DOI:
10.1039/D0RA03258A
(Paper)
RSC Adv., 2020,
10, 27259-27265
Co-cultured Lepista sordida and Pholiota nameko polysaccharide-iron(III) chelates exhibit good antioxidant activity†
Received
11th April 2020
, Accepted 18th May 2020
First published on 22nd July 2020
Abstract
In the present study, the structural characteristics and antioxidant activities of polysaccharide from the co-cultured Lepista sordida and Pholiota nameko and its polysaccharide-iron(III) chelates were determined. Two polysaccharide fractions named CP-1 and CP-3 were isolated previously from polysaccharide of the fermentation liquid of the co-cultured Lepista sordida and Pholiota nameko. And their chemical structures were measured by FT-IR infrared spectroscopy, TG analysis, X-ray diffraction and 1H NMR spectroscopy. The results suggested that polysaccharides were chelated with iron(III) by –OH and –COOH groups, forming a stable structure of β-FeOOH and improving crystallinity. Furthermore, the antioxidant activities of polysaccharide-iron(III) chelates exhibited stronger hydroxyl radical and superoxide radical scavenging activity than the polysaccharides. Therefore, the polysaccharide-iron(III) chelates could be used as a potential iron supplement.
1. Introduction
Metal ions are believed to play important physiological functions and participate in life activities which include transmitting bioelectronics, regulating biochemical reactions, and enzyme active centers for human. Iron is the essential mineral of the core of hemoglobin and myoglobin, participating in the transportation of oxygen, maintaining normal levels of cellular oxidative metabolism and also communicating in enzyme reaction to improve enzyme activity.1,2 It is reported by the World Health Organization (WHO) that about one half of children aged five to fourteen and pregnant women were anemic, which is caused by iron deficiency.3,4 Therefore, external iron supplementation is an important means to treat and prevent iron deficiency anemia.5,6
Polysaccharides are an active metabolite of fungi fruiting bodies, fermentation liquids and mycelia, exhibiting structure–activity relationship between the chemical structure and biological activity. And Zhang et al.7 have reported the iron content of polysaccharide-iron(III) chelates is also related to the structure and molecular weight of polysaccharides and the reaction conditions such as mass ratio, pH, time and temperature.8 Studies have shown that polysaccharide-iron(III) chelates of larger molecular weight polysaccharide contained higher iron content.9 As a third-generation iron supplement, polysaccharide-iron(III) chelates could be absorbed and utilized to avoid irritation to the gastrointestinal tract after being reduced by reducing substances to divalent iron in the body. Also it exhibited high bioavailability, stability, solubility and reducing ability comparing with traditional iron supplement.10–12 It is reported that polysaccharide-iron(III) chelates can directly or indirectly reduce the excessive production of free radicals theoretically and thus reduce oxidative stress, increasing the activity of antioxidant enzymes and the resistance to oxidation.13
At present, most studies involved the use of polysaccharide-iron chelates such as Fructus mori L. polysaccharide-iron chelates7 and Flammulina velutipes polysaccharide-iron chelates,14 and little on the structure and antioxidant activity of polysaccharide from the co-cultured Lepista sordida and Pholiota nameko and its polysaccharide-iron(III) chelates were known. In this study, the changes of structural characteristics and antioxidant properties of CP-1, CP-3 and their polysaccharide-iron(III) chelates were compared and discussed. The present work aimed to look for a promising candidates for organic iron supplements with good antioxidant activity.
2. Materials and methods
2.1 Materials and chemicals
Previously, three polysaccharide fractions (CP-1, CP-2 and CP-3) were obtained by purifying the polysaccharide from the fermentation liquid co-cultured by Lepista sordida and Pholiota nameko. Among them, CP-1 and CP-3 exhibited better antioxidant activity than CP-2. Polysaccharides, referred to as CP-1, CP-3 were prepared of polysaccharide iron chelate.
The 1,1-diphenyl-2-picrylhydrazyl (DPPH) radical was purchased from Solarbio Technology Co. LTD. CP-1 and CP-3 was prepared from Qingdao Agricultural University. All other chemical reagents were analytical grade.
2.2 Preparation of polysaccharide-iron(III) chelates
The polysaccharide-iron(III) was chelated following the method of Jia et al.15 Briefly, the solution which CP-1 or CP-3 at 1
:
1 (m/m) with trisodium citrate dissolved in distilled water was placed in a water bath (Jinghong DK-S24, Shanghai) at 70 °C for 10 min. 2 M FeCl3·6H2O was added dropwise to the solution with continuous stirring until the reddish brown deposit was appeared, adjusting pH 7.0 to 8.0 with 2.0 M HCl or 2.0 M NaOH at 70 °C for 2 h. The mixtures were centrifuged at 5000 rpm for 10 min. The supernatant was further dialysed, precipitated with anhydrous ethanol and lyophilized to obtain the polysaccharide-iron(III) chelates named CP-1-Fe and CP-3-Fe. The iron contents of CP-1-Fe and CP-3-Fe were determined by phenanthroline colorimetry with ammonium ferrous sulfate standard solution.
2.3 FT-IR spectrum analysis
The structure of CP-1, CP-3, CP-1-Fe and CP-3-Fe was determined by FT-IR (Nicolet iS10, USA). The infrared spectrum was saved with a coaddition of 32 scans at wavelength ranged from 4000–400 cm−1.
2.4 TG analysis
The TG analysis of CP-1, CP-3, CP-1-Fe and CP-3-Fe (4.00–5.00 mg) were carried out in 20–800 °C with a heating rate of 10 °C min−1 using a thermogravimetric analyzer (METTLER TOLEDO, USA). And the TG and DTG curve were recorded.
2.5 XRD analysis
The crystallinity of CP-1, CP-3, CP-1-Fe and CP-3-Fe was performed by using an X-ray diffractometer (Bruker D8ADVANCE, Swit.), and the XRD pattern was recorded with a scanning speed of 5° min−1 from 5° to 50°.
2.6 1H NMR analysis
The 1H NMR spectra of CP-1, CP-3, CP-1-Fe and CP-3-Fe (20.00 mg) were recorded by a Bruker Advance III 500 MHz instrument (Bruker AVIII500, Swit.). CP-1, CP-3, CP-1-Fe and CP-3-Fe (20 mg) were dissolved in D2O, freeze-dried three times and then placed in a NMR tube at 400 MHz at room temperature.
2.7 Antioxidant activity analysis
2.7.1 Hydroxyl radical (·OH) scavenging assay. The scavenging ability on hydroxyl radical was determined according to Gao et al.16 The reaction mixture containing 1.0 mL of sample or ascorbic acid at different concentrations (0–4.0 mg mL−1), 0.1 mL iron vitriol (9 mM), 0.1 mL salicylic acid ethanolic solution (9 mM) and 0.1 mL H2O2 (0.03%, v/v) was incubated at 37 °C for 1 h. Then the absorbance was determined at 510 nm. The hydroxyl radical scavenging capability was calculated as follows:
where A0 is the absorbance of water, iron vitriol, salicylic acid ethanolic solution and H2O2, A1 is the absorbance of the sample, iron vitriol, salicylic acid ethanolic solution and H2O2, A2 is the absorbance of the sample, iron vitriol, salicylic acid ethanolic solution and distilled water instead of H2O2, respectively.
2.7.2 Superoxide radical scavenging assay. The scavenging ability on superoxide radical was determined following the method of Chen and Huang17 with slight modifications. Briefly, 4.5 mL of Tris–HCl buffer (pH 8.2, 50 mM) was incubated at 25 °C for 20 min. Then 1 mL of sample or ascorbic acid at various concentrations (0–4.0 mg mL−1) and pyrogallol (0.4 mL, 25 mM) were mixed. The mixtures were reacted at 25 °C for 5 min. The absorbance was measured at 325 nm by adding HCl (1 mL, 0.2 M) to terminate the reaction. The superoxide DPPH radical scavenging capability was calculated as follows:
where A0 is the absorbance of distilled water, Tris–HCl and pyrogallol, A1 is the absorbance of Tris–HCl, the sample and pyrogallol, A2 is the absorbance of Tris–HCl, the sample and distilled water instead of pyrogallol, respectively.
2.7.3 DPPH radical scavenging assay. The scavenging ability on DPPH radical was determined using a previously reported method.18 Briefly, 2 mL sample or ascorbic acid at different concentrations (0–2.0 mg mL−1) was mixed with DPPH–ethanol solution (2 mL, 0.1 mM). The mixture was incubated at room temperature in dark for 30 min and absorbance was measured at 517 nm. The sample was displaced by VC as the positive control. The DPPH radical scavenging capability was calculated as follows:
where A0 is the absorbance of water and DPPH solution, A1 is the absorbance of the sample and DPPH solution, A2 is the absorbance of the sample and ethanolic, respectively.
2.7.4 Determination of reducing power. Reducing power of sample was carried out according to Zhang et al.19 1.5 mL of sample or ascorbic acid at varying concentrations (0–4.0 mg mL−1) were mixed with 1.5 mL of phosphate buffer (0.2 M, pH 6.6) and 1.5 mL of potassium ferricyanide (1%, w/v). The reaction mixture was stood at 50 °C for 20 min and cooled down. Subsequently, 1.5 mL trifluoroacetic acid (10%, w/v) was added to terminate reaction and then mixed with 1.2 mL of ferric chloride (0.1%, w/v). The absorbance at 700 nm was measured. VC was used as the positive control.
2.8 Statistical analysis
The data were shown as mean value ± standard deviations (n ≥ 3) and differences between experimental groups were performed by one-way analysis of variance (SPSS 16.0). A value of p < 0.05 was considered to be statistically significant.
3. Results
3.1 Iron(III) chelating capacity
The iron chelating ability of polysaccharide depend on functional groups of polysaccharides and the reaction conditions, such as pH.7,8 As shown in Fig. 1, the iron contents of CP-1-Fe and CP-3-Fe were 2.63 ± 0.03% and 2.49 ± 0.15%, respectively (p > 0.05).
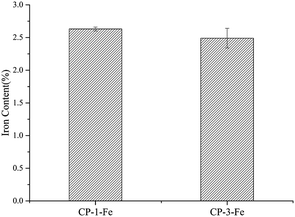 |
| Fig. 1 Iron contents of CP-1-Fe and CP-3-Fe. | |
3.2 FT-IR spectroscopy
The FT-IR spectrum identify the changes of characteristic functional groups and chemical structures after polysaccharides were transformed to polysaccharide-iron(III) chelates. The FT-IR spectrum of CP-1, CP-3 and their polysaccharide-iron(III) chelates ranging from 400 to 4000 cm−1 were shown in Fig. 2. The range of 3200 to 3600 cm−1, 2800 to 3000 cm−1 and 1400 to 1200 cm−1 were the characteristic absorption peaks of polysaccharide.20 The typical absorption band at 3300 cm−1 is related to O–H stretching vibration. And the characteristic absorption bands at 2900 and 1400 cm−1 were due to the stretching vibration and variable-angle vibration of C–H. Furthermore, the absorption peak around 1600 cm−1 was attribute to the stretching vibration of symmetric and asymmetric C
O bonds.20,21 As we can see from Fig. 2, the absorption band at 3300 cm−1 changed slightly after chelation mainly due to the participation of hydroxyl groups during the chelation process.22 After chelation modification, the absorption peak at around 1600 cm−1 shifted to a low wave number and the peak intensity increased, indicating C
O of the polysaccharide may involved in the chelation reaction and the peak intensity increased.23 400 to 1000 cm−1 were characteristic peaks of iron core structure “β-FeOOH” of polysaccharide-iron(III) chelates.24 In our study, the weak absorption peak of CP-1-Fe and CP-3-Fe at 450 and 850 cm−1 were observed, respectively. The absorption peak at around 1060 cm−1 in polysaccharide and polysaccharide-iron(III) was indicated the existence of a pyran-ring structure.25 As shown in Fig. 2(a), the peak at 879.5 cm−1 was speculated that the CP-1 was connected by β-glycosidic bonds.26
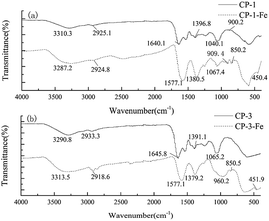 |
| Fig. 2 FT-IR spectrum of CP-1 (a), CP-1-Fe (a), CP-3 (b) and CP-3-Fe (b). | |
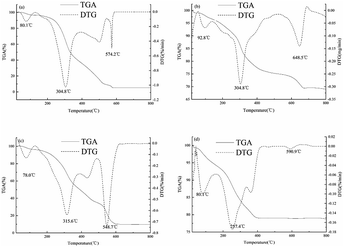 |
| Fig. 3 TG and DTG curves of CP-1 (a), CP-1-Fe (b), CP-3 (c) and CP-3-Fe (d). | |
3.3 TG analysis
The change of weight of polysaccharides and polysaccharide-iron(III) chelates with temperature was determined by TG and DTG. The thermal stabilities of CP-1, CP-1-Fe, CP-3 and CP-3-Fe were investigated by TG from 20 °C to 800 °C were shown in Fig. 3. The thermal decomposition are divided into three stages and the materials with small molecular weight are decomposed first. It can be seen from Fig. 3(a) and (b), the weight loss of CP-1 was 3.84% from 20 °C to 130 °C, due to evaporation of the free water. CP-1 decomposed drastically with the average rate of weight loss of 0.21% min−1 from 130 °C to 588 °C and the rate of mass loss reached 1.02% min−1 at 304.8 °C. It has indicated that the chemical bond of CP-1 was destruction and the polysaccharide chelate has been decomposed.27 The rates of weight loss of CP-1 gradually showed stable tendency after the temperatures reached to 588 °C. Comparable to that of CP-1, the weight loss of CP-1-Fe was 3.45% from 20 °C to 138 °C, due to evaporation of the free water. In the second stage, the average rate of weight loss of 0.051% min−1 from 138 °C to 690 °C and the rate of mass loss of reached 0.29% min−1 at 304.8 °C. The wide interval between the two peaks and the residual mass indicates that CP-1-Fe had stronger thermal stability and more stable structure which could be consistent with previous study.15,16 As shown in Fig. 3(c) and (d), the mass loss of CP-3 was 3.73% caused by water from 20 °C to 130 °C. The average rate of weight loss was 0.18% min−1 from 130 °C to 620 °C. At 315.6 °C, the rate of mass loss was 0.63%/min. The weight loss of CP-3-Fe was 5.63% from 20 °C to 151 °C, due to evaporation of the free water. In the second stage, the average rate of weight loss of CP-3-Fe was 0.026% min−1 from 151 °C to 644 °C. At 257.4 °C, the rate of mass loss was 0.17% min−1. The results showed the CP-3-Fe possessed higher stability from 20 °C to 275 °C compared with CP-3.
3.4 XRD analysis
XRD is an method through crystalline substance which has specific chemical composition and structural parameters, including lattice type, unit cell size, number of particles in the unit cell and coordinates to genera specific diffraction pattern, correspond to a series of specific interplanar distances and relative intensity values. As shown in Fig. 4, the XRD curve were similar, implying that chelation was not change the basic skeleton of the polysaccharide structure. The peak shape changed at around 25° owing to the introduction of iron(III) weakened the interaction of hydrogen bonds, increased the stereochemical steric hindrance and reduced the group concentration.28 Some new peaks appeared for CP-1-Fe at 14.82° and 38.16° and for CP-3-Fe at 14.82° and 36.78° after chelation, which indicated that structure changes induced with iron chelating of CP-1 and CP-3 to have certain degrees of crystallinity. And it had been reported that the peaks about 15° and 35° were the characteristic peaks of iron appear.17 The results further confirmed that the chelation of CP-1and CP-3 with iron.
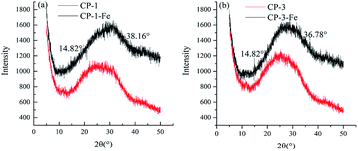 |
| Fig. 4 XRD of CP-1 (a), CP-1-Fe (a), CP-3 (b) and CP-3-Fe (b). | |
3.5 1H NMR analysis
The 1H NMR spectra to analyze the structural feature of CP-1, CP-3 and their iron chelates CP-1-Fe, CP-3-Fe were shown in Fig. 5. A peak at around δH 4.70 for all four substances was the characteristic peak of solvent deuterated water. According to the principle of chemical shifts, the main distribution of the anomeric proton signals at δH 4.0–5.6 ppm can distinguish the type of polysaccharide glycosides. The end-substrate signal mostly at δH 5.0–5.6 ppm of 1H NMR spectrum indicated that the type of polysaccharide glycosides were mainly α-glycoside while the anomeric protons signals mostly at δH 4.0–5.0 ppm arised of β-glycoside.29 CP-1 signal mainly distributed at δH 4.0–5.0 ppm with significant peaks at δH 4.23 ppm and δH 4.59 ppm, consistent with CP-3 at δH 4.12 ppm. The results presumed that CP-1 and CP-3 all contained β-glycosides. The signals at δH 3.80 ppm and δH 3.74 ppm should be assigned to the –O–CH3. The signals at δH 2.02 ppm and δH 2.03 ppm were attribute to acetyl groups.
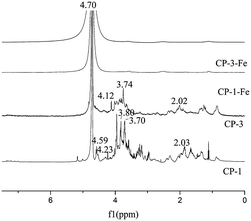 |
| Fig. 5 1H NMR spectra of polysaccharides and polysaccharide-iron(III) chelates. | |
3.6 Antioxidant activity analysis
Hydroxyl radicals regulate the homeostasis in living organisms owing to its strong oxidizing ability. Excess hydroxyl radicals were generated during metabolism, causing biological macromolecules such as cell membranes, proteins and enzymes oxidized and resulting in cell oxidation, aging, and apoptosis.30 As shown in Fig. 6(a), with VC as the positive control, the scavenging activity on hydroxyl radical of CP fractions and CP-iron(III) chelates were in a concentration-dependent manner at concentrations ranging from 0 to 4 mg mL−1. The IC50 values of CP-1-Fe and CP-3-Fe were 0.785 ± 0.030 and 0.740 ± 0.043 mg mL−1, respectively, which were lower than those of CP-1 (IC50, 4.472 ± 0.745 mg mL−1) and CP-3 (IC50, 4.333 ± 0.298 mg mL−1). In the range of 0 to 4 mg mL−1, the scavenging activity on hydroxyl radical of CP-1-Fe was significantly better than that of CP-1 (p < 0.05). In the range of 3.2 to 4 mg mL−1, the scavenging rate of CP-1-Fe was close to VC. When the concentration at 3.2 mg mL−1, the hydroxyl radical scavenging activities was 99.24%, and 130.04% higher than the same concentration of CP-1 (p < 0.05). CP-3-Fe exhibited a significantly better scavenging rate for hydroxyl radicals than CP-3 in the ranged from 0 to 4 mg mL−1 (p < 0.05). When the concentration reached 4 mg mL−1, the scavenging rate of CP-3-Fe can reach 97.86 ± 0.65%, 367.42% higher compared with the same concentration CP-3 (p < 0.05).
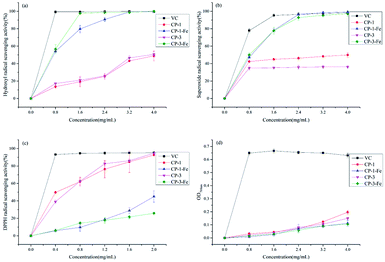 |
| Fig. 6 Scavenging abilities on hydroxyl radical (a), superoxide radical (b), DPPH radical (c) and reducing power (d) of polysaccharides and polysaccharides-iron(III) chelates. | |
The superoxide radicals triggered oxidation by generating more active hydroxyl ions or singlet oxygen closely related to aging and pathology, which provided with good antioxidant activity.7 The scavenging ability on superoxide radical of CP fractions and CP-iron(III) compared with VC as the positive control were shown in Fig. 6(b). The results indicated that CP-1-Fe and CP-3-Fe had scavenging abilities in concentration-dependent manners in the range of 0 to 4 mg mL−1. The scavenging ability of CP-1 and CP-3 were first strengthened and then basically stable. The IC50 of CP-1-Fe and CP-3-Fe were 0.869 ± 0.016 mg mL−1 and 0.821 ± 0.052 mg mL−1, respectively, which were lower than those of CP-1 and CP-3. When the concentration reached 2.4 mg mL−1, the scavenging rates of CP-1-Fe and CP-3-Fe can reach 96.41% and 92.83 ± 2.78%, were 108.45% and 159.37% higher than that of the same concentration CP-1 and CP-3, respectively (p < 0.05). The results showed that the scavenging ability of superoxide radicals were significantly enhanced by inducing with iron.
DPPH radical scavenging assay generally was be used to evaluate the antioxidant activity, which can produce stable nitrogen-centered radicals react with antioxidants.31 The scavenging abilities of CP fractions and CP-iron(III) chelates on DPPH radicals compared with VC as the positive control were shown in Fig. 6(c). It can be seen from Fig. 6(c) that in the range of 0 to 2 mg mL−1, the scavenging activity on DPPH free radical of CP fractions and CP-iron(III) chelates were concentration-dependent. When the concentration reached 0.4 mg mL−1, the scavenging rates of VC reached 93.22 ± 0.47%. The IC50 values of CP-1-Fe and CP-3-Fe were 2.487 ± 0.533 and 5.952 ± 0.561 mg mL−1, respectively; these were significantly higher than those of CP-1 and CP-3, which were 0.457 ± 0.045 and 0.540 ± 0.025 mg mL−1. When the concentration reached 2 mg mL−1, the scavenging rates of CP-1-Fe and CP-3-Fe were lower 51.47% and 72.15% than that of the same concentration CP-1 and CP-3, respectively (p < 0.05). The results indicated chelation with iron decreased the scavenging activity on DPPH radical.
The substance with strong reducing power can donate more electrons, which causing the scavenging radicals. Therefore, reducing power is the method to evaluate the antioxidant activity.14 The reducing power of CP fractions and CP-iron(III) chelates were shown in Fig. 6(d). It can be seen from Fig. 6(d) the solubility dependence at a given concentration. Comparing with VC as positive control, the reducing power of all four complexes were low. The total reducing power of CP-1, CP-1-Fe, CP-3, CP-3-Fe were relatively close ranging from 0 to 3.2 mg mL−1. When the concentration exceeded 3.2 mg mL−1, the total reducing power of CP-1 and CP-3 were higher than that of CP-1-Fe and CP-3-Fe, respectively. When the concentration reached 4 mg mL−1, the reducing power of the chelated polysaccharides CP-1-Fe was 43.15% lower than those of CP-1, and CP-3-Fe was 29.33% lower than those of CP-3, respectively.
4. Discussion
Polysaccharide-iron chelates were used as an iron supplement, playing a role in anti-oxidation, regulating immunity, anti-virus and other physiological activities after being absorbed by the body.32–34 Gao et al.35 have reported that the sulfated polysaccharide isolated from marine green algae Ulva pertusa and its iron(III) complex exhibited hematopoietic capacity due to the supplement of iron and enhancing the immunity of body. Shi et al.22 have proved that the anti-radiation and anti-oxidative activity of Ulva pertusa polysaccharides-iron(III) complex. Therefore, it is necessary to modify polysaccharides by iron chelation.
Cells produce a series of reactive oxygen species during metabolism and growth, such as hydroxyl radical, superoxide radical and hydrogen peroxide, which destroy proteins and nucleic acids in the body, leading to disease. Chen and Huang36 have studied the antioxidant effects of garlic polysaccharide (PS) and polysaccharide-Fe(III) complex (FePS) by co-heating method and found that the scavenging ability on hydroxyl radical and superoxide radical of FePS was higher than PS, showing synergy effect. Dong et al.14 have investigate the iron chelation of Flammulina velutipes polysaccharide (FVP) and its purified component (FVP2) on quenching superoxide radical improved. These consisted with the findings of this study. In this study, the antioxidant analysis found that CP-1-Fe and CP-3-Fe had significantly higher scavenging capacity on hydroxyl radicals and superoxide radical than CP-1 and CP-3, indicating the antioxidant activities improved after chelation.
The reaction mechanism of DPPH free radicals to evaluate the antioxidant capacity can be divided into reactions based on H-atom transfer (HAT) and reactions based on electron transfer (ET).37 When the radical scavenger occur, the scavenger can directly supply hydrogen atoms to the DPPH radical, forming a non-radical form of the DPPH-H (HAT reaction); or transferring electrons from the scavenger to the DPPH radical (ET reaction). When DPPH captures an electron paired with a free electron, the dark purple DPPH radical is reduced to a yellow non-radical form of DPPH-H and the degree of discoloration is directly proportional to the number of electrons accepted.38 Dong et al.14 also have studied DPPH radical scavenging activities of Flammulina velutipes polysaccharides (FVP) and polysaccharides fraction (FVP2) and their polysaccharide-iron chelates, and resulted shown that while DPPH radical scavenging activities of FVP, FVP2, FVP-Fe, FVP2-Fe were 44.32%, 43.78%, 20.00%, 25.14%, respectively. FVP-Fe and FVP2-Fe had lower DPPH free radical scavenging effects than FVP and FVP2. The study also found that CP-1-Fe and CP-3-Fe had lower scavenging ability on DPPH free radicals than CP-1 and CP-3. A stable “β-FeOOH” iron core structure was formed in chelating, which weakened the hydrogen supply ability and pair with DPPH free radicals, and reduced the degree of discoloration, leading to an increase in the absorbance at 517 nm to lower the scavenging ability on DPPH radical.
In this study, compared with the CP-1 and CP-3, the signals for CP-1-Fe and CP-3-Fe were disappeared except for the water peak at δH 4.72 ppm, which was in accordance with the study reported by Domracheva et al.39 This may be due to metallic iron with paramagnetism, which interfere with the magnetic environment around the ligand. The presence of this region of the polysaccharide ligand caused the proton signal to be amplified and the relaxation time becomes longer to broaden the spectral peak, forming an apparently invisible iron core blind zone causing the peaks in this area to disappear.40
5. Conclusions
In this study, the polysaccharides, CP-1 and CP-3, were chelated with iron(III) by –OH and C
O groups to form a stable structure of β-FeOOH and improve crystallinity. The antioxidant activities assay showed that polysaccharide-iron(III) chelates, CP-1-Fe and CP-3-Fe exhibited antioxidant activities. Particularly, CP-1-Fe and CP-3-Fe showed stronger hydroxide radical and superoxide radical scavenging ability than CP-1, CP-3. The CP-1-Fe and CP-3-Fe has potential to be used as an iron supplement for the treatment of iron deficiency anemia (IDA).
Conflicts of interest
All authors declare that there is no conflict of interest.
Acknowledgements
The authors would like to thank the Shandong Province Modern Agricultural Industry Technology System [SDAIT-07-07] of China for supporting this research.
References
- M. M. Tang, D. F. Wang, Y. F. Hou, P. Buchili and L. P. Sun, Preparation, characterization, bioavailability in vitro and in vivo of tea polysaccharides-iron complex, Eur. Food Res. Technol., 2012, 236, 341–350 CrossRef.
- V. Corce, S. G. Gouin, S. Renaud, F. Gaboriau and D. Deniaud, Recent advances in cancer treatment by iron chelators, Bioorg. Med. Chem. Lett., 2016, 26, 251–256 CrossRef CAS PubMed.
- L. Xu, Y. B. Meng, Y. Liu, Q. H. Meng, Z. D. Zhang, J. Li and Q. Lu, A novel iron supplements preparation from Grifola frondosa polysaccharide and assessment of antioxidant, lymphocyte proliferation and complement fixing activities, Int. J. Biol. Macromol., 2018, 108, 1148–1157 CrossRef CAS PubMed.
- S. C. Cottin, L. Gambling, H. E. Hayes, V. J. Stevens and H. J. McArdle, Pregnancy and maternal iron deficiency stimulate hepatic CRBPII expression in rats, J. Nutr. Biochem., 2016, 32, 55–63 CrossRef CAS PubMed.
- F. Ren, X. H. Qian and X. L. Qian, Astragalus polysaccharide upregulates hepcidin & reduces iron overload in mice via activation of p38 mitogen-activated protein kinase, Biochem. Biophys. Res. Commun., 2016, 472, 163–168 CrossRef CAS PubMed.
- J. Wang, H. X. Chen, Y. W. Wang and L. S. Xing, Synthesis and characterization of a new Inonotus obliquus polysaccharide-iron(III) complex, Int. J. Biol. Macromol., 2015, 75, 210–217 CrossRef CAS PubMed.
- J. Q. Zhang, C. Chen and X. Fu, Fructus mori L. polysaccharide-iron chelates formed by self-embedding with iron(iii) as the core exhibit good antioxidant activity, Food Funct., 2019, 10, 3150–3160 RSC.
- Y. Z. Chi, Y. P. Li, G. L. Zhang, Y. Q. Gao, H. Ye, J. Gao and P. Wang, Effect of extraction techniques on properties of polysaccharides from Enteromorpha prolifera and their applicability in iron chelation, Carbohydr. Polym., 2018, 181, 616–623 CrossRef CAS PubMed.
- C. Jiang, Q. Xiong, D. Gan, Y. Jiao, J. Liu, L. Ma and X. Zeng, Antioxidant activity and potential hepatoprotective effect of polysaccharides from Cyclina sinensis, Carbohydr. Polym., 2013, 91, 262–268 CrossRef CAS PubMed.
- Q. Lu, L. Xu, Y. B. Meng, Y. Liu, J. Li, Y. G. Zu and M. H. Zhu, Preparation and characterization of a novel Astragalus membranaceus polysaccharide-iron (III) complex, Int. J. Biol. Macromol., 2016, 93, 208–216 CrossRef CAS PubMed.
- R. K. Saini, P. Manoj, N. P. Shetty, K. Srinivasan and P. Giridhar, Dietary iron supplements and Moringa oleifera leaves influence the liver hepcidin messenger RNA expression and biochemical indices of iron status in rats, Nutr. Res., 2014, 34, 630–638 CrossRef CAS PubMed.
- F. E. Viteri, E. Casanueva, M. C. Tolentino, J. Diaz-Frances and A. B. Erazo, Antenatal iron supplements consumed daily produce oxidative stress in contrast to weekly supplementation in Mexican non-anemic women, Reprod. Toxicol., 2012, 34, 125–132 CrossRef CAS PubMed.
- H. Xian, P. Wang, H. Jing, G. Q. Chen, D. F. Cheng, F. Ji, S. Song and L. Zhang, Comparative study of components and anti-oxidative effects between sulfated polysaccharide and its iron complex, Int. J. Biol. Macromol., 2018, 118, 1303–1309 CrossRef CAS PubMed.
- Y. R. Dong, S. J. Cheng, G. H. Qi, Z. P. Yang, S. Y. Yin and G. T. Chen, Antimicrobial and antioxidant activities of Flammulina velutipes polysaccharides and polysaccharide-iron(III) complex [corrected], Carbohydr. Polym., 2017, 161, 26–32 CrossRef CAS PubMed.
- N. Jia, H. R. Qiao, W. Zhu, M. H. Zhu, Q. H. Meng, Q. Lu and Y. G. Zu, Antioxidant, immunomodulatory, oxidative stress inhibitory and iron supplementation effect of Astragalus membranaceus polysaccharide-iron (III) complex on iron-deficiency anemia mouse model, Int. J. Biol. Macromol., 2019, 132, 213–221 CrossRef CAS PubMed.
- C. G. Gao, Y. H. Wang, C. Y. Wang and Z. Y. Wang, Antioxidant and immunological activity in vitro of polysaccharides from Gomphidius rutilus mycelium, Carbohydr. Polym., 2013, 92, 2187–2192 CrossRef CAS PubMed.
- J. F. Chen and G. L. Huang, Antioxidant activities of garlic polysaccharide and its phosphorylated derivative, Int. J. Biol. Macromol., 2019, 125, 432–435 CrossRef CAS PubMed.
- S. Yarnpakdee, S. Benjakul and T. Senphan, Antioxidant Activity of the Extracts from Freshwater Macroalgae (Cladophora glomerata) Grown in Northern Thailand and Its Preventive Effect against Lipid Oxidation of Refrigerated Eastern Little Tuna Slice, Turk. J. Fish. Aquat. Sci., 2019, 19, 209–219 Search PubMed.
- C. Zhang, S. S. Li, J. J. Zhang, C. L. Hu, G. Che, M. Zhou and L. Jia, Antioxidant and hepatoprotective activities of intracellular polysaccharide from Pleurotus eryngii SI-04, Int. J. Biol. Macromol., 2016, 91, 568–577 CrossRef CAS PubMed.
- W. S. Wang, X. L. Song, Z. Gao, H. J. Zhao, X. Wang, M. Liu and L. Jia, Anti-hyperlipidemic, antioxidant and organic protection effects of acidic-extractable polysaccharides from Dictyophora indusiata, Int. J. Biol. Macromol., 2019, 129, 281–292 CrossRef CAS PubMed.
- H. Gao, W. C. Zhang, B. S. Wang, A. L. Hui, B. Du, T. T. Wang, L. Meng, H. X. Bian and Z. Y. Wu, Purification, characterization and anti-fatigue activity of polysaccharide fractions from okra (Abelmoschus esculentus (L.) Moench), Food Funct., 2018, 9, 1088–1101 RSC.
- J. M. Shi, C. L. Cheng, H. Zhao, J. T. Jing, N. Gong and W. H. Lu, In vivo anti-radiation activities of the Ulva pertusa polysaccharides and polysaccharide-iron(III) complex, Int. J. Biol. Macromol., 2013, 60, 341–346 CrossRef CAS PubMed.
- J. F. Cui, Y. P. Li, P. Yu, Q. P. Zhan, J. F. Wang, Y. Z. Chi and P. Wang, A novel low molecular weight Enteromorpha polysaccharide-iron (III) complex and its effect on rats with iron deficiency anemia (IDA), Int. J. Biol. Macromol., 2018, 108, 412–418 CrossRef CAS PubMed.
- H. He, F. P. An, H. Teng, Q. Huang and H. B. Song, Preparation and characterisation of a novel agar oligosaccharide-iron (III) complex, Int. J. Food Sci. Technol., 2019, 54, 170–182 CrossRef CAS.
- Y. L. Zhang, X. Y. Lu, Z. B. Fu, Z. B. Wang and J. B. Zhang, Sulphated modification of a polysaccharide obtained from fresh persimmon (Diospyros kaki L.) fruit and antioxidant activities of the sulphated derivatives, Food Chem., 2011, 127, 1084–1090 CrossRef CAS PubMed.
- M. Kozarski, A. Klaus, M. Niksic, D. Jakovljevic, J. P. F. G. Helsper and L. J. L. D. Van Griensven, Antioxidative and immunomodulating activities of polysaccharide extracts of the medicinal mushrooms Agaricus bisporus, Agaricus brasiliensis, Ganoderma lucidum and Phellinus linteus, Food Chem., 2011, 129, 1667–1675 CrossRef CAS.
- L. Wang, C. Li, Q. Huang and X. Fu, Biofunctionalization of selenium nanoparticles with a polysaccharide from Rosa roxburghii fruit and their protective effect against H2O2-induced apoptosis in INS-1 cells, Food Funct., 2019, 10, 539–553 RSC.
- N. K. Lazaridis, G. Z. Kyzas, A. A. Vassiliou and D. N. Bikiaris, Chitosan derivatives as biosorbents for basic dyes, Langmuir, 2007, 23, 7634–7643 CrossRef CAS PubMed.
- X. Q. Xu, J. Li and Y. Hu, Polysaccharides from Inonotus obliquus sclerotia and cultured mycelia stimulate cytokine production of human peripheral blood mononuclear cells in vitro and their chemical characterization, Int. Immunopharmacol., 2014, 212, 69–78 Search PubMed.
- H. Dong, X. C. Yang, J. P. He, S. Cai, K. J. Xiao and L. Zhu, Enhanced antioxidant activity, antibacterial activity and hypoglycemic effect of luteolin by complexation with manganese(ii) and its inhibition kinetics on xanthine oxidase, RSC Adv., 2017, 7, 53385–53395 RSC.
- C. D. Porto, S. Calligaris, E. Celotti and M. C. Nicoli, Antiradical properties of commercial cognacs assessed by the DPPH test, J. Agric. Food Chem., 2000, 48, 4241–4245 CrossRef PubMed.
- W. Y. Zhao, B. Z. Sun, M. Y. Chen, Y. F. Wang and W. Du, Synthesis of rose roxburghii tratt polysaccharide-Fe(III) complex and determination of Fe, Liaoning J. Tradit. Chin. Med., 2012, 39, 2256–2257 CAS.
- M. Wang, F. N. Guo, W. J. Na, Z. J. Liu, X. Y. Hou and L. M. Jin, Antioxidant activity of lycium barbarum polysaccharide-Fe complex, The Food Industry, 2016, 37, 143–145 Search PubMed.
- L. Chen and G. L. Huang, The antiviral activity of polysaccharides and their derivatives, Int. J. Biol. Macromol., 2018, 115, 77–82 CrossRef CAS PubMed.
- X. Gao, H. Qu, Z. L. Gao, D. Y. Zeng, J. P. Wang, D. Baranenko, Y. Z. Li and W. H. Lu, Protective effects of Ulva pertusa polysaccharide and polysaccharideiron (III) complex on cyclophosphamide induced immunosuppression in mice, Int. J. Biol. Macromol., 2019, 133, 911–919 CrossRef CAS PubMed.
- X. Chen and G. L. Huang, Synthesis and antioxidant activities of garlic polysaccharide-Fe(III) complex, Int. J. Biol. Macromol., 2020, 145, 813–818 CrossRef CAS PubMed.
- S. Kordali, A. Cakir, A. Mavi, H. Kilic and A. Yildirim, Screening of chemical composition and antifungal and antioxidant activities of the essential oils from three Turkish Artemisia species, J. Agric. Food Chem., 2005, 53, 1408–1416 CrossRef CAS PubMed.
- A. Kumaran and R. Joel karunakaran, Antioxidant & free radical scavenging activity of an aqueous extract of Coleus aromaticus, Food Chem., 2006, 97, 109–114 CrossRef CAS.
- N. E. Domracheva, V. E. Vorobeva, A. V. Pyataev and A. G. Ivanova, Magnetic Properties of Novel Dendrimeric Iron(III) Complexes of the First Generation: EPR and Mössbauer Study, Appl. Magn. Reson., 2016, 47, 903–913 CrossRef CAS.
- I. Bertini, C. Luchinat, G. Parigi and R. Pierattelli, NMR spectroscopy of paramagnetic metalloproteins, J. Biol. Chem., 2005, 6, 1536–1549 CAS.
Footnote |
† Electronic supplementary information (ESI) available. See DOI: 10.1039/d0ra03258a |
|
This journal is © The Royal Society of Chemistry 2020 |