRef CAS.
-
(a) M. D. Burke and S. L. Schreiber, A Planning Strategy for Diversity-Oriented Synthesis, Angew. Chem., Int. Ed., 2004, 43, 46–58 CrossRef;
(b) R. J. Spandl, A. Bender and D. R. Spring, Diversity-oriented synthesis; a spectrum of approaches and results, Org. Biomol. Chem., 2008, 6, 1149–1158 RSC;
(c) S. L. Schreiber, Organic chemistry: Molecular diversity by design, Nature, 2009, 457, 153–154 CrossRef CAS;
(d) E. Ruijter, R. Scheffelaar and R. V. A. Orru, Multicomponent reaction design in the quest for molecular complexity and diversity, Angew. Chem., Int. Ed., 2011, 50, 6234–6246 CrossRef CAS;
(e) W. R. J. D. Galloway and D. R. Spring, Better leads come from diversity, Nature, 2011, 470, 43 Search PubMed.
- S. Oh and S. B. Park, A design strategy for drug-like polyheterocycles with privileged substructures for discovery of specific small-molecule modulators, Chem. Commun., 2011, 47, 12754–12761 RSC.
-
(a) M. Koch, A. Schuffenhauer, M. Scheck, S. Wetzel, M. Casaulta, A. Odermatt, P. Ertl and H. Waldmann, Charting biologically relevant chemical space: A structural classification of natural products (SCONP), Proc. Natl. Acad. Sci. U.S.A., 2005, 102, 17272–17277 CrossRef CAS;
(b) R. S. Bon and H. Waldmann, Bioactivity-Guided Navigation of Chemical Space, Acc. Chem. Res., 2010, 43, 1103–1114 CrossRef CAS;
(c) S. Wetzel, R. S. Bon, K. Kumar and H. Waldmann, Biology-Oriented Synthesis, Angew. Chem., Int. Ed., 2011, 50, 10800–10826 CrossRef CAS.
-
(a) B. R. Stockwell and S. L. Schreiber, Probing the role of homomeric and heteromeric receptor interactions in TGF-β signaling using small molecule dimerizers, Curr. Biol., 1998, 8, 761–773 CrossRef CAS;
(b) S. L. Schreiber, Target-Oriented and Diversity-Oriented Organic Synthesis in Drug Discovery, Science, 2000, 287, 1964–1969 CrossRef CAS;
(c) B. R. Stockwell, Exploring biology withsmall organic molecules, Nature, 2004, 432, 846–856 CrossRef CAS;
(d) S. L. Schreiber, et. al., Towards patient-based cancer therapeutics, Nat. Biotechnol., 2010, 28, 904–906 CrossRef CAS;
(e) S. Murrison, S. K. Maurya, C. Einzinger, B. McKeever-Abbas, S. Warriner and A. Nelson, Synthesis of Skeletally Diverse Alkaloid-Like Small Molecules, Eur. J. Org. Chem., 2011, 12, 2354–2359 CrossRef;
(f) P. J. Hajduk, Small molecules, great potential, Nature, 2011, 470, 42 CrossRef CAS;
(g) S. L. Schreiber, et. al., An Interactive Resource to Identify Cancer Genetic and Lineage Dependencies Targeted by Small Molecules, Cell, 2013, 154, 1151–1161 CrossRef.
- G. Vasuki and K. Kumaravel, Rapid four-component reactions in water: synthesis of pyranopyrazoles, Tetrahedron Lett., 2008, 49, 5636–5638 CrossRef CAS.
- B. Ganem, Strategies for Innovation in Multicomponent Reaction Design, Acc. Chem. Res., 2009, 42, 463–472 CrossRef CAS.
- K. Kumaravel and G. Vasuki, Four-component catalyst-free reaction in water: Combinatorial library synthesis of novel 2-amino-4-(5-hydroxy-3-methyl-1H-pyrazol-4-yl)-4Hchromene- 3-carbonitrile derivatives, Green Chem., 2009, 11, 1945–1947 RSC.
- K. Kumaravel and G. Vasuki, Unpublised result.
-
(a) T. R. Kasturi, B. N. Mylari, A. Balasubramanyam and C. N. R. Rao, Spectroscopic studies of keto-enol equilibria, Can. J. Chem., 1962, 40, 2272–2277 CrossRef CAS;
(b) T. R. Kasturi, V. K. Sharma, A. Srinivasan and G. Subrahmanyam, Nitrile-ketenimine tautomerism in substituted alkylidene malononitriles and alkylidene cyanoacetates, Tetrahedron, 1973, 29, 4103–4109 CrossRef CAS.
-
(a) J.-L. Wang, D. Liu, Z.-J. Zhang, S. Shan, X. Han, S. M. Srinivasula, C. M. Croce, E. S. Alnemri and Z. Huang, Structure-based discovery of an organic compound that binds Bcl-2 protein and induces apoptosis of tumor cells, Proc. Natl. Acad. Sci. U.S.A., 2000, 97, 7124–7129 CrossRef CAS;
(b) M. J. Gorczynski, R. M. Leal, S. L. Mooberry, J. H. Bushweller and M. L. Brown, Synthesis and evaluation of substituted 4-aryloxy- and 4-arylsulfanyl-phenyl-2-aminothiazoles as inhibitors of human breast cancer cell proliferation, Bioorg. Med. Chem., 2004, 12, 1029–1036 CrossRef CAS;
(c) M. Vosooghi, S. Rajabalian, M. Sorkhi, M. Badinloo, M. Nakhjiri and A. S. Negahbani, et. al., Synthesis and cytotoxic activity of some 2-amino-4-aryl-3-cyano-7-(dimethylamino)-4H-chromenes, Res. Pharm. Sci., 2010, 5, 9–14 CAS;
(d) A. M. Shestopalov, Y. M. Litvinov, L. A. Rodinovskaya, O. R. Malyshev, M. N. Semenova and V. V. Semenov, Polyalkoxy Substituted 4H-Chromenes: Synthesis by Domino Reaction and Anticancer Activity, ACS Comb. Sci., 2012, 14, 484–490 CrossRef CAS.
Footnote |
† Electronic supplementary information (ESI) available. See DOI: 10.1039/d0ra05105e |
|
This journal is © The Royal Society of Chemistry 2020 |
Click here to see how this site uses Cookies. View our privacy policy here.