DOI:
10.1039/D0RA01048K
(Paper)
RSC Adv., 2020,
10, 22176-22182
Coupling hydrophilic interaction chromatography materials with immobilized Fe3+ for phosphopeptide and glycopeptide enrichment and separation†
Received
4th February 2020
, Accepted 24th May 2020
First published on 9th June 2020
1. Introduction
Protein phosphorylation and glycosylation are two types of most significant post-translational modification (PTM), which take part in the regulation of many vital biological processes.1 Reversible protein phosphorylation modulates cellular processes such as cell cycle and signaling.2 Protein glycosylation is related to tumor invasion and metastasis, cell adhesion, and immune response.3 The terminals of glycans in glycoproteins are often modified with sialic acids (SAs).4,5 Increasing evidence shows the increased expression of sialylated glycoproteins on the surface of tumor cells.6 Changes in the level of sialylation interplay with alternation in phosphorylation.7,8 Therefore, to further elucidate these biological processes of protein phosphorylation and glycosylation at the molecular level, it is very essential to simultaneously profile these two kinds of PTMs in biological and clinical samples.
Mass spectrometry (MS) is the method of choice for profiling protein phosphorylation and glycosylation. However, enrichment of PTM-peptides is always required prior to MS analysis owing to the low abundance of PTM-peptides in complex samples and ion suppression effect resulting from non-modified peptides in MS. Therefore, continuous efforts have been endeavored to enrich individual subset of PTM-peptides in order to reduce the complexity of samples. The commonly used methods toward phosphopeptide enrichment include immobilized metal ion affinity chromatography (IMAC),9 metal oxide affinity chromatography (representative with TiO2 and ZrO2),10,11 and hydrophilic interaction chromatography (HILIC).12 Meanwhile, lectin affinity chromatography,13,14 TiO2 (ref. 15–17) and HILIC18,19 have been used for glycopeptide enrichment. During the last decade, HILIC materials have made tremendous progress and widely applied to enrich glycopeptides in the form of Carbon-based materials,20 polymers21 and ZIC-HILIC.22,23
In recent years, several materials have been developed and applied to simultaneous enrichment of phosphopeptides and glycopeptides. These materials include metal oxide affinity chromTiO2/TiO2 (ref. 17, 24 and 25) derivatives, IMAC26,27 and HILIC.26,28,29 Even though these materials could be used to simultaneously capture both phosphopeptides and glycopeptides, these two PTM-peptides are co-eluted from materials, which are still very complex to be analysed with MS. To reduce the complexity of the samples, the second dimensional separation has been employed with HILIC columns or reverse phase liquid chromatography. For example, phosphopeptides and glycopeptides were enriched with TiO2; After the treatment of PNGase F overnight, phosphopeptides and the deglycosylated peptides were subsequently fractionated with HILIC.30,31 However, phosphopeptides and the deglycosylated peptides could be found in each HILIC fraction. The sever overlap of phosphopeptides and deglycosylated peptides in HILIC originates from the insufficient hydrophilicity differences between PTMs peptides. Moreover, removal of glycans is carried out under basic condition overnight, which leads to hydrolyze of co-existing phosphopeptides. Thus, it will be ideal that phosphopeptides are separated from intact glycopeptides before the deglycosylation step.
In our previous study, we synthesized bioinspired polymers Ser-Asp (SD) modified silica (denoted as polySD-SiO2) to enrich glycopeptides.32 In this article, we incorporated iron ions onto polySD-SiO2 materials and used Fe3+ immobilized polySD-SiO2 to simultaneously enrich phosphopeptides and glycopeptides. Then the co-enriched PTM-peptides were further separated into phosphopeptides and intact glycopeptides fractions with polySD-SiO2 under HILIC mode (Scheme 1). This method was validated with the tryptic digests of the phosphoprotein, glycoprotein and non-modified protein and even HeLa S3 cell lysate.
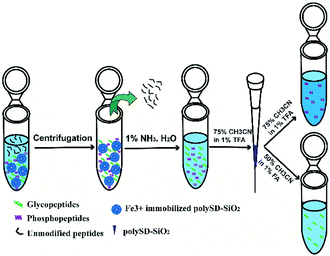 |
| Scheme 1 The process of simultaneous enrichment and subsequent separation of phosphopeptides and glycopeptides with Fe3+ immobilized polySD-SiO2 and polySD-SiO2. | |
2. Experimental
2.1 Materials and reagents
Bovine fetuin, a-casein and bovine serum albumin (BSA), ammonium bicarbonate (NH4HCO3), glycolic acid, guanidine hydrochloride, dl-dithothreitol (DTT), iodoacetamide (IAA), and peptide N-Glycosidase F (PNGase F) were obtained from Sigma-Aldrich (St. Louis, MO, USA). Sequencing grade trypsin was purchased from Promega (Madison, WI, USA). Trifluoroacetic acid (TFA) was obtained from TEDIA (Fairfield, USA). Acetonitrile (ACN) was purchased from Merck (Darmstadt, Germany). Acetic acid and formic acid (FA) was obtained from Acros Organics (Geel, Belgium). Ammonium hydroxide was purchased from Fluka (Buchs, Switzerland). GELoader tips were purchased from Eppendorf (Madison, WI). Water was prepared with a Milli-Q system (Millipore, Bedford, MA, USA).
2.2 Synthesis and characterization of Fe3+ immobilized polySD-SiO2
The previously prepared polySD-SiO2 (500 mg) were suspended in water and washed twice with 1 mL 50 mM EDTA in 1 M NaCl. After centrifugation, the supernatant was removed and the remaining materials were incubated with 1 mL 0.1 M FeCl3 in 0.1 M acetic acid. After incubation of 1 h, the suspension was centrifuged and the pallet was subsequently washed with 1 mL 1 M NaC and 0.1 M acetic acid. After washing with water, the materials were dried under 60 °C. X-ray photoelectron spectroscopy (XPS) was obtained with a VG Multilab 2000. Scanning electron microscopy (SEM) spectra were recorded on a Hitachi S-4800 SEM.
2.3 Trypsin digestion of proteins
Each standard protein (1 mg) was dissolved in 100 μL of 6 M guanidine hydrochloride/50 mM ammonium bicarbonate. The denatured protein solution was reduced with 5 μL of 200 mM DTT/50 mM ammonium bicarbonate for 45 min at 56 °C. Free sulfhydryl groups were alkylated with 20 μL of 200 mM IAA/25 mM ammonium bicarbonate and followed by incubation for 30 min at room temperature in the dark. The solution was diluted to 1 mL with 25 mM ammonium bicarbonate, mixed with trypsin at an enzyme/protein ratio of 1
:
20 (w/w) and incubated overnight at 37 °C. The digestion was stopped by adding 10% formic acid at the final concentration of 1%.
2.4 Enrichment of phosphopeptides and glycopeptides with Fe3+ immobilized polySD-SiO2
Fe3+ immobilized polySD-SiO2 (2.0 mg) was slurried in 40 μL of ACN and pushed into a GELoader tip. The micro-column was equilibrated with 40 μL of loading buffer (80% CAN/5% TFA). The tryptic peptide mixture was dried, redissolved in 40 μL loading buffer and loaded onto the Fe3+ immobilized polySD-SiO2 packed micro-column. The absorbed peptides were washed twice with 40 μL of 80% ACN/5% TFA, and eluted with 40 μL of 1.0% ammonium hydroxide. The elution fraction was immediately acidified with 1% FA.
2.5 Separation of phosphopeptides and glycopeptides with PolySD-SiO2
PolySD-SiO2 packed micro-column was used to separate phosphopeptides from glycopeptides. Elution fraction from Fe3+ immobilized polySD-SiO2 was dried and redissolved in 40 μL of 80% ACN/5% TFA. For micro-column packing, 40 μL of ACN slurry/the PolySD-SiO2 (2.0 mg) was packed into the GELoader tip. After washing with 40 μL of 50% ACN/0.1% FA, the mirco-column was equilibrated with 40 μL of 80% ACN/5% TFA. Then, the peptides were loaded onto the column and the flow-through was collected. The micro-column were rinsed twice with 40 μL of 80% ACN/5% TFA and eluted with 40 μL of 50% ACN/1% TFA. The phosphopeptides were obtained by pooling the flow-through and eluant from 80% ACN/5% TFA. The glycopeptides fraction resulting form 50% ACN/1% TFA was dried and redissolved in 40 μL of 50 mM NH4HCO3. After addition of 2 unit of PNGase F to glycopeptides solution, the solution was incubated overnight at 37 °C.
HeLa cells culture, protein extraction and protein digestion were carried out by using the reported methods.32 The phosphopeptides and glycopeptides were enriched with the abovementioned method.
2.6 Enrichment and separation of phosphopeptides and glycopeptides with the reported method
For the simultaneous enrichment of phosphopeptides and glycopeptides, TiO2 based method was applied as described previously.31 The eluates from TiO2 were deglycosylated with 2 unit of PNGase F. After deglycosylation, the peptides were desalted and subsequently subjected to HILIC mirco-column packed with TSKGel Amide materials (3 μm particle size, Tosoh Bioscience). The mirco-column were eluted with 40 μL of 75% ACN/0.1% TFA and eluted with 40 μL of 50% ACN/0.1% TFA, respectively.
2.7 Validating the established method
To investigate the recovery of the established method, stable isotope dimethyl labeling protocol was followed.34 The standard monophosphopeptide (peptide sequence: HpSPIAPSSPSPK, p: phosphorylation site), diphosphopeptide (peptide sequence: HpSPIApPSSPSPK, p: phosphorylation site) and a glycopeptide (peptide sequence: KVANKT, N: glycosylation site) were used in this experiments. Each standard peptide was dissolved in sodium acetate buffer (0.1 M, pH 6) and mixed with formaldehyde (4% in water, 1 μL) and formaldehyde-D2 (4% in water, 1 μL), separately. After vortex for 5 min, the solution was then mixed immediately with freshly prepared sodium cyanoborohydride (260 mM, 1 μL). The mixture was vortexed again and then allowed to react for 5 min. After the stable isotope dimethyl labeling, the heavily labeled standard peptides were treated with the established. Before the treated samples were injected for MS analysis, the same amount of lightly labeled standard peptides was mixed with the treated samples. The recovery was calculated based on the intensity of lightly and heavily labeled standard peptides.
2.8. MS analysis and data searching
The peptide fractions from digests of standard samples were characterized with nano electrospray ionization (ESI)-quadrupole time-of-flight (Q-TOF) tandem mass spectrometer (Waters, Manchester, UK). Modified peptides from HeLa S3 cell lysate were analyzed were analyzed by LTQ Orbitrap Velos (Thermo, San Jose, CA) with Accela 600 HPLC system (Thermo, San Jose, CA). The peptides were loaded on a C18 capillary trap column (150 μm i.d. × 4 cm) packed with C18 AQ beads (5 μm, 120 Å) by a void capillary as previous described. The peptides were separated on analytical column (75 μm i.d. ×15 cm) packed with C18 AQ beads (3 μm, 120 Å). The mobile phases for separation were 0.1% FA in water and 0.1% FA in ACN, the flow rate was 200 nL min−1 for LC-MS/MS analysis. The gradient from 5 to 35% (v/v) ACN was performed in 150 min. The MS and MS/MS spectra were collected by CID at 35% energy in a data dependent mode that 1 MS scan from 400 to 1850 m/z with the mass resolution of 60
000 at m/z 400 in the Orbitrap analyzer followed with 20 MS/MS scans in the ion trap, respectively. The dynamic exclusion repeat count was 1 with duration time of 30 s, the exclusion list size was 500 with exclusion time of 60 s, and the charge state rejection function was enabled, with single and “unassigned” charge states rejected.
The RAW files collected by Xcalibur 2.1 were converted to *.MGF by Proteome Discoverer (v1.2.0.208, Thermo, San Jose, CA) and searched with Mascot (version 2.3.0, Matrix Science, London, UK). Cysteine carboxamidomethylation was set as a fixed modification of 57.0215 Da, oxidation of methionine, deamidation of asparagine and phosphorylation of serine, threonine and tyrosine were set as variable modifications. Up to two missing cleavages of trypsin were allowed. Mass tolerances were 20 ppm and 0.2 Da for the parent and fragment ions, respectively. A false discovery rate of 1% was set at both the peptide and site levels.
3. Results and discussion
3.1 Preparation of Fe3+ immobilized polySD-SiO2
The Fe3+ immobilized polySD-SiO2 materials were prepared by incubation of polySD-SiO2 with FeCl3. The prepared materials were characterized with scanning electron microscope (SEM) and XPS. SEM images indicated that the materials maintained their integrity after incorporating with Fe3+ (Fig. 1a and b). The XPS spectrum implies that Fe3+ has been modified on the polySD-SiO2 (Fig. 1c). These results indicated that Fe3+ ions modified polySD-SiO2 materials have been successfully prepared.
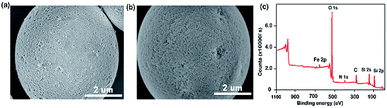 |
| Fig. 1 Characterization of Fe3+ immobilized polySD-SiO2 with SEM (a and b) and XPS (c). (a and b) SEM image of materials before (a) and after(b) immobilization wih Fe3+. | |
3.2 Enrichment of phosphopeptides and glycopeptides with Fe3+ immobilized polySD-SiO2
To investigate whether Fe3+ immobilized polySD-SiO2 can enrich glycopeptides, tryptic digests of sialoglycoprotein bovine fetuin and bovine serum albumin at molar ratio of 1
:
100 were used as model samples. The number of glycopeptide signals is 30 (detailed information of glycopeptides in Table S1†) with the Fe3+ immobilized polySD-SiO2 materials (Fig. S1†), which is comparable to that reported with polySD-SiO.28 To further enrich both phosphopeptides and glycopeptides, tryptic digests of phosphoprotein a-casein and fetuin were used as model samples. At first, the tryptic digests of a-casein and fetuin at molar ratio of 1
:
3 were used to evaluate the prepared materials. Fig. 2 demonstrates that the mass spectra of peptides before and after treatment with Fe3+ immobilized polySD-SiO2. Before enrichment with our materials, the mass spectrum was occupied with signals of non-modified peptides. The signals of glycopeptides could not be detected and only two phosphopeptides could be observed (Fig. 2a). The poor signals of PTM-peptides were mainly resulted from their low abundance. After enrichment with our materials, the signals of glycopeptides and phosphopeptides dominated the mass spectrum because the abundant non-PTM peptides were thoroughly removed. A total of 7 phosphopeptide signals (detailed information of phosphopeptides in Table S2†) and 20 glycopeptides could be readily found (Fig. 2b). Even 200 molar folds of BSA was mixed as interference with the tryptic digests of a-casein and fetuin at molar ratio of 1
:
3, our materials maintained its selectivity toward both glycopeptides signals and phosphopeptides. There are still 6 phosphopeptides and 19 glycopeptide signals could be easily found in the mass spectrum (Fig. 3a). This result implied that Fe3+ immobilized polySD-SiO2 could selectively enrich both glycopeptides and phosphopeptides.
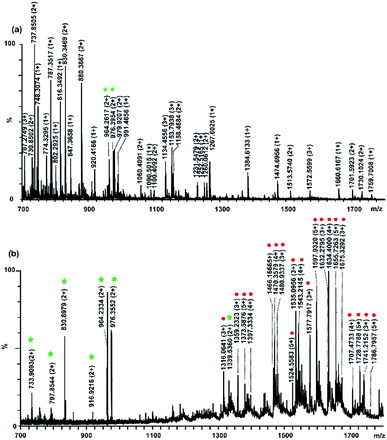 |
| Fig. 2 MS spectra obtained from the tryptic digests of a-casein and fetuin before (a) and after (b) treatment with Fe3+ immobilized polySD-SiO2 (b). The tryptic digests were a-casein: fetuin at molar ratio of 1 : 3. The phosphopeptides and glycopeptides are marked with green asterisks and red circles, respectively. | |
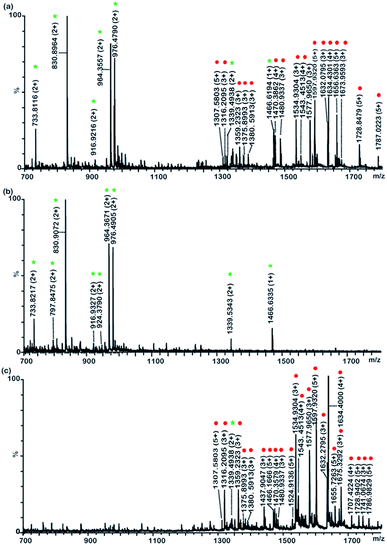 |
| Fig. 3 MS spectra obtained from the tryptic digests of a-casein and fetuin at molar ratio of 1 : 2 : 400 after enrichment with Fe3+ immobilized polySD-SiO2 (a) and separation with PolySD-SiO2 (b and c). (a) Phosphopeptides and glycopeptides enrichment with polySD-SiO2; b: phosphopeptides fraction treated with Fe3+ immobilized polySD-SiO2 and PolySD-SiO2; (c): glycopeptides fraction treated with Fe3+ immobilized polySD-SiO2 and PolySD-SiO2; the phosphopeptides and glycopeptides are marked with green asterisks and red circles, respectively. | |
3.3 Separation of phosphopeptides and glycopeptides with polySD-SiO2
After glycopeptides and phosphopeptides were eluted from Fe3+ immobilized polySD-SiO2, these two species were further separated with PolySD-SiO2 under HILIC mode. In order to reduce the retention of charged peptides and enlarge the hydrophilicity difference between glycopeptides and non-glycosylated peptides, 5% TFA was added to the mobile phases.33 The tryptic digests of a-casein: fetuin: BSA = 1
:
2
:
400 (molar ratio) were treated with Fe3+ immobilized polySD-SiO2. After the treatment our materials, the high-abundance non-modified peptides were efficiently removed and 7 phosphopeptide signals and 16 glycopeptides were readily observed in the mass spectrum of the eluates. After subsequent separation with polySD-SiO2 under HILIC mode, phosphopeptides and glycopeptides were efficiently separated into two fractions. Because the further separation reduced the complexity of sample and also decreased the ion suppression effect in MS, nine phosphopeptide signals and 22 glycopeptide signals were easily detected in the mass spectrum of phosphopeptides (Fig. 3b) and glycopeptides fraction (Fig. 3c), respectively. Glycopeptides couldn't be observed in the phosphopeptide fraction, while very low intensities of one triple phosphopeptide (m/z = 1339.5343) were found in the glycopeptide fraction, which might result from the strong electrostatic interaction between the triple phosphopeptides and polySD-SiO2 materials. These results demonstrated that Fe3+ immobilized polySD-SiO2 enrichment coupled with PolySD-SiO2 fractionation could efficiently separate phosphopeptides and glycopeptides from the peptide pool and further divide these two kinds of PTM-peptides into two fractions.
3.4 Enrichment and separation of phosphopeptides and glycopeptides with the reported method
We then compared the efficiency of previously reported method toward phosphopeptides and glycopeptides.31 At first, the tryptic digests of a-casein: fetuin: BSA = 1
:
2
:
400 (molar ratio) were treated with TiO2. The phosphopeptides and glycopeptides could be enriched with TiO2 and 7 phosphopeptide signals and 12 glycopeptide signals could be detected (Fig. 4a), while some non-PTM peptides could also be detected, such as peptide at m/z 7874.3626(2+). Then the enriched peptides from TiO2 was further deglycosylated with PNGase F to remove glycans. The deglycosylated peptides and phosphopeptides were further separated with TSKGel Amide mirco-column under HILIC mode. Only 4 phosphopeptide signals and 4 deglycosylated peptide signals were found in the 75% ACN/0.1% TFA fraction (Fig. 4b), while there were still 2 multiple phosphopeptides and 1 deglycosylated peptides in the 50% ACN/0.1% TFA fraction. It turns out that phosphopeptides and deglycosylated peptides were co-eluted in both two fractions, which is consistent with the previous report.31 Taking together the afore-mentioned results, these data demonstrated that phosphopeptides and deglycosylated peptides overlapped in these two fractions. It is beneficial to separate phosphopepides and glycopeptides before deglycosylation with HILIC column.
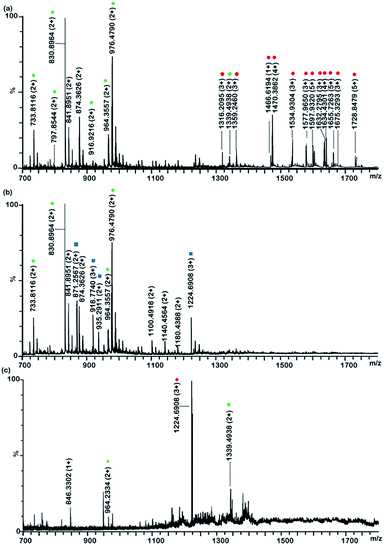 |
| Fig. 4 MS spectra obtained from the tryptic digests of a-casein and fetuin at molar ratio of 1 : 2 : 400 after enrichment with TiO2 (a) and separation of phosphopeptides and deglycosylated peptides with TSKGEL-amide (b and c). (a): Phosphopeptides and glycopeptides enrichment with TiO2; (b): peptides eluted from TSKGEL-amide with 75% ACN/0.1% TFA; (c): peptides eluted from TSKGEL-amide with 50% ACN/0.1% TFA. The phosphopeptides, glycopeptides and deglycosylated glycopeptides are marked with green asterisks, red circles and blue squares, respectively. | |
3.5 The validation of the developed strategy
To evaluate the recovery of the developed strategy, stable isotope dimethyl labeling method were employed (details in 2.7 Validating the established method).34 The average recovery was 85.2 ± 3.2% (n = 3), 79.2 ± 4.1% and 84.6 ± 2.3% (n = 3) for standard momo-phosphopeptide, di-phosphopeptide and glycopeptide, respectively. For comparison, the recovery of the reported method was also performed, which separate phosphopeptides and glycan removed glycopeptides after TiO2 enrichment. It turned out that the recovery of the reported method was much lower than that obtained with our developed protocol. They were 53.3 ± 1.8% and 62.9 ± 3.4% (n = 3) for phosphopeptides and glycopeptides, respectively. To investigate the reproducibility of the established strategy, three parallel experiments were performed to enrich and separate phosphopeptides and glycopeptides from the tryptic digests of a-casein and fetuin at molar ratio of 1
:
3. The number of identified phosphopeptides was 9, 9 and 10 in three experiments and the number of detected glycopeptides was 20, 21 and 20 in three experiments. These results demonstrated that proposed method is reproducible and superior to the reported one in the aspect of recovery.
The developed strategy was used for identification of phosphopeptides and glycopeptides from 20 μg HeLa S3 cell lysate. The cell lysate was digested with trypsin and the tryptic peptides were treated with our established method. The phosphopeptides fraction and the deglycosylated glycopeptides fraction were analyzed by Orbitrap mass spectrometer. The selectivity of the established method for phosphopeptides and glycopeptides was 75.3%. More than 1903 phosphopeptides from 542 proteins were characterized in the phosphopeptides fraction (Table S3†) and 139 unique sites from 83 glycoproteins in glycopeptides fraction (Table S4†). No glycopeptide was detected in the phosphopeptides fraction while 20 multiply phosphorylated peptides were characterized in the glycopeptide fraction. This overlapping ratio between phosphopeptides and glycopeptides achieved with our strategy (<5%) is much lower than that with the literature (43%).31 The result further demonstrated the advantages of our method for enrichment and fractionation of phosphopeptides and glycopeptides.
4. Conclusions
In summary, we have developed an efficient method for simultaneous enrichment and subsequent separation of phosphopeptides and glycopeptides, taking advantages of the strong affinity between Fe3+ immobilized polySD-SiO2 and PTM-peptides and the hydrophilicity difference between phosphopeptides and glycopeptides on polySD-SiO2. The established method has the advantages of high recovery and selectivity toward the target peptides and low overlapping between phosphopeptides and glycopeptides. We provide a powerful tool to tackle the challenge of simultaneous and selective analysis of phosphopeptides and glycopeptides. This tool may promote the discovery of more PTM-sites from limited amount of biosamples, especially clinic samples.
Conflicts of interest
There are no conflicts to declare.
Acknowledgements
We thank the National Natural Science Foundation of China (81472487 and 81773197), the Natural Science Foundation of Hubei Province (2017CFB412).
References
- A. Bah, R. M. Vernon, Z. Siddiqui, M. Krzeminski, R. Muhandiram, C. Zhao, N. Sonenberg, L. E. Kay and J. D. Forman-Kay, Folding of an intrinsically disordered protein by phosphorylation as a regulatory switch, Nature, 2015, 519, 106–U240 CrossRef CAS PubMed.
- J.-J. Chung, S.-H. Shim, R. A. Everley, S. P. Gygi, X. Zhuang and D. E. Clapham, Structurally Distinct Ca2+ Signaling Domains of Sperm Flagella Orchestrate Tyrosine Phosphorylation and Motility, Cell, 2014, 157, 808–822 CrossRef CAS PubMed.
- S. S. Pinho and C. A. Reis, Glycosylation in cancer: mechanisms and clinical implications, Nat. Rev. Cancer, 2015, 15, 540–555 CrossRef CAS PubMed.
- M. Perdicchio, J. M. Ilarregui, M. I. Verstege, L. A. M. Cornelissen, S. T. T. Schetters, S. Engels, M. Ambrosini, H. Kalay, H. Veninga, J. M. M. den Haan, L. A. van Berkel, J. N. Samsom, P. R. Crocker, T. Sparwasser, L. Berod, J. J. Garcia-Vallejo, Y. van Kooyk and W. W. J. Unger, Sialic acid-modified antigens impose tolerance via inhibition of T-cell proliferation and de novo induction of regulatory T cells, Proc. Natl. Acad. Sci. U. S. A., 2016, 113, 3329–3334 CrossRef CAS PubMed.
- I. Quast, C. W. Keller, M. A. Maurer, J. P. Giddens, B. Tackenberg, L.-X. Wang, C. Muenz, F. Nimmerjahn, M. C. Dalakas and J. D. Luenemann, Sialylation of IgG Fc domain impairs complement-dependent cytotoxicity, J. Clin. Invest., 2015, 125, 4160–4170 CrossRef PubMed.
- Y. Ren, X. Liu, R. Geng, Q. Lu, R. Rao, X. Tan, X. Yang and W. Liu, Increased Level of alpha 2,6-Sialylated Glycans on HaCaT Cells Induced by Titanium Dioxide Nanoparticles under UV Radiation, Materials, 2018, 8, 253 Search PubMed.
- F. Liu, K. Iqbal, I. Grundke-Iqbal and C. X. Gong, Involvement of aberrant glycosylation in phosphorylation of tau by cdk5 and GSK-3 beta, FEBS Lett., 2002, 530, 209–214 CrossRef CAS PubMed.
- A. C. Leney, D. El Atmioui, W. Wu, H. Ovaa and A. J. R. Heck, Elucidating crosstalk mechanisms between phosphorylation and O-GlcNAcylation, Proc. Natl. Acad. Sci. U. S. A., 2017, 114, E7255–E7261 CrossRef CAS PubMed.
- D. Jiang, X. Li, J. Ma and Q. Ji, Development of Gd3+-immobilized glutathione-coated magnetic nanoparticles for highly selective enrichment of phosphopeptides, Talanta, 2018, 180, 368–375 CrossRef CAS PubMed.
- T. E. Thingholm, T. J. D. Jorgensen, O. N. Jensen and M. R. Larsen, Highly selective enrichment of phosphorylated peptides using titanium dioxide, Nat. Protoc., 2006, 1, 1929–1935 CrossRef CAS PubMed.
- I. Kuroda, Y. Shintani, M. Motokawa, S. Abe and M. Furuno, Phosphopeptide-selective column-switching RP-HPLC with a titania precolumn, Anal. Sci., 2004, 20, 1313–1320 CrossRef CAS PubMed.
- D. E. McNulty and R. S. Annan, Hydrophilic interaction chromatography reduces the complexity of the phosphoproteome and improves global phosphopeptide isolation and detection, Mol. Cell. Proteomics, 2008, 7, 971–980 CrossRef CAS PubMed.
- J. T. Waniwan, Y.-J. Chen, R. Capangpangan, S.-H. Weng and Y.-J. Chen, Glycoproteomic Alterations in Drug-Resistant Nonsmall Cell Lung Cancer Cells Revealed by Lectin Magnetic Nanoprobe-Based Mass Spectrometry, J. Proteome Res., 2018, 17, 3761–3773 CrossRef CAS PubMed.
- J. Zhou, W. Yang, Y. Hu, N. Hoti, Y. Liu, P. Shah, S. Sun, D. Clark, S. Thomas and H. Zhang, Site-Specific Fucosylation Analysis Identifying Glycoproteins Associated with Aggressive Prostate Cancer Cell Lines Using Tandem Affinity Enrichments of Intact Glycopeptides Followed by Mass Spectrometry, Anal. Chem., 2017, 89, 7623–7630 CrossRef CAS PubMed.
- M. R. Larsen, S. S. Jensen, L. A. Jakobsen and N. H. H. Heegaard, Exploring the sialiome using titanium dioxide chromatography and mass spectrometry, Mol. Cell. Proteomics, 2007, 6, 1778–1787 CrossRef CAS PubMed.
- D. Jiang, X. Li, X. Lv and Q. Jia, A magnetic hydrazine-functionalized dendrimer embedded with TiO2 as a novel affinity probe for the selective enrichment of low-abundance phosphopeptides from biological samples, Talanta, 2018, 185, 461–468 CrossRef CAS PubMed.
- D. P. Xu, G. Q. Yan, M. X. Gao, C. H. Deng and X. M. Zhang, Highly selective SiO2-NH2@TiO2 hollow microspheres for simultaneous enrichment of phosphopeptides and glycopeptides, Anal. Bioanal. Chem., 2017, 409, 1607–1614 CrossRef CAS PubMed.
- G. Jin, D. Yu, Z. Guo, D. Yang, H. Zhang, A. Shen, J. Yan and X. Liang, Preparation of glyco-silica materials via thiol-ene click chemistry for adsorption and separation, RSC Adv., 2016, 6, 8584–8587 RSC.
- X. Dong, H. Qin, J. Mao, D. Yu, X. Li, A. Shen, J. Yan, L. Yu, Z. Guo, M. Ye, H. Zou and X. Liang, In-depth analysis of glycoprotein sialylation in serum using a dual-functional material with superior hydrophilicity and switchable surface charge, Anal. Chem., 2017, 89, 3966–3972 CrossRef CAS PubMed.
- Y. Zhang, H. Jing, T. Wen, Y. Wang, Y. Zhao, X. Wang, X. Qian and W. Ying, Phenylboronic acid functionalized C3N4 facultative hydrophilic materials for enhanced enrichment of glycopeptides, Talanta, 2019, 191, 509–518 CrossRef CAS PubMed.
- H. Park, S. Walta, R. R. Rosencrantz, A. Koerner, C. Schulte, L. Elling, W. Richtering and A. Boeker, Micelles from self-assembled double-hydrophilic PHEMA-glycopolymer-diblock copolymers as multivalent scaffolds for lectin binding, Polym. Chem.c, 2016, 7, 878–886 RSC.
- C. Xia, F. Jiao, F. Gao, H. Wang, Y. Lv, Y. Shen, Y. Zhang and X. Qian, Two-dimensional mos2-based zwitterionic hydrophilic interaction liquid chromatography material for the specific enrichment of glycopeptides, Anal. Chem., 2018, 90, 6651–6659 CrossRef CAS PubMed.
- Y. Chen, Z. Xiong, L. Zhang, J. Zhao, Q. Zhang, L. Peng, W. Zhang, M. Ye and H. Zou, Facile synthesis of zwitterionic polymer-coated core-shell magnetic nanoparticles for highly specific capture of N-linked glycopeptides, Nanoscale, 2015, 7, 3100–3108 RSC.
- J. Y. Yan, X. L. Li, L. Yu, Y. Jin, X. L. Zhang, X. Y. Xue, Y. X. Ke and X. M. Liang, Selective enrichment of glycopeptides/phosphopeptides using porous titania microspheres, Chem. Commun., 2010, 46, 5488–5490 RSC.
- D. P. Xu, M. X. Gao, C. H. Deng and X. M. Zhang, Synthesis of bifunctional TiO2@SiO2-B(OH)@Fe3O4@TiO2 sandwich-like nanosheets for sequential selective enrichment of phosphopeptides and glycopeptides for mass spectrometric analysis, Anal. Bioanal. Chem., 2016, 408, 5489–5497 CrossRef CAS PubMed.
- X. J. Zou, J. Z. Jie and B. Yang, Single-Step Enrichment of N-Glycopeptides and Phosphopeptides with Novel Multifunctional Ti4+-Immobilized Dendritic Polyglycerol Coated Chitosan Nanomaterials, Anal. Chem., 2017, 89, 7520–7526 CrossRef CAS PubMed.
- Y. Hong, H. Zhao, C. Pu, Q. Zhan, Q. Sheng and M. Lan, Hydrophilic Phytic Acid-Coated Magnetic Graphene for Titanium(IV) Immobilization as a Novel Hydrophilic Interaction Liquid Chromatography-Immobilized Metal Affinity Chromatography Platform for Glyco- and Phosphopeptide Enrichment with Controllable Selectivity, Anal. Chem., 2018, 90, 11008–11015 CrossRef CAS PubMed.
- J. X. Wang, Y. A. Wang, M. X. Gao, X. M. Zhang and P. Y. Yang, Facile synthesis of hydrophilic polyamidoxime polymers as a novel solid-phase extraction matrix for sequential characterization of glyco- and phosphoproteomes, Anal. Chim. Acta, 2016, 907, 69–76 CrossRef CAS PubMed.
- P. Hao, H. Zhang and S. K. Sze, Application of electrostatic repulsion hydrophilic interaction chromatography to the characterization of proteome, glycoproteome, and phosphoproteome using nano LC-MS/MS, Methods Mol. Biol., 2011, 790, 305–318 CrossRef CAS PubMed.
- G. Palmisano, B. L. Parker, K. Engholm-Keller, S. E. Lendal, K. Kulej, M. Schulz, V. Schwammle, M. E. Graham, H. Saxtorph, S. J. Cordwell and M. R. Larsen, A Novel Method for the Simultaneous Enrichment, Identification, and Quantification of Phosphopeptides and Sialylated Glycopeptides Applied to a Temporal Profile of Mouse Brain Development, Mol. Cell. Proteomics, 2012, 11, 1191–1202 CrossRef PubMed.
- M. N. Melo-Braga, M. Schulz, Q. Liu, A. Swistowski, G. Palmisano, K. Engholm-Keller, L. Jakobsen, X. Zeng and M. R. Larsen, Comprehensive Quantitative Comparison of the Membrane Proteome, Phosphoproteome, and Sialiome of Human Embryonic and Neural Stem Cells, Mol. Cell. Proteomics, 2014, 13, 311–328 CrossRef PubMed.
- B. Zhang, R. Z. Yu, Y. H. Yu, C. Peng, R. Xie, Y. Zhang and J. Y. Chen, Lectin inspired polymers based on the dipeptide Ser-Asp for glycopeptide enrichment, Analyst, 2018, 143, 5090–5093 RSC.
- S. Mysling, G. Palmisano, P. Hojrup and M. Thaysen-Andersen, Utilizing Ion-Pairing Hydrophilic Interaction Chromatography Solid Phase Extraction for Efficient Glycopeptide Enrichment in Glycoproteomics, Anal. Chem., 2010, 82, 5598–5609 CrossRef CAS PubMed.
- O. Kleifeld, A. Doucet, A. Prudova, U. A. D. Keller, M. Gioia, J. N. Kizhakkedathu and C. M. Overall, Identifying and quantifying proteolytic events and the natural N terminome by terminal amine isotopic labeling of substrates, Nat. Protoc., 2011, 6, 1578–1611 CrossRef CAS PubMed.
Footnotes |
† Electronic supplementary information (ESI) available. See DOI: 10.1039/d0ra01048k |
‡ These authors contributed equally to this work. |
|
This journal is © The Royal Society of Chemistry 2020 |
Click here to see how this site uses Cookies. View our privacy policy here.