DOI:
10.1039/D0RA02810J
(Review Article)
RSC Adv., 2020,
10, 25339-25351
Prospects of kefiran as a food-derived biopolymer for agri-food and biomedical applications
Received
27th March 2020
, Accepted 26th June 2020
First published on 3rd July 2020
Abstract
There is a huge demand for food-derived polysaccharides in the field of materials research due to the increasing concerns posed by synthetic biopolymers. The scientific community is extensively searching for other natural, food-derived or bio-inspired polymers that possess promising potentials and advantageous properties that can be promptly utilized for multifarious applications. Kefiran, a food-derived microbial exopolysaccharide extracted from kefir grains has exhibited evidence of non-toxicity, anti-microbial activity, nutritional value, and other favourable characteristics. This review aims to shed light on the properties of kefiran and provide an overview of its applications in the agri-food and biomedical sectors. The present work also discusses the challenges and prospects that lie ahead for kefiran in finding its place amongst the existing spectrum of natural and biodegradable polymers.
1. Introduction
Kefir is a fermented milk drink that people of Caucasus, since ancient times, have produced from kefir grains. Kefir has become a popular natural probiotic drink with high nutritional value since the 20th century, in Asia, America, and Europe, attributed to its superior nutritional content such as vitamin K2, vitamin B12, magnesium, calcium, enzymes, folate, and probiotics.1 In fact, kefir is sometimes compared to yogurt in terms of its characteristics and benefits, owing to their similar probiotic effects and enhanced lactose digestion.2–4
Kefir grains are structurally soft, gelatinous, water-insoluble, and irregular in shape with a variety of sizes (0.3–3.0 cm in diameter). The origins of kefir grains are varied, ranging from Argentina,5 Brazil,6 Belgium,7 China,8 Ireland,9 Malaysia,10 Russia,11 and Turkey.12 Kefir grains consist of a complex microbial consortium capable of producing metabolites with tremendous health-promoting effects, including hypocholesterolemic, anti-atherogenic, antioxidant, anticolitis, and higher β-galactosidase activity.2 They also offer protection against pathogens due to the synergistic effects of ethanol, lactic acids, acetic acids, bacteriocins, as well as other bioactive compounds and probiotic microorganisms.
Kefir production takes place through controlled milk fermentation of the kefir grains in a protein–polysaccharide matrix.2,13,14 These are rice grain-sized symbiotic microbial associations of different types of bacteria (Lactobacillus, Lactococcus, Leuconostoc, Acetobacter and Streptococcus) and various form of yeasts (Kluyveromyces, Torula, Candida and Saccharomyces),3,15 making them excellent starter culture for fermentation of various food products such as cheese, milk, bread and kefir. The major species found in kefir grains are reported to be Lactobacillus kefiranofaciens.2,16 Since kefir has been consumed by people for centuries, it is considered safe for human health and is classified by the US Food and Drug Administration (FDA) as generally considered as safe (GRAS).17
In 1967, Riviére et al. first isolated an unknown exopolysaccharide called “kefiran” from kefir grains.18 Exopolysaccharides (EPS) are biopolymers secreted by bacteria and fungi forming part of their native environment. Hence, kefiran is classified as a food-derived biopolymer obtained specifically from the food source – kefir grains. Earlier characterization of kefiran puts this natural polymer within a molecular mass range of 1.0 × 104 to 6.0 × 106 Da,19 depending on the type of carbon source used. This range of molecular weight is similar to other natural polysaccharides such as starch (×104 to 107 Da)20,21 and chitosan (1.6–5.6 × 105 Da).22 Together with an assortment of proteins and lipids, kefiran holds the symbiotic cell structure together and protects it against external influences, such as nutrient deficiency, desiccation, bacteriophages, toxicity, and osmotic stress.13,16,23–25 Kefiran degrades into several non-hazardous polysaccharide intermediates and eventually into D-galactose and D-glucose.26 This occurs through a series of slow enzymatic degradation processes that breaks up variable glycosidic linkages between the glucose and galactose units of kefiran, as seen in Fig. 1. Undoubtedly, the consumption of kefiran gives tremendous nutritional values. Therefore, recent research has focused on isolating and extracting kefiran from kefir grains, instead of using kefir grains for food applications, due to their better health benefits offered.
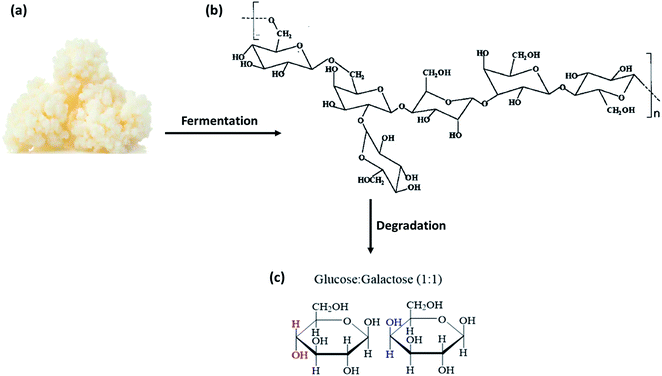 |
| Fig. 1 (a) Kefir grains that can be fermented to produce kefiran. (b) The chair configuration of the proposed molecular structure of kefiran, showing kefiran as a heteropolysaccharide. (c) The chemical structures of its constituent monomers (glucose and galactose) upon enzymatic degradation of kefiran.27 | |
Till date, kefiran remains a prime subject of interest owing to its GRAS status, rheological features and health-benefiting properties, such as antibacterial, antifungal, and antitumoral.17 Whilst there are several reviews reporting on the overall characteristics and advantages of kefir and kefiran for human health,24,27–30 none have discussed in detail on the prospects and challenges that this material encounters in order to be completely accepted into the biopharmaceutical and agri-food sectors. Potential applications of kefiran, specifically for biopharmaceutical drug delivery and other biomedical applications are also discussed. This review therefore aims to provide an overview on the prospects of kefiran as a natural material candidate for food packaging, biopharmaceutical, and other therapeutic purposes.
2. Optimizing and scaling of kefiran production
Kefiran has been traditionally extracted from kefir grains which house conglomerates of complex microflora with milk as the nutritional culture medium.31 Lactobacillus kefiranofaciens has been identified as the dominant microbial species for kefiran production and the role of other strains involved in kefiran production have also been extensively documented in literature.32,33 Several studies to mass produce kefiran from this bacterial strain have been conducted.34,35 However, it was found that during kefiran production, accumulation of lactic acid impacted the growth of Lactobacillus kefiranofaciens. Therefore, in order to improve the production of kefiran, yeast strains (S.cerevisiae amongst others) were introduced into the culture medium due to their inherent property to consume the generated lactic acid. Other conventional methods to eliminate lactic acid include adopting separation systems using membranes or electro-dialyzers, but they are proven to be extremely cost ineffective and complex.36,37 Consequently, co-culture systems were employed whereby specific species of yeast was selected to remove lactic acid, as well as enhance both cell growth and kefiran production.38
The location of yeasts (on surface or interior of the grain), is another parameter that determines their roles in the fermentation process.39 Other factors such as effects of metabolites, shear stress, dissolved oxygen, temperature, availability of phosphate source in the medium, and the effect of stirring were investigated to a relatively lesser extent.40,41 Notwithstanding, the main contributor towards optimizing the production of kefiran is narrowed down to the ideal carbon source.23,42–44 In this regard, the investigated carbon sources are sucrose, glucose, fructose, xylose, and lactose. Out of these, lactose has proven to be the best carbon source for kefiran production, whereas sucrose was an ideal carbon source for xanthan production.34,45,46 It was also demonstrated that increased carbon
:
nitrogen ratio can significantly reduce the total production of kefiran. In addition, inorganic phosphate salts were often added in the medium to improve bacterial growth. Dailin et al.23 optimized the production of kefiran from L. kefiranofaciens in a semi-industrial scale by 58.02% using a medium containing sucrose, yeast extract, and potassium phosphate dibasic (K2HPO4). Another study revealed the optimal conditions required to culture and grow maximal amount of kefiran. These conditions include 25 °C temperature and 80 rpm agitation rate in addition to 0.1% (w/v) thiamine, 0.1% (w/v) iron(III) chloride, and 5% (w/v) lactose to achieve a high kefiran production yield in 24 hours.40 Besides that, the presence of whey was showed to improve kefiran production effectively. Blandón et al.47 indicated that whey-supplemented media together with 15% (w/v) glucose at 30 °C for 10 hours of fermentation was capable of generating 0.20 g L−1 h−1of purified kefiran from 4.2 g L−1 kefir grains without losing its biocidal and antimicrobial characteristics. A comprehensive list of diverse bacteria and yeast strains used in the production of kefiran is presented in Table 1.48–52 A few literature sources23,34,41,48–52 have provided an in-depth understanding of the production and extraction methodologies of kefiran. Hence, this review presents an overall summary of the most significant findings pertaining to this area.
Table 1 Bacteria and yeasts present in kefir and kefir grains
|
Strain |
References |
Bacteria |
Lactobacilli |
Lactobacillus kefir, Lactobacillus viridescens, Lactobacillus helveticus, Lactobacillus fermentum, Lactobacillus acidophilus, Lactobacillus hilgardii, Lactobacillus plantarum, Lactobacillus fructivorans, Lactobacillus brevis, Lactobacilli paracasei, Lactobacillus parakefir, Lactobacillus casei, Lactobacillus kefirgranum, Lactobacillus rhamnosus, Lactobacillus kefiranofaciens, Lactobacillus delbrueckii |
7 and 54–57 |
Streptococci |
Streptococcus thermophilus |
54 |
Enterococci |
Enterococcus durans |
58 |
Leuconostocs |
Leuconostoc spp., Leuconostoc mesenteroides |
7, 55 and 59 |
Acetobacter |
Acetobacter sp., Acetobacter aceti |
7, 55 and 57 |
Bacillus |
Bacillus sp., Bacillus subtilis |
57 |
![[thin space (1/6-em)]](https://www.rsc.org/images/entities/char_2009.gif) |
Yeast |
Saccharomyces |
Saccharomyces delbrueckii, Saccharomyces dairensis, Saccharomyces turicensis |
54 and 57 |
Candida |
Candida tannotelerans, Candida valida, Candida inconspicua, Candida holmii, Candida kefir, Candida valida, Candida lambica |
54, 57 and 60 |
Pichia |
Pichia fermentans |
54 and 61 |
Issatchenkia |
Issatchenkia occidentalis |
60 |
Brettanomyces |
Brettanomyces anomalush |
61 |
Torulaspora |
Torulaspora delbrueckiia |
54, 57 and 61 |
There has been a significant interest in the scientific community in boosting kefiran production to a semi-industrial scale with the use of bioreactors.23 The extraction of kefiran from the kefir grains suffers from inherent disadvantages such as low-yield, batch to batch variations, and associated cost structures. As a result, extensive research efforts have been channelled towards the extraction of kefiran via water-based fermentation medium with a carbon source in the presence of yeasts and bacteria.35 Subsequently, other studies focused on determining if there are any observed changes to the chemical structures of kefiran that are isolated using different sugar sources.53 However, the low-yield issue still remains to be resolved. The current production technique is inefficient and only allows the use of kefiran in small-scale and high-margin applications, such as medical technology23 due to the less productive extraction techniques. Large-scale applications such as food packaging requires a more efficient and cost-effective manufacturing process.
3. Potential applications of kefiran
3.1. Food industry: biodegradable and edible food packaging
With increasing environmental pollutions due to the manufacturing of traditional food packaging materials made from non-renewable sources or synthetic polymers, there is an urgent need for alternative materials to mitigate elevating environmental concerns.62 In recent years, great efforts have been placed to develop renewable packaging materials as substitutes to replace the traditional plastic packaging. The commonly reported substitutes are food-grade ingredients or food wastes. Hence, natural carbohydrate-based polymers like cellulose, starch, soy protein, and kefiran are considered potential substitutes in numerous studies and reviews.63–65 However, none has discussed the properties of kefiran-based film exclusively as a food packaging material. Besides possessing excellent strengths and characteristics comparable with traditional packaging materials, kefiran offers other benefits including being compostable, biodegradable, and/or edible. These characteristics render kefiran to be environmentally friendly and more sustainable for a host of applications,27,66–68 as seen in Fig. 2. Consequently, this could create substantial commercial opportunities for kefiran to be considered for the packaging industry.
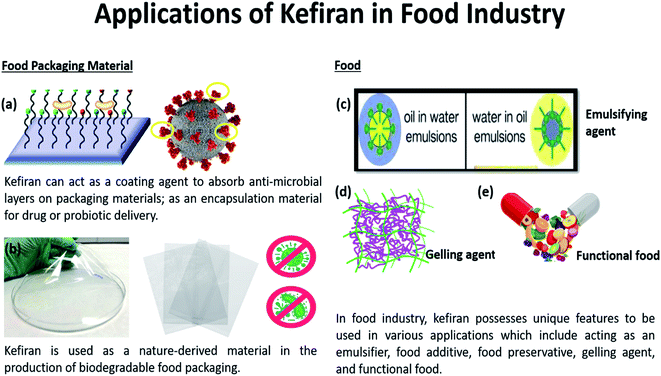 |
| Fig. 2 Kefiran offers a wide spectrum of applications in the food industry as (a) antimicrobial coating to enhance food shelf life, (b) biodegradable food packaging material as a replacement for the non-biodegradable petroleum-based packaging, (c) emulsifier, (d) gelling agent and (e) functional food due to its good rheological features and distinctive functional properties.84,85 | |
3.1.1 Improved oxygen barrier and water vapour permeability (WVP). The establishment of emerging renewable materials has demonstrated that oxygen barrier will pave a way to create new markets for agro-based products. Oxygen barrier is one of the biggest challenges encountered when natural polymers, such as kefiran, is used as a packaging material. Oxygen and moisture barrier are important parameters to be considered for an ideal packaging material because food quality is easily altered by the mass transport of oxygen and fluids between the packaging materials and their environment. Loss or gain of water and oxygen are vital factors in food deterioration.69 Both the oxidation and high moisture content of nutritional components can compromise the package and drastically reduce food shelf-life due to the action of moulds or other microorganisms.70 Oxygen and water provide favourable environment in boosting microbial growth of harmful microorganisms. Therefore, it is essential to obtain a packaging material with high resistance to oxygen and moisture permeation. Oxygen barrier is often measured based on the oxygen permeation measurement with ASTM standards.71,72 ASTM standard states that films possess poor oxygen barrier if their oxygen transmission rate is more than 200 cm3 per m2 per day per atm, which is highly dependent on film thickness.71Zolfi et al. reported that kefiran and whey protein isolate (WPI) based bionanocomposites fabricated with nanofillers have potential applications as effective oxygen and moisture barriers.73 They synthesized kefiran packaging film via solvent casting and solvent-evaporation techniques with increased tensile strength, higher Young's modulus, and minimized water vapour permeability (WVP), when (50
:
50 (v/v)) of kefiran
:
WPI and an addition of 5% (w/w) montmorillonite (MMT) were used. The results revealed that biodegradable kefiran–WPI–MMT formulation is an ideal food packaging material with a longer shelf life.
In another study, kefiran was blended with corn starch as a new edible film. The findings indicated that blended kefiran–starch film was compatible with one another for synergetic effects when >50% (v/v) starch was used. It possesses decreased WVP, enhanced tensile strength, and elasticity which are essential as food packaging films.74 Decreased WVP value indicated that both kefiran and starch molecules were interacted favourably via the formation of strong hydrogen bonds between them. As a result, this serves as a protection against the diffusion of water through blended films and hence, lowered the WVP value.
Besides, Sabaghi et al. further demonstrated the good compatibility, WVP, and antioxidant activities of kefiran-blended chitosan films when applied as a bio-packaging material.75 In addition, WVP value is largely affected by plasticizers. For instance, Piermaria et al.67 reported that high concentrations (>25 g of glycerol per 100 g of kefiran) of glycerol can enhance the water activity and moisture content of the films and thus, indirectly affecting the WVP value.
3.1.2 UV-protective properties. A more recent study76 revealed the promising potentials of a low-cost UV-protective starch/kefiran/ZnO packaging film using solution casting approach. The presence of 3% nano ZnO was shown to drastically enhance the film properties including thermal, mechanical, and UV-protective properties as well as its decomposition temperature and rate. Effective UV-blocking is a crucial factor especially in edible food packaging. This is due to the adverse impacts of UV rays on light-susceptible nutrients, flavour quality, food discoloration, and lipids photo oxidation. The UV-protective behaviours of this film were confirmed in the UV-A, UV-B, and UV-C regions, showing its capability as an effective packaging film. Interestingly, Shahabi-Ghahfarrokhi et al. noted the positive effects of UV treatment (UV-C light) in improving the overall properties of starch–kefiran–ZnO (SKZ). The findings displayed that increased UV-C exposure time has significantly reduced water-related properties such as WVR and WVP. Also, it offers enhanced mechanical strength, water contact angle, and better UV-protective effects due to photo-modification. All these are attributed to the better distribution of ZnO nanofillers in the biopolymer mixtures with the use of UV as compatibilizer. UV-modified SKZ can therefore be utilized for both sanitizing and food packaging applications concurrently.77
3.1.3 Enhanced mechanical strength. Good mechanical strength and adequate flexibility are required for edible films to provide mechanical barriers for food content. Pure kefiran films exhibit high tensile strength and brittleness during mechanical tests. Kefiran films (10 g kg−1) prepared by solvent casting were reported to possess the tensile strength of 40.92 ± 7.83 MPa and the elongation at break of 2.70 ± 0.47%,67 making them a strong candidate as a food packaging material with tensile strength highly comparable to conventional plastics. The addition of plasticizers such as glycerol and sorbitol is a common method to turn kefiran films into satisfactory food packaging or edible films with increased elasticity and flexibility.67 Nanotechnology has also been applied to tailor the mechanical characteristics of kefiran films for better packaging properties. For instance, 1 wt% cellulose nanocrystals were incorporated into the glycerol-plasticized kefiran films as reinforcements. The work displayed a maximum tensile strength of 8.14 ± 1.27 MPa and the highest elongation at break value of 252.17 ± 27.66%.63 However, it is important to note that higher content of nano-composites can destroy the integrity of the polymer matrix and lead to poorer mechanical strengths. The distribution of the reinforcements, and the interfacial adhesion between the reinforcements and the matrix also play vital roles in determining the mechanical properties of kefiran-based composite films. Other reinforcements in kefiran-based packaging films such as TiO2 and ZnO nanoparticles have also been reported in the literature.63,73,76–78
3.1.4 Anti-microbial strength. Last but not least, it is vital to develop effective sustainable food packaging solutions to increase shelf life of food products. Food packaging materials should interact safely with food, behave as chemical barrier against the environment, and also possess anti-microbial properties. Kefiran-based films are capable of improving food safety, attributed to its natural antibacterial and antimicrobial properties.27,29 Antimicrobial packaging is classified as a form of active packaging, wherein it interacts with the food within the headspace to inhibit microbial growth. Nisin is the lantibiotic presented in kefiran which contributes to the antibacterial features of kefiran.79 The presence of Nisin could trigger both the CD4/CD8 T-lymphocyte cell counts for a stronger immune system. Nisin/methylcellulose coating on polyethylene was illustrated as an example of coating or adsorbing anti-microbial layers on the packaging polymer surface.80 This is an ideal approach for antimicrobials that cannot tolerate the temperatures attained during the polymer formation and thus, can be coated onto the polymer after the formation. The journey of using kefiran to achieve sustainable anti-microbial packaging solutions has become an active topic of interest in recent years. Electrospun kefiran and polyethylene nanofibers were investigated as bioactive agents for food packaging and preservation. These nanofibers displayed anti-microbial activity against common Staphylococcus and Pseudomonas strains due to the presence of oxidizing functional groups on them.81 Another group fabricated kefiran–carboxymethylcellulose–copperoxide nanobiocomposite films which exhibited inhibitory effects against Staphylococcus aureus and bactericidal or bacteriostatic effects against Escherichia coli bacteria,82 showing the crucial role of kefiran against the Staphylococcal food-borne disease (SFD).83 This further highlights the great potential of kefiran packaging in benefitting future food industries in terms of maintaining food safety and security, as well as reducing food spoilage.
3.2. Biomedical applications of kefiran
As aforementioned, kefiran possesses a myriad of superior features to be an ideal candidate in diverse biopharmaceutical applications. It can be applied as a coating agent, drug conjugate, biologically active agent, or targeted delivery vehicle for clinical therapies. Here, we will focus on the potentials of kefiran for biomedical applications, focusing on drug delivery, tissue engineering and its use as a bioactive agent.
3.2.1 For probiotic and drug delivery. It is of great interest to utilise kefiran as the base material in the fabrication of thin films or delivery vehicles for biopharmaceutical drug delivery.86 It enhances both the pharmacokinetics and pharmacodynamics of encapsulated drugs to facilitate a faster recovery and even improve the general well-being of the individual. In addition, it can be embraced easily in comparison to synthetic materials.87 Piermaria et al. have demonstrated the efficacy of edible kefiran films as delivery carriers for probiotic living microorganisms such as Kluyveromyces marxianus CIDCA 8154 and Lactobacillus plantarum CIDCA 8327.66 Experimental results showed that the kefiran film was capable in preserving the viability of probiotics with a small loss after 35 days of storage at 20 °C. The susceptibility of free probiotic microorganisms to acidic condition was largely reduced when being encapsulated within kefiran matrix. This revealed the shielding effects of kefiran under acidic environment with a better bile resistance against gastrointestinal conditions. Hence, this demonstrated kefiran film to be a suitable vehicle to deliver probiotics for the treatment of gastrointestinal disorders. Also, its physiochemical and biological properties were not affected by the encapsulated ingredients, further supporting that kefiran can provide health-promoting values whilst acting as a delivery vehicle,66 as illustrated in Fig. 3.
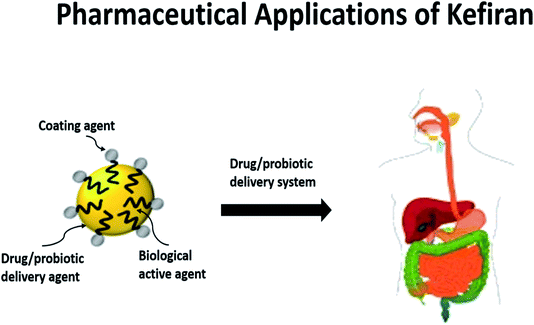 |
| Fig. 3 For pharmaceutical applications, kefiran can be developed into an encapsulation material for the delivery of drugs or probiotics and in the process enhance their bioavailability. Kefiran, as an encapsulation material, also possesses health-benefitting properties such as antimicrobial and antiatherogenic characteristics.89 | |
A study by Jenab et al. has developed a novel platelet-loaded kefiran polymer as a biological drug to treat wounds.79 In this study, glycoprotein Ib and IIb/IIIa receptors of platelets are responsible for the binding between platelets and kefiran. Rhanmosus-glucose derived from kefiran is responsible for the stimulation of platelet aggregation. Recently, kefiran has also been exploited as a drug vehicle to deliver an antibiotic – ciprofloxacin, orally via the novel kefiran-alginate gel microspheres.88 The study illustrated that ciprofloxacin-encapsulated kefiran-alginate gel microspheres are capable of improving the bactericidal performance towards common pathogens with antimicrobial outcomes. Besides, these gel microspheres provide a controlled drug release profile with ∼80% drug encapsulation efficiency. Its antimicrobial effects against pathogens such as E. coli, Salmonella, Staphylococcus, and Pseudomonas were due to the synergistic actions of both kefiran and ciprofloxacin. Also, the release rate of ciprofloxacin was faster in simulated alkaline intestinal conditions as compared to the harsh gastric environment, showing the possibility of increasing drug accumulation at the intestinal site.88 This study clearly showed both the effective antimicrobial delivery and antibacterial action of kefiran against pathogens.
3.2.2 For tissue engineering. Tissue engineering refers to the artificial production of cell tissue to repair, replace, restore, and regenerate destroyed or diseased tissues. The prerequisites of tissue engineering are the synergistic effects exhibited by cells, growth factors or signals, and scaffolds.90 Scaffolds serve as a matrix on which the cells grow to reach the desired shape. Scaffolds made using kefiran polysaccharide are biodegradable and their degradation products are classified as GRAS, denoting their safety and quality.67,91 Also, due to the possibility of controllable 3D morphology and their commendable mechanical properties, polymeric kefiran scaffolds are promising materials for tissue engineering as displayed in Fig. 4.92
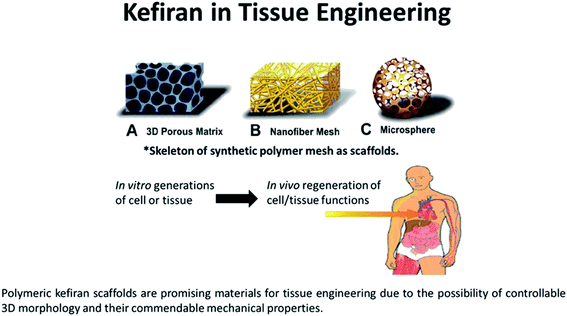 |
| Fig. 4 The biodegradable and biocompatible properties of kefiran makes it an ideal scaffold material for tissue engineering. Kefiran-based scaffolds incorporated with tissue grafts or other bioactive materials can aid in regenerating new tissues. As a scaffold, it can take the form of (a) a three-dimensional porous matrix, (b) nanofibrous mesh, or even as a (c) hollow microsphere for cell growth and proliferation.96,97 | |
Ghasemlou et al. demonstrated kefiran films with good tensile strength in the range of 5–18 MPa and elongation of 40–160%, suggesting kefiran as a desirable polymeric matrix in tissue engineering applications.93 These films were fabricated from pure kefiran with the use of glycerol or sorbitol as the plasticizer. Radhouani et al. synthesized a novel 3D kefiran-based scaffold via the free gelation technique for tissue engineering. Human adipo-derived stem cells were reported to remain metabolically active after 72 hour culturing onto kefiran scaffold. The synthesized kefiran scaffold from kefiran cryogels was characterized with high stability, elastic behaviour, and a porous 3D structure, which are key fundamental features for any tissue engineering scaffolds.94 A patent (WO2018042405A1) has also been filled that innovates the use of kefiran (extracted from Portuguese kefir grains) in regenerative medicine and/or tissues engineering, compositions, and scaffolds.95 However, it is important to note that the scaffold material should have the same mechanical properties as the tissue to be replaced. Hence, the choice of the material relies remarkably on the intended applications.
3.2.3 As bioactive agent: antimicrobial, stimulating, antiatherogenic, modulating agent. Kefiran can act as a biologically active agent that improves the effects of therapeutic treatments with its health-promoting benefits. For example, Rodrigues et al. demonstrated the efficiency of kefiran in treating Candida albicans bacteria by enhancing protective effect to dermal connective tissue and promoting wound healing in an in vivo study.98 They also indicated the presence of nisin in kefiran as the antibacterial lantibiotic.79Besides, kefiran can act as a stimulant to the human intestinal immune system by altering the number of immune cells99 and promoting immunomodulation.100 Kefiran is also capable of inhibiting the phosphorylation of glycogen synthase kinase 3β, Akt, and extracellular signal-regulated kinases upon the stimulation of antigen. This reveals its potential in treating mast cells-dependent allergic diseases.19 In addition, it was reported to prevent the antigen (FcεRI)-triggered mast cell activation. This includes the mast cell degranulation, Ca2+ mobilization, and production of tumour necrosis factor-α in the mast cells of bone marrow due to its anti-inflammatory effect.
In another in vivo study,101 kefiran was discovered to have antiatherogenic effect in preventing atherosclerosis due to its hypocholesterolemic, antioxidant and anti-inflammatory actions. The results detected a significant low level of atherosclerotic lesions, reduced lipid peroxidation, negligible number of T-lymphocytes, and lower cholesterol level in the liver of tested rabbits.
Serafini et al. have revealed the efficacies of kefiran in enhancing the growth of Bifidobacterium bifidum PRL 2010 strain by modulating its gene expression. Bifidobacteria is one of the predominant microorganism species colonizing the gastrointestinal tract (GIT) of human infants. The experimental data revealed kefiran is capable of increasing the transcription of genes which are responsible for the metabolism of dietary glycans and expression of host-microbe responsive molecule effector.1 Therefore, kefiran can contribute significantly to probiotic therapy. Based on literature, kefiran has been studied extensively since past decades for different applications in the biopharmaceutical and biomedical industries. There are many other reported studies displayed in Table 2 on kefiran-based formulations with different commercial values.
Table 2 A summary of some kefiran-based formulations used in various biopharmaceutical applications
Kefiran-based formulation |
Descriptions |
References |
Kefiran extracted from Portuguese kefir grains |
• Kefiran possesses pseudoplastic behaviour and adhesive performance which help the adhesion of drug molecules to biological surfaces or for biofilm formation |
68 |
• Its pseudoplastic behaviour and gelation ability make it as a suitable polymer to be the matrix environments of various therapeutic agents such as stem cells, proteins, and genetically engineered cells |
• Kefiran has a higher resistance towards the hyaluronidase degradation (enzymatic resistance) |
• It owns high potentials in bone defects and articular cartilage applications due to its mechanical and viscoelastic characteristics |
Kefiran |
• Oral administration of kefiran enhances the balance of immune cells in intestinal mucosa due to its probiotic effects |
99 |
• Kefiran is capable to modify cytokine and immunoglobulin profiles |
• It is possible to trigger an immune response. Therefore, contribute to intestinal homeostasis |
Kefiran |
• Orally administrated kefiran from Lb. kefiranofaciens can promote the number of IgA+ cells in both small and large intestine lamina propria without affecting the number of IgG+ cells |
100 |
• It can induce immune response and enhance the intestinal homeostasis |
Kefiran |
• Kefiran has the potential to be a stress-reducing food supplement due to its capability in improving the production of noradrenaline and interferon B-cortisol in human cell lines |
102 |
Kefiran-derived from Tibetan kefir grains |
• The isolated kefiran was identified with high antioxidant activity which shields proteins from oxidative damage in a dose-dependent pattern |
103 |
• It has a good thermal stability |
• It is an ideal candidate as a natural antioxidant supplement |
• It can be also be applied widely in food industries as functional food or ingredients |
Lactobacillus kefiranofaciens-derived kefiran |
• Kefiran is effective in lowering the blood pressure, cholesterol, and glucose levels in rats and mice |
104 |
• The in vivo study also indicated kefiran is capable to improve the fecal wet weight and moisture level in constipated rats |
Kefiran |
• Orally administrated kefiran possesses bifidogenic effect on the intestinal microbiota of the studied mice (BALB/c) in animal trials |
105 |
• The increased Bifidobacterium level in the GIT of infants helps to minimize the incidence of allergic disease in later stage of life |
4. Challenges and prospective
4.1. Challenges
One of the challenges encountered with the practical applications of kefiran is to have a standardized nutritional content and scalability in its production. Nutritional values and productions may vary based on the type of growth medium and culturing conditions. Apart from that, there is a lack of standardization or protocols for the generation of kefiran, leading to batch-to-batch variation in controlling both the quality and quantity of kefiran produced. Further studies are therefore required to achieve good repeatability across batches and research groups. These future studies are essential to further provide clarity and consistency on the nutritional content of kefiran as well as standardizing its production protocols. This can eventually lead to a better control of its health-promoting functions for wider applications of kefiran, especially in the agri-food and biomedical industries.
Besides that, EPS such as kefiran may contribute to food spoilage and inhibit productivity in certain situations. EPS produced by lactic acid bacteria (during the fermentation of cider and wine) have led to unpleasant rheological characteristics.25 Furthermore, some EPS cause excessive biofilm formation that can affect general hygiene and lead to poor productivity in dairy industries, owing to the increased surface corrosion, heat flow obstruction, fluid frictional resistance, and heat loss.106 Biofilm is mainly produced by microorganisms that are capable of aggregating and growing into microcolonies leading to a biofilm. Kefiran, as an extracellular polymeric compound, can also function as the attachment site for microorganisms to be protected against sanitizers.106
Despite the immense potentials of kefiran, scalable fabrication persists as one of the major challenges limiting the practical applications of kefiran. Difficulties in scaling up the manufacturing process can be attributed to several factors. This includes the costly production of kefiran when carbon and nitrogen are used as energy sources.27 It is also difficult to develop suitable fermentation medium in a shorter production time due to the unavailability of medium components and nutrients. The reduced product recovery due to inefficient downstream processing has limited commercial exploitation for kefiran. In addition, the optimal conditions for maximal production is often challenged by parameters such as pH, amount of accumulated lactic acid, and temperature.107 This is mainly because kefiran is highly sensitive to changes in the environment. Hence, the lack of optimal production process can impact the contribution of kefiran towards the biopolymer market. Further studies on the biosynthesis and bioprocess technologies of kefiran can possibly address the above problems.
Lastly, the highly water-soluble properties of kefiran can impede its potentials for some biomedical applications, significantly affecting its applicability in industry and for commerce. On the other hand, its water solubility is also an advantageous feature in other applications. Water solubility is useful for food product packaging where thermal solubility in water is required. Water-soluble kefiran is edible, and as a food packaging, its solubility may provide an environmental-friendly route for disposal.67 In addition, the hydrophilicity of kefiran can also enhance its cell adhesion and proliferation capacity on targeted cells for a sustained drug delivery. Furthermore, highly water-soluble polymers are desirable for drug delivery applications to afford higher solubility, improved bio-adhesion, and enhanced bioavailability of the delivered encapsulates.108
4.2. Prospective
Kefiran can act as a functional food or as biopharmaceutical agents to improve human health, as summarized in Fig. 2–4. As a food-derived biopolymer and labelled as GRAS, it is a favourable for both agri-food applications.66 Its health-benefiting properties such as immunomodulatory effects19,99 and efficacy against pathogenic Bacillus cereus,109 amongst others, clearly display its potentials in the biopharmaceutical field.
4.2.1 Ultrasound-based kefiran production. A very recent study by a research team of South Ural State University, claimed to have developed a super healthy kefiran with the use of low-frequency ultrasound. Potoroko et al. have applied ultrasound to increase the kefiran content and functional properties of the kefir milk drink. The ultrasound was applied prior to the fermentation stage to provide a better environment for the bacterial fermentation. They discovered the stimulation effects of ultrasound in the production of health-promoting microorganisms. Their work has identified ultrasound as a promising candidate in increasing the accumulation of kefiran during the fermenting process of kefir as well as offering higher nutritional values of the fermented end-product. Further studies have to be conducted to understand the mechanism of ultrasound in enhancing the active synthesis of kefiran and searching for more hidden potentials of kefiran produced from ultrasound-based kefir fermentation.110 In addition, scaling the production of kefiran is an area that should be extensively research for kefiran to be a viable material for agri-food and biopharmaceutical applications.
4.2.2 Controlled delivery for agricultural use. Nature derived biopolymers and biomaterials are highly desired in agricultural sector to improve crop productivity, environmental monitoring, food security, and nutritional values. They are commonly used as targeted delivery agents or bioactive agents in herbicides, pesticides, fertilizers, and environmental monitoring sensors.111 Kefiran which is a natural exopolysaccharides serves as a promising candidate for diverse agricultural applications. It can act as a controlled delivery agent with easily tunable physiochemical properties that imparts controlled release pattern of encapsulated bioagents. This can certainly help to specifically deliver pesticides and fertilizers locally to crops without causing environmental pollutions to the surrounding air, water, and soil.112 Kefiran is capable of crosslinking with other biomaterials as effective delivery carriers. For instance, kefiran can be crosslinked with alginate to deliver encapsulated active agents.88 This is due to its Newtonian behaviour that results in pseudoplastic characteristics and formation of gels. In addition, kefiran can interact with different biomaterials via various bonding.79
4.2.3 Probiotic encapsulation material. Natural polysaccharides are most extensively studied for encapsulation techniques and applications because they are biodegradable and highly biocompatible. Kefiran is capable of protecting any encapsulated active pharmaceutical compounds from the harsh physiological environment either as a coating or as a matrix formulation. Since kefiran is regarded as safe for human consumption, it offers multiple benefits for biopharmaceutical delivery. This includes improved target specificity and bioavailability of the encapsulated drug molecules.68Probiotics have been developed for human consumption to improve health. Kefiran as a prebiotic is capable of delivering probiotics specifically to intestinal sites. This is because kefiran is non-digestible and non-absorbable in the upper part of GIT (stomach) as it is resistant against acidic conditions and the digestive enzymes of the GIT.113 Kefiran is degraded by intestinal microbiota or bacterial enzymes in the intestinal system or colon, where it can be taken up by specific bacteria to release the encapsulated probiotics. This makes kefiran potentially competent in the development of intestinal/colon-targeted delivery system for various probiotics. Intestinal microbes possess significant impact on the health of a human host. There are a diverse kind of microorganisms resided in the intestinal tract within a range of 1014 to 1000 different bacterial species. It was reported that oral administration of kefiran can enhance the intestinal bifidobacterial populations and microbiota, indicating the bifidogenic effect of kefiran.105,114 This further supports its great potentials to encapsulate and deliver probiotics for a more effective therapeutic outcome on human health.
4.2.4 Skin wound healing. Skin wound healing is a complex process and requires time for skin tissues to repair themselves. The use of tissue grafts or scaffolds can therefore significantly improve healing. For instance, kefiran-based scaffolds incorporated with tissue grafts or other bioactive materials can be applied for successful regeneration of damaged skin tissues. Tissue scaffolds can provide mechanical support, allow for influx of essential nutrients and a bioactive environment for cells to develop and regenerate into tissues.115 Kefiran has demonstrated wound healing properties and can be a suitable scaffold material due to its biodegradable and biocompatible properties as well as its ideal surface features, porosity, geometry and mechanical strength.116 Natural polymers such as kefiran are among the first choice of biodegradable scaffold materials to be exploited for clinical purposes. This is attributed to their better polymer–cell interactions with diverse cell types, and lack of immune responses or toxicity.117
4.2.5 Cross-linked kefiran. Kefiran possesses potentials to be cross-linked with other natural polymers and polysaccharides to form various complex such as hydrogel films and polymeric nano/microparticles for useful applications, including transdermal or oral drug delivery, tissue engineering and encapsulation of therapeutic agents. Furthermore, kefiran can be conjugated or coupled with different biomacromolecules, proteins, peptides, and amino acids to develop bio-complex with favourable biochemical and biophysical properties compared to synthetic polymers.118 This is attributed to its varying degree of structural branching that allows for cross-linking with other biomaterials to create novel materials.68,119 Therefore, this aspect is an essential research endeavour for future work to investigate the cross-linking ability of kefiran to expand its potential to myriad of applications.
5. Conclusion
Kefiran, the EPS isolated from kefir, possesses advantageous biological, physicochemical, and rheological characteristics, with strong potential for the agri-food and biomedical industries. This article presents a detailed review on the functionalities, structural compositions, health benefits, competence as well as the latest applications of kefiran in major sectors covering food packaging, biomedical and biopharmaceuticals. More research is vital for the scientific community to successfully identify and develop new potential applications in the near future. More importantly, efforts are needed to optimize production of kefiran towards industrial scales, as well as addressing the aforementioned drawbacks so as to fully maximize its potential for the global community.
Conflicts of interest
The authors declare no conflict of interest.
Acknowledgements
The authors wish to acknowledge the financial support from the Singapore Centre for Environmental Life Sciences Engineering (SCELSE) (MOE/RCE: M4330019.C70), Ministry of Education AcRF-Tier 1 grant (RG19/18), Agri-Food & Veterinary Authority of Singapore (APF LCK102), Biomedical Research Council (BMRC) – Therapeutics Development Review (TDR-G-004-001), NTU-HSPH grant (NTU-HSPH 17002), and the Bill and Melinda Gates Foundation (OPP1199116).
References
- F. Serafini, et al., Kefir fermented milk and kefiran promote growth of Bifidobacterium bifidum PRL2010 and modulate its gene expression, Int. J. Food Microbiol., 2014, 178, 50–59 CrossRef CAS PubMed
. - S. Plessas, et al., Microbiological Exploration of Different Types of Kefir Grains, Fermentation, 2017, 3(1), 1 CrossRef
. - K. Zajšek, M. Kolar and A. Goršek, Characterisation of the exopolysaccharide kefiran produced by lactic acid bacteria entrapped within natural kefir grains, Int. J. Dairy Technol., 2011, 64(4), 544–548 CrossRef
. - L. Morelli, Yogurt, living cultures, and gut health, Am. J. Clin. Nutr., 2014, 99(5), 1248S–1250S CrossRef CAS PubMed
. - M. A. Golowczyc, et al., Characterization of homofermentative lactobacilli isolated from kefir grains: potential use as probiotic, J. Dairy Res., 2008, 75(2), 211–217 CrossRef CAS PubMed
. - K. T. Magalhães, et al., Brazilian kefir: structure, microbial communities and chemical composition, Braz. J. Microbiol., 2011, 42(2), 693–702 CrossRef
. - N. Korsak, et al., Short communication: evaluation of the microbiota of kefir samples using metagenetic analysis targeting the 16S and 26S ribosomal DNA fragments, J. Dairy Sci., 2015, 98(6), 3684–3689 CrossRef CAS PubMed
. - J. Gao, et al., Investigation on culturable microflora in Tibetan kefir grains from different areas of China, J. Food Sci., 2012, 77(8), M425–M433 CrossRef CAS PubMed
. - A. Dobson, et al., High-throughput sequence-based analysis of the bacterial composition of kefir and an associated kefir grain, FEMS Microbiol. Lett., 2011, 320(1), 56–62 CrossRef CAS PubMed
. - N. R. Zamberi, et al., 16S Metagenomic Microbial Composition Analysis of Kefir Grain Using MEGAN and BaseSpace, Food Biotechnol., 2016, 30(3), 219–230 CrossRef CAS
. - I. Mainville, et al., Polyphasic characterization of the lactic acid bacteria in kefir, Syst. Appl. Microbiol., 2006, 29(1), 59–68 CrossRef CAS PubMed
. - Z. Kesmen and N. Kacmaz, Determination of lactic microflora of kefir grains and kefir beverage by using culture-dependent and culture-independent methods, J. Food Sci., 2011, 76(5), M276–M283 CrossRef CAS PubMed
. - A. M. Leite, et al., Assessment of the microbial diversity of Brazilian kefir grains by PCR-DGGE and pyrosequencing analysis, Food Microbiol., 2012, 31(2), 215–221 CrossRef CAS PubMed
. - E. R. Farnworth, Kefir: from folklore to regulatory approval, Journal of Nutraceuticals, Functional and Medical Foods, 1999, 1(4), 57–68 CrossRef
. - A. M. d. O. Leite, et al., Microbiological, technological and therapeutic properties of kefir: a natural probiotic beverage, Braz. J. Microbiol., 2013, 44(2), 341–349 CrossRef PubMed
. - M. R. Prado, et al., Milk kefir: composition, microbial cultures, biological activities, and related products, Front Microbiol., 2015, 6, 1177 Search PubMed
. - J. Piermaria, et al., Shear and extensional properties of kefiran, Carbohydr. Polym., 2016, 152, 97–104 CrossRef CAS PubMed
. - J. W. Riviére, P. Kooiman and K. Schmidt, Kefiran, a novel polysaccharide produced in the kefir grain by Lactobacillus brevis, Arch. Microbiol., 1967, 59(1), 269–278 Search PubMed
. - T. Furuno and M. Nakanishi, Kefiran suppresses antigen-induced mast cell activation, Biol. Pharm. Bull., 2012, 35(2), 178–183 CrossRef CAS PubMed
. - D. Domene-López, et al., Influence of Starch Composition and Molecular Weight on Physicochemical Properties of Biodegradable Films, Polymers, 2019, 11(7), 1084 CrossRef PubMed
. - J. F. Robyt, Starch: Structure, Properties, Chemistry, and Enzymology, in Glycoscience: Chemistry and Chemical Biology, ed. B. O. Fraser-Reid, K. Tatsuta and J. Thiem, Springer Berlin Heidelberg, Berlin, Heidelberg, 2008, pp. 1437–1472 Search PubMed
. - G. A. M. Ruiz and H. F. Z. Corrales, Chitosan, Chitosan Derivatives and their Biomedical Applications, in Biological Activities and Application of Marine Polysaccharides, 2017 Search PubMed
. - D. J. Dailin, et al., Bioprocess development for kefiran production by Lactobacillus kefiranofaciens in semi industrial scale bioreactor, Saudi J. Biol. Sci., 2016, 23(4), 495–502 CrossRef CAS PubMed
. - A. M. de Oliveira Leite, et al., Microbiological, technological and therapeutic properties of kefir: a natural probiotic beverage, Braz. J. Microbiol., 2013, 44(2), 341–349 CrossRef PubMed
. - S. Patel, A. Majumder and A. Goyal, Potentials of exopolysaccharides from lactic acid bacteria, Indian J. Microbiol., 2012, 52(1), 3–12 CrossRef CAS PubMed
. - C. Pop, et al., Influence of extraction conditions on characteristics of microbial polysaccharide kefiran isolated from kefir grains biomass, J. Food Nutr. Res., 2016, 55, 121–130 CAS
. - Z. Moradi and N. Kalanpour, Kefiran, a branched polysaccharide: preparation, properties and applications: a review, Carbohydr. Polym., 2019, 223, 115100 CrossRef CAS PubMed
. - S. M. John and S. Deeseenthum, Properties and Benefits of Kefir – A Review, Songklanakarin J. Sci. Technol., 2015, 37(3), 275–282 Search PubMed
. - V. Manthani and P. Rao, Kefiran – A therapeutic Biofilm: A Review, Int. J. Food Sci. Nutr., 2017, 6(4), 82–89 Search PubMed
. - A. A. Bengoa, et al., Kefir micro-organisms: their role in grain assembly and health properties of fermented milk, J. Appl. Microbiol., 2019, 126(3), 686–700 CrossRef CAS PubMed
. - B. Cheirsilp, H. Shimizu and S. Shioya, Enhanced kefiran production by mixed culture of Lactobacillus kefiranofaciens and Saccharomyces cerevisiae, J. Biotechnol., 2003, 100(1), 43–53 CrossRef CAS PubMed
. - H. C. Chen, S. Y. Wang and M. J. Chen, Microbiological study of lactic acid bacteria in kefir grains by culture-dependent and culture-independent methods, Food Microbiol., 2008, 25(3), 492–501 CrossRef CAS PubMed
. - S.-Y. Wang, et al., Investigation of microorganisms involved in biosynthesis of the kefir grain, Food Microbiol., 2012, 32(2), 274–285 CrossRef CAS PubMed
. - H. Yokoi and T. Watanabe, Optimum culture conditions for production of kefiran by Lactobacillus sp. KPB-167B isolated from kefir grains, J. Ferment. Bioeng., 1992, 74(5), 327–329 CrossRef CAS
. - T. Mitsue, K. Tachibana and Y. Fujio, Efficient kefiran production by a mixed culture of Lactobacillus kefiranofaciens KF-75 and yeast strains, J. Biosci. Bioeng., 1999, 3(87), 400 Search PubMed
. - K. Hayakawa, et al., High density culture of Lactobacillus casei by a cross-flow culture method based on kinetic properties of the microorganism, J. Ferment. Bioeng., 1990, 70(6), 404–408 CrossRef CAS
. - Y. Nomura, K. Yamamoto and A. Ishizaki, Factors affecting lactic acid production rate in the built-in electrodialysis fermentation, an approach to high speed batch culture, J. Ferment. Bioeng., 1991, 71(6), 450–452 CrossRef CAS
. - H. Shimizu, et al., Nisin Production by a Mixed-Culture System Consisting of Lactococcus lactis and Kluyveromyces marxianus, Appl. Environ. Microbiol., 1999, 65(7), 3134–3141 CrossRef CAS PubMed
. - M. Wydes, H. Spillmann and Z. Puhan, Investigation of the yeast flora in dairy products: a case study of kefyr, Food Technol. Biotechnol., 1997, 35, 299–304 Search PubMed
. - K. Zajšek, A. Goršek and M. Kolar, Cultivating conditions effects on kefiran production by the mixed culture of lactic acid bacteria imbedded within kefir grains, Food Chem., 2013, 139(1), 970–977 CrossRef PubMed
. - E. A. Günter and Y. S. Ovodov, Effect of calcium, phosphate and nitrogen on cell growth and biosynthesis of cell wall polysaccharides by Silene vulgaris cell culture, J. Biotechnol., 2005, 117(4), 385–393 CrossRef PubMed
. - B. Cheirsilp, et al., Co-production of functional exopolysaccharides and lactic acid by Lactobacillus kefiranofaciens originated from fermented milk, kefir, J. Food Sci. Technol., 2018, 55(1), 331–340 CrossRef CAS PubMed
. - C. Cottet, et al., Biobased Materials from Microbial Biomass and Its Derivatives, Materials, 2020, 13(6) CrossRef PubMed
. - B. Cheirsilp, et al., Interactions between Lactobacillus kefiranofaciens and Saccharomyces cerevisiae in mixed culture for kefiran production, J. Biosci. Bioeng., 2003, 96(3), 279–284 CrossRef CAS PubMed
. - J. D. Daniel, et al., Development of cultivation medium for high yield kefiran production by Lactobacillus kefiranofaciens, Int. J. Pharm. Pharm. Sci., 2015, 7(3) Search PubMed
. - E. Enshasy and A. Homosany, Enhanced xanthan production process in shake flasks and pilot scale bioreactors using industrial semi-defined medium, Afr. J. Biotechnol., 2011, 10(6), 1029–1038 Search PubMed
. - L. Blandón Garcia, et al., Optimization of culture conditions for kefiran production in whey: the structural and biocidal properties of the resulting polysaccharide, Bioact. Carbohydr. Diet. Fibre, 2018, 16, 14–21 CrossRef
. - Z. B. Guzel-Seydim, et al., Functional properties of kefir, Crit. Rev. Food Sci. Nutr., 2011, 51(3), 261–268 CrossRef CAS PubMed
. - L. Chin-Wen, C. Hsiao-Ling and J.-R. Liu, Identification and characterisation of lactic acid bacteria and yeasts isolated from kefir grains in Taiwan, Aust. J. Dairy Technol., 1999, 54(1), 14 Search PubMed
. - M. E. Pintado, A. Macedo and F. Malcata, Technology, chemistry and microbiology of whey cheeses, Food Sci. Technol. Int., 2001, 7(2), 105–116 CrossRef CAS
. - L. Angulo, E. Lopez and C. Lema, Microflora present in kefir grains of the Galician region (North-West of Spain), J. Dairy Res., 1993, 60(2), 263–267 CrossRef CAS PubMed
. - X. Dousset and F. Caillet, Aspects microbiologiques et biochimisues de la fermentation du kéfir, Microbiol., Aliments, Nutr., 1993, 11(4), 463–470 CAS
. - T. Mukai, et al., Structural microheterogeneity of kefiran from kefir grains, Jpn. J. Zootech. Sci., 1988, 59(2), 167–176 CAS
. - S. Ed, et al., Lactic acid bacteria and yeasts in kefir grain and kefir made from them, J. Ind. Microbiol. Biotechnol., 2002, 28, 1–6 CrossRef PubMed
. - R. C. Witthuhn, T. Schoeman and T. J. Britz, Isolation and characterization of the microbial population of different South African kefir grains, Int. J. Dairy Technol., 2004, 57(1), 33–37 CrossRef
. - M. G. d. C. P. Miguel, et al., Diversity of bacteria present in milk kefir grains using culture-dependent and culture-independent methods, Food Res. Int., 2010, 43(5), 1523–1528 CrossRef
. - C. Garofalo, et al., Bacteria and yeast microbiota in milk kefir grains from different Italian regions, Food Microbiol., 2015, 49, 123–133 CrossRef CAS PubMed
. - P. Carasi, et al., Safety and potential beneficial properties of Enterococcus strains isolated from kefir, Int. Dairy J., 2014, 39(1), 193–200 CrossRef CAS
. - H.-C. Chen, S.-Y. Wang and M.-J. Chen, Microbiological study of lactic acid bacteria in kefir grains by culture-dependent and culture-independent methods, Food Microbiol., 2008, 25(3), 492–501 CrossRef CAS PubMed
. - G. Diosma, et al., Yeasts from kefir grains: isolation, identification, and probiotic characterization, World J. Microbiol. Biotechnol., 2014, 30(1), 43–53 CrossRef CAS PubMed
. - A. J. Marsh, et al., Sequencing-based analysis of the bacterial and fungal composition of kefir grains and milks from multiple sources, PLoS One, 2013, 8(7), e69371 CrossRef CAS PubMed
. - S. Guilbert, N. Gontard and L. G. Gorris, Prolongation of the shelf-life of perishable food products using biodegradable films and coatings, LWT--Food Sci. Technol., 1996, 29(1–2), 10–17 CrossRef CAS
. - I. Shahabi-Ghahfarrokhi, et al., Green bionanocomposite based on kefiran and cellulose nanocrystals produced from beer industrial residues, Int. J. Biol. Macromol., 2015, 77, 85–91 CrossRef CAS PubMed
. - V. Goudarzi, I. Shahabi-Ghahfarrokhi and A. Babaei-Ghazvini, Preparation of ecofriendly UV-protective food packaging material by starch/TiO2 bio-nanocomposite: characterization, Int. J. Biol. Macromol., 2017, 95, 306–313 CrossRef CAS PubMed
. - S.-Y. Wang, et al., Preparation and characterization of TIO2/SPI composite film, Mater. Lett., 2012, 83, 42–45 CrossRef CAS
. - J. Piermaria, et al., Edible kefiran films as vehicle for probiotic microorganisms, Innovative Food Sci. Emerging Technol., 2015, 32, 193–199 CrossRef CAS
. - J. A. Piermaria, et al., Films based on kefiran, an exopolysaccharide obtained from kefir grain: development and characterization, Food Hydrocolloids, 2009, 23(3), 684–690 CrossRef CAS
. - H. Radhouani, et al., Kefiran biopolymer: evaluation of its physicochemical and biological properties, J. Bioact. Compat. Polym., 2018, 33(5), 461–478 CrossRef CAS
. - J. Wang, et al., Moisture and Oxygen Barrier Properties of Cellulose Nanomaterial-Based Films, ACS Sustainable Chem. Eng., 2018, 6(1), 49–70 CrossRef CAS
. - M. Schmid and K. Müller, Whey Protein-Based Packaging Films and Coatings, in Whey Proteins, ed. H. C. Deeth and N. Bansal, Academic Press, 2019, ch. 11, pp. 407–437 Search PubMed
. - ASTM International, ASTM F1927-14, Standard Test Method for Determination of Oxygen Gas Transmission Rate, Permeability and Permeance at Controlled Relative Humidity Through
Barrier Materials Using a Coulometric Detector, 2014, West Conshohocken, PA Search PubMed
. - ASTM International, ASTM D3985-02e1, Standard Test Method for Oxygen Gas Transmission Rate Through Plastic Film and Sheeting Using a Coulometric Sensor, 2002, West Conshohocken, PA Search PubMed
. - M. Zolfi, et al., The improvement of characteristics of biodegradable films made from kefiran–whey protein by nanoparticle incorporation, Carbohydr. Polym., 2014, 109, 118–125 CrossRef CAS PubMed
. - A. A. Motedayen, F. Khodaiyan and E. A. Salehi, Development and characterisation of composite films made of kefiran and starch, Food Chem., 2013, 136(3–4), 1231–1238 CrossRef CAS PubMed
. - M. Sabaghi, Y. Maghsoudlou and P. Habibi, Enhancing structural properties and antioxidant activity of kefiran films by chitosan addition, Food Struct., 2015, 5, 66–71 CrossRef
. - A. Babaei-Ghazvini, I. Shahabi-Ghahfarrokhi and V. Goudarzi, Preparation of UV-protective starch/kefiran/ZnO nanocomposite as a packaging film: characterization, Food Packag. Shelf Life, 2018, 16, 103–111 CrossRef
. - I. Shahabi-Ghahfarrokhi and A. Babaei-Ghazvini, Using photo-modification to compatibilize nano-ZnO in development of starch–kefiran–ZnO green nanocomposite as food packaging material, Int. J. Biol. Macromol., 2019, 124, 922–930 CrossRef CAS PubMed
. - V. Goudarzi and I. Shahabi-Ghahfarrokhi, Development of photo-modified starch/kefiran/TiO2 bio-nanocomposite as an environmentally-friendly food packaging material, Int. J. Biol. Macromol., 2018, 116, 1082–1088 CrossRef CAS PubMed
. - A. Jenab, R. Roghanian and G. Emtiazi, Encapsulation of Platelet in Kefiran Polymer and Detection of Bioavailability of Immobilized Platelet in Probiotic Kefiran as a New Drug for Surface Bleeding, J. Med. Bacteriol., 2015, 4(3, 4), 45–55 Search PubMed
. - K. Cooksey, Utilization of Antimicrobial Packaging Films for Inhibition of Selected Microorganisms, in Food Packaging, American Chemical Society, 2000, pp. 17–25 Search PubMed
. - A. Jenab, et al., Manufacturing and structural analysis of antimicrobial kefiran/polyethylene oxide nanofibers for food packaging, Iran. Polym. J., 2017, 26(1), 31–39 CrossRef CAS
. - S.-M. Hasheminya, et al., Physicochemical, mechanical, optical, microstructural and antimicrobial properties of novel kefiran–carboxymethyl cellulose biocomposite films as influenced by copper oxide nanoparticles (CuONPs), Food Packag. Shelf Life, 2018, 17, 196–204 CrossRef
. - J. Kadariya, T. C. Smith and D. Thapaliya, Staphylococcus aureus and staphylococcal food-borne disease: an ongoing challenge in public health, BioMed Res. Int., 2014, 2014, 827965 Search PubMed
. - C.-S. Wang, et al., A gelation mechanism for gelatin/polysaccharide aqueous mixtures, Food Hydrocolloids, 2018, 79, 462–472 CrossRef CAS
. - K. Bazaka, et al., Anti-bacterial surfaces: natural agents, mechanisms of action, and plasma surface modification, RSC Adv., 2015, 5(60), 48739–48759 RSC
. - Z. Ahmed, et al., Kefir and health: a contemporary perspective, Crit. Rev. Food Sci. Nutr., 2013, 53(5), 422–434 CrossRef PubMed
. - P. Ballard, et al., The right compound in the right assay at the right time: an integrated discovery DMPK strategy, Drug Metab. Rev., 2012, 44(3), 224–252 CrossRef CAS PubMed
. - L. M. Blandon, et al., Kefiran-alginate gel microspheres for oral delivery of ciprofloxacin, Colloids Surf., B, 2016, 145, 706–715 CrossRef CAS PubMed
. - V. Truong-Le, P. M. Lovalenti and A. M. Abdul-Fattah, Stabilization Challenges and Formulation Strategies Associated with Oral Biologic Drug Delivery Systems, Adv. Drug Delivery Rev., 2015, 93, 95–108 CrossRef CAS PubMed
. - K. M. Kennedy, A. Bhaw-Luximon and D. Jhurry, Cell-matrix mechanical interaction in electrospun polymeric scaffolds for tissue engineering: implications for scaffold design and performance, Acta Biomater., 2017, 50, 41–55 CrossRef CAS PubMed
. - N. Bhardwaj, D. Chouhan and B. B. Mandal, Tissue Engineered Skin and Wound Healing: Current Strategies and Future Directions, Curr. Pharm. Des., 2017, 23(24), 3455–3482 CrossRef CAS PubMed
. - M. E. Gomes and R. L. Reis, Tissue engineering: key elements and some trends, Macromol. Biosci., 2004, 4(8), 737–742 CrossRef CAS PubMed
. - M. Ghasemlou, F. Khodaiyan and A. Oromiehie, Physical, mechanical, barrier, and thermal properties of polyol-plasticized biodegradable edible film made from kefiran, Carbohydr. Polym., 2011, 84(1), 477–483 CrossRef CAS
. - H. Radhouani, et al., Kefiran cryogels as potential scaffolds for drug delivery and tissue engineering applications, Mater. Today Commun., 2019, 20, 100554 CrossRef CAS
. - R. Hajer, et al., Kefiran for use in regenerative medicine and/or tissue engineering, in Patent Cooperation Treaty (PCT), WO/2018/042405, W.I.P. Organization, 2019
. - S. Tverdokhlebov, E. Bolbasov and E. Shesterikov, Scaffold Materials Based on Fluorocarbon Composites Modified with RF Magnetron Sputtering, 2012 Search PubMed
. - E. S. Place, et al., Synthetic polymer scaffolds for tissue engineering, Chem. Soc. Rev., 2009, 38(4), 1139–1151 RSC
. - K. L. Rodrigues, et al., Antimicrobial and healing activity of kefir and kefiran extract, Int. J. Antimicrob. Agents, 2005, 25(5), 404–408 CrossRef CAS PubMed
. - M. Medrano, et al., Oral administration of kefiran induces changes in the balance of immune cells in a murine model, J. Agric. Food Chem., 2011, 59(10), 5299–5304 CrossRef CAS PubMed
. - G. Vinderola, et al., Effects of the oral administration of the exopolysaccharide produced by Lactobacillus kefiranofaciens on the gut mucosal immunity, Cytokine, 2006, 36(5–6), 254–260 CrossRef CAS PubMed
. - M. Uchida, et al., Kefiran Reduces Atherosclerosis in Rabbits Fed a High Cholesterol Diet, J. Atheroscler. Thromb., 2010, 17(9), 980–988 CrossRef CAS PubMed
. - Y. Wang, et al., Physicochemical properties of exopolysaccharide produced by Lactobacillus kefiranofaciens ZW3 isolated from Tibet kefir, Int. J. Biol. Macromol., 2008, 43(3), 283–288 CrossRef CAS PubMed
. - Z. Chen, et al., Chemical and physical characteristics and antioxidant activities of the exopolysaccharide produced by Tibetan kefir grains during milk fermentation, Int. Dairy J., 2015, 43, 15–21 CrossRef CAS
. - H. Maeda, et al., Effects of an exopolysaccharide (kefiran) on lipids, blood pressure, blood glucose, and constipation, Biofactors, 2004, 22(1–4), 197–200 CrossRef CAS PubMed
. - M. F. Hamet, et al., Oral administration of kefiran exerts a bifidogenic effect on BALB/c mice intestinal microbiota, Benefic. Microbes, 2016, 7(2), 237–246 CrossRef CAS PubMed
. - R. A. N. Chmielewski and J. Frank, Biofilm Formation and Control in Food Processing Facilities, Compr. Rev. Food Sci. Food Saf., 2006, 2, 22–32 CrossRef
. - B. Cheirsilp and S. Radchabut, Use of whey lactose from dairy industry for economical kefiran production by Lactobacillus kefiranofaciens in mixed cultures with yeasts, New Biotechnol., 2011, 28(6), 574–580 CrossRef CAS PubMed
. - T. Miao, et al., Polysaccharide-Based Controlled Release Systems for Therapeutics Delivery and Tissue Engineering: From Bench to Bedside, Adv. Sci., 2018, 5(4), 1700513 CrossRef PubMed
. - M. Medrano, P. F. Perez and A. G. Abraham, Kefiran antagonizes cytopathic effects of Bacillus cereus extracellular factors, Int. J. Food Microbiol., 2008, 122(1–2), 1–7 CrossRef CAS PubMed
. - I. Potoroko, et al., Ultrasound effects based on simulation of milk processing properties, Ultrason. Sonochem., 2018, 48, 463–472 CrossRef CAS PubMed
. - R. Prasad, A. Bhattacharyya and Q. D. Nguyen, Nanotechnology in Sustainable Agriculture: Recent Developments, Challenges, and Perspectives, Front. Microbiol., 2017, 8, 1014 CrossRef PubMed
. - G. A. Valencia, et al., Self-Assembled Carbohydrate Polymers for Food Applications: A Review, Compr. Rev. Food Sci. Food Saf., 2019, 18(6), 2009–2024 CrossRef CAS
. - N. Castro-Bravo, et al., Interactions of Surface Exopolysaccharides From Bifidobacterium and Lactobacillus Within the Intestinal Environment, Front Microbiol., 2018, 9, 2426 CrossRef PubMed
. - D. Jeong, et al., Modulation of gut microbiota and increase in fecal water content in mice induced by administration of Lactobacillus kefiranofaciens DN1, Food Funct., 2017, 8(2), 680–686 RSC
. - P. R. Sivashankari and M. Prabaharan, Bioactive nanomaterials/chitosan composites as scaffolds for tissue regeneration, in Polysaccharide Carriers for Drug Delivery, ed. S. Maiti and S. Jana, Woodhead Publishing, 2019, ch. 19, pp. 559–584 Search PubMed
. - S. Stratton, et al., Bioactive polymeric scaffolds for tissue engineering, Bioact. Mater., 2016, 1(2), 93–108 CrossRef PubMed
. - N. B. Shelke, R. K. Nagarale and S. G. Kumbar, Polyurethanes, in Natural and Synthetic Biomedical Polymers, S. G. Kumbar, C. T. Laurencin and M. Deng, Elsevier, Oxford, 2014, ch. 7, pp. 123–144 Search PubMed
. - Z. Shariatinia and A. Barzegari, Polysaccharide hydrogel films/membranes for transdermal delivery of therapeutics, in Polysaccharide Carriers for Drug Delivery, ed. S. Maiti and S. Jana, Woodhead Publishing, 2019, ch. 22, pp. 639–684 Search PubMed
. - R. Bhosale, R. Osmani and A. Mmmm, Review on natural polysaccharide based particulate drug delivery systems: an inimitable tactic in novel drug delivery systems, Int. J. Curr. Pharm. Rev. Res., 2015, 6, 8–21 CrossRef
.
|
This journal is © The Royal Society of Chemistry 2020 |
Click here to see how this site uses Cookies. View our privacy policy here.