DOI:
10.1039/D0RA03323E
(Paper)
RSC Adv., 2020,
10, 22230-22233
Atom-efficient synthesis of 2,4,6-trisubstituted 1,3,5-triazines via Fe-catalyzed cyclization of aldehydes with NH4I as the sole nitrogen source†
Received
14th April 2020
, Accepted 1st June 2020
First published on 9th June 2020
Abstract
An atom-efficient, straightforward method for the synthesis of 2,4,6-triaryl-1,3,5-triazines via iron-catalyzed cyclization of aldehydes with NH4I as the sole nitrogen source is demonstrated. This strategy works smoothly under air atmosphere, and affords symmetrical 2,4,6-trisubstituted and unsymmetrical 1,3,5-triazines with yields from 18% to 72%. Compared to other methods, the present protocol provides a straightforward and atom-efficient approach to 2,4,6-trisubstituted 1,3,5-triazines using an inexpensive, easily available ammonium salt as the sole nitrogen source. Research into the preliminary mechanism indicates that N-benzylidenebenzimidamides are involved in this cyclization reaction.
Introduction
The derivatives of 1,3,5-triazine are well-known compounds of considerable interest because of their applications in many different fields.1 Actually, these compounds serve as pharmaceuticals,2 liquid crystals,3 transition-metal catalysts,4 building blocks for supramolecular chemistry,5 reactive dyes6, organic light-emitting diodes (OLEDs),7 and chemical reagents for selected transformations.8 Although the substituted 1,3,5-triazines have extensive applications, the method of synthesis of these compounds is still very limited. Traditional methods of substituted 1,3,5-triazines preparation involved cyclotrimerization of nitriles,9 or cyclization of imidates10 and amidine derivatives,11 in which nitriles, imidates and amidine12 served as nitrogen sources. For example, Forsberg and co-workers found that lanthanum and yttrium trifluoromethanesulfonates could catalyze a reaction between ammonia salts and aromatic nitriles to yield symmetrically 2,4,6- substituted 1,3,5-triazine13 (Scheme 1a). However, ammonia salts were used as cocatalysts rather than a nitrogen source. Condensation of aromatic aldehydes with amidines was found to produce symmetrically and unsymmetrically 2,4,6- substituted 1,3,5-triazine14 (Scheme 1b), in which, aromatic amidines were the nitrogen source. Recently, the coupling of halogenated 1,3,5-triazines and aryl boronic acids based on Suzuki-coupling reactions has been explored.15 Oxidative coupling reaction of amidine hydrochlorides and alcohol has been developed to synthesize 1,3,5-triazine derivatives.16 In this procedure, amidine was the nitrogen sources. Therefore, it is remarkable significance to find a cheap, readily available nitrogen source for 1,3,5-triazine synthesis.
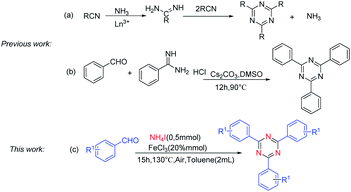 |
| Scheme 1 Methods for the synthesis of 1,3,5-triazine derivatives. | |
Ammonium iodide (NH4I) as nitrogen source was widely used to synthesis nitrogen-containing heterocycles and nitrile. For instance, Deng and co-works reported NH4I as a nitrogen source to construct pyrimidines and pyridines.17 Guo and co-works reported cyanation of ketones using ammonium salts as the nitrogen source in the presence of TBAI.18 In continuation with our ongoing work on cyclization approaches in organic synthesis,19 herein, we demonstrate that NH4I can be used as the sole nitrogen source for the cyclotrimerization of aldehydes (Scheme 1c).
Results and discussion
Initially, we investigated the reaction between benzaldehyde (1a) and NH4I under the condition of air and chlorobenzene as the solvent. It was found that chlorobenzene is a good solvent for high yield synthesis of nitrogen-containing heterocyclic compounds using NH4I as the nitrogen source.20.Therefore, we chose chlorobenzene as the initial solvent, and obtained 62% yield of 2a with adding 0.2 equiv. of FeCl3 (Table 1, entries 1). Moderate yields of 2a were observed in the presence of other catalysts, such as CuCl2, CoCl2 and CuO (Table 1, entries 2–4). This result encouraged us to choose FeCl3 as the catalyst. Next, reaction temperature was scanned to improve the yield, 130 °C was determined as optimum for the cyclotrimerization of aldehydes (Table 1, entries 6). Finally, the screening of solvents was carried out, toluene was found to be the most suitable medium for this cyclotrimerization reaction compared with chlorobenzene, DMF, and DMSO (Table 1, entries 6, 8–10). After screening on different parameters, the highest yield of 2a (72%) was achieved2a when the reaction was carried out with benzaldehyde (0.5 mmol), NH4I (0.5 mmol), FeCl3 (20% mmol) at 130 °C under the atmosphere of air in toluene (entry 8; Table 1).
Table 1 Optimization of reaction conditionsa
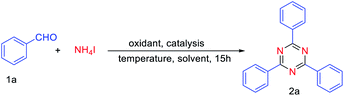
|
Entry |
Catalysis |
Temperature [°C] |
Solvent |
Yieldb |
Reaction conditions: benzaldehyde 1a (0.5 mmol), NH4I (0.5 mmol), catalysis (20% mmol), solvent (2.0 mL). Yield calculated by GC-MS. DMF = dimethylformamide. 24 h. |
1 |
FeCl3 |
150 |
Chlorobenzene |
62% |
2 |
CuCl2 |
150 |
Chlorobenzene |
53% |
3 |
CoCl2 |
150 |
Chlorobenzene |
49% |
4 |
CuO |
150 |
Chlorobenzene |
32% |
5 |
FeCl3 |
140 |
Chlorobenzene |
61% |
6 |
FeCl3 |
130 |
Chlorobenzene |
60% |
7 |
FeCl3 |
120 |
Chlorobenzene |
32%d |
8 |
FeCl3 |
130 |
Toluene |
72% |
9 |
FeCl3 |
130 |
DMSO |
— |
10 |
FeCl3 |
130 |
DMFc |
— |
With the optimized reaction conditions in hand, the substrate scope of aldehyde was firstly evaluated for the synthesis of various symmetrical 2,4,6-trisubstituted 1,3,5-triazines (Table 2). A series of electron-donating or electron-withdrawing group-substituted benzaldehyde could be employed in this reaction (Table 2, 2b–2q), affording the desire 2,4,6-trisubstituted 1,3,5-triazines in 41–72% yields. Among them, the yield of aldehyde bearing electron-donating group such as –Me, –OMe (Table 2, 2b, 2c, 2m, 2n) has been found no significant difference compared with that of aldehyde bearing electron-withdrawing ones such as –F, –Cl,–Br (Table 2, 2j, 2k, 2l). Both of them provided the desired products in good yields. Moreover, the steric effect of substituted benzaldehydes was also explored. Reaction of para and meta-subsitituted benzaldehyde (Table 2, 2b, 2c, 2k) with NH4I gave the corresponding products in higher yields compared with ortho-substituted ones (Table 2, 2d, 2h). Unfortunately, when disubstituted benzaldehyde and trisubstituted benzaldehyde were used as substrates, the lower yield of corresponding products was obtained (Table 2, 2o, 2r). When heteroaryl carbaldehydes were used as substrates (Table 2, 2e, 2f), the desired products also produced in 64% and 65% yields.
Table 2 Scope of various aldehyde and NH4Ia
Reaction conditions: aldehyde (0.5 mmol), NH4I 2 (0.5 mmol), FeCl3 (20% mmol), toluene (2.0 mL) were heated at 130 °C for 15 h. |
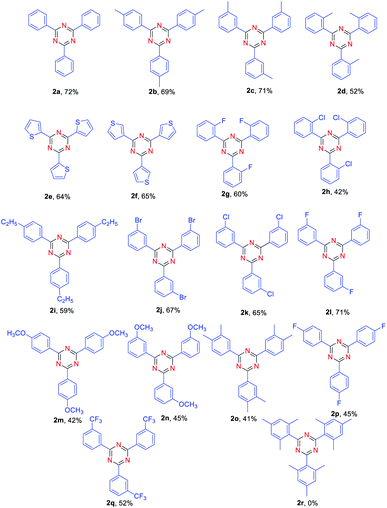 |
Synthesis of unsymmetrical 2,4,6-trisubstituted 1,3,5- triazines was also explored. To our delight, the reaction proceeded very well for benzaldehyde and 4-methylbenzaldehyde as substrates, providing the corresponding products in moderate yields (Table 3, 3h, 4h). Moreover, the reactions of benzaldehyde and thiophene-2-carbaldehyde or thiophene-3-carbaldehyde were also found to be effective (Table 3, 3b, 4b, 4c). However, the reaction of 3-methylbenzaldehyde and thiophene-2-carbaldehyde gave a relatively low yield compared with benzaldehyde and thiophene-2-carbaldehyde as substances (Table 3, 3f). Notably, when ortho-substituted aldehydes were used to react with another aldehyde, no desired product could be obtained. It is confirmed that the steric effect is critical for the reaction.
Table 3 Scope of different aldehyde and NH4Ia
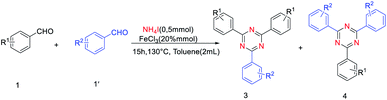
|
Reaction conditions: aldehyde 1 (0.3 mmol), aldehyde 1, (0.2 mmol), NH4I (0.5 mmol), FeCl3 (20% mmol), toluene (2.0 mL) were heated at 130 °C for 15 h. |
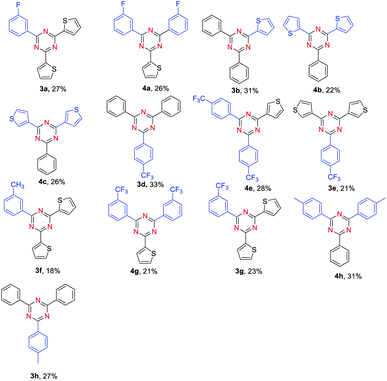 |
In order to gain insight into the reaction mechanism, a couple of control experiments were performed (Scheme 2). Firstly, when a variety of ammonium salts other than NH4I were heated in toluene at 130 °C for 15 h, no product was observed (Scheme 2a). With NH4I as nitrogen source, the desired product was similarly not observed without addition of FeCl3 (Scheme 2b). Reaction of benzaldehyde and other ammonium salts also offered corresponding products with addition of KI or NaI (Scheme 2c). Next, the model reaction was detected by GC-Ms for 1 h up to 10 h. The N-benzyl-1-phenylmethanimine was the main product (40%) at 4 h (Scheme 2d). Afterward, we used N-benzyl-1-phenylmethanimine as substance to determine whether it participated in the reaction under standard conditions. It was found that N-benzyl-1-phenylmethanimine still existed after reaction (Scheme 2e). We then used N-benzyl-1-phenylmethanimine and benzaldehyde as substances (Scheme 2f), the desired product was obtained with a yield of 67%. This yield is similar with that of the reaction with only aldehyde as substance. To our surprising, a large amount of N-benzyl-1-phenylmethanimine maintained after detection of GC-MS (ESI Fig. 1†). Therefore, N-benzyl-1-phenylmethanimine may be not an intermediate of the reaction.
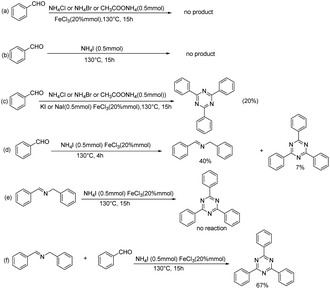 |
| Scheme 2 Control experiments. | |
Based on control experiments and literature results,21,22 a plausible mechanism is depicted in Scheme 3. Firstly, aldehydes and NH4I react to form imines A. Meanwhile, Fe3+ oxidizes I− to form I2, which then oxidizes the imine intermediate to obtain N-iodo aldimine intermediate B.21 The condensation reaction of imines A and intermediate B affords an imine intermediate C. Subsequently, under the oxidation of oxygen, the cyclization reaction of imines A and intermediate C yields intermediate E by two steps. Finally, the intermediate E undergoes an oxidation reaction to offer the desired product 1,3,5-triazine (2).
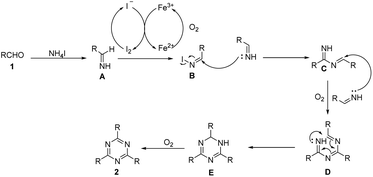 |
| Scheme 3 Proposed reaction mechanism. | |
Conclusions
In summary, we have developed a Fe-catalyzed cyclization of aldehydes with NH4I for the synthesis of symmetrical and unsymmetrical 2,4,6-trisubstituted 1,3,5-triazines in moderate to good yields. This method has relatively broad substrate scope. Importantly, NH4I was employed as sole nitrogen resource. This protocol provides a simple and atom-efficient route to synthesize valuable 1,3,5-triazines.
Conflicts of interest
There are no conflicts to declare.
Acknowledgements
This work was financially supported by the National Natural Science Foundation of China (21372068, 21572049) and Science and Technology Program of Changsha, China (kq1907114).
Notes and references
-
(a) M. Saleh, S. Abbott, V. Perron, C. Lauzon, C. Penney and B. Zacharie, Bioorg. Med. Chem. Lett., 2010, 20, 945–949 CrossRef CAS PubMed;
(b) M. Amir and K. Shikha, Eur. J. Med. Chem., 2004, 39, 535–545 CrossRef CAS PubMed;
(c) M. N. Kopylovich and A. J. Pombeiro, Coord. Chem. Rev., 2011, 255, 339–355 CrossRef CAS;
(d) G. Giacomelli, A. Porcheddu and L. D. Luca, Curr. Org. Chem., 2004, 8, 1497–1519 CrossRef CAS.
-
(a) T. Misawa, M. T. Salim and M. Okamoto, Heterocycles, 2010, 81, 1419–1426 CrossRef CAS;
(b) M. Saleh, S. Abbott, V. Perron, C. Lauzon, C. Penney and B. Zacharie, Bioorg. Med. Chem. Lett., 2010, 20, 945–949 CrossRef CAS PubMed.
- P. Zassowski, P. Ledwon, A. Kurowska, A. P. Herman, G. Sych, M. Lapkowski, V. Cherpak, Z. Hotra, P. Turyk, K. Ivaniuk, P. Stakhira, G. Sych, D. Volyniuk and J. Grazulevicius, Dyes Pigm., 2018, 149, 804–811 CrossRef CAS.
-
(a) D. A. Safin, A. Pialat, I. Korobkov and M. Murugesu, Chem.–Eur. J., 2015, 21, 6144–6149 CrossRef CAS PubMed;
(b) M. M. Najafpour, M. Hołyńska, A. N. Shamkhali, M. Amini, S. H. Kazemi and T. Lis, Polyhedron, 2012, 34, 202–209 CrossRef CAS;
(c) P. K. Santra and P. Sagar, J. Mol. Catal. A: Chem., 2003, 197, 37 CrossRef CAS.
-
(a) Y. Zhu, J. Yuan, Y. Li, M. Gao and A. Wu, Synlett, 2011, 2011, 52–56 CrossRef;
(b) X. Zhu, C. Tian, S. M. Mahurin, S. H. Chai and S. Dai, J. Am. Chem. Soc., 2012, 134, 10478–10484 CrossRef CAS PubMed;
(c) S. Naik, M. Kumaravel, J. T. Mague and M. S. Balakrishna, Inorg. Chem., 2014, 53, 1370–1381 CrossRef CAS PubMed.
- A. Herrera, A. Riano and R. Martínez-Alvarez, J. Org. Chem., 2014, 79, 7012–7024 CrossRef CAS PubMed.
- S. J. Su, H. Sasabe and J. Kido, Adv. Mater., 2010, 22, 3311–3316 CrossRef CAS PubMed.
- A. Khalafi-Nezhad, A. Zare and G. R. Nejabat, Phosphorus, Sulfur, Silicon Relat. Elem., 2007, 182, 657–666 CrossRef CAS.
-
(a) G. Thiele, B. Wagner and S. Dehnen, Eur. J. Inorg. Chem., 2015, 5329–5334 CrossRef CAS;
(b) D. R. Armstrong, K. W. Henderson, M. MacGregor, R. E. Mulvey, M. J. Ross, W. Clegg and P. A. O'Neil, J. Organomet. Chem., 1995, 486, 79–93 CrossRef CAS;
(c) Y. Ma, S. Breslin, I. Keresztes, E. Lobkovsky and D. B. Collum, J. Org. Chem., 2008, 73, 9610–9618 CrossRef CAS PubMed;
(d) N. Yu, C. Wang, F. Zhao, L. Tao, W. Zhang and Z. Xi, Chem.–Eur. J., 2008, 14, 5670–5679 CrossRef CAS PubMed.
-
(a) A. R. Tiwari, S. R. Nath, K. A. Joshi and B. M. Bhanage, J. Org. Chem., 2017, 82, 13239–13249 CrossRef CAS PubMed;
(b) A. R. Tiwari and B. M. Bhanage, ChemistrySelect, 2016, 3, 343–346 CrossRef;
(c) G. Shi, F. He, Y. Che, C. Ni and Y. Li, Russ. J. Gen. Chem., 2016, 86, 380–386 CrossRef CAS.
-
(a) P. Debnath and K. C. Majumdar, Tetrahedron Lett., 2014, 55, 6976–6978 CrossRef CAS;
(b) X. Meng, X. Bi, Y. Wang, G. Chem, B. Chem, Z. Jing and P. Zhao, Catal. Commun., 2017, 89, 34–39 CrossRef CAS;
(c) Y. Yan, Z. Li, C. Cui, H. Li, M. Shi and Y. Liu, Org. Biomol. Chem., 2018, 16, 2629 RSC;
(d) H. Huang, W. Guo, W. Wu, C. Li and H. Jiang, Org. Lett., 2015, 17, 2894–2897 CrossRef CAS PubMed;
(e) Y. Yan, C. Cui, J. Wang, S. Li and Y. Liu, Adv. Synth. Catal., 2019, 361, 1166–1170 CrossRef CAS.
- J. Shie and J. Fang, J. Org. Chem., 2007, 72, 3141–3144 CrossRef CAS PubMed.
- J. Forsberg, V. Spaziano, T. Balasubramanian, S. Kinsley, C. Duckworth, J. Poteruca, P. Brown and J. Miller, J. Org. Chem., 1987, 52, 1017–1021 CrossRef CAS.
- S. Biswas and S. Batra, Eur. J. Org. Chem., 2012, 3492–3499 CrossRef CAS.
-
(a) F. Feizpour, M. Jafarpour and A. Rezaeifard, Catal. Lett., 2019, 149, 1595–1610 CrossRef CAS;
(b) M. Esmaeilpour and J. Javidi, J. Chin. Chem. Soc., 2015, 62, 614–626 CrossRef CAS;
(c) M. Niakan, Z. Asadi and M. Masteri-Farahani, ChemistrySelect, 2019, 4, 1766–1775 CrossRef CAS;
(d) A. Isfahani, I. Mohammadpoor-Baltork, V. Mirkhani, A. R. Khosropour, M. Moghadam, S. Tangestaninejad and R. Kia, Adv. Synth. Catal., 2013, 355, 957–972 CrossRef CAS.
-
(a) A. R. Tiwari, T. Akash and B. M. Bhanage, Org. Biomol. Chem., 2015, 13, 10973–10976 RSC;
(b) Q. You, F. Wang, C. Wu, T. Shi, D. Min, H. Chen and W. Zhang, Org. Biomol. Chem., 2015, 13, 6723–6727 RSC;
(c) J. Shen and X. Meng, Catal. Commun., 2019, 127, 58–63 CrossRef CAS;
(d) F. Xie, M. Chen, X. Wang, H. Jing and M. Zhang, Org. Biomol. Chem., 2014, 12, 2761 RSC.
- J. Chen, H. Meng, F. Zhang, F. Xiao and G. Deng, Green Chem., 2019, 21, 5201–5206 RSC.
- B. Xu, Q. Jiang, A. Zhao, J. Jia, Q. Liu, W. Luo and C. Guo, Chem. Commun., 2015, 51, 11264–11267 RSC.
-
(a) M. Fu, H. Li, M. Su, Z. Chao, Y. Liu, Q. Liu and C. Guo, Adv. Synth. Catal., 2019, 361, 3420–3429 CrossRef CAS;
(b) Z. Cao, H. Lv, Y. Liu, Z. Nie, H. Liu, T. Yang, W. Luo, Q. Liu and C. Guo, Adv. Synth. Catal., 2019, 361, 1632–1640 CrossRef CAS;
(c) Y. Liu, Y. Hu, Z. Cao, X. Zhan, W. Luo, Q. Liu and C. Guo, Adv. Synth. Catal., 2018, 360, 2691–2695 CrossRef CAS;
(d) Y. Liu, Y. Hu, Z. Cao, X. Zhan, W. Luo, Q. Liu and C. Guo, Adv. Synth. Catal., 2019, 361, 1084–1091 CrossRef CAS.
-
(a) J. Chen, H. Meng, F. Zhang, F. Xiao and G. Deng, Green Chem., 2019, 21, 5201–5206 RSC;
(b) J. Chen, D. Chang, F. Xiao and G. Deng, Green Chem., 2018, 20, 5459–5463 RSC.
-
(a) H. Chen, S. J. Sun and Y. H. Zhou, Tetrahedron Lett., 2019, 60, 1434–1436 CrossRef CAS;
(b) C. Fang, M. Li, X. Hu, W. Mo, B. Hu, N. Sun, L. Jin and Z. Shen, RSC Adv., 2017, 7, 1484–1489 RSC;
(c) J. Shie and J. Fang, J. Org. Chem., 2003, 68, 1158–1160 CrossRef CAS PubMed;
(d) M. Okimoto and T. Chiba, J. Org. Chem., 1988, 53, 219–221 CrossRef.
-
(a) J. Chen, H. Meng, F. Zhang, F. Xiao and G. Deng, Green Chem., 2019, 21, 5201–5206 RSC;
(b) J. Chen, D. Chang, F. Xiao and G. Deng, Green Chem., 2018, 20, 5459–5463 RSC;
(c) F. Ke, P. Zhang, C. Lin, X. Lin, J. Xu and X. Zhou, Org. Biomol. Chem., 2018, 16, 8090–8094 RSC.
Footnote |
† Electronic supplementary information (ESI) available. See DOI: 10.1039/d0ra03323e |
|
This journal is © The Royal Society of Chemistry 2020 |
Click here to see how this site uses Cookies. View our privacy policy here.