DOI:
10.1039/D0RA02798G
(Paper)
RSC Adv., 2020,
10, 19534-19541
Discovery of hydrazide-based pyridazino[4,5-b]indole scaffold as a new phosphoinositide 3-kinase (PI3K) inhibitor for breast cancer therapy†
Received
26th March 2020
, Accepted 28th April 2020
First published on 21st May 2020
Abstract
Herein, the mono and dialkylation of pyridazino[4,5-b]indole were achieved with a set of alkylating agents, including amyl bromide, allyl bromide, benzyl bromide and ethyl chloroacetate in the presence of K2CO3/acetone or KOH/DMSO. The hydrazinolysis of mono and di-esters 10 and 11 gave the target hydrazides 12 and 13, which displayed promising, potent, and significant cytotoxic activity against the MCF-7 cell line with IC50 values of 4.25 and 5.35 μm compared to that of the standard drug 5-FU (IC50 6.98 μm), respectively. RT-PCR analysis of the most active compound 12 was performed to determine its mode of action through the up-regulation of pro-apoptotic genes and inhibition of anti-apoptotic and PI3K/AKT/mTOR genes. The findings were consistent with the proposed mechanism illustrated in the in silico study. Further, the in vivo analysis exhibited its potent anti-cancer activity through the prolongation of survival parameters, and inhibition of ascetic fluid parameters in EAC-bearing mice.
1. Introduction
Targeted drug therapy for cancer treatment is one of the most important challenges in the new era because traditional cancer treatments have adverse side effects and drug resistance problems, resulting in the urgent need for safer, more effective targeted drug therapies with less side effects, which from an economical point of view is still a major challenge.1
One of the targeted receptors that play a significant role in cancer treatment is phosphatidylinositol 3-kinase (PI3K),2 which is a lipid kinase and plays a crucial role in the signaling pathway of the PI3K/AKT/mTOR genes.3,4 In diseases such as cancer and diabetes, the PI3K pathway has a high impact on the cell growth of cancer, motility, survival and metabolism. Thus, the design and development of drugs that may work as an inhibitor of PI3K singling in tumor therapy are highly interesting. Pyridazino[4,5-b]indol-4-ones and its analogues display significance in cancer therapy via the inhibition of DYRK1A5 and PI3Kα in various human cancer cell lines.6 Additionally, this interesting scaffold has been discovered to exhibit other pharmacological features such as HIV-1 reverse transcriptase inhibition,7 and blood platelet aggregation,8 as a selective thromboxane synthetase, and anti-hypertensiveness9,10 and antimicrobial activities.11,12 Indeed, pyridazino[4,5-b]indoles are considered as aza-heterocycle alkaloids of both the β-carboline and γ-carboline scaffolds,8–10 which exhibit cytotoxic, mutagenic, and genotoxic activities with high binding affinity to DNA.13
Several examples based on the carbazole scaffold show pharmacological features such as the SSR180575 analogue derived from pyridazino[4,5-b]indole, which showed highly potency and was a specific ligand for the PBR or TSPO receptor with high binding affinity (Kd = 1.95 ± 0.22 and 4.58 ± 0.83 nM, respectively).14,15 Harmane is a β-carboline alkaloid, which has been discovered to be an anti-tumor agent with high binding affinity to DNA and works as a Topo I inhibitor.16 Mahanine is another example derived from the carbazole scaffold, which is utilized for the treatment of breast cancer and subtype cell lines via the activation of caspases and AKT deactivation.17,18 Additionally, ellipticine is considered to be a potent antineoplastic agent,19 as shown in Fig. 1.
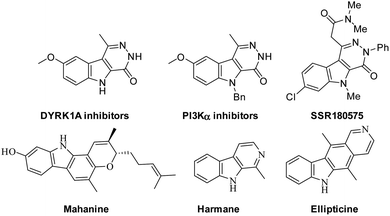 |
| Fig. 1 Bioactive compounds containing indole with other rings. | |
Based on the above findings and in continuation of our research on the discovery and development of novel anti-cancer leads,20–22 the present study, we established new pyridazino[4,5-b]indole derivatives and evaluated them against a breast cancer cell line (in vitro, in vivo and in silico). Two hits showed potent inhibition activity against phosphatidylinositol 3-kinase. These leads are expected to be future drugs and efficient for further investigations.
2. Results and discussion
2.1. Synthesis and characterization
The reaction of ethyl 3-formyl-1H-indole-2-carboxylate 1 with hydrazine hydrate in ethanol via reflux afforded 3,5-dihydro-4H-pyridazino[4,5-b]indol-4-one 2 in good yield. The Michael addition of nucleophile 2 to acrylonitrile as the Michael acceptor in ethanol containing Et3N yielded the Michael adduct 3 in excellent yield (Scheme 1).
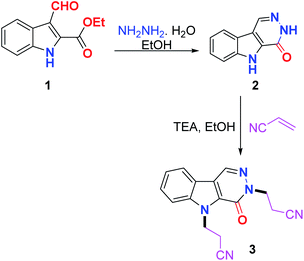 |
| Scheme 1 Synthesis of 2 and 3. | |
Alkylation of 2 with a set of alkylating agents, namely amyl bromide, allyl bromide and benzyl bromide in the presence of K2CO3 in acetone afforded a mixture of two products, which were separated by column chromatography and identified to include alkylation at the indole nitrogen 4–6 and alkylation at both the indole and pyridazine nitrogens 7–9. The bis(alkylated) products 7–9 were selectively obtained in excellent yields either from 2 or from the respective monoalkylated products 4–6 using KOH as a base in dimethyl sulfoxide as a solvent (Scheme 2).
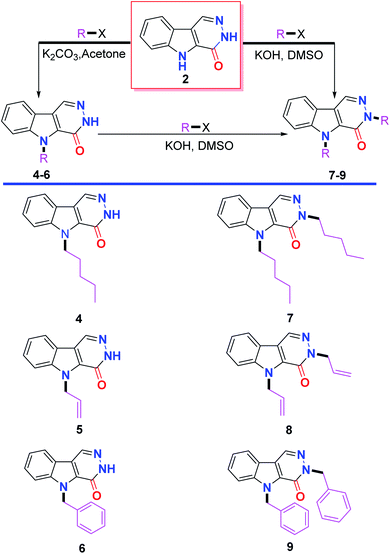 |
| Scheme 2 Alkylation of 3,5-dihydro-4H-pyridazino[4,5-b]indol-4-one 2. | |
Coupling of 2 with ethyl chloroacetate using K2CO3 in acetone afforded a mixture containing the monoester product 10 and the bis(ester) product 11, while, KOH/DMSO afforded the bis(ester) 11. Hydrazinolysis of the monoester 10 gave the monohydrazide 12, whereas, hydrazinolysis of the bis(ester) 11 afforded the bis-hydrazide 13 (Scheme 3).
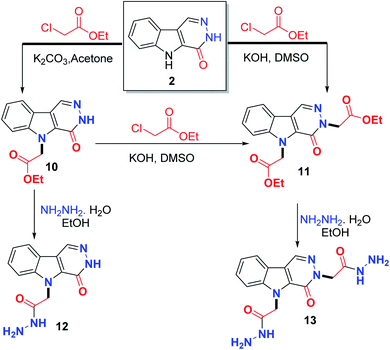 |
| Scheme 3 Synthesis of the target hit compounds 12 and 13. | |
2.2. Structural assignments
The NMR spectrum of 2 showed the four aromatic protons as two doublet of doublets and two doublets at δ 7.33, 7.51, 7.63 and 8.16 ppm. The pyridazine CH appeared as a singlet at δ 8.74 ppm, whereas, the indole and pyridazine NHs were found at δ 12.66 and 12.76 ppm, respectively. Its 13C NMR spectrum displayed the pyridazine C
O at 156.19 ppm.
The NMR spectrum of the Michael adduct 3 did not show any NH signal, additionally the four methylene triplet signals appeared at δ 3.08, 3.15, 4.49 and 5.06 ppm. The methylene carbons appeared at δ 17.04, 19.39, 40.79, and 46.26 ppm, respectively, which confirm that the reaction proceed via aza-Michael not oxa-Michael addition.
The NMR spectra of the mono-alkylated products 4–6 and 10 showed only one NH signal at around δ 12.80 ppm, proving the alkylation of the other NH. The N-alkylation was deduced from the methylene carbon signal which was found in the 13C NMR spectra of the abovementioned compounds at δ 44.74, 46.93, 47.86 and 45.99 ppm, respectively.
The dialkylated products 7–9, and 11 did not exhibit any NH signals, which supports the dialkylation. Both alkylations were achieved by alkylation at the indole and pyridazine nitrogens. This result was obvious from 13C NMR spectra, which displayed the methylene carbon signals directly attached to the indole and pyridazine nitrogens at 44.70 and 50.38 ppm in 7, δ 46.93 and 52.95 ppm in 8, δ 47.87 and 54.04 in 9 and δ 46.47 and 52.86 ppm in 11, respectively.
The NMR spectrum of our targeted hit 12 revealed that the hydrazide (–CONHNH2) signals were D2O exchangeable, which appeared at δ 4.26 ppm for NH2 and 9.35 ppm for NH. The carbonyl carbon of the hydrazide group was observed at δ 167.00 ppm. The structure of the other hit molecule 13 was deduced from the exchangeable signals of the two hydrazino groups at 4.12 ppm for 2NH2 and δ 9.23 and 9.34 ppm for the two NH. The carbonyl carbons were detected at δ 166.96 ppm.
2.3. In vitro results
2.3.1. Cytotoxic screening against two cancer cell lines and normal cell line. The cytotoxic activity of the synthesized derivatives was screened against two cancer cell lines, MCF-7 and HepG2, and non-cancer cell line, GMSC, to investigate their activity and safety. The results illustrated in Table 1 reveal potential cytotoxic activity, especially for compounds 12 and 13 with IC50 values of 4.24 and 5.35 μM, respectively, compared to 5-FU (6.98 μM) against the MCF-7 cell line, as shown in Fig. 2. Some derivatives, including 2, 10 and 11 showed moderate activity with IC50 values of 9.43, 12.4, and 9.07 μM, respectively. On the other hand, the tested derivatives showed a weak to moderate cytotoxic effect against the HepG2 cell line. In the trial to investigate the safety of the treatment with the tested compounds, they were found to be non-toxic against the normal cell line GMSC with non-detectable or high IC50 values. The structure–activity relationship of the potent hits 12 and 13 is attributed to the presence of the hydrazide group attached to pyridazino[4,5-b]indol-4-one, which provides hydrogen bond acceptors and hydrogen bond donors that best fit with the molecular targets, and thus this type of hit is expected to act as an interesting pharmacophore for anti-tumor activity.
Table 1 Cytotoxic activity against MCF-7 and HepG2 cancer cell lines and a normal cell linea
Compound |
IC50 (μM) |
MCF-7 |
HepG2 |
GMSC |
ND = not determined. |
2 |
9.43 |
22.78 |
ND |
3 |
ND |
>50 |
ND |
5 |
>50 |
>50 |
>50 |
6 |
29.51 |
ND |
>50 |
8 |
16.99 |
ND |
ND |
9 |
>50 |
ND |
ND |
10 |
12.4 |
36.67 |
>50 |
11 |
9.07 |
18.76 |
>50 |
12 |
4.25 |
12.75 |
>50 |
13 |
5.35 |
ND |
>50 |
5-FU |
6.98 |
ND |
>50 |
 |
| Fig. 2 Sigmoidal IC50 calculation curve for the most promising derivatives against the MCF-7 cell line. | |
2.3.2. RT-PCR results. Compound 12 was found to be the most active member in this series with an IC50 value of 4.25 μM against the MCF-7 breast cancer cell line. To control the apoptosis, both the intrinsic and extrinsic genes were investigated by gene expression analysis. The following genes including pro-apoptotic (P53, Bax, MDM2, and PUMA) and anti-apoptotic (Bcl-2, PI3K, AKT, and mTOR) plus β-actin, as a housekeeping gene, were collected for real-time PCR analysis. Fig. 3 illustrates that compound 12 has potential in inducing the expression of pro-apoptotic genes, such as P53, MDM2, Bax, and PUMA, with fold changes of 5.75, 6.01, 3.39, and 6.88, respectively. Moreover, it inhibited the expression of anti-apoptotic genes, such as Bcl-2, PI3K, AKT, and mTOR, with fold changes of 0.38, 0.316, 0.283, and 0.44, respectively, relative to β-actin (fold change = 1) as a house-keeping gene. Thus, the up-regulation of pro-apoptotic genes and down-regulation of anti-apoptotic genes by compound 12 relative to the control indicate its induction of apoptosis through the inhibition of the PI3K/AKT/mTOR pathway in the treated breast cancer cells. Previous results demonstrated that 5H-pyridazino[4,5-b]indoles exhibit promising and significant anti-proliferative effects in various cell types through the PI3K/AKT/mTOR pathway.6
 |
| Fig. 3 RT-PCR analysis of (A) pro-apoptotic genes (P53, Bax, MDM2, and PUMA) and (B) anti-apoptotic genes (Bcl-2, PI3K, AKT, and mTOR) was performed after the MCF-7 cells were treated with compound 12 (5 μM) for 48 h. Red column represents that of the house-keeping gene (Fold change = 1). | |
The in silico study indicated that compound 12 could bind efficiently with the 1e7v protein, representing the PI3K/mTOR targets. Validation of this binding was assessed on the gene expression level through the down-regulation of PI3K, AKT and mTOR.
Besides down-regulation of the PI3K/AKT/mTOR pathway, this was further validated by the activation of both extrinsic and intrinsic pathways, as a set of P53 and BAX genes, which were significantly up-regulated, while the down-regulation of the anti-apoptotic gene BCL-2 gene is in agreement with the report by Khodair et al., 2019.23 Therefore, inhibition of the PI3K/AKT/mTOR pathway resulted in the mediation of apoptosis. Our idea of using the gene expression level for elucidating the PI3K/AKT/mTOR pathway agrees with the previous study Kattan et al., 2020,24 which linked the gene expression to the inhibition of the PI3K/AKT/mTOR pathway and apoptosis.
2.4. In vivo results
In vivo experiments were performed to test the activity of compound 12 against EAC-bearing mice, which were divided into three groups, EAC-bearing mice as the positive control, compound 12-treated, and 5-FU-treated EAC-bearing mice. For the analysis of the measured parameters, the survival analysis and anti-cancer efficacy of the ascetic peritoneal fluid of the mice at the end of the experiment duration (day 18) are discussed similarly as in the report by El Gohary et al.25
Treatment with compound 12 significantly increased the mean survival time (MST) to 17.2 days compared to 10 days in the EAC control, increased the life span (ILS%) by 72% and the mean survival ratio to (T/C%) 172% compared to the EAC control group. The 5-FU treatment group showed an increase in the survival parameters to 16.6 days, 66%, and 166%, respectively, as shown in Fig. 4A. Additionally, treatment with compound 12 inhibited the increase in body weight (IBW%) to 19.27% relative to the untreated EAC control values. An increase in life span is an essential criterion for anti-cancer efficiency and is directly related to a decrease in the number of viable cells and the accumulation of ascetic fluid.
 |
| Fig. 4 In vivo anti-tumor activity in 12-treated EAC-bearing mice. (A) Survival parameters, including mean survival time (MST), percentage increase in life span (ILS%), percentage MST ratio (T/C%), and percentage increase in body weight (IBW%). (B) Anti-tumor potential against ascetic fluid, including tumor volume, tumor cell count, packed cell volume (PCV%), percentage of viable cells, and cell growth inhibition (CGI%). | |
Fig. 4B shows the effects of compound 12 and 5-FU treatments on the ascetic peritoneal fluid of the EAC-bearing mice. Compound 12 treatment significantly decreased the tumor volume from 19.5 to 11.9 mL, packed cell volume (PCV%) from 36.72% to 19.32%, total tumor cell count from 19.9 to 9.8 × 107 cells per mL, percentage of viable cells from 91.9% to 59.6%, and body weight from 43.65% to 15.56% relative to the untreated EAC control. Accordingly, the percentage growth inhibition (CGI%) of compound 12 significantly increased to 50.74%, proving that its anti-cancer effectiveness is superior to that of 5-FU. Treatment with 5-FU decreased the tumor volume to 12.6 mL, packed cell volume (PCV%) to 23.78%, total tumor cell count to 12.2 × 107 cells per mL, and percentage of viable cells to 68.46%. Accordingly, its percentage growth inhibition (CGI%) significantly increased to 38.67%. The decrease in the parameters of ascetic peritoneal fluid may be due to the anti-proliferative action against EAC cells. The in vivo results followed the same trend for the elucidation of the anti-cancer activity as that by Medhat et al., 2017.26
According to the in vivo results, hit 12 exhibited better activity than 5-FU, which in accordance with the in vitro results.
2.5. In Silico study
The synthesized compounds were docked for their binding affinities towards phosphoinositide 3-kinase inhibition (PDB: 1e7v) as a molecular target.27 According to the in silico results, the following molecules, 2, 10, 11, 12, and 13, have similar interaction inside the pocket matching with the co-crystallized ligand via the amino acid Val882, with good binding energies (−10.6 to −18.87 kcal mol−1) and also exhibit good lipophilic interaction inside the pocket of the target protein with Ile 881, Val 882, Met 953, Ile 879, Met 804, Trp 812, Phe 961 and Ile 963, as illustrated in the 2D images in Table 1, ESI.† The other derivatives did not show this type of interaction with this receptor binding site. Therefore, the molecular docking study demonstrates the same perspective as the RT-PCR results to give an overview for the mode of action of compound 12 against MCF-7 cells, which may be due to the inhibition of the PI3K/AKT/mTOR pathway (Fig. 5).
 |
| Fig. 5 Binding disposition and ligand–receptor interactions of the co-crystallized ligand (orange) and docked compounds (green). (A) compound 12 and (B) compound 13. | |
3. Experimental
General: all general information about the apparatus used to confirm the chemical structure assignment can be found in the ESI.†
3.1. Synthesis of the target compounds
3.1.1. Synthesis of 3,5-dihydro-4H-pyridazino[4,5-b]indol-4-one 2. A mixture of 1 (1.0 mmol) and NH2NH2·H2O (2.0 mmol) was refluxed in 5 mL ethanol for 3 h, and left to cool, and the formed crystals were collected by filtration and recrystallized from ethanol.
3.1.2. 3,5-Dihydro-4H-pyridazino[4,5-b]indol-4-one 2. Yield: 75%, mp 322–323 °C [Lit. 324–326 °C
10]. 1H NMR (400 MHz, DMSO-d6) δ 7.33 (dd, 1H, J 7.2, 7.6 Hz), 7.53 (dd, 1H, J 7.2, 8.0 Hz), 7.63 (d, 1H, J 8.4 Hz), 8.16 (d, 1H, J 8.0 Hz), 8.74 (s, 1H, CH), 12.66 (s, H, NH), 12.76 (s, H, NH); 13C NMR (100 MHz, DMSO-d6): δ 113.45, 117.98, 121.26, 121.79, 121.89, 127.44, 132.14, 133.78, 139.39, 156.19; CHN analysis calc. for C10H7N3O [185.0]: C, 64.86; H, 3.81; N, 22.69 found: C, 64.66; H, 3.91; N, 22.55.
3.1.3. Synthesis of 3,3′-(4-oxo-3H-pyridazino[4,5-b]indole-3,5(4H)-diyl)dipropanenitrile 3. A mixture of 2 (1.0 mmol) and acrylonitrile (2.2 mmol) in 10 mL ethanol containing Et3N (3 mmol) was refluxed for 4 h, and left to cool at room temperature, and the precipitate was collected by filtration, dried and recrystallized from ethanol. Yield: 89%, mp 197–198 °C. 1H NMR (400 MHz, DMSO-d6) δ 3.08 (t, 2H, J 6.4 Hz, CH2), 3.15 (t, 2H, J 6.4 Hz, CH2), 4.49 (t, 2H, J 6.4 Hz, CH2), 5.06 (t, 2H, J 6.4 Hz, CH2), 7.42 (dd, 1H, J 7.2, 8.0 Hz), 7.62 (dd, 1H, J 7.2, 8.4 Hz), 7.94 (d, 1H, J 8.4 Hz), 8.21 (d, 1H, J 8.0 Hz), 8.85 (s, 1H, CH); 13C NMR (100 MHz, DMSO-d6): δ 17.04 (CH2), 19.39 (CH2), 40.79 (NCH2), 46.26 (NCH2), 112.03, 118.29, 118.73, 118.92, 120.38, 122.05, 122.76, 128.05, 129.89, 133.72, 140.04, 155.12; CHN analysis calc. for C16H13N5O [291.1]: C, 65.97; H, 4.50; N, 24.04 found: C, 66.01; H, 4.44; N, 23.99.
3.2. General procedure for the alkylation
3.2.1. Method A. A mixture of 2 (1.0 mmol) and K2CO3 (2.2 mmol) in a mixture of acetone/DMF (10: 2 mL) was stirred at room temperature for 1 h, then the appropriate alkyl halide (2.2 mmol) was added dropwise, and stirring was continued overnight. Then the solvent was evaporated under vacuum, water was added, and the precipitate was filtered. The product was purified via column chromatography using silica and 2
:
8 EA/n-hexane.
3.2.2. Method B. A mixture of 2 (1.0 mmol) and KOH (2.2 mmol) in 10 mL DMSO was stirred at room temperature for 1 h, and then the appropriate alkyl halide (2.2 mmol) was added dropwise, and subsequently stirred overnight (monitored by TLC until all 2 was converted to the dialkylated product). Then water was added, the precipitate was filtered and the products were purified either by recrystallization from ethanol or column chromatography using silica and 2
:
8 EA/n-hexane.
3.2.3. 5-Pentyl-3,5-dihydro-4H-pyridazino[4,5-b]indol-4-one 4. Yield: 44%Method A, mp 161–162 °C. 1H NMR (400 MHz, DMSO-d6) δ 0.82 (t, 3H, J 7.2 Hz, CH3), 1.23–1.32 (m, 4H, 2 CH2), 1.77–1.84 (m, 2H, CH2), 4.80 (t, 2H, J 7.2 Hz, NCH2), 7.37 (dd, 1H, J ≈ 7.6 Hz), 7.58 (dd, 1H, J 8.0, 7.6 Hz), 7.79 (d, 1H, J 8.4 Hz), 8.20 (d, 1H, J 8.0 Hz), 8.74 (s, 1H), 12.72 (s, 1H, NH); 13C NMR (100 MHz, DMSO-d6): δ 14.19 (CH3), 18.79 (CH2), 22.20 (CH2), 28.60 (CH2), 30.38 (CH2), 44.74 (NCH2), 111.84, 117.84, 120.59, 122.01, 127.56, 130.54, 133.58, 139.75, 156.46; CHN analysis calc. for C15H17N3O [255.1]: C, 70.56; H, 6.71; N, 16.46 found: C, 70.44; H, 6.73; N, 16.33.
3.2.4. 5-Allyl-3,5-dihydro-4H-pyridazino[4,5-b]indol-4-one 5. Yield: 35%Method A, mp 237–238 °C. 1H NMR (400 MHz, DMSO-d6) δ 4.92 (dd, 1H, Jtrans 17.1, 1.4 Hz, Htrans), 5.12 (dd, 1H, Jcis 10.3, 1.3 Hz, Hcis), 5.48 (d, 2H, J 5.2 Hz, NCH2), 6.02–6.08 (m, 1H, –CH
CH2), 7.38 (dd, 1H, J 7.3, 7.6 Hz), 7.58 (dd, 1H, J 8.1, 8.2 Hz), 7.79 (d, 1H, J 8.4 Hz), 8.22 (d, 1H, J 8.0 Hz), 8.78 (s, 1H), 12.83 (s, 1H, NH); 13C NMR (100 MHz, DMSO-d6): δ 46.93 (NCH2), 112.14, 117.04, 117.97, 120.61, 122.08, 122.26, 127.66, 130.29, 133.74, 134.50, 139.70, 156.49; CHN analysis calc. for C13H11N3O [225.1]: C, 69.32; H, 4.92; N, 18.66 found: C, 69.21; H, 4.79; N, 18.59.
3.2.5. 5-Benzyl-3,5-dihydro-4H-pyridazino[4,5-b]indol-4-one 6. Yield: 51%Method A, mp 231–232 °C. 1H NMR (400 MHz, DMSO-d6) δ 6.10 (s, 2H, NCH2), 7.37–7.30 (m, 5H), 7.37 (dd, 1H, J 7.6, 7.4 Hz), 7.54 (dd, 1H, J ≈ 7.7 Hz), 7.75 (d, 1H, J 8.40 Hz), 8.23 (d, 1H, J 8.0 Hz), 8.82 (s, 1H), 12.92 (s, 1H, NH); 13C NMR (150 MHz, DMSO-d6): δ 47.86 (NCH2), 112.33, 118.16, 120.81, 122.17, 122.39, 127.58, 127.80, 127.89, 129.07, 130.34, 133.86, 138.19, 139.69, 156.70; CHN analysis calc. for C17H13N3O [275.1]: C, 74.17; H, 4.76; N, 15.26 found: C, 74.20; H, 4.86; N, 15.24.
3.2.6. 3,5-Dipentyl-3,5-dihydro-4H-pyridazino[4,5-b]indol-4-one 7. Yield: 29% Method A, 71%Method B, oil. 1H NMR (400 MHz, DMSO-d6) δ 0.82 (t, 3H, J 6.8 Hz, CH3), 0.87 (t, 3H, J 6.8 Hz, CH3), 1.24–1.37 (m, 8H, 4 CH2), 1.78–1.81 (m, 2H, CH2), 4.22 (t, 2H, J 7.2 Hz, NCH2), 4.82 (t, 2H, J 7.2 Hz, NCH2), 7.38 (dd, 1H, J 7.2, 7.6 Hz), 7.58 (dd, 1H, J 7.2, 8.0 Hz), 7.79 (d, 1H, J 8.4 Hz), 8.20 (d, 1H, J 8.0 Hz), 8.76 (s, 1H); 13C NMR (100 MHz, DMSO-d6): δ 14.22 (CH3), 22.20 (CH3), 28.47 (CH2), 28.61 (CH2), 28.74 (CH2), 30.41 (CH2), 44.70 (NCH2), 50.38 (NCH2), 111.88, 117.42, 120.36, 121.95, 122.14, 127.59, 130.54, 130.23, 132.92, 140.05, 155.10; CHN analysis calc. for C20H27N3O [325.2]: C, 73.81; H, 8.36; N, 12.91 found: C, 73.91; H, 8.31; N, 12.90.
3.2.7. 3,5-Diallyl-3,5-dihydro-4H-pyridazino[4,5-b]indol-4-one 8. Yield: 33% Method A, 69%Method B, oil. 1H NMR (600 MHz, DMSO-d6) δ 4.83 (d, 2H, J 5.5 Hz, NCH2), 4.93 (d, 1H, Jtrans 17.1, Htrans), 5.11 (d, 1H, Jcis 10.1 Hz, Hcis), 5.13 (d, 1H, Jtrans 17.0 Hz, Htrans), 5.19 (d, 1H, Jcis 10.3 Hz, Hcis), 5.50 (d, 2H, J 5.0 Hz, NCH2), 6.01–6.07 (m, 2H, 2 –CH
CH2), 7.40 (dd, 1H, J 7.3, 7.7 Hz), 7.59 (dd, 1H, J 8.2, 7.2 Hz), 7.73 (d, 1H, J 8.4 Hz), 8.23 (d, 1H, J 7.9 Hz), 8.82 (s, 1H); 13C NMR (150 MHz, DMSO-d6): δ 46.93 (NCH2), 52.95 (NCH2), 112.21, 117.08, 117.66, 117.71, 120.40, 122.08, 122.43, 127.78, 129.96, 133.41, 133.83, 134.47, 140.03, 154.91; CHN analysis calc. for C16H15N3O [265.1]: C, 72.43; H, 5.70; N, 15.84 found: C, 72.46; H, 5.84; N, 15.93.
3.2.8. 3,5-Dibenzyl-3,5-dihydro-4H-pyridazino[4,5-b]indol-4-one 9. Yield: 41%Method A, 86%Method B mp 147–148 °C. 1H NMR (400 MHz, DMSO-d6) δ 5.45 (s, 2H, NCH2), 6.10 (s, 2H, NCH2), 7.22–7.40 (m, 11H), 7.55 (m, 1H), 7.76 (d, 1H, J 8.5 Hz), 8.23 (d, 1H, J 8.0 Hz), 8.88 (s, 1H); 13C NMR (100 MHz, DMSO-d6): δ 47.87 (NCH2), 54.04 (NCH2), 112.04, 117.99, 120.57, 122.15, 122.62, 127.47, 127.84, 127.99, 128.15, 128.19, 128.93, 129.07, 129.10, 130.08, 133.69, 138.03, 138.12, 140.07, 155.38; CHN analysis calc. for C24H19N3O [365.1] C, 78.88; H, 5.24; N, 11.50 found: C, 78.99; H, 5.44; N, 11.44.
3.2.9. Ethyl 2-(4-oxo-3,4-dihydro-5H-pyridazino[4,5-b]indol-5-yl)acetate 10. Yield: 48%Method A, mp 255–256 °C. 1H NMR (600 MHz, DMSO-d6) δ 1.22 (t, 3H, J 7.0 Hz, CH3) 4.17 (q, 2H, J 7.0 Hz, CH2), 5.68 (s, 2H, NCH2), 7.41 (dd, 1H, J 7.4, 7.6 Hz), 7.58 (dd, 1H, J 7.6, 7.9 Hz), 7.80 (d, 1H, J 8.40 Hz), 8.23 (d, 1H, J 7.9 Hz), 8.81 (s, 1H), 12.87 (s, 1H, NH); 13C NMR (150 MHz, DMSO-d6): δ 14.1 (CH3), 45.99 (NCH2), 61.57 (OCH2), 111.97, 121.88, 127.23, 127.80, 132.49, 133.17, 140.47, 148.48, 168.61 (C
O); CHN analysis calc. for C14H13N3O3 [271.1] C, 61.99; H, 4.83; N, 15.49 found: C, 62.03; H, 4.86; N, 15.48.
3.2.10. Diethyl 2,2′-(4-oxo-3H-pyridazino[4,5-b]indole-3,5(4H)-diyl)diacetate 11. Yield: 38%Method A, 75%Method B, mp 153–154 °C. 1H NMR (400 MHz, DMSO-d6) δ 1.18–1.22 (m, 6H, 2 CH3) 4.12–4.19 (m, 4H, 2 CH2), 4.99 (s, 2H, NCH2), 5.66 (s, 2H, NCH2), 7.43 (dd, 1H, J 7.4, 7.6 Hz), 7.61 (dd, 1H, J 7.2, 8.3 Hz), 7.81 (d, 1H, J 8.4 Hz), 8.25 (d, 1H, J 7.8 Hz), 8.87 (s, 1H); 13C NMR (100 MHz, DMSO-d6): δ 14.44, 14.47 (2 CH3), 46.47 (NCH2), 52.86 (NCH2), 61.51 (OCH2), 61.68 (OCH2), 111.89, 118.34, 120.28, 122.14, 122.81, 128.16, 130.18, 133.81, 140.67, 155.38, 168.41 (C
O), 168.83 (C
O); CHN analysis calc. for C18H19N3O5 [357.1] C, 60.50; H, 5.36; N, 11.76 found: C, 60.64; H, 5.37; N, 11.73.
3.3. General procedures for hydrazinolysis
To the selected ester 10 or 11 in 10 mL ethanol, hydrazine hydrate (2.0 mmol10 or 4.0 mmol11) was added and refluxed for 2 h until all the esters were consumed (reaction completion was monitored by TLC), and then the reaction mixture was left to cool, and the formed precipitate was filtered, dried and recrystallized from ethanol.
3.3.1. 2-(4-Oxo-3,4-dihydro-5H-pyridazino[4,5-b]indol-5-yl)acetohydrazide 12. Yield: 91%, mp 280decomp. °C. 1H NMR (400 MHz, DMSO-d6) δ 4.26 (brs, 2H, NH2, D2O exchangeable), 5.49 (s, 2H, NCH2), 7.37–7.63 (m, 3H), 8.21 (d, 1H, J 7.6 Hz), 8.77 (s, 1H), 9.35 (brs, 1H, NH, D2O exchangeable), 12.76 (s, 1H, NH, D2O exchangeable); 13C NMR (100 MHz, DMSO-d6): δ 14.1 (CH3), 46.20 (NCH2), 111.80, 118.07, 120.69, 121.96, 122.28, 127.61, 131.12, 133.76, 140.52, 156.71, 167.00 (C
O); CHN analysis calc. for C12H11N5O2 [257.1] C, 56.03; H, 4.31; N, 27.22 found: C, 56.11; H, 4.31; N, 27.12.
3.3.2. 2,2′-(4-Oxo-3H-pyridazino[4,5-b]indole-3,5(4H)-diyl)di(acetohydrazide) 13. Yield: 89%, mp 293–294 °C. 1H NMR (400 MHz, DMSO-d6) δ 4.26 (brs, 4H, 2 NH2, D2O exchangeable), 4.79 (s, 2H, NCH2), 5.81 (s, 2H, NCH2), 7.39–7.65 (m, 3H), 8.22 (d, 1H, J 8 Hz), 8.79 (s, 1H), 9.23 (brs, 1H, NH, D2O exchangeable), 9.34 (brs, 1H, NH, D2O exchangeable); 13C NMR (100 MHz, DMSO-d6): δ 46.17 (NCH2), 52.36 (NCH2), 111.95, 117.97, 120.49, 121.93, 122.46, 127.72, 130.69, 133.39, 140.82, 155.55, 166.96 (2C
O); CHN analysis calc. for C14H15N7O3 [329.1] C, 51.06; H, 4.59; N, 29.77 found: C, 51.13; H, 4.55; N, 29.76.
3.4. Biological evaluation
The cytotoxic screening using the MTT assay,28,29 and RT-PCR23 are provided in the ESI.†
3.5. In vivo protocol
All mice were purchased from the Laboratory of the Faculty of Veterinary Medicine, Suez Canal University. The EAC cells were purchased from the National Cancer Institute, Cairo, Egypt. In our protocol, we used a safe concentration of DMSO (5 μL/25 g for each mouse), and the treated doses were freshly prepared.
The in vivo experiment regarding the implantation of Ehrlich Ascites Carcinoma (EAC), experiment design, and the measurement of the parameters in this study were performed according to Nafie et al., 2020.30 and Salem et al., 2016.31 (see ESI†). All animal procedures were performed in accordance with the Guidelines for the Care and Use of Laboratory Animals of Suez Canal University and approved by the Animal Ethics Committee of the Faculty of Science. From day zero of the experiment, the mortality and body weight of the mice were monitored daily for the survival analysis (mean survival time (MST), percentage of MST ratio (T/C%), percentage increase in life span (ILS%), and increase in body weight (IBW%)). At the end of the experiment, on day 18, the mice were anesthetized and sacrificed to determine the anti-cancer potentiality of the compounds (tumor volume, packed cell volume (PCV%), tumor cell count, trypan blue exclusion assay,32 and percentage cell growth inhibition (CGI%)).
3.6. In silico
All parameters and software used for the molecular docking study are provided in the ESI.†26,32–34
4. Conclusions
In summary, a series of new mono and dialkylated pyridazino[4,5-b]indol-4-ones 4–10 were synthesized. All the alkylation reactions were found to proceed via N-alkylation. Hydrazinolysis of the mono and di-esters 10 and 11 afforded the target hits mono- and bis-hydrazides 12 and 13, respectively. The hit compound 12 exhibited interesting PI3K inhibition activity, as supported by the in vitro, in vivo and in silico results.
Conflicts of interest
The authors declare no conflict of interest.
Acknowledgements
The authors would like to extend their sincere appreciation to the Researchers Supporting project number (RSP-2019/64), King Saud University, Riyadh, Saudi Arabia.
References
- M. Sriram, J. J. Hall, N. C. Grohmann, T. E. Strecker, T. Wootton, A. Franken, M. L. Trawick and K. G. Pinney, Bioorg. Med. Chem., 2008, 16, 8161–8171 CrossRef CAS PubMed.
- A. Akinleye, P. Avvaru, M. Furqan, Y. Song and D. Liu, J. Hematol. Oncol., 2013, 6, 88 CrossRef PubMed.
- J. C. Chamcheu, T. Roy, M. B. Uddin, S. Banang-Mbeumi, R.-C. N. Chamcheu, A. L. Walker, Y.-Y. Liu and S. Huang, Cells, 2019, 8, 803 CrossRef CAS PubMed.
- C. Porta, C. Paglino and A. Mosca, Front. Oncol., 2014, 4, 64 Search PubMed.
- A. Bruel, R. Bénéteau, M. Chabanne, O. Lozach, R. Le Guevel, M. Ravache, H. Bénédetti, L. Meijer, C. Logé and J.-M. Robert, Bioorg. Med. Chem. Lett., 2014, 24, 5037 CrossRef CAS PubMed.
- A. Bruel, C. Logé, M. L. de Tauzia, M. Ravache, R. Le Guevel, C. Guillouzo, J.-F. Lohier, J. S. Oliveira Santos, O. Lozach, L. Meijer, S. Ruchaud, H. Bénédetti and J.-M. Robert, Eur. J. Med. Chem., 2012, 57, 225 CrossRef CAS PubMed.
- M. Font, A. Monge, A. Cuartero, A. Elorriaga, J. J. MartinezIrujo, E. Alberdi, E. Santiago, I. Prieto, J. J. Lasarte, P. Sarobe and F. Borras, Drug Des. Discovery, 1997, 14, 305 CAS.
- A. Monge, M. I. Aldana, T. Alvarez, M. Font, E. Santiago, J. A. Latre, M. J. Bermejillo and M. J. Lopez-Unzu, J. Med. Chem., 1991, 34, 3023 CrossRef CAS.
- A. Monge, P. Parrado, M. Font and E. Fernandezalvarez, J. Med. Chem., 1987, 30, 1029 CrossRef CAS PubMed.
- A. El-Gendy and H. El-Banna, Arch. Pharmacal Res., 2001, 24, 21 CrossRef CAS PubMed.
- I. Avan, A. Guven and K. Guven, Turk. J. Chem., 2013, 37, 271 CAS.
- A. El-Gendy, M. M. Said, N. Ghareb, Y. M. Mostafa and E. S. H. El Ashry, Arch. Pharm., 2008, 341, 294 CrossRef CAS PubMed.
- J. Chen, X. Dong, T. Liu, J. Lou, C. Jiang, W. Huang, Q. He, B. Yang and Y. Hu, Bioorg. Med. Chem., 2009, 17, 3324 CrossRef CAS PubMed.
- V. Vin, N. Leducq, F. Bono and J. M. Herbert, Biochem. Biophys. Res. Commun., 2003, 310, 785 CrossRef CAS PubMed.
- V. Papadopoulos, M. Baraldi, T. R. Guilarte, T. B. Knudsen, J. J. Lacapère, P. Lindemann, M. D. Norenberg, D. Nutt, A. Weizman, M.-R. Zhang and M. Gavish, Trends Pharmacol. Sci., 2006, 27, 402 CrossRef CAS PubMed.
- R. Cao, W. Peng, H. Chen, Y. Ma, X. Liu, X. Hou, H. Guan and A. Xu, Biochem. Biophys. Res. Comm., 2005, 338, 1557 CrossRef CAS PubMed.
- M. Das, R. Kandimalla, B. Gogoi, K. N. Dutta, P. Choudhury, R. Devi, P. P. Dutta, N. C. Talukdar and S. K. Samanta, Pharmacol. Res., 2019, 146, 104330 CrossRef CAS PubMed.
- S. Sinha, B. C. Pal, S. Jagadeesh, P. P. Banerjee, A. Bandyopadhaya and S. Bhattacharya, Prostate, 2006, 66, 1257 CrossRef CAS PubMed.
- M. Stiborová, J. Poljaková, E. Martínková, L. Bořek-Dohalská, T. Eckschlager, R. Kizek and E. Frei, Interdiscip. Toxicol., 2011, 4, 98 Search PubMed.
- A. T. A. Boraei, M. S. Gomaa, E. S. H. El Ashry and A. Duerkop, Eur. J. Med. Chem., 2017, 125, 360 CrossRef CAS PubMed.
- A. T. A. Boraei, H. A. Ghabbour, M. S. Gomaa, E. S. H. El Ashry and A. Barakat, Molecules, 2019, 24, 4471 CrossRef PubMed.
- A. T. A. Boraei, P. K. Singh, M. Sechi and S. Satta, Eur. J. Med. Chem., 2019, 182, 111621 CrossRef CAS PubMed.
- A. I. Khodair, M. A. Alsafi and M. S. Nafie, Carbohydr. Res., 2019, 486, 107832 CrossRef PubMed.
- S. W. Kattan, M. S. Nafie, G. A. Elmgeed, W. Alelwani, M. Badar and M. A. Tantawy, J. Steroid Biochem. Mol. Biol., 2020, 105604 CrossRef CAS PubMed.
- N. S. El-Gohary, M. T. Gabr and M. I. Shaaban, Bioorg. Chem., 2019, 89, 102976 CrossRef CAS PubMed.
- D. Medhat, J. Hussein, M. E. El-Naggar, M. F. Attia, M. Anwar, Y. A. Latif, H. F. Booles, S. Morsy, A. R. Farrag, W. K. B. Khalil and Z. El-Khayat, Biomed. Pharmacother., 2017, 91, 1006 CrossRef CAS PubMed.
- E. H. Walker, M. E. Pacold, O. Perisic, L. Stephens, P. T. Hawkins, M. P. Wymann and R. L. Williams, Mol. Cell, 2000, 6, 909–919 CrossRef CAS PubMed.
- T. Mosmann, J. Immunol. Methods, 1983, 65, 55–63 CrossRef CAS PubMed.
- R. I. Freshney, Culture of animal cells: a manual of basic technique and specialized applications, Wiley-Blackwell, Hoboken, N.J., 2010 Search PubMed.
- M. S. Nafie, N. K. Arafa, N. K. Sedky, A. A. Alakhdar and R. K. Arafa, Chem.-Biol. Interact., 2020, 324, 109087 CrossRef CAS PubMed.
- M. L. Salem, N. M. Shoukry, W. K. Teleb, M. M. Abdel-Daim and M. A. Abdel-Rahman, SpringerPlus, 2016, 5, 570 CrossRef PubMed.
- W. Strober, Curr. Protoc. Immunol., 2001, A.3B.1–A.3B.2 Search PubMed.
- D. C. Young, Computational Drug Design, John Wiley & Sons, Inc., Hoboken, NJ, USA, 2009 Search PubMed.
- M. S. Nafie, M. A. Tantawy and G. A. Elmgeed, Steroids, 2019, 152, 108485 CrossRef CAS PubMed.
Footnotes |
† Electronic supplementary information (ESI) available. See DOI: 10.1039/d0ra02798g |
‡ M. S. N. carried out the experimental and discussion of all the biological assays. |
|
This journal is © The Royal Society of Chemistry 2020 |
Click here to see how this site uses Cookies. View our privacy policy here.