DOI:
10.1039/D0RA02706E
(Paper)
RSC Adv., 2020,
10, 31502-31510
Fluorescence regional integration combined with parallel factor analysis to quantify fluorescencent spectra for dissolved organic matter released from manure biochars
Received
24th March 2020
, Accepted 18th August 2020
First published on 26th August 2020
Abstract
Dissolved Organic Matter (DOM) in biochars is important to carbon dynamics and contaminant transport in soils. Fluorescence excitation-emission matrices (EEMs) have been widely used to characterize dissolved organic matter (DOM). In this study, fluorescence regional integration (FRI) and parallel factor analysis (PARAFAC) applied to EEM allows good quantitative assessment of the composition of DOM derived from manure biochars. Manure biochars were produced using four types of manure, chicken, pig, cow, and sheep manure under various pyrolysis temperatures (300–600 °C) and holding times (0–120 min). The results from the determination of dissolved organic carbon (DOC), SUVA254, and humification index (HIX) reflected that high pyrolysis temperature and long holding time led to a significant decrease in DOM quantity, aromaticity and humification. The FRI result showed that the pyrolysis process of DOM released from manure biochars included three changes, aromatic protein-like substance and microbial by-product-like substance generation (300–600 °C), fulvic-acid like substance decomposition (300–500 °C) and humic acid-like substance decomposition (600 °C). The PARAFAC modeling result showed that the pyrolysis process of DOM released from manure biochars contained two changes: three high molecular-weight humic-like substances decomposition and a low molecular-weight humic-like substance generation. The effect of the holding time on biochar-DOM is more significant at higher pyrolysis temperatures than lower pyrolysis temperatures. The correlation analysis result revealed that the generation of aromatic proteins, microbial by-products and fulvic acid came from the decomposition of humic-like substances including marine humic-like, UVA humic-like, and UVC + UVA humic-like substances. The results obtained in this study would be beneficial to guide the rational production and application of manure biochars.
Introduction
With the development of animal husbandry, the output of livestock manure is increasing. Currently, China's annual output is approximately 4 billion tons. The chemical oxygen demand of livestock manure is 12.68 million tons, and there are many related pathogenic microorganisms, antibiotics, heavy metals and other pollutants. Therefore, if the excrement of livestock and poultry is left in the environment without treatment, it will present a serious burden to the environment. Manures can be considered as organic fertilizer for soil improvement due to their high content of nutrients, such as carbon, nitrogen, and phosphorus. Composting is a simple way to produce organic fertilizer from livestock and poultry manure.1,2 Composting uses microorganisms to ferment and degrade organic matter, kill pathogenic microorganisms and remove odours. However, composting cannot completely degrade antibiotics in manure,3 and a risk of heavy metal pollution remains.4 Therefore, methods for recycling the excrement of livestock and poultry more efficiently and in an environmentally friendly manner has remained a focus of scientific researchers.
In recent years, biochar has been paid increasing attention for soil amendment or wastewater treatment materials.5,6 Biochar is a form of organic matter with a high carbon content, aromaticity, and stability, which is produced under high temperatures and anaerobic conditions. Pyrolysis of animal manure produces biochar with multiple beneficial uses for improving soil quality and the environment. Compared with biochar from straw and lignin, biochar from livestock manure has a higher pH value and nutrients content,7 which can effectively counteract soil acidification and supplement nutrients to poor soil.
Dissolved Organic Matter (DOM) is a heterogeneous mixture of aromatic and aliphatic organic compounds containing oxygen, nitrogen, and sulphur functional groups. In terms of operation definition, DOM refers to the part of the mixture that can dissolve in water and cannot pass through a 0.45 μm filter membrane.8 The impact of DOM on ecosystems is very important because it involves multiple processes related to ecosystem balance. DOM is beneficial to the nutrient uptake of plants and promotes the biochemical cycle of carbon.9 Conversely, the migration or bioconcentration of pollutants can be promoted by DOM due to the strong complexation of DOM with organic pollutants or heavy metals.10,11 The environmental behaviour of DOM is closely related to the concentration and chemical composition of DOM. By tracking DOM, one can better predict the impact of DOM on natural processes. To obtain useful information about the production and application of biochar, it is necessary to characterize the DOM released from biochar. However, much plant biochar DOM has already been characterized.12–14 The composition of manure-derived biochar has not yet been understood. It is known that there are significant differences between the chemical composition of animal manure DOM and plant DOM.15 Accordingly, it is necessary to investigate the composition of DOM from manure biochar.
Fluorescence excitation-emission matrices (EEMs) spectroscopy is widely used to identify DOM in natural or engineering systems.16,17 The rich fluorescence information of EEMs can provide key composition information related to DOM. Fluorescence Regional Integration (FRI) was proposed to obtain quantification information of DOM EEMs.18 It was developed on the basis of the traditional peak method. According to the research purpose, the EEMs spectrum was artificially divided into different regions, and the change of fluorescent substances was quantitatively characterized by calculating the volume percentage of the given region. FRI can comprehensively analyse the information from the total fluorescence spectrum, and comparing the results of different studies is simple owing to the standardized division and nomenclature.18,19 The disadvantage of FRI is that it cannot solve the problem of overlapping fluorescence peaks. Parallel factor analysis (PARAFAC) can be used to deconvolute complex EEMs into the dominant fluorescent DOM components, which can realize chemometric separation of complex fluorescence spectra and give quantitative changes. It has been widely used to characterize DOM in natural ecosystem and laboratory samples.13,14,19 However, there is no uniform standard for the definition and name of fluorescent components in the PARAFAC modelling method, which makes it difficult to compare the results of different studies.
Therefore, this study intends to combine FRI and PARAFAC to characterize DOM released from manure derived biochar to get the quantitative information better. The main objective of this study is to explore the effect mechanism of various pyrolysis temperatures and holding times on the composition of the DOM of manure-derived biochar.
Materials and methods
Biochar production
Seven kinds of manure were selected as raw materials of biochar, which include two chicken manures, one pig manure, two cow manures, and two sheep manures. These manures were acquired from several farms in Meizhou City, Guangdong Province, China. Raw manures were air-dried naturally followed by drying at 105 °C in an oven. The dried manures were crushed and passed through a 10-mesh sieve. The prepared manures were pyrolyzed in a tubular furnace (OTL1200, Nanjing University Instrument Factory, China) with a heating rate of 10 °C min−1 and a nitrogen concentration of 500 cm3 min−1. 112 biochar samples were prepared at four pyrolysis temperatures (300 °C, 400 °C, 500 °C, 600 °C) and four holding times (0 min, 30 min, 60 min, 120 min). The biochars were stored in a vacuum dryer for subsequent determination.
DOC and UV-vis analyses
The DOM of 7 raw manures and 112 biochars were extracted by ultra-pure water with the water-solid ratio of 100
:
1 v/m. The mixtures were shaken in a water bath at 25 °C for 24 hours and then filtered with a 0.45 μm filter membrane. The DOM samples were analysed for dissolved organic carbon (DOC) using a TOC/TN analyser (TOC-VCPH, Shimadzu, Japan). UV-visible absorbance spectra on each DOM sample were collected using a UV-vis spectrometer (UV2000, Shimadzu, Japan), within 1 cm quartz cuvettes, with a wavelength of 200 to 600 nm, and Millipore water as the blank. The specific UV absorbance at 254 nm (SUVA254), as an indicator of aromaticity, was determined by dividing the UV absorbance measured at 254 nm by the sample's DOC concentration.
EEMs analysis
The DOM sample was diluted to 10 mg L−1 for the fluorescence determination. If the DOC concentration of DOM sample was lower than 10 mg L−1, the original solution was determined for the fluorescence determination. The fluorescence EEMs spectra of 119 DOM samples were determined using a fluorescence spectrophotometer (LS55, Perkin Elmer, USA). The scanning range of excitation spectrum and emission spectrum is 200–500 nm and 300–600 nm, respectively.
The excitation wavelengths and emission wavelengths were incrementally increased at 10 nm and 0.5 nm steps, respectively. The pH of all DOM samples was adjusted to 7 by adding 0.1 M HCl or NaOH.
The data of the EEM spectra were analysed by FRI which delineated EEM into five excitation-emission regions based on the fluorescence of model compounds.18 Fluorescence intensity was integrated beneath each of the five EEM regions. The percent fluorescence response (Pi,n) were calculated for reference.18
The data of the EEM spectra were analysed by PARAFAC modelling. The excitation wavelengths were restricted in the range of 250–500 nm for PARAFAC analysis. The PARAFAC modelling was conducted with MATLAB 2016a software with DOM Fluor toolbox (V1.7).19 The models were based on the fluorescence intensity and proportional distribution of the four PARAFAC components.
Results and discussion
DOC, SUVA254, and HIX
DOC. The effect of pyrolysis temperature and holding time on DOC concentration was shown in Fig. 1. The DOC concentration of seven raw manures differed within the range of 4.57–18.70 g C kg−1 of manure. The average DOC concentration was 10.08 g C kg−1 of manure. The biochar-DOC concentrations were below the average manure-DOC concentration. ANOVA analysis showed that there was a significant effect of pyrolysis temperature and holding time on the DOC concentration of biochar (p < 0.05). When the holding time was 0 min, the effect of pyrolysis temperature on the DOC concentration was small. When the holding time was more than 30 min, DOC concentration decreased significantly with pyrolysis temperature increasing. This indicated that the decomposition of manure DOM required a specific period under any pyrolysis temperature. When the pyrolysis temperature was higher than 400 °C, the DOC concentration decreased significantly with the increase of holding time. At a pyrolysis temperature of 600 °C, the DOC concentration changed a little between 30–120 min holding time, which showed that the DOM component decomposed quickly and achieved stability at 600 °C. The DOC concentration of DOM released from high temperature biochar was lower than the DOM released from low temperature biochar, which has been reported in many studies.13,20,21
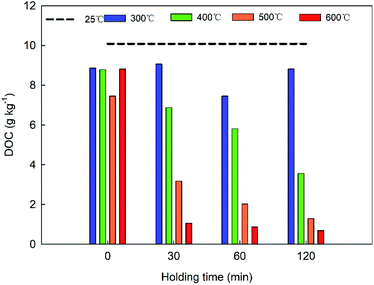 |
| Fig. 1 The concentration of DOC released from biochar produced under different pyrolysis conditions (25 °C-raw manure). | |
SUVA254. The effect of pyrolysis temperature and holding time on DOM SUVA254 was shown in Fig. 2. SUVA254 has a significant positive correlation with the aromatic carbon in DOM, which is used to indicate the aromaticity of DOM.22,23 The SUVA254 value of DOM released from raw manure was between 4.75–7.18 L mg−1 m−1. This indicated that the manure DOM had medium aromaticity and medium hydrophobicity.24 The SUVA254 value of biochar-DOM was lower than that of manure-DOM, which may be due to the decomposition of the aromatic components in manure-DOM in the pyrolysis process to produce simple, non-aromatic substances. It is worthy of note that the change of the SUVA254 value under pyrolysis condition was not entirely consistent with the change of DOC concentration. The decrease of DOC concentration at 500 °C was clear, but the change of the SUVA254 value at 500 °C was not significant. This suggested that the decrease of DOC concentration at 500 °C was predominantly due to the decomposition of non-aromatic components, while at 600 °C it was predominantly due to the decomposition of aromatic components.
 |
| Fig. 2 The SUVA254 value of DOM released from biochar produced under different pyrolysis conditions. | |
HIX. The effect of pyrolysis temperature and holding time on DOM. HIX was shown in Fig. 3. The ratio of fluorescence intensity in the range of an excitation wavelength of 254 nm, an emission wavelength between 435–480 and an emission wavelength 300–345 nm was used to reflect the humification index (HIX) of the DOM.25 The HIX value of DOM was 7.47–25.87 and the average value was 15.39, which demonstrates that the manure was not fresh, because the HIX value of fresh manure is less than 5.26 The HIX values in soil and sediment are 10–30 and 6–20, respectively.16 This demonstrated that the humification degree of the raw manure used in this study was close to that of soil and sediment. The HIX value of biochar-DOM was lower than that of raw manure, indicating that the humification degree of biochar DOM was lower than the raw manure. The effect of holding time on the HIX value was little when the pyrolysis temperature was in the range of 300 °C to 500 °C. When the pyrolysis temperature reached at 600 °C, the HIX value decreased obviously with the holding time increasing.
 |
| Fig. 3 The HIX value of DOM released from biochar produced under different pyrolysis conditions. | |
According to the changes of DOC, SUVA254 and HIX values, the DOC concentration, aromaticity, and humification of biochar were lower than that of manure. Under a pyrolysis temperature of 300–400 °C, the concentration, aromaticity, and humification of biochar-DOM did not significantly change. Under a pyrolysis temperature of 500 °C, the DOC concentration decreased significantly, the SUVA254 value changed slightly, and the HIX value increased significantly. This indicated that the pyrolysis process at 500 °C involved the decomposition of non-aromatic substances and an increase of humic substances. When the pyrolysis temperature was 600 °C, the decrease of the three parameters suggested the decomposition of all DOM components.
FRI
The whole EEMs spectrum was divided into five regions by the FRI method in accordance with the literature.18 The five regions were assigned as region I-aromatic protein I, region II-aromatic protein II, region III-fulvic acid-like, region IV-microbial by-product–like, and region V-humic acid-like (Fig. 4a). The percentage of fluorescence response in a specific region (Pi) was calculated as the ratio of the EEM volume in the specific region and the EEM volume in the entire EEM(Fig. 4b–f).18,27
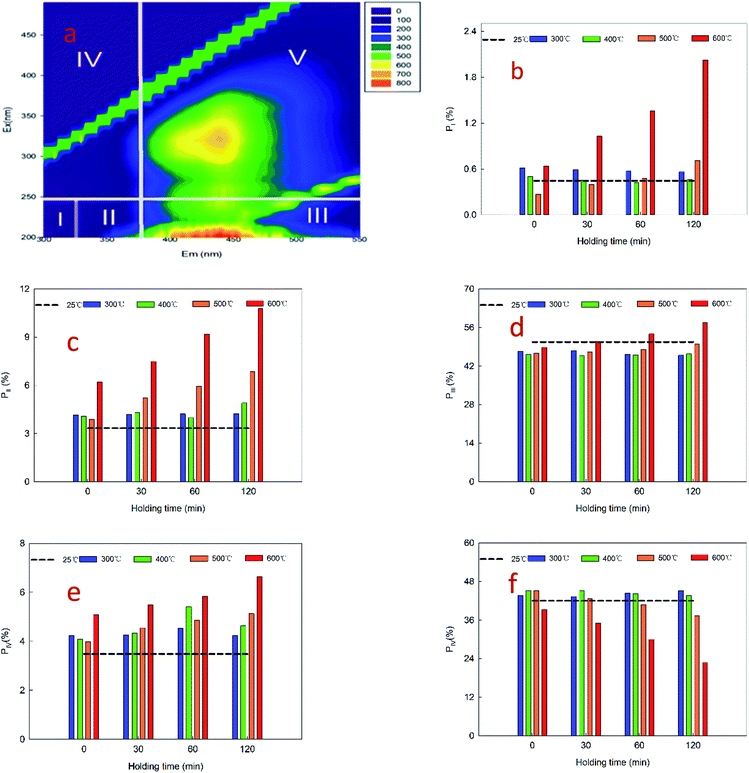 |
| Fig. 4 Percentage distribution of biochar-DOM fractions from FRI analysis: (a) schematic diagram of fluorescence area division; (b) the percentage of fluorescence response of aromatic protein I; (c) the percentage of fluorescence response of aromatic protein II; (d) the percentage of fluorescence response of fulvic acid like substances; (e) the percentage of fluorescence response of microbial by-product–like substances; (f) the percentage of fluorescence response of humic acid-like substances. | |
As shown in Fig. 4b, c, and e, the PI, PII, and PIV of DOM released from any pyrolysis conditions was higher than that of raw manure-DOM, which indicated that aromatic protein substances and microbial by-product substance were generated during the pyrolysis process, and particularly at 600 °C. The PIII of DOM released from biochars produced at 300–500 °C (including 600 °C – 0 min) was lower than that of raw manure DOM. The PIII of DOM released from biochars produced at 600 °C was higher than that of raw manure DOM. This indicated that the fulvic acid-like substance decomposed at lower pyrolysis temperatures and was produced at high pyrolysis temperatures. The PV of biochar-DOM was higher than that of raw manure-DOM when the pyrolysis temperature was between 300–400 and was lower at 600 °C. This indicates that the humic acid-like substance was generated at lower temperatures and decomposed at high pyrolysis temperatures. It has been found that there was a significant relationship between molecular weight and its fluorescence characteristics.28 The molecular weight of protein-like substance was usually less than 0.5 kDa, and the peak molecular weight of fulvic acid and microbial by-product was 0.5–1 kDa, while the molecular weight of humic acid was usually greater than 1 kDa. Therefore, the pyrolysis process at lower pyrolysis temperature was accompanied with the decomposition of medium molecular weight into lower molecular weight and the formation of higher molecular weight.
In summary, at 300–500 °C, the fulvic acid-like substance was the main decomposed component. Aromatic protein-like substance, microbial by-product-like substance, and humic acid-like substance were produced. At 600 °C, humic acid-like substance was the main decomposed component which generated the aromatic protein-like substance, the microbial by-product-like substance, and the fulvic acid-like substance.
PARAFAC analysis
In this study, 7 fluorescence EEM spectra of raw manure-DOM, and 119 fluorescence EEM spectra of manure biochar-DOM were acquired. The excitation ranges were restricted to 250–500 nm to avoid the deteriorating signal/noise ratio in the low excitation wavelength region for PARAFAC analysis.29,30
Four fluorescent components (C1–C4) were identified by PARAFAC modelling, and the EEM contours of each individual component were illustrated in Fig. 5. As reported in the literature, the C1 component (λEx/λEm = 310/404 nm) was categorized as UVA marine humic-like,14,31 and terrestrial humic-like (665–1000 Da),13 microbial humic-like,29 and humic-like highly processed terrigenous.32 The C2 component (λEx/λEm = 350/436 nm) was assigned a microbial reduced quinone-like component,14 UVA humic acid-like,12,33 and humic-like less processed terrigenous.32 The C3 component (λEx/λEm = 340/383 nm) was ascribed microbially-processed substance.32 The C4 component (λEx/λEm = 260, 400/490 nm) was categorized as UVC + UVA humic-like,31 UVA humic-like, terrestrial reduced quinone-like component,14 and humic-like > 1000 Da.24 Therefore, according to the literature the C1–C4 components were assigned as: C1-marine humic-like, C2-UVA humic-like, C3-microbial by-product, C4-UVC + UVA humic-like.
 |
| Fig. 5 EEM contours and the relative distribution of biochar-DOM components from PARAFAC modeling. (component1-marine humic-like, component2-UVA humic-like, component3-microbial by-product, component4-UVC + UVA humic-like). | |
The relative distribution of the four PARAFAC components in each DOM sample was calculated in accordance with the literature(Fig. 5).13,14 The average proportional distribution of component1 (C1%), C2 (C2%), C3 (C3%), and C4 (C4%) in DOM released from raw manure was 38.0%, 34.0%, 5.52%, and 22.4%, respectively. It should be noted that a high proportion of marine humic-like substance was found in manure-DOM. This may be due to the existence of some similar components in the marine DOM and manure-DOM. The composition of marine DOM includes mainly protein and polysaccharide substances, metabolized by phytoplankton,34 which also exist in manure-DOM.35
As shown in Fig. 5, the effect of pyrolysis temperature and holding time on C1% and C2% was similar. When the pyrolysis temperature was 300–400 °C, the C1% and C2% of biochar-DOM were generally higher than that of raw manure-DOM, which indicated that the low-temperature pyrolysis increased the proportion of marine humic-like14 and UVA humic-like components. When the pyrolysis temperature was 500–600 °C, the C1% and C2% of biochar DOM were basically lower than that of raw manure-DOM which indicated that marine humic-like and UVA humic-like components could not resist high-temperature pyrolysis. The C3% of DOM released from biochars produced at any pyrolysis conditions was higher than that of raw manure-DOM. This indicated that the microbial by-product could be generated under different pyrolysis conditions. The optimum condition for C3 production was 600 °C for 60 min. The C4% of DOM released from biochars produced at all pyrolysis conditions was lower than that of raw manure-DOM. The decrease of C4% at 300 °C at 0 min indicated that the UVC + UVA humic-like substance decomposed easily, even at low-temperatures with a short holding time. The longer emission wavelength of the C4 component indicated that it contained higher molecular weight and more hydrophobic components.31 The molecular weight of C4 is usually greater than 1000 Da,26 which is generally defined as reducing quinone like components.36 The decomposition of C4 and the generation of C3 indicated that the high molecular weight DOM has been decomposed into low molecular weight substance.12,14
In summary, the pyrolysis temperature and holding time have a significant effect on the composition of DOM released from manure-derived biochars. The high-weight-molecular humic-like substance (C4) decomposed, and the low-weight-molecular microbial by-products (C3) were generated in the entire pyrolysis temperature range. The medium-weight-molecular humic-like substance (C1 and C2) was generated at low-temperature and decomposed at high temperature.
Relationship between DOM composition indices
Correlation analysis of SUVA254, HIX, Pi% and Ci% was employed to elucidate the interactions between different DOM composition indices (Table 1). As shown in Table 1, SUVA254 had a positive correlation with PV%, C1%, C2% and C4%(p < 0.001). It indicated that humic like substances owned higher aromaticity. HIX had a positive correlation with PV% and C3% (p < 0.001), and had a negative correlation with PI%, PII% and PIII% (p < 0.001). It indicated that the decrease of HIX with pyrolysis temperature increasing was due to the decomposition of humic-like substances and the production of fresh fluorescences including aromatic proteins and microbial by-product substance. PI%, PII%, PIII% and PIV% had negative correlation with C1%, C2% and C3%, which indicated that the generation of aromatic proteins, microbial by-product and fulvic acid are come from the decomposition of humic –like substances including marine humic-like, UVA humic like, UVC + UVA humic-like substances. The Pearson correlation result indicated that the FRI method and PARAFAC modelling both could elucidate the effect of pyrolysis temperature and holding time on biochar-DOM composition.
Table 1 Pearson correlation among SUVA254 and fluorescence related indices of DOM released from the biochar produced under different pyrolysis conditions (n = 112)a,b
Green color: positive correlation is significant at the 0.001 level. Yellow color: negative correlation is significant at the 0.001 level. |
 |
The DOC concentration of biochar-DOM are often detected by TOC analyser. Since the change of DOC concentration under pyrolysis condition are due to the change of DOM components, the DOM composition indices were selected to predict the DOC concentration of biochar-DOM. We predicted the DOC concentration based on the exponential regression analysis between DOC and SUVA254, Pi% and Ci% (Table 2). As shown in Table 2, the DOC concentration could be fitted well with SUVA254, Pi% (except PI%) and Ci% (except C3%). The good agreement between DOC concentration and DOM composition indices demonstrated that UV-vis spectroscopy and fluorescence spectroscopy all could be used to estimate the DOC concentration of biochar-DOM.
Table 2 Exponential regression analysis of DOM composition parameters (i.e., SUVA254) and DOC of different biochar samples (n = 112)a
Parameter |
Fitting equation |
R2 |
**Statistically significant at the probability level of 0.01 (F-test). |
SUVA254 |
Y = 4.4e0.5x |
0.589** |
PI |
Y = 52.1e−72.8x |
0.239** |
PII |
Y = 194.9e−32.7x |
0.614** |
PIII |
Y = 399.3e−52.7x |
0.324** |
PIV |
Y = 220515.7e−18.3x |
0.500** |
PV |
Y = 0.5e10.0x |
0.557** |
C1% |
Y = 1.7e8.4x |
0.267** |
C2% |
Y = 0.41e15.4x |
0.649** |
C3% |
Y = 149.4e−6.0x |
0.540** |
C4% |
Y = 6.7e15.8x |
0.251** |
Conclusions
The EEMs combined with FRI calculation and PARAFAC modelling showed the characterisation of DOM derived from manure biochars depends on the pyrolysis temperature and holding time. At 300 to 500 °C, fulvic-acid-like substance was the main decomposed component, while humic acid-like substance was the main decomposed component at 600 °C. In the pyrolysis temperature of 300–600 °C, the generation of aromatic proteins, microbial by-product and fulvic acid are generated from the decomposition of humic-like substances including marine humic-like, UVA humic like, UVC + UVA humic-like substances in the pyrolysis process of manure biochars. The results in this study suggested that the integration using of FRI and PARAFAC for manure biochars characterisation better understanding of biochar nature and functionality.
Conflicts of interest
There are no conflicts to declare.
Acknowledgements
This research was jointly supported by the Natural Science Foundation of Guangdong Province (No. 2018A030307014), and the Talent Introduction Project of Guangdong University of Petrochemical Technology (2020rc019 and 2020rc012). We would like to thank Editage (www.editage.cn) for English language editing.
Notes and references
- M. P. Bernal, J. A. Alburquerque and R. Moral, Composting of animal manures and chemical criteria for compost maturity assessment. A review, Bioresour. Technol., 2009, 100(22), 5444–5453 CrossRef CAS PubMed.
- C. Mondini, et al., Changes during processing in the organic matter of composted and air-dried poultry manure, Bioresour. Technol., 1996, 55, 243–249 CrossRef CAS.
- H. Dolliver, S. Gupta and S. Nollc, Antibiotic Degradation during Manure Composting, J. Environ. Qual., 2008, 37, 1245–1253 CrossRef CAS PubMed.
- H. J. Ko, et al., Evaluation of maturity parameters and heavy metal contents in composts made from animal manure, J. Waste Manag., 2008, 28(5), 813–820 CrossRef CAS PubMed.
- M. García-Jaramillo, et al., Characterization and selection of biochar for an efficient retention of tricyclazole in a flooded alluvial paddy soil, J. Hazard. Mater., 2015, 286, 581–588 CrossRef PubMed.
- O. Xin, et al., Magnetic biochar combining adsorption and separation recycle for removal of chromium in aqueous solution, Water Sci. Technol., 2017, 75(5), 1177–1184 CrossRef PubMed.
- A. Hass, et al., Chicken manure biochar as liming and nutrient source for acid appalachian soil, J. Environ. Qual., 2012, 41(4), 1096–1196 CrossRef CAS PubMed.
- B. Sulzberger and E. Durisch-Kaiser, Chemical characterization of dissolved organic matter (DOM): a prerequisite for understanding UV-induced changes of DOM absorption properties and bioavailability, Aquat. Sci., 2009, 71(2), 104–126 CrossRef CAS.
- B. A. Biddanda and J. B. Cotner, Enhancement of Dissolved Organic Matter Bioavailability by Sunlight and Its Role in the Carbon Cycle of Lakes Superior and Michigan, J. Great Lakes Res., 2003, 29(2), 228–241 CrossRef CAS.
- M. Haitzer, et al., Effects of dissolved organic matter (DOM) on the bioconcentration of organic chemicals in aquatic organisms – a review, Chemosphere, 1998, 37(7), 1335–1362 CrossRef CAS PubMed.
- T. Li, et al., Complexation with dissolved organic matter and mobility control of heavy metals in the rhizosphere of hyperaccumulator Sedum alfredii, Environ. Pollut., 2013, 182, 248–255 CrossRef CAS PubMed.
- A. U. Rajapaksha, et al., Dissolved organic matter characterization of biochars produced from different feedstock materials, J. Environ. Manage., 2019, 233, 393–399 CrossRef CAS PubMed.
- H. Wu, et al., Revealing the impact of pyrolysis temperature on dissolved organic matter released from the biochar prepared from Typha orientalis, Chemosphere, 2019, 228, 264–270 CrossRef CAS PubMed.
- C. Yang, et al., Characterization of fluorescent dissolved organic matter from green macroalgae (Ulva prolifera)-derived biochar by excitation-emission matrix combined with parallel factor and self-organizing maps analyses, Bioresour. Technol., 2019, 287, 121471 CrossRef CAS PubMed.
- T. Ohno, et al., Molecular weight and humification index as predictors of adsorption for plant- and manure-derived dissolved organic matter to goethite, J. Great Lakes Res., 2007, 58, 125–132 Search PubMed.
- J. E. Birdwell and A. S. Engel, Characterization of dissolved organic matter in cave and spring waters using UV-vis absorbance and fluorescence spectroscopy, Org. Geochem., 2010, 41(3), 270–280 CrossRef CAS.
- R. K. Henderson, et al., Fluorescence as a potential monitoring tool for recycled water systems: a review, Water Res., 2009, 43(4), 863–881 CrossRef CAS PubMed.
- W. Chen, et al., Fluorescence Excitation-Emission Matrix Regional Integration to Quantify Spectra for Dissolved Organic Matter, Environ. Sci. Technol., 2003, 37, 5701–5710 CrossRef CAS PubMed.
- C. A. Stedmon and R. Bro, Characterizing dissolved organic matter fluorescence with parallel factor analysis: a tutorial, Limnol. Oceanogr.: Methods, 2008, 6, 572–579 CrossRef CAS.
- C.-H. Liu, et al., Quantification and characterization of dissolved organic carbon from biochars, Geoderma, 2019, 335, 161–169 CrossRef CAS.
- J. Wei, et al., Pyrolysis temperature-dependent changes in the characteristics of biochar-borne dissolved organic matter and its copper binding properties, Bull. Environ. Contam. Toxicol., 2019, 103, 169–174 CrossRef CAS PubMed.
- J. L. Weishaar, et al., Evaluation of specific ultraviolet absorbance as an indicator of the chemical composition and reactivity of dissolved organic carbon, Environ. Sci. Technol., 2003, 37, 4702–4708 CrossRef CAS PubMed.
- P. Yu, et al., Molecular Weight, Polydispersity, and Spectroscopic Properties of Aquatic Humic Substance, Environ. Sci. Technol., 1994, 28(11), 1853–1858 CrossRef PubMed.
- M. Huang, et al., Application potential of biochar in environment: insight from degradation of biochar-derived DOM and complexation of DOM with heavy metals, Sci. Total
Environ., 2019, 646, 220–228 CrossRef CAS PubMed.
- T. Ohno, Fluorescence inner-filtering correction for determining the humification index of dissolved organic matter, Environ. Sci. Technol., 2002, 36, 742–746 CrossRef CAS PubMed.
- J. F. Hunt and T. Ohno, Characterization of fresh and decomposed dissolved organic matter using excitation-emission matrix fluorescence spectroscopy and multiway analysis, J. Agric. Food Chem., 2007, 55, 2121–2128 CrossRef CAS PubMed.
- N. Peng, et al., Quantifying interactions between propranolol and dissolved organic matter (DOM) from different sources using fluorescence spectroscopy, Environ. Sci. Pollut. Res., 2014, 21(7), 5217–5226 CrossRef CAS PubMed.
- C. W. Cuss and C. Guéguen, Relationships between molecular weight and fluorescence properties for size-fractionated dissolved organic matter from fresh and aged sources, Water Res., 2015, 68, 487–497 CrossRef CAS PubMed.
- K. P. Mangalgiri, et al., PARAFAC Modeling of Irradiation- and Oxidation-Induced Changes in Fluorescent Dissolved Organic Matter Extracted from Poultry Litter, Environ. Sci. Technol., 2017, 51(14), 8036–8047 CrossRef CAS PubMed.
- L. Yang, J. Hur and W. Zhuang, Occurrence and behaviors of fluorescence EEM-PARAFAC components in drinking water and wastewater treatment systems and their applications: a review, Environ. Sci. Pollut. Res., 2015, 22(9), 6500–6510 CrossRef CAS PubMed.
- S. K. L. Ishii and T. H. Boyer, Behavior of reoccurring PARAFAC components in fluorescent dissolved organic matter in natural and engineered systems: a critical review, Environ. Sci. Technol., 2012, 46(4), 2006–2017 CrossRef CAS PubMed.
- A. Stubbins, J.-F. Lapierre and M. Berggren, What's in an EEM? Molecular signatures associated with dissolved organic fluorescence in Boreal Canada, Environ. Sci. Technol., 2014, 48(18), 10598–10606 CrossRef CAS PubMed.
- P. Li and J. Hur, Utilization of UV-vis spectroscopy and related data analyses for dissolved organic matter (DOM) studies: a review, Crit. Rev. Environ. Sci. Technol., 2017, 47(3), 131–154 CrossRef CAS.
- A. Nebbioso and A. Piccolo, Molecular characterization of dissolved organic matter (DOM)_ a critical review, Anal. Bioanal. Chem., 2013, 405, 109–124 CrossRef CAS PubMed.
- O. G. Clark, et al., Manipulation of Dietary Protein and Nonstarch Polysaccharide to Control Swine Manure Emissions, J. Environ. Qual., 2005, 34(5), 1461–1466 CrossRef CAS PubMed.
- R. M. Cory and D. M. McKnight, Fluorescence spectroscopy reveals ubiquitous presence of oxidized and reduced quinones in dissolved organic matter, Environ. Sci. Technol., 2005, 39, 8142–8149 CrossRef CAS PubMed.
|
This journal is © The Royal Society of Chemistry 2020 |
Click here to see how this site uses Cookies. View our privacy policy here.