DOI:
10.1039/D0RA03311A
(Paper)
RSC Adv., 2020,
10, 23510-23521
Intervention effects of lotus leaf flavonoids on gastric mucosal lesions in mice infected with Helicobacter pylori
Received
13th April 2020
, Accepted 15th June 2020
First published on 19th June 2020
Abstract
Helicobacter pylori (H. pylori) is one of the main factors that cause gastric lesions. The lotus leaf is an edible plant used in traditional Eastern medicine. This study evaluates the intervention effects of lotus leaf flavonoids (LLF) on gastric mucosal lesions in mice infected with H. pylori and explores their mechanism of action. High-performance liquid chromatography analysis reveals that LLF contain kaempferitrin (kaempferol-3,7-dirhamnoside), hypericin, astragalin (kaempferol-3-glucoside), phlorizin, and quercetin. LLF can reduce the number of gastric mucosal lesions and tissue lesions in mice with H. pylori-induced gastric lesions. LLF can increase the levels of somatostatin and vasoactive intestinal peptide in the serum of mice with gastric lesions and decrease the levels of substance P and endothelin-1 to inhibit gastric lesions. LLF can also reduce the levels of interleukin (IL)-6, IL-12, tumor necrosis factor (TNF)-α, and interferon-gamma cytokines in the serum of mice with gastric lesions. Using a quantitative polymerase chain reaction assay it can be seen that LLF can downregulate mRNA expressions of TNF-α, IL-1β, myeloperoxidase, keratin (KRT) 16, KRT6b, and transglutaminase 3 epidermal in the gastric tissues of mice with gastric lesions. Western blot analysis indicates that LLF can downregulate the protein expressions of caspase-1, Nod-like receptor protein 3, IL-1β, TNF-α, and Toll-like receptor 4 in the gastric tissues of mice with gastric lesions. LLF have beneficial effects on gastric lesions induced by H. pylori. Meanwhile LLF is more active in competition with ranitidine. LLF represent an active substance that can inhibit H. pylori-induced gastric lesions. The flavones of LLF may enhance the inhibition of gastric mucosal lesions by promoting the interaction between the compounds.
1 Introduction
Lotus leaves are the leaves of Nelumbo nucifera Gaertn., which belongs to the Nymphaeaceae family. Dried lotus leaves are commonly processed into tea or Chinese medicine.1 Traditionally, people drink lotus leaf tea to lose weight and protect their stomach and intestines against inflammatory symptoms or diseases. In traditional Chinese medicine, this herbal ingredient plays a role in reducing fat, with properties for anti-aging, anti-convulsions, anti-fibrosis effects of tissues, etc.2–5 Because the lotus leaf has many functions, it is now used for the development and production of healthy foods. In addition to the carbohydrates, lipids, and proteins commonly contained in plants, the lotus leaf is rich in biologically active ingredients of which flavonoids and alkaloids are the most abundant.6 Therefore, lotus leaves have been used to produce functional foods in order to exert their biological activity. Through the development of high-quality functional foods, several studies have demonstrated that extracted lotus leaf flavonoids (LLF) have better antioxidant, anti-inflammatory, and lipid-lowering effects than ordinary lotus leaves.7–9 LLF have been initially confirmed as a type of biologically active substance, which warrants additional investigation.
A critical physiological balance exists between gastric acid secreted by the body and gastroduodenal defense, keeping the body in a normal state. If the balance is disrupted by factors including anti-inflammatory agents, Helicobacter pylori (H. pylori), bile salts, acids, ethanol, pepsin, etc., the gastric mucosa will be injured, which may lead in severe cases to gastric ulcers.10 Legions engendered by gastric ulcers usually reach or exceed the muscularis of the gastric mucosa. H. pylori is an important cause of ulcers, and the lesions it induces are often serious.11 H. pylori infects people of different ethnicities and regions, with approximately 80% of them living in developing countries and 40% in developed countries. The number of infections among men is slightly higher than that among women.12 H. pylori reaches the gastric mucosa by the mouth, then settles, and causes the infections. Over weeks or months, it leads to chronic and superficial gastritis, which develops into duodenal ulcer, gastric ulcer, lymphoproliferative gastric lymphoma, and chronic atrophic gastritis, etc., over a period of years or decades. The latter will most likely lead to gastric cancer. H. pylori infections increase the risk of gastric cancer from 2.7 to 12 times. Without H. pylori infections, 35% to 89% of gastric cancers would not occur.13 Patients with gastric ulcers induced by H. pylori may suffer from gastroduodenal bleeding, perforation, obstruction, etc. In severe cases, this condition can even lead to death. For patients with peptic ulcers infected by H. pylori, triple or quadruple therapy is generally chosen to clear H. pylori infections and protect the gastric mucosa. Although this therapeutic approach is fast acting, it is prone to drug resistance, it is costly, and it has many side effects.14
Gastric health is closely related to our quality of life. Problems with digestion and absorption, metabolism, and mucosal immune function of gastrointestinal tract will decrease an individual's quality of life. In particular, the recurrence of inflammations and the repairs of gastric mucosal tissues caused by gastric ulcers will lead to “intestinal metaplasia” in the stomach, which might develop into early gastric cancer.15 Protecting the gastric mucosa with active substances in plants has become a hot spot in research. Many types of flavonoids have been extracted from natural plants, most of which have bioactive effects. Studies have confirmed that flavonoids extracted from plants have a variety of biological activities, including antioxidant, antihypertensive, anti-inflammatory, and anticancer effects.16 Meanwhile, more studies have reported that the flavonoid content in plants is high and that the total flavonoid content of dried lotus leaves exceeds 8%, therefore indicating its facile extraction for processing and utilization.17
At this stage, flavonoids from lotus leaves have been partially applied in research. Some preliminary studies have been conducted, but in-depth scientific studies are still lacking, especially studies on the correlation mechanism between its components and its biological activity. Focusing on LLF, this paper performs an in vivo study on the intervention effects of LLF on the gastric mucosal lesions of H. pylori-infected mice, analyzes the chemical composition of LLF, and elaborates on the correlation mechanism between their composition and their activity by combining molecular biological detection technology to provide a theoretical basis for further developments of LLF resources.
2 Materials and methods
2.1 LLF extraction
We added 500 g of frozen and dried lotus leaves (Anhui Xile Garden Food Co., Ltd., Anqing, Anhui, China) to a 5 L beaker; after pulverizing the leaves, we added 5 L of 80% ethanol adhering to a 1
:
10 ratio of lotus leaf to ethanol; we stirred it for 5 h and filtered it to obtain the crude extract of lotus leaf flavonoids liquid. FL-3 microporous resin (Shanghai Yiji Biology Co., Ltd, Shanghai, China) was loaded into a glass column, the extract was poured slowly into a glass column filled with macroporous resin, and the resin was eluted with 80% ethanol until it became colorless. Using a beaker to collect the eluted solution, we removed the ethanol from the solution by rotary evaporation, and finally, we obtained the lotus leaf flavonoids extract.18
2.2 High-performance liquid chromatography detection ingredients
We added 10 mg of accurately weighed LLF extract to 1 mL of dimethyl sulfoxide. The composition of LLF was detected under the following liquid chromatography conditions: C18 chromatographic column (Agilent ZorbaxSB-C, 5 μm, 4.6 × 250 mm), using 0.1% ice acetic acid as the aqueous mobile phase B, pure acetonitrile as the organic mobile phase C; column temperature was controlled at 35 °C, flow rate was 0.5 mL min−1, the detection wavelength was 280 nm, and the injection volume was 10 μL (Ultimate 3000, Thermo Fisher Scientific, Inc., Waltham, MA, USA).19
2.3 Murine models with gastric mucosal lesions
A total of 100 6 week-old male healthy BALB/c mice (SPF grade, Chongqing Medical University, Chongqing, China), weighing between 19 g and 22 g, were randomly divided into five groups: normal group, model group, ranitidine gavage group (positive control group), low-dose LLF (LLF-L) gavage group (50 mg kg−1), and high-dose LLF (LLF-H) gavage group (100 mg kg−1). There were 20 mice per group. After 1 week of acclimatization, except for the normal group, each mouse from the other groups was administered 0.5 mL H. pylori solution (1 × 109 cfu mL−1) in the stomach after 12 h of fasting and 4 h of water deprivation. This procedure was followed by 2 more hours of fasting and water deprivation after gavage. Gavages were administered a total of five times with intervals of 1 day between each gavage. After 10 days, the mice in the normal and model groups were administered 2 mL of normal saline every day; the mice in the ranitidine group were administered 0.2 mL of ranitidine solution at 100 mg per kg per day; and the mice in the LLF-H and LLF-L groups were administered LLF 50 mg kg−1 and 100 mg per kg per day, respectively. The above gavage occurred during a 2 week period. All of the mice were then fasted but were allowed to drink freely. After fasting for 24 h, the mice were killed by neck dissection. Plasma samples were obtained from the heart, and gastric tissues were dissected for use.20 Photographs of the gastric mucosa of the mice were taken, the area of gastric tissue lesions was measured using ImageJ software, and the inhibition rate of gastric lesions was calculated in the following manner: inhibition rate of gastric lesion (%) = (gastric lesion area of model group mouse per mm2 − gastric lesion area of sample group mouse per mm2)/gastric lesion area of model group mouse per mm2 × 100. The study was approved by the Ethics Committee of Chongqing Collaborative Innovation Center for Functional Food (201901008B, Chongqing, China).
2.4 Detection of Hp eradication
The stomach was removed from the mouse, using an aseptic surgical procedure; the stomach cavity was cut along the greater curvature and flushed with saline; and H. pylori eradication by rapid urease test and bacterial culture was observed.21
2.5 Determination of the murine serum level
The plasma was separated by centrifugation at 4000 rpm for 10 min, and the upper blood was separated. The murine serum level was determined using endothelin-1 (ET-1), somatostatin (SS), substance P (SP), and vasoactive intestinal peptide (VIP) kits (Nanjing Jiancheng Bioengineering Institute, Nanjing City, China).22
2.6 Determination of cytokine levels in the murine serum
Interleukin (IL)-6, IL-12, tumor necrosis factor (TNF)-α, and interferon (IFN)-γ cytokine assay kits (Abcam, Cambridge, MA, USA) were used to determine cytokine levels in the murine serum, which was separated by centrifugation.23
2.7 Observation of gastric tissues and pathological changes
We obtained 0.5 cm2 of the murine gastric tissues and fixed it in 10% formalin solution for 48 h. Dehydration, clearing, waxing, embedding, and slicing were performed on the tissues for hematoxylin and eosin staining. The morphological changes of the tissues were observed using an optical microscope (BX43, Olympus, Tokyo, Japan).24
2.8 Quantitative polymerase chain reaction assay for mRNA expression in the murine gastric tissue
The murine gastric tissue was pulverized using a homogenizer. The total RNA was extracted from the tissue using TRIzol™ Reagent (Invitrogen, Carlsbad, CA, USA), and the total RNA was diluted into 1 μg μL−1. We removed 1 μL of the diluted total RNA solution, and a reverse transcription was performed according to the reverse transcription kit method to obtain a cDNA template. We mixed 1 μL of cDNA template with 10 μL of SYBR Green PCR Master Mi, 1 μL of upstream and downstream primers (Thermo Fisher Scientific) (Table 1), and 7 μL of sterile distilled water at 95 °C for 60 s. Forty cycles were carried out at 95 °C, 15 s; 55 °C, 30 s; and 72 °C, 35 s. Finally, detections were conducted at 95 °C, 30 s, and 55 °C, 35 s; and 2−ΔΔCt method was applied to calculate the relative gene expression, using GAPDH as an internal reference.25
Table 1 Sequences of primers used in the qPCR assay
Gene name |
Sequence |
TNF-α |
Forward: 5′-GACCCTCAGACTCAGATCATCCTTCT-3′ |
Reverse: 5′-ACGCTGGCTCAGCCACTC-3′ |
IL-1β |
Forward: 5′-CTCCATGAGCTTTGTACAAGG-3′ |
Reverse: 5′-TGCTGATGTACCAGTTGGGG-3′ |
MPO |
Forward: 5′-TGGGAAAGTCTTCGACGTGAC-3′ |
Reverse: 5′-GTGCATCCTTATCCAGGCAGA-3′ |
KRT16 |
Forward: 5′-GGTGGCCTCTAACAGTGATCT-3′ |
Reverse: 5′-TGCATACAGTATCTGCCTTTGG-3′ |
KRT6b |
Forward: 5′-TGTGTCCTCTCGCTTCTCTTTTT-3′ |
Reverse: 5′-GAAAAATGGGGTTTCCCAGGC-3′ |
TGM3 |
Forward: 5′-CTGGCAGGTGCCTATGAATCG-3′ |
Reverse: 5′-TCAAGACTTCGGTTGCAGACTA-3′ |
GAPDH |
Forward: 5′-AGGTCGGTGTGAACGGATTTG-3′ |
Reverse: 5′-GGGGTCGTTGATGGCAACA-3′ |
2.9 Western blot assay to determine protein expression in the murine gastric tissue
A total of 100 mg of gastric tissue sample was homogenized with 1 mL of RIPA and 10 μL of PMSF (Thermo Fisher Scientific) at 12
000 rpm; it was lysed at 4 °C for 5 min, it was centrifuged at 12
000 rpm and 4 °C for 15 min; the intermediate protein layer solution was removed to perform protein quantification using a BCA protein quantification kit. The samples of each group were diluted to 50 μg mL−1, the diluted protein was mixed with sample buffer at a 4
:
1 ratio, then heated at 100 °C for 5 min followed by 5 min of ice bath. Acrylamide, resolving buffer, stacking buffer, distilled water, 10% APS, and TEMED were mixed in proportion to make SDS-PAGE separation gel and concentrated gel (Thermo Fisher Scientific), which were poured into a running gel plate for later use. Prestained protein ladder and samples were spotted into the sample wells of the running gel plate, respectively, and the protein-loaded SDS-PAGE gel was subjected to vertical gel electrophoresis for 50 min. After being activated with methanol for 1 min, the PVDF membrane (Thermo Fisher Scientific) was transformed into a membrane module in the order of negative electrode, sponge, filter paper, SDS-PAGE adhesive, PVDF membrane, filter paper, sponge, and positive electrode. After the conversion, the PVDF membrane was sealed with 1× TBST solution containing 5% skimmed milk for 1 h. The PVDF membrane was washed with 1× TBST and incubated with primary antibody at 25 °C for 2 h. The PVDF membrane was cleaned with 1× TBST again, and incubated with a secondary antibody at 25 °C for 1 h. Finally, the PVDF membrane was sprayed with Supersignal West Pico PLUS and placed in iBright FL1000 for observation.26 β-Actin was determined as an internal reference protein in this experiment.
2.10 Data analysis
All of the experiments were repeated three times and the results of parallel experiments were averaged; the one-way analysis of variance method was used to analyze whether the data of each group were significantly different from each other at P < 0.05 level (SAS 9.1 statistical software).
3 Results
3.1 Composition analysis of LLF
As shown in Fig. 1 and Table 2, LLF contain five flavonoids, namely, kaempferitrin, hypericin, astragalin, phlorizin, and quercetin. Through detecting the peak areas, it is found that the content of these five compounds decreases in the aforementioned order.
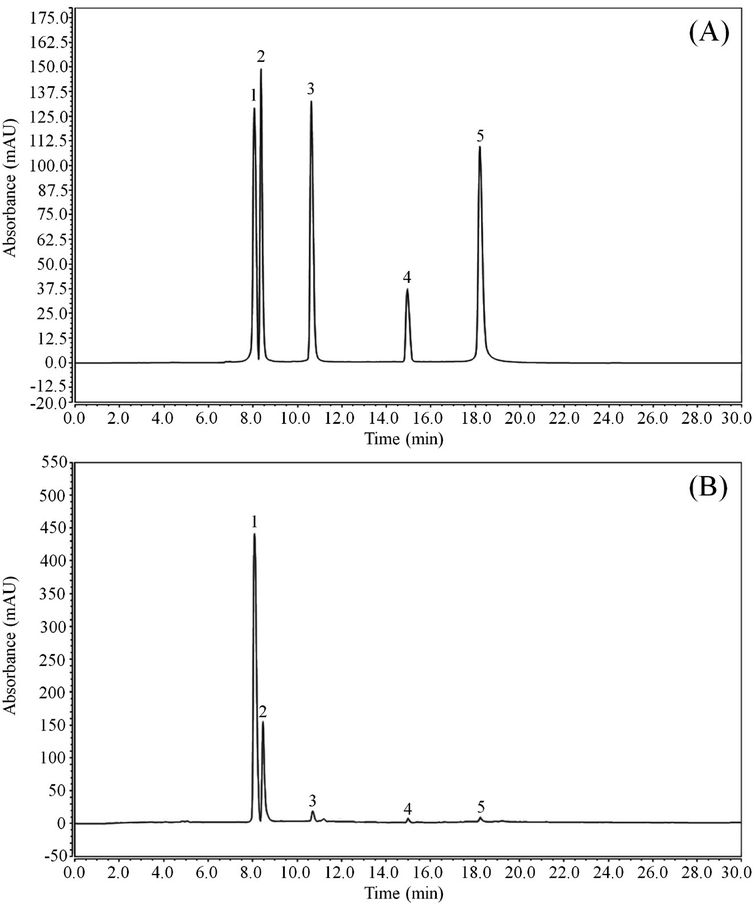 |
| Fig. 1 Flavonoid constituents of lotus leaf. (A) Standard chromatograms; (B) lotus leaf flavonoids chromatograms. (1) kaempferitrin; (2) hypericin; (3) astragalin; (4) phlorizin; (5) quercetin. | |
Table 2 Flavonoid contents of lotus leaf
Flavonoid |
Linear equation |
R2 |
Content (mg g−1) |
Kaempferitrin |
Y = 8.1351X + 3.7426 |
0.9875 |
470.32 ± 1.25 |
Hypericin |
Y = 3.1923X + 9.5106 |
0.9867 |
194.10 ± 1.37 |
Astragalin |
Y = 11.377X + 0.8632 |
0.9912 |
59.02 ± 0.62 |
Phlorizin |
Y = 0.9802X + 0.3006 |
0.9906 |
1.62 ± 0.12 |
Quercetin |
Y = 10.427X − 3.0913 |
0.9934 |
1.03 ± 0.11 |
3.2 Effect of LLF on the degree of gastric mucosal lesions in mice
Fig. 2 and Table 3 show that the area of gastric mucosal lesions in the model murine group is the largest, and that the area of gastric mucosal lesions in the mouse applied with ranitidine, LLF-L, and LLF-H is significantly smaller (P < 0.05). The inhibition rates of gastric lesions in the ranitidine and LLF-H groups were noted to be higher than those in the LLF-L group, and the effect of LLF-H is better than that of ranitidine.
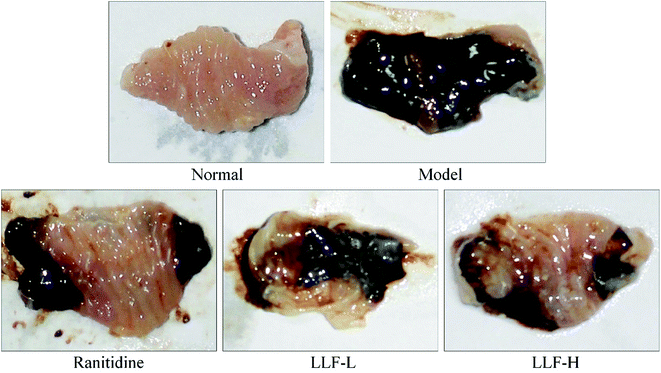 |
| Fig. 2 Observation of gastric mucosal lesions in mice with Helicobacter pylori. Ranitidine group: 100 mg per kg b.w. ranitidine treatment dose; FLL-L group: 50 mg per kg b.w. lotus leaf flavonoids dose; FLL-H group: 100 mg per kg b.w. lotus leaf flavonoids dose. | |
Table 3 Degree of gastric mucosal lesions in mice with Helicobacter pyloria
Group |
Gastric lesion area (mm2) |
Gastric lesion inhibitory rate (%) |
Values presented are the mean ± standard deviation. a−e Mean values with different letters in the same column are significantly different (P < 0.05) according to Duncan's new MRT. Ranitidine group: 100 mg per kg b.w. ranitidine treatment dose; FLL-L group: 50 mg per kg b.w. lotus leaf flavonoids dose; FLL-H group: 100 mg per kg b.w. lotus leaf flavonoids dose. |
Normal |
0.00 ± 0.00e |
100.00 ± 0.00a |
Model |
25.37 ± 2.16a |
0.00 ± 0.00e |
Ranitidine |
9.32 ± 0.52c |
63.26 ± 2.43c |
FLL-L |
16.86 ± 1.03b |
33.54 ± 1.18d |
FLL-H |
6.71 ± 0.43d |
73.55 ± 2.20b |
3.3 Effect of LLF on H. pylori eradication in the murine stomach
Table 4 shows that there are zero cases of eradication in the model group. The eradication rate of the ranitidine group is 75%, and the eradication rates of LLF-L and LLF-H groups are 40% and 85%, respectively, showing a certain dose–response relationship (P < 0.05).
Table 4 Helicobacter pylori eradication in the mouse stomacha
Group |
Negative number of smear |
Negative number of urease test |
Negative number of H. pylori culture |
Eradication number (eradication rate, %) |
Values presented are the mean ± standard deviation. Ranitidine group: 100 mg per kg b.w. ranitidine treatment dose; FLL-L group: 50 mg per kg b.w. lotus leaf flavonoids dose; FLL-H group: 100 mg per kg b.w. lotus leaf flavonoids dose. |
Normal |
20 |
20 |
20 |
— |
Control |
0 |
0 |
0 |
0 |
Ranitidine |
18 |
16 |
15 |
15 (75%) |
LITPL |
12 |
10 |
8 |
8 (40%) |
LITPH |
18 |
17 |
17 |
17 (85%) |
3.4 Effects of LLF on SS, SP, VIP, and ET-1 levels in the murine serum
Table 5 shows that SS and VIP levels in the murine serum of the model group are the lowest, and that SP and ET-1 levels are the highest in the murine serum of the model group. The normal murine group shows the opposite trend to the model murine group. Compared with the model murine group, SS and VIP levels increase significantly (P < 0.05) while SP and ET-1 levels decrease significantly (P < 0.05) after LLF treatment in the mouse with gastric lesions. LLF-H has a stronger ability to regulate these serum levels than that of ranitidine, which makes the serum indicators of the mouse closer to those of a normal mouse.
Table 5 Serum levels of SS, SP, VIP and ET-1 in mice with Helicobacter pyloria
Group |
SS (pg mL−1) |
SP (pg mL−1) |
VIP (pg mL−1) |
ET-1 (pg mL−1) |
Values presented are the mean ± standard deviation. a−e Mean values with different letters in the same column are significantly different (P < 0.05) according to Duncan's new MRT. Ranitidine group: 100 mg per kg b.w. ranitidine treatment dose; FLL-L group: 50 mg per kg b.w. lotus leaf flavonoids dose; FLL-H group: 100 mg per kg b.w. lotus leaf flavonoids dose. |
Normal |
92.05 ± 1.28a |
17.65 ± 0.49e |
85.77 ± 1.31a |
3.82 ± 0.26e |
Control |
37.56 ± 0.67e |
84.33 ± 0.89a |
20.86 ± 0.72e |
44.03 ± 0.69a |
Ranitidine |
66.37 ± 1.04c |
44.39 ± 1.02c |
69.74 ± 1.16c |
9.63 ± 0.58d |
LITPL |
48.36 ± 0.80d |
70.25 ± 1.17b |
48.79 ± 1.42d |
28.97 ± 1.06b |
LITPH |
77.10 ± 1.18b |
27.28 ± 0.92d |
76.02 ± 1.20b |
6.92 ± 0.44c |
3.5 Effects of LLF on IL-6, IL-12, TNF-α, and IFN-γ cytokines in the murine serum
As shown in Table 6, the levels of IL-6, IL-12, TNF-α, and IFN-γ cytokines in the murine serum of the normal group are significantly lower than those in the other groups (P < 0.05). The levels of IL-6, IL-12, TNF-α, and IFN-γ cytokines increase significantly after H. pylori induces gastric lesions. Ranitidine and LLF can significantly inhibit increases in IL-6, IL-12, TNF-α, and IFN-γ levels caused by H. pylori, and the effect of LLF-H is better than that of ranitidine and LLF-L.
Table 6 Serum cytokine levels of IL-6, IL-12, TNF-α and IFN-γ in mice with Helicobacter pyloria
Group |
IL-6 (pg mL−1) |
IL-12 (pg mL−1) |
TNF-α (pg mL−1) |
IFN-γ (pg mL−1) |
Values presented are the mean ± standard deviation. a−e Mean values with different letters in the same column are significantly different (P < 0.05) according to Duncan's new MRT. Ranitidine group: 100 mg per kg b.w. ranitidine treatment dose; FLL-L group: 50 mg per kg b.w. lotus leaf flavonoids dose; FLL-H group: 100 mg per kg b.w. lotus leaf flavonoids dose. |
Normal |
43.64 ± 2.71e |
10.36 ± 0.42e |
35.10 ± 2.43e |
30.86 ± 1.46e |
Control |
156.38 ± 8.10a |
53.67 ± 0.71a |
131.08 ± 7.32a |
108.92 ± 5.32a |
Ranitidine |
78.36 ± 3.84c |
25.47 ± 0.52c |
80.34 ± 5.58c |
61.30 ± 4.88c |
LITPL |
119.31 ± 6.83b |
40.36 ± 0.36b |
105.67 ± 4.38b |
89.15 ± 5.02b |
LITPH |
62.17 ± 2.93d |
17.36 ± 0.31d |
67.12 ± 4.09d |
45.67 ± 2.91d |
3.6 Pathological effects of LLF on the murine gastric mucosa
As shown in Fig. 3, the gastric tissue in the normal group is smooth and soft, and no mucosal swelling or erythema is observed. The distribution of gastric mucosal cells and glands is regular and continuous using light microscopy. The surface of the gastric tissue of the model murine group is swollen; it is not flat enough and is eroded; the glands are arranged in a slightly disorderly way; infiltrated inflammatory cells are observed using a light microscope. In the ranitidine group, the gastric mucosa is smoother; less severe swelling is noticed; and the glands are still continuous. The stomach tissues are not deeply injured and there are some erosions. No visible ulcers are observed. A small amount of infiltrating inflammatory cells can be noticed using a light microscope. The gastric tissue lesions of the LLF-H gavage murine group are significantly fewer than those of the model group. The glands are regularly distributed. A few swellings are evident in some cases. Some point-like erosions are also observed. No ulcer lesions are observed on the mucosa. Only a few inflammatory reactions are observed using a light microscope; gastric tissue lesions in the LLF-L gavage murine group are fewer than those of the model murine group, but tissue swellings, erosions, and the number of inflammatory cells in infiltration are all larger than those of the ranitidine group and LLF-H gavage group.
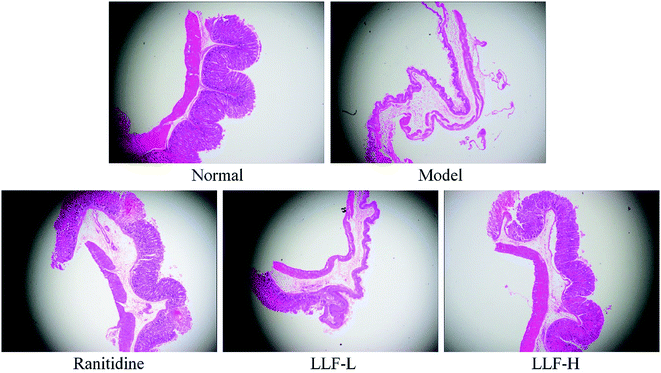 |
| Fig. 3 Pathological observation of gastric mucosal lesions in mice with Helicobacter pylori. Ranitidine group: 100 mg per kg b.w. ranitidine treatment dose; FLL-L group: 50 mg per kg b.w. lotus leaf flavonoids dose; FLL-H group: 100 mg per kg b.w. lotus leaf flavonoids dose. | |
3.7 Effect of LLF on the expression of inflammation-related mRNA in the murine gastric tissue
As shown in Fig. 4, the expressions of TNF-α, IL-1β, and myeloperoxidase (MPO) mRNA in the gastric tissue of the normal murine group are the lowest, while the above expressions in the gastric tissue of the model murine group are the highest. TNF-α, IL-1β, and MPO mRNA expressions of ranitidine, LLF-L, and LLF-H groups are significantly (P < 0.05) lower than those of the model group, and the above expression in the LLF-H group is closest to that of the normal group.
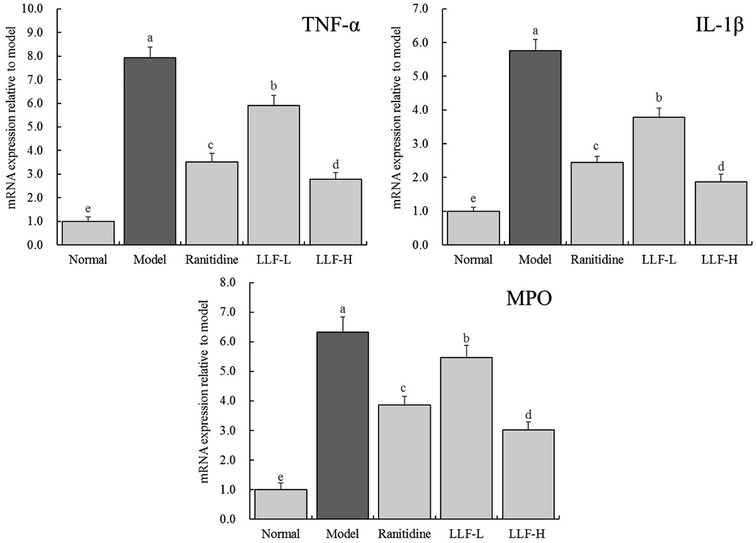 |
| Fig. 4 mRNA expression of TNF-α, IL-1β and MPO in gastric tissue of mice. Values presented are the mean ± standard deviation. a–eMean values with different letters in the bars are significantly different (p < 0.05) according to Duncan's multiple-range test. Ranitidine group: 100 mg per kg b.w. ranitidine treatment dose; FLL-L group: 50 mg per kg b.w. lotus leaf flavonoids dose; FLL-H group: 100 mg per kg b.w. lotus leaf flavonoids dose. | |
3.8 Effect of LLF on keratinization-related mRNA expression in the murine gastric tissue
As shown in Fig. 5, the expression levels of KRT16, KRT6b, and TGM3 mRNA in the model group are 4.08, 3.89, and 2.74 times that of the normal group, respectively. Compared with the model group, the corresponding mRNA expression levels of ranitidine, LLF-H group, and LLF-L group are significantly lower (P < 0.05), and the expression levels of KRT16, KRT6b, and TGM3 mRNA in gastric tissues of the LLF-H mouse are closest to the normal group.
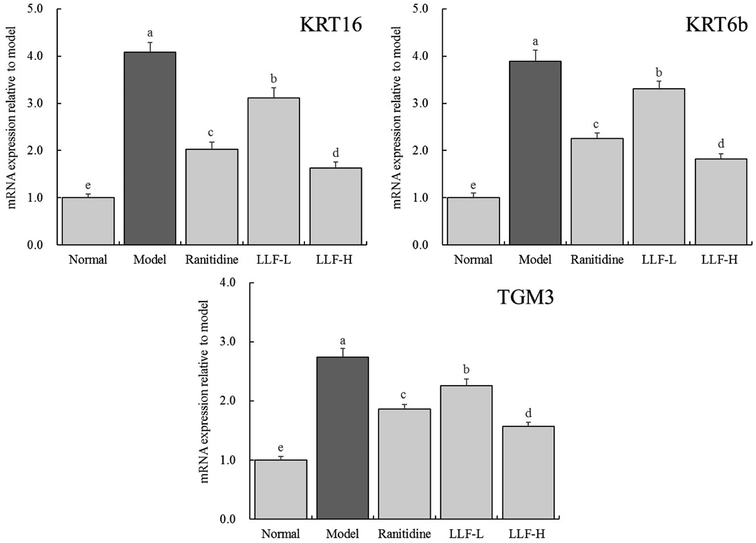 |
| Fig. 5 mRNA expression of KRT16, KRT6b and TGM3 in gastric tissue of mice. Values presented are the mean ± standard deviation. a–eMean values with different letters in the bars are significantly different (p < 0.05) according to Duncan's multiple-range test. Ranitidine group: 100 mg per kg b.w. ranitidine treatment dose; FLL-L group: 50 mg per kg b.w. lotus leaf flavonoids dose; FLL-H group: 100 mg per kg b.w. lotus leaf flavonoids dose. | |
3.9 Effect of LLF on the expression of inflammation-related proteins in the murine gastric tissue
As shown in Fig. 6, the content of caspase-1, NLRP3, IL-1β, TNF-α, and TLR4 in the gastric tissue of the mouse from the model group is significantly higher than that of the normal group (P < 0.05). The content of inflammation-related proteins in gastric tissues in mice that were administrated with LLF significantly decreases (P < 0.05), and it is dose-dependent; compared to ranitidine, LLF-H has a stronger ability to downregulate these expressions.
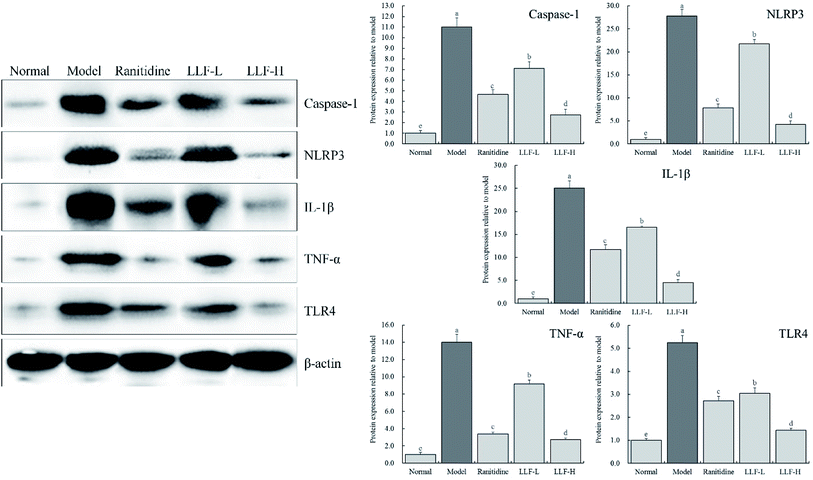 |
| Fig. 6 Protein expression of Caspase-1, NLRP3, IL-1β, TNF-α and TLR4 in gastric tissue of mice. Values presented are the mean ± standard deviation. a–eMean values with different letters in the bars are significantly different (p < 0.05) according to Duncan's multiple-range test. Ranitidine group: 100 mg per kg b.w. ranitidine treatment dose; FLL-L group: 50 mg per kg b.w. lotus leaf flavonoids dose; FLL-H group: 100 mg per kg b.w. lotus leaf flavonoids dose. | |
4 Discussion
H. pylori is a class of Gram-negative microaerobic bacteria that can be implanted in human gastric tissues. It is an important cause of peptic ulcers.27 A large portion of peptic ulcers can be improved or completely cured by clearing H. pylori.28 After a large number of clinical observations and animal experiments, H. pylori clearance is found to be closely related to the diminution of clinical gastric ulcers.29,30 Visual observations of gastric mucosa in animal experiments and pathological morphology of section tissues under a microscope are both visual detection methods to evaluate the degree of gastric lesions.31 Observations in this study clearly show that LLF interfere with H. pylori induction and, therefore, can relieve gastric lesions.
SS can inhibit gastric peristalsis while reducing the blood flow in the wall of the gastrointestinal tract. It also can inhibit the secretion of gastric acid and, thus, alleviate gastric discomfort.32 SP can promote the secretion of gastric acid and pepsin, intensify gastric contraction, and also increase gastric blood flow in the stomach, thereby exacerbating gastric lesions.33 VIP can lead to the massive release of SS from D cells, inhibit gastric acid secretion, and reduce gastric lesions through its own action and that of SS.34 ET-1 is an active substance that promotes vasoconstriction. When ET-1 is present in large amounts, it can cause local hypoxia and acidosis in the stomach as well as aggravate gastric mucosal lesions.35 LLF can inhibit gastric lesions by increasing the levels of SS and VIP and reducing the levels of SP and ET-1 in the serum of mice with gastric lesions, thereby protecting the gastric mucosa.
When the stomach is inflamed, the level of proinflammatory cytokines in the body increases, which leads to lesions aggravating the gastric mucosal cells and gastric tissues. IL-6 is closely related to inflammation of gastric tissues. As a chemokine, IL-6 can make inflammatory cells chemotactic, causing a large number of inflammatory mediators to be produced and released, leading to destruction of the gastric mucosal barrier, aggravation of lesions, and gastric mucosal inflammation.36 IL-12 is also a cytokine closely related to inflammation, which shows in abnormally high levels in the case of inflammation of gastric tissues.37 IFN-γ is a cytokine produced by activated T cells and NK cells. It is apparent in ulcerous tissues and shows in high levels after gastric mucosal tissue lesions.38 TNF-α, like IFN-γ, plays a role in the development of gastric ulcers. It can cause neutrophil infiltrations and the destruction of the cytoskeleton; it can also generate large-scale releases of cytokines, provoking lesions of gastric mucosal cells and exacerbating gastric tissue lesions.39 In this study, the levels of IL-6, IL-12, TNF-α, and IFN-γ cytokines in the murine serum significantly increase after the appearance of gastric lesions induced by H. pylori. When LLF take effect, the levels of these pro-inflammatory cytokines downregulate. At the same treatment concentration, the effect of LLF was even better than silymarin.
Injured gastric tissues will activate the body's immune system and stimulate the production of pro-inflammatory factors, such as TNF-α and IL-1β.32 As an important cytokine with multiple functions, TNF-α has a strong connection with the activation of related pro-inflammatory cytokines, such as IL-1β and IL-6, and is closely related to the progress of inflammatory diseases.40 The activation of IL-1β and IL-6 induces the production of TNF-α, which exacerbates the inflammatory response.41 The activity of MPO is directly proportional to the neutrophil concentration in inflammatory tissues; therefore, it is an important indicator for inflammation and neutrophil infiltration.42 Currently, MPO activity measurement is commonly used for the quantitative detection of experimental gastric lesion, colitis, and inflammations of human gastric ulcers.43 The results show that LLF can downregulate the expressions of TNF-α, IL-1β, and MPO mRNA in gastric tissues and reduce the inflammatory response.
When epithelial cells are in a diseased state, the expressions of KRT6-KRT16 reflects the state of keratinocyte activation and/or selective differentiation.44 KRT16 is a kind of keratin, which is related to cell proliferation and can induce cell activation and over proliferation.45 When the epidermal barrier is stimulated by external stimulus and inflammation, keratinocytes lacking KRT16 cannot properly regulate the generation of signals to resist danger.46 Knockout of the KRT6a and KRT6b genes in mice can cause increased ulcers in its upper digestive tract.47 This indicates that KRT16 and KRT6b, which are keratins related to wound healing, can balance the epithelial barrier. They usually appear to be upregulated after body injuries and play an important role in the reorganization of the keratin network.48 TGM3 is involved in the formation of keratinized envelopes in the late stage of cell differentiation.49 It can cross-link keratinized envelope precursors. The inflammation exacerbates when the TGM3 gene is knocked out.50 This indicates that LLF may affect cell differentiation through KRT16, KRT6b, and TGM3, resulting in reparation of gastric mucosa and improvement of the mucosal barrier function.
As a signal transduction receptor in pattern recognition receptors, Toll-like receptors (TLRs) can mediate innate and acquired immunity. TLR4 is an extremely important member of the TLRs family and plays a crucial role in noninfectious inflammatory responses. After TLR4 recognizes the ligand, it can activate a series of downstream signal transduction pathways, including the NF-κB pathway.51 The pro-inflammatory factor TNF-α also activates the NF-κB pathway. Activation of the NF-κB pathway leads to transcription and translation of many precursors of inflammatory factors, such as IL-1β and IL-18.52 Nod-like receptor protein (NLRP) 3 inflammatory bodies are composed of NLRP3 proteins, adapter proteins, and apoptosis-associated speck-like proteins containing CARD (ASC) and effector caspase-1. NLRP3 inflammatory bodies are currently regarded as important inflammatory regulators. Once activated, NLRP3 can transform precursors of inflammatory factors such as IL-1β and IL-18 into mature IL-1β and IL-18 and release them extracellularly, which causes an inflammatory response.53 The results show that in gastric lesion caused by H. pylori, caspase-1, NLRP3, IL-1β, TNF-α, and TLR4 are involved in the development of gastric inflammation. LLF has the potential to significantly downregulate caspase-1, NLRP3, IL-1β, TNF-α, and TLR4 protein expressions and reduce inflammation.
Kaempferitrin is a flavonoid glycoside, which has analgesic, anti-inflammatory, antidiabetic, and anticancer effects. It has also been found in some plants with gastro–protective effects.54,55 Hypericin is a natural product with a variety of physiological activities such as antioxidant, anti-inflammatory, and antihypertensive effects.56 Astragalin has antioxidant, anti-inflammatory, and blood lipid-lowering effects. It can inhibit the formation of TNF-α, IL-1β, and IL-6 in the case of inflammation.57,58 Phlorizin also has antioxidant, anti-inflammatory, and anticancer effects, etc., and its application has already been marketed.59 Quercetin has antioxidant, anti-inflammatory, and immune-improving effects, and its content in natural products with active effects is often high.60 LLF contain high kaempferitrin and hypericin, which are the core substances of LLFs for the prevention of gastric lesions. The combined effect of these five compounds forms the protective effect of LLF on gastric tissues. Various flavonoids may also promote each other and enhance biological activity. The transporters and metabolizing enzymes in the body can play a role with some flavonoids and promote the absorption of bioactive substances. In this way, a portion of flavonoids and enzymes can promote the absorption of other flavonoids in order to better play the role of mixed flavonoids.61 In addition, after entering the intestinal tract, flavonoids can interact with probiotics in the intestinal tract. Through the biological interaction between microorganisms and flavonoids, it can change the proportion of components in the mixed flavonoids to improve the activity.62 The differences in the structure of different flavonoids can form special conjugates in specific situations, which makes it more convenient for the body to absorb and use, and can also enhance the effect. Meanwhile, due to the ratio between the groups of flavonoids, the mixed flavonoids may play a more favorable role, and enhance the biological activity, including antioxidant effect.63 Therefore, the synergistic effect of different components of LLF on H. pylori-induced gastric mucosal lesions warrants additional investigation. In the future, it is necessary to clarify the synergistic effect and its advantages in the body.
5 Conclusions
By focusing on LLF, this paper evaluated the effects of LLF on gastric lesions induced by H. pylori in animal models. LLF can reduce inflammatory response. The intervention effects of LLF reduce gastric lesions induced by H. pylori. Meanwhile, based on the detection results of keratinized expression, it was speculated that LLF may treat gastric lesions induced by H. pylori by affecting the differentiation of gastric mucosal epithelial cells. Furthermore, compared with the positive drug ranitidine, it was found that in gastric lesions induced by H. pylori, the intervention effects of LLF are stronger than those of ranitidine. The lotus leaf is an edible plant that has long been used in traditional medicine. This study aimed to demonstrate the effects of LLF on the gastrointestinal tract. Research on the main active ingredient of lotus leaf, the flavone, has been sufficiently carried out. Yet, we still know little about the biological activity of LLF and their mechanism of action. Therefore, this study conducted a preliminary investigation at the molecular level to shed light on the mechanism of action of LLF by analyzing their components. Eventually, human clinical studies will be needed to fully clarify the mechanism of action of LLF.
Conflicts of interest
No conflicts of interest in this article.
Acknowledgements
This research was supported by High level Talents Project of Chongqing University of Education (2013BSRC001) and the Scientific Research Foundation for Returned Overseas Chinese Scholars, and the State Education Ministry [Jiaowaisiliu (2014)1685], China.
References
- S. H. Liu, T. H. Lu, C. C. Su, I. S. Lay, H. Y. Lin, K. M. Fang, T. J. Ho, K. L. Chen, Y. C. Su, W. C. Chiang and Y. W. Chen, Am. J. Chin. Med., 2014, 42, 869–889 CrossRef PubMed.
- K. Balani, R. G. Batista, D. Lahiri and A. Agarwal, Nanotechnology, 2009, 20, 305707 CrossRef PubMed.
- C. F. Huang, Y. W. Chen, C. Y. Yang, H. Y. Lin, T. D. Way, W. Chiang and S. H. Liu, J. Agric. Food Chem., 2011, 59, 1087–1094 CrossRef CAS PubMed.
- J. H. Choe, A. Jang, E. S. Lee, J. H. Choi, Y. S. Choi, D. J. Han, H. Y. Kim, M. A. Lee, S. Y. Shim and C. J. Kim, Meat Sci., 2011, 87, 12–18 CrossRef PubMed.
- M. J. Wu, L. Wang, C. Y. Weng and J. H. Yen, Am. J. Chin. Med., 2003, 31, 687–698 CrossRef PubMed.
- A. G. Huang, H. F. Shi, H. Wei, R. J. Dong and W. J. Zhou, Culinary Science Journal of Yangzhou University, 2006, 23, 23–25 Search PubMed.
- G. Q. Xiao, X. Y. Lu, Y. Tian, K. Yi and X. M. Zhou, Chem. Bioeng., 2006, 23, 1–2 CAS.
- B. Tao, M. Y. Chen, X. N. Li, Z. G. Wang and S. Y. Yao, Information on Traditional Chinese Medicine, 2001, 18, 14 Search PubMed.
- X. F. Zhong and G. D. Huang, Food & Machinery, 2006, 22, 138–140 Search PubMed.
- C. E. Rubin and N. Engl, J. Med., 1996, 335, 1841–1842 CAS.
- F. Hirayama, S. Takagi, H. Kusuhara, E. Iwao, Y. Yokoyama and Y. Ikeda, J. Gastroenterol., 1996, 31, 755–757 CrossRef CAS PubMed.
- J. K. Su, M. J. Yu, F. P. Zhou, X. H. Guan, W. L. Huang and Z. P. Yang, Chin. J. Coal Ind. Med., 2009, 12, 636–637 Search PubMed.
- L. Lin, F. Xie, F. Peng and J. C. Zhang, Food Nutr., 2018, 24, 86–88 Search PubMed.
- J. Y. Chen, P. N. Huang and X. Luo, China Mod. Med., 2017, 24, 46–48 Search PubMed.
- W. K. Leung, J. J. Kim, J. G. Kim, D. Y. Graham and A. R. Sepulveda, Am. J. Pathol., 2000, 156, 537–543 CrossRef CAS.
- Z. P. Xiao, Z. Y. Peng, M. J. Peng, W. B. Yan, Y. Z. Ouyang and H. L. Zhu, Mini Rev. Med. Chem., 2011, 11, 169–177 CrossRef CAS PubMed.
- Y. H. Jiang, Trans. Chin. Soc. Agric. Eng., 2004, 20, 168–171 Search PubMed.
- K. Zhu, G. Huang, J. Xie, X. Zhou, J. Mu and X. Zhao, Food Sci. Nutr., 2019, 7, 3808–3818 CrossRef CAS PubMed.
- T. Liu, F. Tan, X. Long, Y. Pan, J. Mu, X. Zhou, R. Yi and X. Zhao, Biomedicines, 2020, 8, 41 CrossRef PubMed.
- Y. Li, J. B. Wang, J. Jiao, Z. Y. Zhao and X. Y. Zhang, Chin. J. Lab. Diagnosis, 2007, 11, 537–540 Search PubMed.
- H. Zhao, Q. Yang and R. Sun, Suzhou Univ. J. Med. Sci., 2010, 30, 277–279 Search PubMed.
- F. Li, G. Huang, F. Tan, R. Yi, X. Zhou, J. Mu and X. Zhao, Food Sci. Nutr., 2020, 8, 379–389 CrossRef CAS PubMed.
- F. Li, D. Y. Lu, Q. Zhong, F. Tan, W. Li, W. Liao and X. Zhao, RSC Adv., 2020, 10, 1–9 RSC.
- X. Long, J. Song, X. Zhao, Y. Zhang, H. Wang, X. Liu and H. Suo, Food Sci. Nutr., 2020, 8, 237–245 CrossRef CAS PubMed.
- G. Li, F. Tan, Q. Zhang, A. Tan, Y. Cheng, Q. Zhou, M. Liu, X. Tan, L. Huang, R. Rouseff, H. Wu, X. Zhao, G. Liang and X. Zhao, J. Funct. Foods, 2020, 67, 103834 CrossRef CAS.
- R. Wang, X. Zeng, B. Liu, R. Yi, X. Zhou, J. Mu and X. Zhao, Food Funct., 2020, 11, 2679 RSC.
- S. Hellmig, S. Ott, P. Rosenstiel, U. Robert Fölsch, J. Hampe and S. Schreiber, Am. J. Gastroenterol., 2006, 101, 29–35 CrossRef CAS PubMed.
- J. P. Gisbert, M. Blanco, J. M. Mateos, L. Fernández-Salazar, M. Fernández-Bermejo, J. Cantero and J. M. Pajares, Dig. Dis. Sci., 1999, 44, 2295–2302 CrossRef CAS PubMed.
- C. Y. Wu, M. S. Wu, Y. J. Chen, C. J. Chen, J. T. Lin and G. H. Chen, Hepatogastroenterology, 2006, 53, 797–803 CAS.
- D. R. Campbell, M. M. Haber, E. Sheldon, C. Collis, N. Lukasik, B. Huang and J. L. Goldstein, Am. J. Gastroenterol., 2002, 97, 2208–2214 CrossRef CAS PubMed.
- A. Lee, M. Chen, N. Coltro, J. O'Rourke, S. Hazell, P. Hu and Y. Li, Zentralbl. Bakteriol., 1993, 280, 38–50 CrossRef CAS PubMed.
- M. L. Schubert, N. F. Edwards, A. Arimura and G. M. Makhlouf, Am. J. Physiol., 1987, 252, 485–490 Search PubMed.
- F. Karmeli, R. Eliakim, E. Okon and D. Rachmilewitz, Gastroenterology, 1991, 100, 1206–1216 CrossRef CAS.
- I. Synnerstad, E. Ekblad, F. Sundler and L. Holm, Gastroenterology, 1998, 114, 284–294 CrossRef CAS.
- S. A. Said and A. M. El-Mowafy, Regul. Pept., 1998, 73, 43–50 CrossRef CAS PubMed.
- Y. Wang, J. Song, Y. Liu, Y. Li and Z. Liu, Med. Sci. Monit., 2016, 22, 3523–3528 CrossRef CAS PubMed.
- R. A. Karttunen, T. J. Karttunen, M. M. Yousfi, H. M. el-Zimaity, D. Y. Graham and F. A. el-Zaatari, Scand. J. Gastroenterol., 1997, 32, 22–27 CrossRef CAS PubMed.
- C. H. Yun, A. Lundgren, J. Azem, A. Sjöling, J. Holmgren, A. M. Svennerholm and B. S. Lundin, Infect. Immun., 2005, 73, 1482–1490 CrossRef CAS PubMed.
- A. Kim, J. W. Lim, H. Kim and H. Kim, Nutr. Res., 2016, 36, 488–497 CrossRef CAS PubMed.
- M. Blaschke, R. Koepp, J. Cortis, M. Komrakova, M. Schieker, U. Hempel and H. Siggelkow, Adv. Clin. Exp. Med., 2018, 27, 45–56 CrossRef PubMed.
- P. F. Han, L. Wei, Z. Q. Duan, Z. L. Zhang, T. Y. Chen, J. G. Lu, R. P. Zhao, X. M. Cao, P. C. Li, Z. Lv and X. C. Wei, Int. Immunopharmacol., 2018, 65, 212–220 CrossRef CAS PubMed.
- E. Singla, V. Dharwal and A. S. Naura, Inflamm. Res., 2020, 69, 423–434 CrossRef CAS PubMed.
- J. Ablin, O. Shalev, E. Okon, F. Karmeli and D. Rachmilewitz, Inflamm. Bowel Dis., 1999, 5, 253–261 CrossRef CAS PubMed.
- T. T. Sun, R. Eichner, W. G. Nelson, S. C. Tseng, R. A. Weiss, M. Jarvinen and J. Woodcock-Mitchell, J. Invest. Dermatol., 1983, 81, 109–115 CrossRef PubMed.
- X. Zhang, M. Yin and L. J. Zhang, Cells, 2019, 8, E807 CrossRef PubMed.
- D. Ghosh, L. Ding, U. Sivaprasad, E. Geh, J. Biagini Myers, J. A. Bernstein, G. K. Khurana Hershey and T. B. Mersha, PLoS One, 2015, 10, e0144316 CrossRef PubMed.
- J. Ofaiche, S. Duchatelet, S. Fraitag, A. Nassif, J. Nougué and A. Hovnanian, Br. J. Dermatol., 2014, 171, 1565–1567 CrossRef CAS PubMed.
- P. Morais, L. Peralta, M. Loureiro and S. Coelho, Acta Dermatovenerol. Croat., 2013, 21, 48–51 Search PubMed.
- Y. Feng, D. Ji, Y. Huang, B. Ji, Y. Zhang, J. Li, W. Peng, C. Zhang, D. Zhang, Y. Sun and Z. Xu, Oncol. Rep., 2020, 43, 864–876 Search PubMed.
- P. Bognar, I. Nemeth, B. Mayer, D. Haluszka, N. Wikonkal, E. Ostorhazi, S. John, M. Paulsson, N. Smyth, M. Pasztoi, E. I. Buzas, R. Szipocs, A. Kolonics, E. Temesvari and S. Karpati, J. Invest. Dermatol., 2014, 134, 105–111 CrossRef CAS PubMed.
- H. X. Yu, X. L. Wang, L. N. Zhang, J. Zhang and W. Zhao, Cell. Physiol. Biochem., 2018, 51, 1645–1657 CrossRef CAS PubMed.
- D. Impellizzeri, R. Siracusa, M. Cordaro, A. F. Peritore, E. Gugliandolo, G. Mancuso, A. Midiri, R. Di Paola and S. Cuzzocrea, Biochem. Pharmacol., 2018, 155, 150–161 CrossRef CAS PubMed.
- Y. He, H. Hara and G. Núñez, Trends Biochem. Sci., 2016, 41, 1012–1021 CrossRef CAS PubMed.
- A. J. Alonso-Castro, E. Ortiz-Sánchez, A. García-Regalado, G. Ruiz, J. M. Núñez-Martínez, I. González-Sánchez, V. Quintanar-Jurado, E. Morales-Sánchez, F. Dominguez, G. López-Toledo, M. A. Cerbón and A. García-Carrancá, J. Ethnopharmacol., 2013, 145, 476–489 CrossRef CAS PubMed.
- M. Del Carmen Juárez-Vázquez, A. Josabad Alonso-Castro and A. García-Carrancá, J. Ethnopharmacol., 2013, 148, 337–340 CrossRef PubMed.
- F. E. Fox, Z. Niu, A. Tobia and A. H. Rook, J. Invest. Dermatol., 1998, 111, 327–332 CrossRef CAS PubMed.
- P. Bonaventura, A. Lamboux, F. Albarède and P. Miossec, PLoS One, 2018, 13, e0196285 CrossRef PubMed.
- C. H. Jang, J. H. Choi, M. S. Byun and D. M. Jue, Rheumatology, 2006, 45, 703–710 CrossRef CAS PubMed.
- W. T. Chang, W. C. Huang and C. J. Liou, Food Chem., 2012, 134, 972–979 CrossRef CAS PubMed.
- K. Morikawa, M. Nonaka, M. Narahara, I. Torii, K. Kawaguchi, T. Yoshikawa, Y. Kumazawa and S. Morikawa, Life Sci., 2003, 74, 709–721 CrossRef CAS PubMed.
- Y. Z. Wang, X. X. Ou and Y. Zheng, Chin. Tradit. Herb. Drugs, 20019, 40, 1659–1663 Search PubMed.
- Y. Liu, S. Y. Jin, J. Chang, P. Wang, C. Q. Liu, Q. Q. Yin, T. Z. Gao, Q. Zhu and F. S. Lu, J. Anhui Agric. Sci., 2017, 45, 123–125 Search PubMed.
- X. Tang, W. Fang, X. L. Shen, Z. F. Fang and Y. F. Guan, Food Sci., 2014, 35, 111–115 CAS.
Footnote |
† These authors contributed equally. |
|
This journal is © The Royal Society of Chemistry 2020 |