DOI:
10.1039/D0RA02644A
(Paper)
RSC Adv., 2020,
10, 19844-19851
Isolation of antioxidant peptides from yak casein hydrolysate
Received
22nd March 2020
, Accepted 14th May 2020
First published on 27th May 2020
Abstract
Enzymatic hydrolysis of protein is a principal method to obtain antioxidant peptides. A yak casein hydrolysate (YCH) was prepared by alcalase and trypsin digestion. An ultrafiltration membrane system was used to divide the hydrolysate into four molecular weight fractions; YCH-4 (<3 kDa) had the highest antioxidant activity. Fraction YCH-4 was separated into six subfractions by gel filtration chromatography; reverse-phase high performance liquid chromatography (RP-HPLC) was then used to partition sixteen antioxidant peptide subfractions. Liquid chromatography/electrospray tandem mass spectrometry (LC-ESI-MS/MS) was used to determine the amino acid sequence of a purified antioxidant peptide to be Arg-Glu-Leu-Glu-Glu-Leu (787.41 Da). Finally, a synthetic Arg-Glu-Leu-Glu-Glu-Leu peptide was evaluated for its superoxide anion and hydroxyl radical scavenging activity (IC50 = 0.52 and 0.69 mg mL−1), which confirmed the activity of the native purified peptide. Our results suggested that isolation and purification of antioxidant peptides from yak casein could be an important means to obtain natural antioxidant peptides.
1. Introduction
Antioxidants from natural sources have been used as the drugs of choice for the prevention and treatment of various human diseases related to reactive oxygen species.1 Natural antioxidant peptides remove free radicals in vivo, and they are effective in preventing damage caused by cellular reactive oxygen species.2 In addition, some synthetic antioxidants, such as butylated hydroxytoluene (BHT), butylated hydroxyanisole (BHA), propyl gallate (PG), and t-butylhydroquinone (TBHQ), have higher antioxidant capacities and lower costs, which make them attractive to the food industry.3 However, synthetic antioxidants can damage proteins and DNA, thus, they pose threats to human health and require strict regulation.4 Therefore, there is a critical need to identify effective, natural antioxidant that do not pose health risks.
Bioactive peptides have many functions, such as improving immunity, inhibiting thrombosis, and dispelling free radicals.5 Bioactive peptides are produced in two principal methods: enzymatic hydrolysis of proteins and fermentation of dairy products.6 Proteolytic enzymes with different cleavage specificities produce many different bioactive peptides. Compared with crude protein, the function and nutritional properties of protein-containing foods have been enhanced by using different proteases to hydrolyze proteins.7 The diversity of protein sources with different amino acid compositions provides a precursor pool of peptides with different biological activities.8 Bioactive peptides usually have fewer than 20 amino acid residues and molecular weights less than 10 kDa. Their biological activities depend mainly on molecular weight, composition, and amino acid sequence.9 Compared with synthetic biologically active peptides, investigators have become increasingly interested in antioxidant peptides from natural sources. By methods such as gastrointestinal digestion, enzymatic hydrolysis, and fermentation, bioactive peptides can be released from natural protein molecules.2,10
Many investigators have assessed the antioxidant properties of peptides from natural sources. Antioxidant peptides with different activities have been purified from sources of milk protein hydrolysates, such as chickpea,9 cow milk,11 buffalo milk,12 camel milk,13 goat milk,14 water hyacinth leaf protein,15 loach protein,16 soybean.17 O'Keeffe and FitzGerald (2015) and Sadat et al. (2011) reported that identified peptide sequences Phe-Pro, His-Gln-Pro, Asn-Ser-Leu-Pro and Ile-Asn-Tyr-Trp and Leu-Asp-Gln-Trp from bovine β-casein and α-lactalbumin, respectively.18,19 Tonolo et al. (2019) subjected milk casein to hydrolysis and obtained two peptides with in vitro antioxidant activities Ala-Val-Pro-Tyr-Pro-Gln-Arg and Lys-Val-Leu-Pro-Val-Pro-Glu-Lys.21 Meanwhile, antioxidant active peptides such as Glu-Ala-Leu-Glu-Lys-Phe-Asp-Lys and Glu-Ala-Leu-Glu-Lys-Phe-Asp-Lys can also be obtained through fermentation and digestion of goat milk.14,15
Yak (Bos grunniens) milk is one of the main ingredients and food source for traditional Tibetan herdsman's in the Tibetan plateau. Special geographical conditions limit the collection and commercialization of yak milk,15 and yak milk is generally used to produce butter. The rest of the yak milk is acidified naturally (microbial fermentation), coagulated, separated, and air-dried to produce crude yak casein.19 Compared with bovine casein, yak casein has higher amounts of nutrients, 4.9–5.3% protein, 5.5–7.2% fat, 16.9–17.7% dry matter, and 7–12% water.19,22 The amino acid composition, lactose, and mineral content also differ from bovine milk.23,24 Therefore, yak casein is considered as a special type of casein. Cow and buffalo milk are generally considered good sources of bioactive compounds with anti-inflammatory, antioxidant, and antibacterial activities.5,22,25 However, there are only a few reports on the antioxidant activities of yak casein hydrolysates, and few reports of the amino acid sequence and structure of yak casein-derived antioxidant peptides after enzymatic hydrolysis.
Hence, the purpose of our study was to identify antioxidant peptides from yak casein. We measured superoxide anion, hydroxyl, 2,2-diphenyl-1-picrylhydrazyl (DPPH) radical scavenging activity, reducing power, and ferrous ion chelating ability of the casein and its hydrolysates. In addition, we purified antioxidant peptides by ultrafiltration, gel filtration chromatography, and RP-HPLC. We determined by LC-ESI-MS/MS the amino acid sequence of one highly active peptide. A chemically synthesized version of this peptide recapitulated the antioxidant activity of the peptide isolated and purified from yak casein.
2. Experimental
2.1. Materials
DPPH, glutathione (GSH), Sephadex G-25, Ferrozine, Alcalase (EC 3.4.21.26, activity 200 U mg−1), and trypsin (EC 3.4.4.4, activity 250 U mg−1) were purchased through Beijing Science & Technology Co., Ltd. (Beijing, China). Other analytical grade reagents were purchased from Shanghai Chemical Reagent Co., Ltd. (Shanghai, China).
2.2. Preparation of yak casein
Yak milk was purchased from Linxia Huaan Biological Products Co. Ltd. (Linxia County, Gansu Province, China). Casein was prepared as follows: first, the pH of the skimmed yak milk was adjusted to pH 4.7 using 1 M HCl (PHS-3C, INESA Scientific Instrument Co., Ltd., China). The precipitated yak casein was separated from whey by high-speed centrifugation (5000g, 30 min, 4 °C) (TGL-20 M, Xiangyi Centrifuge Instrument Co., Ltd., China), then washed with distilled water and centrifuged again to remove residual whey. The recovered casein (95.36% w/w) was lyophilized and stored at −20 °C.
2.3. Preparation of yak casein hydrolysate (YCH)
The enzymatically lyophilized yak casein (6%, w/v) was dissolved in distilled water at pH 8.5 and preheated at 50 °C. Adjusted an alcalase-to-yak casein ratio to 1
:
500 (w/w), and hydrolysis was continued for 3.5 h. The reaction temperature was adjusted to 45 °C, the pH was adjusted to pH 7.5, and trypsin was added to (trypsin-to-substrate was 3
:
1000, w/w) and this reaction continued for another 2.5 h. The reaction was conducted in a water bath with a magnetic stirrer (New Brunswick Scientifics C24, Jintan, China). Throughout the hydrolysis process, the pH was maintained at the desired value by addition of 1 M NaOH every 0.5 h. The reaction was terminated (boiling water bath, 20 min), and a supernatant was then obtained by centrifugation (4000g, 20 min, 4 °C) and lyophilized for further experiments (Zhang et al., 2011).7 The supernatants were lyophilized and stored for further use.
2.4. Purification of antioxidant peptides
2.4.1. Ultrafiltration. The lyophilized yak casein hydrolysate powder was dissolved in distilled water and separated by ultrafiltration with regenerated cellulose membranes (HFM0250, Xiamen Fumei Technology Co., Ltd., China) with a molecular weight cutoffs of 12, 6, and 3 kDa. YCH-1, YCH-2, YCH-3, and YCH-4 represented the fractions with molecular weight (MW) distribution of >12 kDa, 6–12 kDa, 3–6 kDa and <3 kDa, respectively. All permeates and retentates were lyophilized and their antioxidant activities were determined.
2.4.2. Sephadex G-25 gel filtration. Freeze-dried ultrafiltration fractions with desired antioxidant activities were further fractionated through a Sephadex G-25 gel filtration column (1.6 × 90 cm, Solarbio Life Science, Beijing, China). The column was pre-equilibrated and eluted with phosphate buffered saline (PBS) (20 mM, with 0.1% NaCl, pH 7.4). Fractions (2 mL) were collected at a flow rate of 0.3 mL min−1, and assayed at 215 nm (UV759 (PC), Shanghai Yuefeng Instrument Co., Ltd., China). Draw the elution curve with the absorbance value as the abscissa and the elution volume as the ordinate. Fractions with peak absorbances were pooled, lyophilized and assayed for antioxidant activity before further separation and purification.
2.4.3. Reverse-phase high-performance liquid chromatography analysis. The fraction with the greatest antioxidant activity eluted by gel chromatography was further purified. The sample was dissolved in Milli Q water (filtered through 0.22 μm filters), loaded onto a C18 column (Dionex C18, Φ4.6 mm × 200 mm, 5 μm), and purified by RP-HPLC (Dionex UltiMate 3000, Thermo Fisher Scientific, USA). Peptides were eluted with a mobile phase consisting of eluent A (0.1% (v/v) tri-fluoroacetic acid (TFA) in acetonitrile) and eluent B (0.1% (v/v) TFA in Milli Q water) with a flow rate of 0.6 mL min−1, and the elution peak was measured at 215 nm. A linear gradient of solvent A was applied with the following time schedule: 0–6 min, 5–15%; 6–10 min, 15–25%; 13–16 min, 30–35%; 16–20 min, 35–45%; 20–25 min, 45–75%; 25–30 min, 75–80%; 30–35 min, 80–85%; 35–37 min, 85–95%; 37–40 min, 95–5%. The purification step was repeated to obtain enough material for activity determination and sequence analysis. Chromatographic analyses were performed with a Waters 600E automated gradient controller pump, a Waters Wisp 717 automatic sampling device, and a Waters 996 photodiode array detector. Millennium software was used to acquire, plot, and analyze data. The antioxidant activities of the eluted peaks were determined. Spectral and chromatographic data were stored on a NEC Image 466 computer.
2.4.4. Identification of antioxidant peptides. Molecular mass determination and peptide sequencing were conducted in a positive ion mode using electrospray ionization-mass spectrometry (ESI-MS) and tandem mass spectrometry (MS/MS), respectively. ESI-MS was performed using the triple quadrupole instrument Applied Biosystems API 3000 (PE Sciex, Toronto, ON, Canada), equipped with an electrospray ion source. The freeze-dried samples from RP-HPLC were dissolved in acetonitrile/water (80%; v/v) containing 0.1% forming acid. The solution (5.0 μL) was injected (nebulized) uninterrupted by a pump (an Ultimate 3000 system coupled with an Orbitrap Elite™ Hybrid Ion Trap-Orbitrap Mass spectrometer, Thermo Fisher Scientific, USA) with a flow rate of 300 nL min−1, with the following LC linear gradient: 6–9%, 8 min; 9–14%, 16 min; 14–30%, 36 min; 30–40%, 15 min; 40–95%, 3 min; 95%, 7 min (A: acetonitrile with 0.1% (v/v) formic acid) (ReproSil-Pur C18-AQ (100 μm × 10 cm, 3 μm, 120 Å, water)). The peptide sequence was determined from the CID spectrum of the protonated analysis [M + H]+ by MS/MS experiments. The mass spectral data were analyzed and the amino acid sequence of the antioxidant peptide was determined based on with the bioanalysts software (Applied Biosystems, South San Francisco, CA, USA).Fraction P-4-8 was a mixture of multiple peptides, for peptide identification we used synchronous ionization mass spectrometry. The major precursor ions of the polypeptide were analyzed by the MS/MS detection method to determine the amino acid sequence of the active polypeptide. We further analyzed and queried the amino acid sequences, molecular weight, and isoelectric point of the identified antioxidant peptides with the NCBI database, BioPepDB database (http://bis.zju.edu.cn/biopepdbr/index.php), and ExPASy (https://web.expasy.org/protparam/).
2.5. Peptide synthesis
According to the results of LC-ESI-MS/MS, the solid-phase chemical synthesis method was used to prepare the identified active peptide (Shanghai Gil Biochemical Co., Ltd. Shanghai, China). The antioxidant properties of the synthetic polypeptide was determined according to the follows methods.
2.6. Antioxidant activity
2.6.1. Measurement of superoxide anion radical scavenging activity. Superoxide anion radical scavenging activity of yak casein and its hydrolysates was measured followed the method of Li et al. (2012) with slight adjustments.26 Samples of 2.0 mL were mixed with 2.95 mL Tris–HCl buffer (pH 7.4) and 50 μL 5.0 mmol L−1 pyrogallol solution (prepared in 10.0 mmol L−1 HCl). The absorbances of the mixtures at 325 nm were immediately measured every 30 s for 5 min. The YCH was replaced by distilled water as a blank, and the casein and its hydrolysates were replaced by GSH as a positive control. The superoxide anion free radical scavenging activity was calculated using the following formula (1): |
 | (1) |
where AS is the absorbance of sample and AB is the absorbance of blank.
2.6.2. Determination of hydroxyl radical scavenging activity. Hydroxyl radical scavenging activity was measured by the method of Zhang, Duan and Zhuang (2012) with slight modification.27 One milliliter aliquots of different concentrations of sample were mixed with 1.0 mL PBS (pH 6.6, 1.865 mmol L−1) and 1.0 mL FeSO4·7H2O (1%, w/v). Then 1.0 mL H2O2 (0.03%, v/v) was added. The mixtures were incubated for 1 h at 37 °C, and then centrifuged at 3000g for 10 min. The absorbances of the supernatants were measured at 532 nm. The samples were replaced by distilled water as a blank, and distilled water was added instead of H2O2 for a negative control, which was measured with same method. The hydroxyl radical scavenging activity was calculated by formula (2): |
 | (2) |
where AS is the absorbance of sample, AB is the absorbance of the blank, and AC is the absorbance of control.
2.6.3. Measurement of DPPH radical scavenging activity. The DPPH radical scavenging activity of YCH was measured by the method of Tai, Iomori and Ito (2017) with slight modification.28 Briefly, 2.0 mL aliquots of YCH (2.0 and 5.0 mg mL−1) were added to 2.0 mL 0.1 mmol L−1 DPPH radical solution freshly prepared in ethanol. Absorbance of the supernatant at 517 nm was determined after the solution was kept at room temperature for 30 min in the dark and centrifuged (3000g, 15 min, 4 °C). For a blank, distilled water was used instead of YCH samples, and ethanol was added instead of DPPH as a control. The DPPH radical scavenging activity calculation equation is shown as formula (3): |
 | (3) |
where AS is the absorbance of sample, AB is the absorbance of the blank and AC is the absorbance of control.
2.6.4. Determination of reducing power. The reducing power of YCH was determined by the method of Gu, Abbas and Zhang (2009) with small modifications.29 One milliliter aliquots of hydrolysate at 2.0 and 5.0 mg mL−1 were mixed with 2.5 mL PBS (pH 6.6, 0.2 mol L−1) and 2.5 mL potassium ferricyanide (K3Fe(CN)6) (1%, w/v). The mixtures were keep in a water bath (50 °C, 20 min). After cooling to room temperature, 2.5 mL trichloroacetic acid (10%, v/v) was added, and supernatants were obtained by centrifugation (3000g, 10 min, 4 °C). Supernatants (2.5 mL) were mixed with 2.5 mL of double distilled water and 0.5 mL ferric chloride (FeCl3) (0.1%, w/v). Absorbance of the mixture at 700 nm was measured after the mixture remained at room temperature for 10 min.
2.6.5. Determination of Fe2+chelating activity. The ferrous ion (Fe2+) chelating activities of the samples were determined by the method of Carrasco-Castilla et al. (2012) with some modifications.30 One milliliter YCH solution at 2.0 mg mL−1 and 5.0 mg mL−1 was mixed with 0.1 mL 2.0 mmol L−1 FeCl2 and 3.7 mL double distilled water. Then 0.2 mL 5.0 mmol L−1 ferrozine solution was added. Absorbance of the mixture at 562 nm was determined after the mixture remained at room temperature for 20 min and centrifuged 5 min at 3000g. The blank was used in the same manner except that double distilled water was added instead of YCH solution. The Fe2+ chelating activity was calculated with the following formula (4): |
 | (4) |
where AB is the absorbance of the blank and AS is the absorbance of sample.
2.7. Statistical analysis
All results are presented are means ± standard (SD) of triplicate determinations. An analysis of variance (ANOVA) was performed, and the mean comparisons were analyzed by Duncan's multiple range test, P < 0.05 was considered statistically significant. The statistical analyses were accomplished using an SPSS package (SPSS 24.0 for Windows, SPSS Inc.).
3. Results and discussion
We hydrolyzed yak casein with alcalase and trypsin, separated the peptides by several techniques, and evaluated their antioxidant activities.
3.1. Ultrafiltration grading
As shown in Table 1, compared with other separated components, YCH fraction #4 (MW < 3 kDa) had the highest free radical scavenging activity and metal chelating activity at 5.0 mg mL−1. There was a statistically significant difference between the free radical scavenging ability of YCH-4 and the other fractions. The superoxide anion radical scavenging at 5.0 mg mL−1 of the GSH was 0.05 mg mL−1. Ultrafiltration fractions of different molecular weights all had higher free radical scavenging capacity compared with the crude casein hydrolysate. Smaller molecular weight peptides more easily form reaction precursors,31 probably because smaller peptides expose more electron-donating side chains, and smaller peptides can be reduced more easily by contact with Fe3+/ferricyanide complexes.30 Generally, low molecular weight peptides were considered to be powerful antioxidants Lou et al. (2010) who evaluated a loach protein hydrolysate with MW of 0–3 kDa.16 Similarly, O'Keeffe and FitzGerald (2015) also found that, compared with higher MW fractions, peptide fractions <1 kDa possessed the highest antioxidant ability.18 Meanwhile, Panchal, Hati and Sakure (2019) reported that the 3 kDa retentate of ultrafiltered fermented goat milk had higher antioxidant activity compared with a 10 kDa molecular weight cutoff fraction.20 In summary, antioxidant activity of components is dependent on molecular weight, and ultrafiltration can increase the free radical scavenging and metal ion chelation specific activities of an unfractionated enzymatic hydrolysate.32 Therefore, we used the following process to isolate the peptides in YCH-4.
Table 1 Antioxidant activities of yak casein hydrolysate ultrafiltration fractionsa
|
Control |
YCH-1 (>12 kDa) |
YCH-2 (6–12 kDa) |
YCH-3 (3–6 kDa) |
YCH-4 (<3 kDa) |
The antioxidant activities of the ultrafiltration fractions were measured at a concentration of 5.0 mg mL−1. Yak casein hydrolysate (YCH) without ultrafiltration served as the control. Values followed by the same letter in the same row are not significantly different (P > 0.05). |
Superoxide anion radical scavenging (%) |
76.23 ± 0.75e |
81.69 ± 1.00d |
83.94 ± 0.65c |
88.01 ± 0.72b |
91.18 ± 0.57a |
Hydroxyl radical scavenging (%) |
72.12 ± 1.72e |
76.24 ± 0.18d |
79.54 ± 1.81c |
83.66 ± 1.68b |
85.74 ± 0.70a |
DPPH radical scavenging (%) |
49.86 ± 1.01d |
50.92 ± 0.72d |
59.14 ± 0.97c |
67.16 ± 0.82b |
72.02 ± 0.86a |
Reducing power A (700) |
0.488 ± 0.016c |
0.530 ± 0.017bc |
0.569 ± 0.023b |
0.654 ± 0.023a |
0.533 ± 0.017bc |
Fe2+ chelating activity (%) |
52.97 ± 2.66e |
56.51 ± 2.28d |
63.52 ± 2.46c |
69.56 ± 0.84b |
76.81 ± 0.27a |
3.2. Fractionation by Sephadex G-25 gel filtration
Molecular weight, concentration, and amino acid composition and sequence are contributing factors for the function of antioxidant peptides. The YCH-4 sample (MW < 3 kDa) was dissolved in PBS (20 mmol L−1, with 0.1% NaCl, pH 7.4) and loaded onto an equilibrated Sephadex G-25 gel filtration column. YCH-4 eluted in six peak fractions (Fig. 1). The fractions were lyophilized and assayed for antioxidant capacity. Table 2 shows that fraction P-4 exhibited the highest superoxide anion and hydroxyl radical scavenging ability, and the scavenging rates were, respectively, 50.29% and 59.79% at 2.0 mg mL−1. There was a statistically significant difference between the free radical scavenging activity of P-4 and other the fractions (P < 0.05). Compared with the component YCH-4, further purification of P-4 increased its antioxidant activity, a result that was similar to findings by Ren et al. (2008).33 With increasing retention time, the antioxidant activity of the eluted fraction became weaker. The fraction P-4 obtained by further separation and purification by Sephadex G-25 gel filtration contains a certain range of molecular mass expressing the highest antioxidant activity. Therefore, we selected fraction P-4 for further separation and purification.
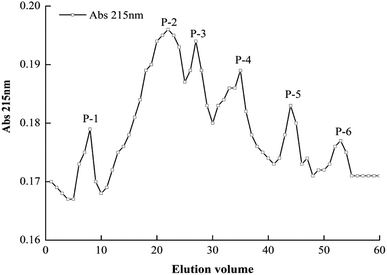 |
| Fig. 1 Sephadex G-25 separation of antioxidant peptides from yak casein hydrolysate. The column (1.6 × 90 cm) was equilibrated and eluted with PBS (20 mmol L−1, with 0.1% NaCl, pH 7.4) at a flow rate of 0.3 mL min−1. Elution was monitored by absorbance at 215 nm. Six peptide fractions were obtained according to molecular size. | |
Table 2 Free radical scavenging activity, metal chelating activity, and reducing power of YCH-4 (<3 kDa) fractionated by Sephadex G-25 gel filtrationa
|
P-1 |
P-2 |
P-3 |
P-4 |
P-5 |
P-6 |
P-1, P-2, etc. refer to the fractions in Fig. 1. Their antioxidant activities were measured at a concentration of 2.0 mg mL−1. Values followed by the same letter in the same row are not significantly different (P > 0.05). |
Superoxide anion radical scavenging (%) |
20.21 ± 0.48e |
26.40 ± 1.50d |
33.56 ± 0.97b |
50.29 ± 0.09a |
29.21 ± 0.02c |
6.85 ± 0.97f |
Hydroxyl radical scavenging (%) |
17.96 ± 1.28f |
22.20 ± 1.97e |
25.96 ± 0.25d |
59.79 ± 1.18a |
42.71 ± 0.85b |
29.69 ± 0.45c |
DPPH radical scavenging (%) |
17.54 ± 1.91e |
24.77 ± 1.44d |
35.24 ± 1.31c |
67.61 ± 1.78a |
38.29 ± 0.90b |
6.97 ± 1.97f |
Reducing power A (700) |
0.106 ± 0.003c |
0.156 ± 0.007b |
0.154 ± 0.029bc |
0.348 ± 0.043a |
0.145 ± 0.001bc |
0.172 ± 0.004b |
Fe2+ chelating activity (%) |
14.60 ± 1.12e |
18.65 ± 1.84d |
31.32 ± 0.53b |
56.16 ± 1.61a |
24.95 ± 0.93c |
13.42 ± 0.71e |
3.3. Fractionation of P-4 by semi-preparative, reverse-phase high performance liquid chromatography (RP-HPLC)
To identify the antioxidant peptides, RP-HPLC has become an important method for separating and purifying peptides with relative molecular weights <5 kDa. Based on the difference in polarity between the molecules, and a C18 column was used to separate bioactive peptides. We subjected fraction P-4 to semi-preparative RP-HPLC using a C18 column (4.6 mm × 200 mm, 5 μm). The elution was separation into sixteen fractions, P-4-1 to P-4-16 (Fig. 2). Fig. 3 shows that, when the concentration of the sample was 1.0 mg mL−1, the fraction P-4-8 exhibited the highest antioxidant capacity, its superoxide anion and hydroxyl radical removal rates were 66.78% and 56.10% (P < 0.05). The superoxide anion radical scavenging at 1.0 mg mL−1 of the GSH was 0.05 mg mL−1. Generally, the components are collected according to the retention time. The retention time is long, it indicates that the target component contains hydrophobic amino acids. Previous studies have shown that antioxidant activity is associated with hydrophobic amino acids.15 Therefore, the structural characteristics of fraction P-4-8 were further identified.
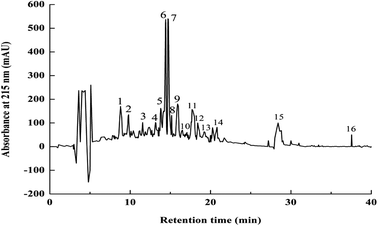 |
| Fig. 2 RP-HPLC elution profile of P-4 fraction from Dionex C18 column. Flow rate, 0.6 mL min−1; monitoring absorbance, 215 nm. Numbers 1–16 represent elution peaks (P-4-1 to P-4-16). | |
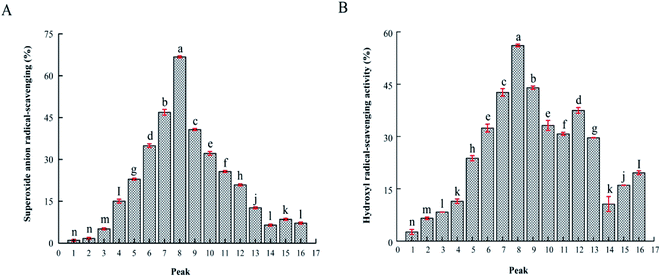 |
| Fig. 3 Antioxidant activities of semi-preparative RP-HPLC fractions at 1.0 mg mL−1. Antioxidant activity was measured by superoxide anion radical scavenging (A) and hydroxyl radical scavenging (B). Columns that do not share the same letter are significantly different from each other at P < 0.05. | |
3.4. LC-ESI-MS/MS identification of antioxidant peptides of P-4-8
Peptide antioxidant properties are functions of their composition, structure, hydrophobicity, and amino acid sequences. We identified six purified peptides in fraction P-4-8 (Table 3 and Fig. 4). The peptides contained 2–10 amino acids, and all peptides displayed higher free radical scavenging ability compared with the crude yak casein. Previous research has indicated that the most effective antioxidant peptides were relatively low in molecular weight and contained less than 20 amino acids.34 According to previous researched by Singh, Vij and Hati (2014) reported that peptides with molecular weight between 200–3000 Da had higher antioxidant activity than macromolecular peptides, and most antioxidant peptides derived from food sources had sizes of 500–1500 Da.9
Table 3 Amino acid sequences and antioxidant activities of P-4-8 peptidesa
Amino acid sequence |
Calculated mass (Da) |
Superoxide anion radical scavenging (IC50, mg mL−1) |
Hydroxyl radical scavenging (IC50, mg mL−1) |
The peptide antioxidant activities. Each line that does not share the same letter was significantly different from each other at P < 0.05. |
Arg-Glu-Leu-Glu-Glu-Leu (RELEEL) |
787.41 |
0.55 ± 0.08a |
0.78 ± 0.11a |
Gly-Lys-Glu-Lys-Val-Asn-Glu-Leu (GKEKVNEL) |
915.50 |
0.70 ± 0.17b |
0.88 ± 0.09b |
Leu-Pro-Val-Pro-Gln (LPVPQ) |
552.33 |
2.39 ± 0.10c |
2.20 ± 0.04c |
His-Pro-His-Pro-His-Leu (HPHPHL) |
736.38 |
2.05 ± 0.03c |
1.71 ± 0.13c |
Val-Leu-Pro-Val-Pro (VLPVP) |
523.34 |
2.44 ± 0.13c |
2.74 ± 0.07c |
Val-Pro-Tyr-Pro-Gln (VPYPQ) |
602.31 |
2.69 ± 0.07c |
3.18 ± 0.05c |
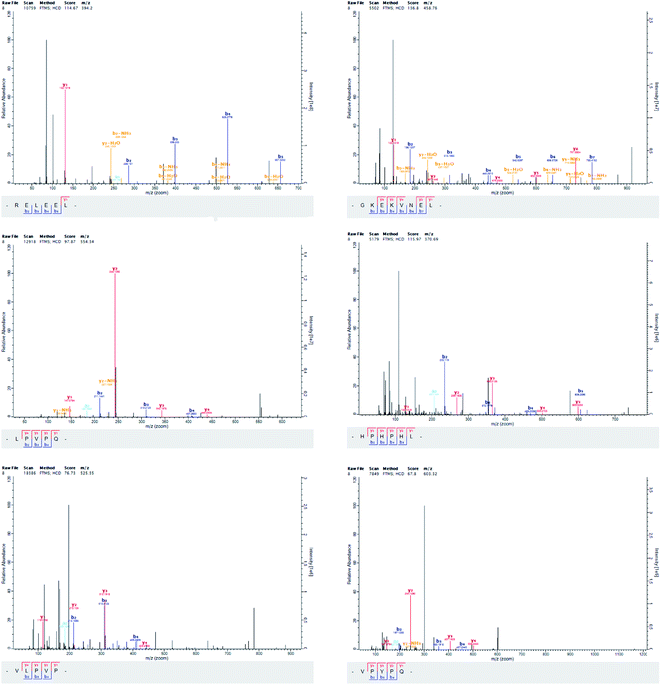 |
| Fig. 4 MS/MS spectrum of P-4-8 peptides. | |
As shown in Table 3, Arg-Glu-Leu-Glu-Glu-Leu had the highest superoxide anion and hydroxyl radical scavenging activities (45.54% and 32.19% at 0.5 mg mL−1, respectively). The superoxide anion radical scavenging at 0.5 mg mL−1 of the GSH was 0.05 mg mL−1. Glutamic acid, leucine, proline, and histidine were the major amino acids of all the peptides. Furthermore, fraction P-4-8 contained the hydrophobic amino acids leucine and valine at the N-termini of several peptides, and glutamine, leucine, and proline were present at the C-termini. According to the Power, Jakeman and FitzGerald (2012) reported that N- and C-terminal hydrophobic amino acids may engender higher antioxidant activity compared with peptides lacking those residues.35
P-4-8 contained glutamic acid and leucine within the sequence Arg-Glu-Leu-Glu-Glu-Leu and Gly-Lys-Glu-Lys-Val-Asn-Glu-Leu. Lee et al. (2013) reported that hydrophobic amino acid such as leucine could increase the scavenging of free radicals generated in the lipid phase.34 Sila and Bougatef (2016) manifested that glutamic acid was important in high antioxidant activity.36 Suetsuna (2000) reported that the preferred sequences for antioxidant peptides were Glu-Leu > Tyr-Phe-Tyr-Pro-Glu-Leu > Phe-Tyr-Pro-Glu-Leu > Tyr-Pro-Glu-Leu > Pro-Glu-Leu.37 These findings indicated that the Glu-Leu sequence enhanced the antioxidant activity of the casein-derived polypeptide. Consistent with our findings, Zhang et al. (2012) similarly reported that Glu-Leu was important in radical-scavenging potency.27
The peptide His-Pro-His-Pro-His-Leu contained histidine and proline. Histidine was linked to antioxidant ability by donating an electron through a resonant structure that converts radicals to stable molecules.17 Ahmed, Tawfik, Laila and Hisham (2015) reported that the proline residues in Ala-Arg-His-Pro-His-Pro-Leu-Ser-Phe-Met and Lys-Ile-His-Pro-Phe conferred high antioxidant activity.14 Furthermore, Shanmugam, Kapila, Sonfack and Kapila (2015) reported that the Ala-Arg-His-Pro-His-Pro-Leu-Ser-Phe peptide from buffalo casein had antioxidant activity.24 Apparently, histidine and proline contribute to relatively high antioxidant activities of peptides. Leucine, valine, and proline residues with the sequence Val-Leu-Pro-Val-Pro have been found in antioxidant peptides. Shanmugam, Kapila, Sonfack and Kapila (2015) obtained the highly active peptide Val-Leu-Pro-Val-Pro-Gln-Lys by hydrolyzing buffalo casein with trypsin.24
Previous research has indicated that the antioxidant activity of a polypeptide is enhanced when one or more residues of histidine, tyrosine, proline, phenylalanine or hydrophobic amino acids were present.33 The carboxyl group of the glutamic acid side chain contributes to antioxidant activity. Polypeptides with aromatic side chains (phenylalanine, tryptophan, and tyrosine) have strong antioxidant activity, mainly because the protons of the aromatic side chain contribute free radicals with antioxidants activity.15 Ghribi et al. (2015) reported that proteolytic digestion of chickpea protein yielded two antioxidant peptides Asp-His-Gly and Val-Gly-Asp-Ile.17 Shazly et al. (2017) identified Arg-Glu-Leu-Glu-Glu and Pro-Tyr-Pro-Gln peptides that had high radical scavenging potential.5
The results of our study are consistent with previous research which has shown that small-molecule peptides have stronger antioxidant potential compared with large-molecule active peptides. Antioxidant polypeptides generally consist of 2–20 different amino acids. Antioxidant activity of peptides is closely related to amino acid sequence. Due to the amino acid sequence, the polypeptide has special properties, and the antioxidant capacity of the active polypeptide is generally higher than the free amino acid.16 Finally, we study evaluated the possible toxicity of the Arg-Glu-Leu-Glu-Glu-Leu peptide by using the computer simulation of ToxinPred (http://crdd.osdd.net/raghava/toxinpred/index.html). The peptide was predicted to be non-toxic.
3.5. Antioxidant activities of synthesized peptides
To further confirm determine the antioxidant activity of peptides Arg-Glu-Leu-Glu-Glu-Leu identified by LC-ESI-MS/MS, we prepared a chemically synthesized peptide of the same sequence. The purity of the synthetic peptide was 98.53%. As shown (Fig. 5), the superoxide anion and hydroxyl radical scavenging activity of synthetic Arg-Glu-Leu-Glu-Glu-Leu peptide were determined (IC50 = 0.52 mg mL−1 and 0.69 mg mL−1), which was compared with GSH. Meanwhile, Shazly et al. (2017) identification of antioxidant peptide RELEE from buffalo and bovine casein hydrolysates has the potential to scavenge free radicals.5 Suetsuna, Ukeda and Ochi has been reported that the C50 values of Glu-Leu isolated from casein and further synthesized antioxidant peptide was 63.1 μM.37 These results confirmed the identity of the purified antioxidant peptide.
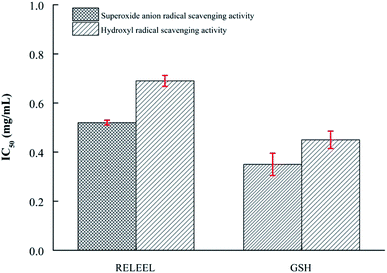 |
| Fig. 5 Superoxide anion and hydroxyl radical scavenging activity (IC50) of synthesized peptides RELEEL and GSH. | |
4. Conclusion
We hydrolyzed yak casein with alcalase and trypsin can effectively produce antioxidant active peptides. The YCH exhibited scavenging activities for superoxide anion, hydroxyl, and DPPH radicals, reducing power and Fe2+ chelating activity. Subsequently, we fractionated the YCH by ultrafiltration. Then, we consecutively fractionated the sample with highest scavenging activity by gel filtration chromatography and semi-preparative RP-HPLC. The results showed that the purified peptide with the highest antioxidant activity was Arg-Glu-Leu-Glu-Glu-Leu (788.85 Da). When compared with the crude YCH, the purified peptide antioxidant scavenging activity was significantly greater. The hydrophobic amino acid leucine, acidic amino acid glutamic, and basic amino acid arginine appeared to contribute to antioxidant activity. All these results indicated that yak casein can be used as a natural source for the production of active compounds. To confirm its in vivo antioxidant activity, it is necessary to evaluate the effect of the synthesized peptides in an appropriate animal model. Yak casein can provide a way to obtain natural antioxidant peptides by enzymatic hydrolysis and purification. Antioxidant peptides isolated and purified from YCH will be more economical and safer than synthetic antioxidants when used as functional ingredients in food. At the same time, the bioavailability and effectiveness of yak casein in vivo should be further studied.
Compliance with ethics requirements
The study did not include any research involving humans and animals.
Conflicts of interest
The authors declare that there are no conflicts of interest.
Acknowledgements
The authors thank the National Natural Science Foundation of China (No. 31560430), and the Key Project of Food Research and Development in Gansu province of China (No. 18YF1NA076) with financial support for this research.
References
- C. F. Chi, F. Y. Hu, B. Wang, T. Li and G. F. Ding, J. Funct. Foods, 2015a, 15, 301–313 Search PubMed.
- C. F. Chi, B. Wang, Y. M. Wang, B. Zhang and S. G. Deng, J. Funct. Foods, 2015b, 12, 1–10 Search PubMed.
- S. C. Ko, D. Kim and Y. J. Jeon, Food Chem. Toxicol., 2012, 50, 2294–2302 CrossRef CAS PubMed.
- X. Hu, X. Yang, T. Wang, L. Li, Y. Wu, Y. Zhou and L. You, Food Chem. Toxicol., 2019, 110882, DOI:10.1016/j.fct.2019.110882.
- A. B. Shazly, Z. He, M. A. El-Aziz, M. Zeng, S. Zhang, F. Qin and J. Chen, Food Chem., 2017, 232, 753–762 CrossRef CAS PubMed.
- H. Korhonen and A. Pihlanto, Curr. Pharm. Des., 2003, 9, 1297–1308 CrossRef CAS PubMed.
- Y. Zhang, H. Zhang, L. Wang, X. Guo, X. Qi and H. Qian, Eur. Food Res. Technol., 2011, 232, 941–950 CrossRef CAS.
- F. Hu, A.-T. Ci, H. Wang, Y.-Y. Zhang, J.-G. Zhang, K. Thakur and Z.-J. Wei, Food Chem., 2018, 261, 301–310 CrossRef CAS.
- B. P. Singh, S. Vij and S. Hati, Peptides, 2014, 54, 171–179 CrossRef CAS.
- Q. Sun, Y. Luo, H. Shen, X. Li and L. Yao, Int. J. Food Sci. Technol., 2011, 47, 148–154 CrossRef.
- A. Zulueta, A. Maurizi, A. Frígola, M. J. Esteve, R. Coli and G. Burini, Int. Dairy J., 2009, 19, 380–385 CrossRef CAS.
- F. M. Assem, M. A. M. Abd El-Gawad, J. M. Kassem and M. H. Abd El-Salam, Int. J. Dairy Technol., 2017, 71, 236–242 CrossRef.
- M. Salami, A. A. Moosavi-Movahedi, F. Moosavi-Movahedi, M. R. Ehsani, R. Yousefi, M. Farhadi, A. Niasari-Naslaji, A. A. Saboury, J.-M. Chobert and T. Haertlé, J. Dairy Res., 2011, 78, 471–478 CrossRef CAS.
- S. A. Ahmed, T. El-Bassiony, L. M. Elmalt and H. R. Ibrahim, Food Res. Int., 2015, 74, 80–88 CrossRef.
- Y. Zhang, Y. Shen, H. Zhang, L. Wang, H. Zhang, H. Qian and X. Qi, Eur. Food Res. Technol., 2017, 244(1), 83–96 CrossRef.
- X. Lou, B. Yuan, L. Wang, H. Xu and M. Hanna, Int. J. Food Sci. Technol., 2019, 55(4), 1679–1688 CrossRef.
- A. M. Ghribi, A. Sila, R. Przybylski, N. Nedjar-Arroume, I. Makhlouf, C. Blecker, H. Attia, P. Dhulster, A. Bougatef and S. Besbes, J. Funct. Foods, 2015, 12, 516–525 CrossRef CAS.
- M. B. O'Keeffe and R. J. FitzGerald, Food Chem., 2015, 184, 140–146 CrossRef PubMed.
- L. Sadat, C. Cakir-Kiefer, M. A. N'Negue, J. L. Gaillard, J. M. Girardet and L. Miclo, Int. Dairy J., 2011, 21, 214–221 CrossRef CAS.
- G. Panchal, S. Hati and A. Sakure, LWT–Food Sci. Technol., 2019, 108887, DOI:10.1016/j.lwt.2019.108887.
- F. Tonolo, A. Folda, L. Cesaro, V. Scalcon, O. Marin, S. Ferro, A. Bindolib and M. P. Rigobello, J. Funct. Foods, 2019, 103696, DOI:10.1016/j.jff.2019.103696.
- X. Ji, X. Li, Y. Ma and D. Li, Food Chem., 2016, 221, 1822–1827 CrossRef.
- X. Y. Mao, H. Y. Yang, J. P. Song, Y. H. Li and F. Z. Ren, J. Agric. Food Chem., 2008, 55, 638–642 CrossRef PubMed.
- V. P. Shanmugam, S. Kapila, T. K. Sonfack and R. Kapila, Int. Dairy J., 2015, 42, 1–5 CrossRef CAS.
- R. Branciari, D. Ranucci, M. Trabalza-Marinucci, M. Codini, M. Orru, R. Ortenzi, F. Claudio, M. R. Ceccarini and A. Valiani, Int. J. Food Sci. Technol., 2014, 50, 558–565 CrossRef.
- X. Li, J. Agric. Food Chem., 2012, 60, 6418–6424 CrossRef CAS PubMed.
- Y. Zhang, X. Duan and Y. Zhuang, Peptides, 2012, 38, 13–21 CrossRef PubMed.
- A. Tai, A. Iomori and H. Ito, Bioorg. Med. Chem., 2017, 25, 5303–5310 CrossRef CAS PubMed.
- F. L. Gu, S. Abbas and X. M. Zhang, LWT–Food Sci. Technol., 2009, 42, 1374–1379 CrossRef CAS.
- J. Carrasco-Castilla, A. J. Hernández-Álvarez, C. Jiménez-Martínez, C. Jacinto-Hernández, M. Alaiz, J. Girón-Calle, J. Vioque and G. Dávila-Ortiz, Food Chem., 2012, 135, 1789–1795 CrossRef CAS PubMed.
- L. You, M. Zhao, J. M. Regenstein and J. Ren, Food Res. Int., 2010, 43, 1167–1173 CrossRef CAS.
- M. J. Tsou, F. J. Kao, C. K. Tseng and W. D. Chiang, Food Chem., 2010, 122, 243–248 CrossRef CAS.
- J. Ren, M. Zhao, J. Shi, J. Wang, Y. Jiang, C. Cui, K. Yukio and S. J. Xue, Food Chem., 2008, 108, 727–736 CrossRef CAS PubMed.
- S.-J. Lee, S. H. Cheong, Y.-S. Kim, J.-W. Hwang, H.-J. Kwon, S.-H. Kang, S.-H. Moon, B.-T. Jeon and P.-J. Park, Food Chem. Toxicol., 2013, 62, 276–280 CrossRef CAS PubMed.
- O. Power, P. Jakeman and R. J. FitzGerald, Amino Acids, 2012, 44, 797–820 CrossRef PubMed.
- A. Sila and A. Bougatef, J. Funct. Foods, 2016, 21, 10–26 CrossRef CAS.
- K. Suetsuna, H. Ukeda and H. Ochi, Mar. Biotechnol., 2000, 2, 5–10 CrossRef CAS PubMed.
|
This journal is © The Royal Society of Chemistry 2020 |
Click here to see how this site uses Cookies. View our privacy policy here.