DOI:
10.1039/D0RA01043J
(Paper)
RSC Adv., 2020,
10, 8586-8593
The synthesis of quinolines via denitrogenative palladium-catalyzed cascade reaction of o-aminocinnamonitriles with arylhydrazines†
Received
3rd February 2020
, Accepted 20th February 2020
First published on 3rd March 2020
Abstract
The first example of the palladium-catalyzed cascade reaction of o-aminocinnamonitriles with arylhydrazines has been achieved, providing an efficient synthetic pathway to access quinolines with moderate to good yields. Preliminary mechanistic experiments indicate that this cascade process involves sequential denitrogenative addition followed by an intramolecular cyclization.
Introduction
Quinoline derivatives have become increasingly popular in the past few years due to their ubiquity in natural products, bioactive compounds, and other functional molecules. For example, quinoline scaffolds are privileged structures in medicinal chemistry, and therapeutic agents with such cores on the market or in clinical trials for the treatment of urological malignancies such as renal cell carcinoma (e.g., lenvatinib,1 cabozantinib2) and the treatment of head and neck cancer (e.g., camptothecin3) (Fig. 1). Therefore, the development of the effective methods for the synthesis of quinolines has been actively pursued during the past several decades.4 However, less attention has been paid to the preparation of quinolines from nitriles.
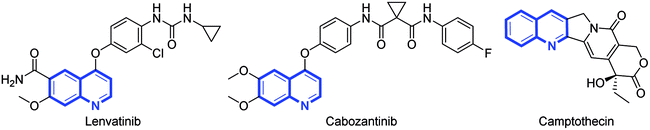 |
| Fig. 1 Representative bioactive quinoline derivatives. | |
Nitriles are well-known as solvents or ligands in organometallic reactions because of the inherently inert nature of the C
N bond.5 For example, acetonitrile or benzonitrile are usually used as solvents or ligands in many transition-metal-catalyzed reactions.6 The development of transition-metal-catalyzed inert C
N bond activation and further transformation has received significant attention from organic chemists because nitriles are normally very stable and abundant and cheap feedstock chemicals. Substantial progress towards this goal made in recent years, the transition-metal-catalyzed transformation of nitriles offers an attractive route for the synthesis of arylketones or further cyclization products by several other groups.7 Our group also developed transition-metal-catalyzed a series of tandem addition/cyclization reaction of nitriles with organoboron reagents for the synthesis of structurally diverse 5-membered, 6-membered, and 7-membered N-heterocycles.8 In addition, arylhydrazines are used as an important class of molecular building blocks in both synthetic and medicinal chemistry for preparing various nitrogen-containing compounds because of their high reactivity, low cost, and ready availability.9 Recent progresses in the development of using arylhydrazines as aryl source by denitrogenation have been documented.10 Despite the remarkable advances in transition-metal-catalyzed addition reactions of nitriles with arylating reagents into a valuable array of products to date, less attention has been paid to the using arylhydrazines as aryl sources by denitrogenation presumably due to the high dissociation energy from direct activation of the C–N bond.11 To our knowledge, only two examples of denitrogenative palladium-catalyzed reaction of nitriles with arylhydrazines for the synthesis of aryl ketones have been reported (Scheme 1a).12
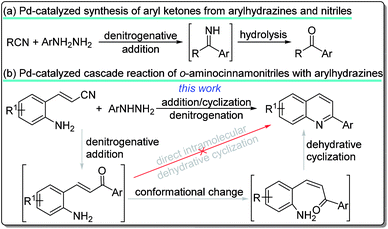 |
| Scheme 1 Design of new approach to quinolines. | |
We envisioned that a Pd-catalyzed sequential denitrogenative addition followed by an intramolecular dehydrative cyclization of readily available o-aminocinnamonitriles with arylhydrazines would result in a tandem procedure for the preparation of 2-arylquinolines (Scheme 1b). It is worth noting that the amino group cannot approach the carbonyl group to achieve the intramolecular cyclization because of the steric configuration. Recently, Cheon group developed the synthesis of 2-substituted quinolines by the dehydrative cyclization of 2-aminostyryl ketones.13 These strategies involve the change of unreactive (E)-2-aminostyryl ketones into the unstable but reactive Z-configuration intermediate to undergo dehydrative cyclization reaction by using iodide13a or benzylamine13b as the nucleophilic catalyst. Recently, we reported the Pd-catalyzed tandem reaction of 2-aminostyryl nitriles with arylboronic acids for the synthesis of 2-arylquinolines.14 As part of the continuing efforts in our laboratory toward the development of novel transformations of nitriles,8,14 we herein report the first example of the denitrogenative Pd-catalyzed cascade reaction of o-aminocinnamonitriles with arylhydrazines to afford 2-arylquinolines (Scheme 1b).
Results and discussion
We begin our investigation with readily available (E)-3-(2-aminophenyl)but-2-enenitrile (1a) and phenylhydrazine (2a) for the optimization of reaction conditions (Table 1).
Table 1 Optimization of reaction conditionsa
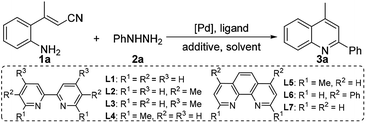
|
Entry |
[Pd] |
Ligand |
Additive |
Solvent |
Yieldb (%) |
Conditions: 1a (0.3 mmol), 2a (0.6 mmol), Pd catalyst (10 mol%), ligand (20 mol%), additive (2 equiv.), solvent (2 mL), 90 °C, 24 h, air. Isolated yield. Under O2. |
1 |
Pd(PPh3)4 |
L1 |
TFA |
THF |
0 |
2 |
Pd(OM)2 |
L1 |
TFA |
THF |
12 |
3 |
Pd(CF3CO2)2 |
L1 |
TFA |
THF |
19 |
4 |
Pd(PPh3)2Cl2 |
L1 |
TFA |
THF |
17 |
5 |
PdCl2 |
L1 |
TFA |
THF |
31 |
6 |
Pd2(dba)3 |
L1 |
TFA |
THF |
21 |
7 |
Pd(acac)2 |
L1 |
TFA |
THF |
23 |
8 |
PdCl2 |
L1 |
TFA |
2-MeTHF |
37 |
9 |
PdCl2 |
L1 |
TFA |
DMF |
41 |
10 |
PdCl2 |
L1 |
TFA |
DMA |
47 |
11 |
PdCl2 |
L1 |
TFA |
1,4-Dioxane |
23 |
12 |
PdCl2 |
L1 |
TFA |
Toluene |
56 |
13 |
PdCl2 |
L1 |
TFA |
H2O |
18 |
14 |
PdCl2 |
L2 |
TFA |
Toluene |
53 |
15 |
PdCl2 |
L3 |
TFA |
Toluene |
51 |
16 |
PdCl2 |
L4 |
TFA |
Toluene |
Trace |
17 |
PdCl2 |
L5 |
TFA |
Toluene |
Trace |
18 |
PdCl2 |
L6 |
TFA |
Toluene |
41 |
19 |
PdCl2 |
L7 |
TFA |
Toluene |
51 |
20 |
PdCl2 |
L1 |
AcOH |
Toluene |
Trace |
21 |
PdCl2 |
L1 |
TsOH·H2O |
Toluene |
33 |
22 |
PdCl2 |
L1 |
MsOH |
Toluene |
21 |
23 |
PdCl2 |
L1 |
TfOH |
Toluene |
79(81)c |
24 |
|
L1 |
TfOH |
Toluene |
0 |
25 |
PdC12 |
|
TfOH |
Toluene |
0 |
We initially found that the desired product 2-phenylquinoline (3a) could be obtained in 12% yield under the combination of Pd(OAc)2, trifluoroacetic acid (TFA) and 2,2′-bipyridine (L1) in THF under air atmosphere (entry 2). Various palladium catalysts were tested (entries 3–7) and PdCl2 exhibited the highest catalytic reactivity in 31% yield (entry 5). Delightedly, the yield of 3a could be improved to 56% using toluene as the solvent. Other solvents, including DMF, dimethylacetamide (DMA), 1,4-dioxane and H2O were less efficient (entries 8–13). Replacement of L1 with other bidentate N-ligands (entries 13–19), resulted in lower yields. However, trace amounts of 3a was detected when sterically hindered ligands, such as 6,6′-dimethyl-2,2′-bipyridine (L4) and 2,9-dimethyl-1,10-phenanthroline (L5) were used (entries 16–17). An investigation of the effect of additive revealed that the yield of 3a was greatly increased to 81% under O2 atmosphere (entry 23). The product 3a was not observed if either Pd catalyst or ligand was absent (entries 24 and 25).
Having the optimized reaction conditions in hand, we examined the substrates scope of this cascade reaction. First, the cascade reaction of (E)-3-(2-aminophenyl)but-2-enenitrile (1a) with different arylhydrazines were evaluated under optimized conditions (Table 2). The reactivities of para-, meta-, and ortho-tolylhydrazine were evaluated, and the results demonstrated that the steric effect of the substituent had an obvious impact on the reaction. For example, the reactions of 1a with para- and meta-tolylhydrazine afforded 85% and 79% yields of 3b and 3c, respectively, while the ortho-tolylhydrazine gave the desired product 3d with a diminished yield of 52%. Moderately electron-withdrawing halogens, such as fluoro (3e–3f), chloro (3g), and bromo (3h) groups, were compatible with this reaction, providing 42–76% yields. The substrate bearing a strong electron-withdrawing group (e.g., CF3) could also be well tolerated, albeit giving the desired 3i in a slightly lower yield.
Table 2 Denitrogenative Pd-catalyzed cascade reaction of 1a with arylhydrazinesa
Conditions: 1a (0.3 mmol), 2 (0.6 mmol), PdCl2 (10 mol%), L1 (20 mol%), toluene (2 mL), TfOH (2 equiv.), 90 °C, 24 h, O2, isolated yield. |
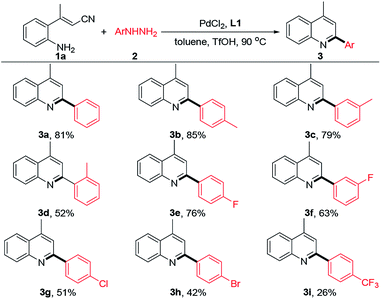 |
We next turned our attention to the effect of the reactions between substituted o-aminocinnamonitriles (1) and phenylhydrazine (2a) under standard conditions (Table 3). First, the influence of a variety of functional groups (R1) on the phenyl ring of the 2-(benzylideneamino)benzonitriles was evaluated. Both electron-donating groups, such as methyl (3j) and methoxy (3k) moieties, and electron-withdrawing groups, such as fluoro (3l–3m), chloro (3n–3p) and bromo (3q) moieties, were also compatible, affording the corresponding desired products with moderate to good yields. Treatment of a strong electron-withdrawing trifluoromethyl substituent with 2a delivered product 3r in 36% yield. Finally, several representative examples of substituents (R2) on the carbon–carbon double bond were investigated. The R2-substituted products 3t, 3u and 3u was obtained in 71%, 62% and 57% yields, respectively, compared to relatively low yield of the product 3s in 51% yield. It is worth noting that the presence of the halogen in the products (e.g., 3h, 3q) is very useful for further synthetic elaborations by cross-coupling reaction thereby broadening the diversity of the products.
Table 3 Denitrogenative Pd-catalyzed reaction of o-aminocinnamonitriles with arylhydrazinesa
Conditions: 1 (0.3 mmol), 2 (0.6 mmol), PdCl2 (10 mol%), L1 (20 mol%), toluene (2 mL), TfOH (2 equiv.), 90 °C, 24 h, O2, isolated yield. |
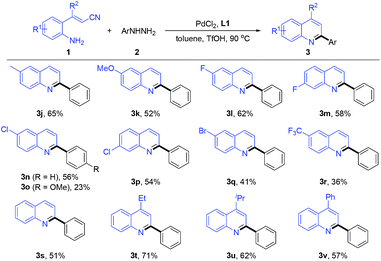 |
To gain insight into the mechanism of this cascade reaction, several control experiments were conducted (Scheme 2). The desired product 3a was not detected when the reaction was performed under N2 atmosphere (Scheme 2a), which revealed that the reaction required the presence of oxygen. As expected, the reactions of (E)-3-(2-aminophenyl)-1-phenylprop-2-en-1-one (4a) under optimized conditions in the absence of phenylhydrazine achieved the product 2-phenylquinoline (3r) in 72% yield, indicating that 4a was proposed as a possible intermediate for this transformation (Scheme 2b). The cyclization of 4a gave the desired product 3r in 65% yield in the absence of TfOH (Scheme 2c). The cyclization of 4a could also proceed to afford 3r in 63% yield in the absence of PdCl2/L1 (Scheme 2d). However, we attempted to perform the reaction in the absence of palladium catalyst and additive failed to give 3r (Scheme 2e).
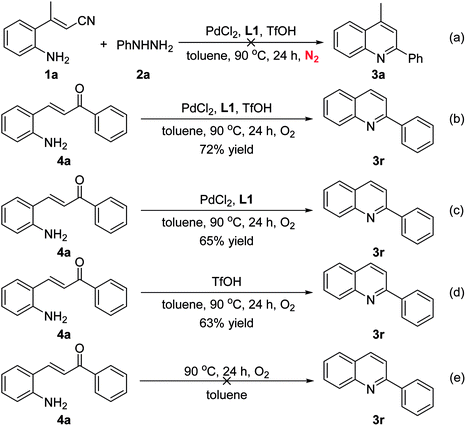 |
| Scheme 2 Control experiments. | |
On the basis of the above experimental results and previous reports,12a a possible reaction mechanism for the formation of quinolines is shown in Scheme 3. The first step may involve metathesis between the palladium catalyst and arylhydrazide to form the palladiaziridine intermediate A, which is followed by the coordination of cyano group affording intermediate B. Oxidative addition of the intermediate B to palladium(0) species to give the corresponding two palladium(II) centered intermediate C via C–N bond cleavage. Thereafter, cracking of the intermediate C gives the intermediate D and the palladiaziridine intermediate E, which would be decomposed into palladium(0), N2 and H2O in the presence of oxygen. Then, carbopalladation of the cyano group affords the imine-Pd intermediate F. Protonation of F by TfOH gives the imine intermediate G and regenerates palladium(II) species. Hydrolysis of G under acidic conditions would deliver the ketone intermediate H. Finally, the ketone intermediate H undergoes C–C bond E/Z configurational tautomerization to give I in the presence of TfOH and/or palladium(II) species, which is followed by intramolecular cyclization to generate the desired quinolines 3.
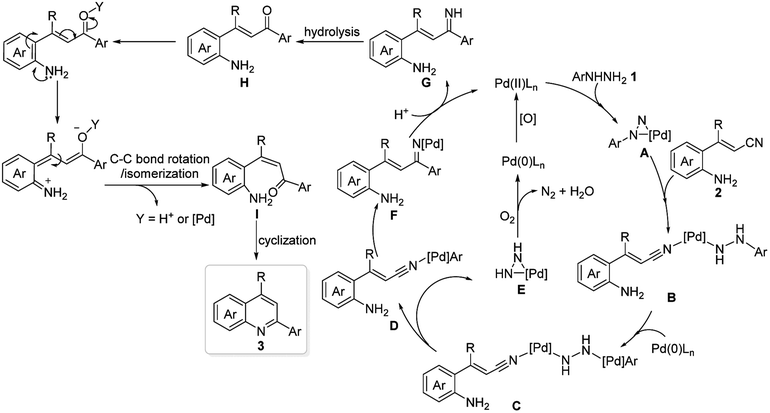 |
| Scheme 3 Plausible reaction mechanism for the formation of quinolines. | |
Conclusions
In summary, we have developed a new and complementary strategy for the synthesis of 2-arylquinolines in moderate to good yields by palladium-catalyzed cascade denitrogenative addition and intramolecular cyclization of o-aminocinnamonitriles with arylhydrazines.
Experimental section
General methods
Melting points are uncorrected. 1H NMR and 13C NMR spectra were measured on a 500 MHz spectrometer using CDCl3 as the solvent with tetramethylsilane (TMS) as an internal standard at room temperature. Chemical shifts are given n δ relative to TMS, and the coupling constants J are given in hertz. The starting materials o-aminocinnamonitriles15 and 4a16 were synthesized according to the method described in the literatures. Column chromatography was performed using EM silica gel 60 (300–400 mesh).
General procedure for the synthesis of quinolines
Under O2 atmosphere, a Teflon-valve-sealed Schlenk tube was charged with o-aminocinnamonitriles and arylhydrazines, PdCl2, L1, TfOH and toluene at room temperature. The reaction mixture was stirred for 10 minutes at room temperature for proper mixing of the reactants, and then heated at 90 °C (oil bath) with vigorous stirring for 24 hours. After the reaction equilibrium, the mixture was poured into ethyl acetate, which was washed with saturated NaHCO3 (2 × 10 mL) and then brine (10 mL). The aqueous layer was extracted with ethyl acetate, the combined organic layers were dried over anhydrous Na2SO4 and evaporated under vacuum. The residue was purified by flash column chromatography (hexane/ethyl acetate) to afford the desired products.
4-Methyl-2-phenylquinoline (3a)17. White solid (53.2 mg, 81%), mp 62–63 °C. 1H-NMR (500 MHz, CDCl3) δ 8.22 (d, J = 10.5 Hz, 1H), 8.19–8.17 (m, 2H), 7.97 (d, J = 10.5 Hz, 1H), 7.75–7.70 (m, 2H), 7.56–7.52 (m, 3H), 7.49–7.46 (m, 1H), 2.73 (s, 3H); 13C-NMR (125 MHz, CDCl3) δ 157.0, 148.2, 144.8, 139.8, 130.3, 129.3, 129.2, 128.8, 127.6, 127.3, 126.0, 123.6, 119.7, 19.0.
4-Methyl-2-(p-tolyl)quinoline (3b)18. Yellow oil (59.4 mg, 85%). 1H-NMR (500 MHz, CDCl3) δ 8.20 (d, J = 10.5 Hz, 1H), 8.09–8.07 (m, 2H), 7.98 (d, J = 10.0 Hz, 1H), 7.74–7.70 (m, 2H), 7.55–7.51 (m, 1H), 7.34 (d, J = 10.0 Hz, 2H), 2.74 (s, 3H), 2.45 (s, 3H); 13C-NMR (125 MHz, CDCl3) δ 157.0, 148.1, 144.7, 139.3, 136.9, 130.2, 129.5, 129.3, 127.4, 127.2, 125.9, 123.6, 119.6, 21.3, 19.0.
4-Methyl-2-(m-tolyl)quinoline (3c)18. Yellow oil (55.2 mg, 79%). 1H-NMR (500 MHz, CDCl3) δ 8.22 (d, J = 8.0 Hz, 1H), 8.02–7.98 (m, 2H), 7.94 (d, J = 8.0 Hz, 1H), 7.74–7.70 (m, 2H), 7.56–7.52 (m, 1H), 7.44–7.41 (m, 1H), 7.29 (d, J = 7.5 Hz, 1H), 2.75 (s, 3H), 2.50 (s, 3H); 13C-NMR (125 MHz, CDCl3) δ 157.3, 148.1, 144.7, 139.8, 138.4, 130.3, 130.0, 129.3, 128.7, 128.3, 127.3, 126.0, 124.7, 123.6, 119.9, 21.6, 19.0.
4-Methyl-2-(o-tolyl)quinoline (3d)19. Yellow oil (36.3 mg, 52%). 1H NMR (500 MHz, CDCl3) δ 8.20 (d, J = 8.5 Hz, 1H), 8.04–8.03 (m, 1H), 7.76–7.73 (m, 1H), 7.60–7.57 (m, 1H), 7.51–7.49 (m, 1H), 7.39 (s, 1H), 7.37–7.31 (m, 3H), 2.76 (s, 3H), 2.43 (s, 3H); 13C-NMR (125 MHz, CDCl3) δ 160.3, 147.9, 144.3, 140.5, 136.0, 130.3, 130.0, 129.6, 129.3, 128.3, 126.0, 123.6, 123.1, 20.3, 18.8.
2-(4-Fluorophenyl)-4-methylquinoline (3e)20. Yellow oil (54.1 mg, 76%). 1H-NMR (500 MHz, CDCl3) δ 8.18 (d, J = 10.5 Hz, 1H), 7.99 (d, J = 10.5 Hz, 1H), 7.92–7.90 (m, 2H), 7.75–7.71 (m, 1H), 7.67 (s, 1H), 7.58–7.54 (m, 1H), 7.50–7.45 (m, 1H), 7.17–7.13 (m, 1H), 2.76 (s, 3H); 13C-NMR (125 MHz, CDCl3) δ 163.8 (d, J = 247.5 Hz), 155.9, 148.1, 145.0, 135.9 (d, J = 2.5 Hz), 130.2, 129.4, 129.3 (d, J = 7.5 Hz), 127.2, 126.1, 123.6, 119.4, 115.7 (d, J = 22.5 Hz), 19.0.
2-(3-Fluorophenyl)-4-methylquinoline (3f)21. Yellow oil (44.8 mg, 63%). 1H-NMR (500 MHz, CDCl3) δ 8.18 (m, 3H), 7.86–7.85 (m, 1H), 7.61–7.50 (m, 2H), 7.43–7.42 (m, 1H), 7.09–7.07 (m, 2H), 2.62 (s, 3H); 13C-NMR (125 MHz, CDCl3) δ 163.4 (d, J = 243.8 Hz), 155.5 (d, J = 2.5 Hz), 148.0, 145.1, 142.1 (d, J = 7.5 Hz), 130.4, 130.2 (d, J = 8.8 Hz), 129.5, 127.4, 126.4, 123.6, 123.0 (d, J = 3.8 Hz), 119.4, 116.0 (d, J = 21.3 Hz), 114.4 (d, J = 22.5 Hz), 19.0.
2-(4-Chlorophenyl)-4-methylquinoline (3g)22. White solid (38.8 mg, 51%), mp 73–74 °C. 1H-NMR (500 MHz, CDCl3) δ 8.16 (d, J = 10.5 Hz, 1H), 8.11–8.09 (m, 2H), 7.98 (d, J = 10.5 Hz, 1H), 7.74–7.70 (m, 1H), 7.65 (s, 1H), 7.57–7.53 (m, 1H), 7.49–7.47 (m, 2H), 2.74 (s, 3H); 13C-NMR (125 MHz, CDCl3) δ 155.5, 147.9, 144.9, 138.0, 135.3, 130.1, 129.4, 128.8, 128.6, 126.2, 126.1, 123.5, 119.1, 18.8.
2-(4-Bromophenyl)-4-methylquinoline (3h)21. White solid (37.5 mg, 42%), mp 66–67 °C. 1H-NMR (500 MHz, CDCl3) δ 8.22–8.15 (m, 2H), 8.05–8.03 (m, 1H), 8.00–7.98 (m, 1H), 7.74–7.71 (m, 1H), 7.66–7.63 (m, 2H), 7.57–7.51 (m, 2H), 2.77–2.76 (m, 3H); 13C-NMR (125 MHz, CDCl3) δ 155.3, 148.0, 145.2, 141.8, 132.1, 130.6, 130.4, 130.3, 129.6, 127.4, 126.4, 126.1, 123.7, 123.1, 119.5, 19.0.
4-Methyl-2-(4-(trifluoromethyl)phenyl)quinoline (3i)23. Yellow oil (22.4 mg, 26%). 1H-NMR (500 MHz, CDCl3) δ 8.27 (d, J = 8.0 Hz, 2H), 8.20 (d, J = 8.5 Hz, 1H), 8.02 (d, J = 8.0 Hz, 1H), 7.78–7.24 (m, 4H), 7.60–7.57 (m, 1H), 2.79 (s, 3H); 13C-NMR (125 MHz, CDCl3) δ 155.5, 148.0, 145.5, 143.0, 131.0 (d, J = 32.5 Hz), 130.4, 129.7, 127.9, 127.5, 126.6, 125.7 (q, J = 3.8 Hz), 124.2 (d, J = 270.0 Hz), 123.7, 119.6, 19.0.
6-Methyl-2-phenylquinoline (3j)24. White solid (42.7 mg, 65%), mp 68–69 °C. 1H NMR (500 MHz, CDCl3) δ 8.18–8.16 (m, 2H), 8.10 (d, J = 11.0 Hz, 2H), 7.82 (d, J = 11.0 Hz, 1H), 7.57–7.52 (m, 4H), 7.48–7.45 (m, 1H), 2.55 (s, 3H); 13C-NMR (125 MHz, CDCl3) δ 156.5, 146.8, 139.8, 136.2, 136.1, 132.0, 129.4, 129.2, 128.8, 127.5, 127.2, 126.3, 119.0, 21.6.
6-Methoxy-2-phenylquinoline (3k)25. White solid (36.7 mg, 52%), mp 125–126 °C. 1H-NMR (500 MHz, CDCl3) δ 8.15–8.14 (m, 1H), 8.13 (s, 1H), 8.11 (d, J = 6.0 Hz, 1H), 8.08 (d, J = 6.0 Hz, 1H), 7.83 (d, J = 11.5 Hz, 1H), 7.52 (dd, J1 = 9.5 Hz, J2 = 9.0 Hz, 2H), 7.45 (d, J = 9.5 Hz, 1H), 7.39 (dd, J1 = 3.5 Hz, J2 = 11.5 Hz, 1H), 7.08 (d, J = 3.5 Hz, 1H), 3.94 (s, 3H); 13C-NMR (125 MHz, CDCl3) δ 157.8, 155.0, 144.4, 139.8, 135.5, 131.2, 128.9, 128.8, 128.2, 127.3, 122.3, 119.2, 105.1, 55.5.
6-Fluoro-2-phenylquinoline (3l)16. White solid (41.5 mg, 62%), mp 94–95 °C. 1H-NMR (500 MHz, CDCl3) δ 8.21–8.19 (m, 2H), 8.17–8.15 (m, 2H), 7.91 (d, J = 10.5 Hz, 1H), 7.56–7.44 (m, 5H); 13C-NMR (125 MHz, CDCl3) δ 160.4 (d, J = 248.0 Hz), 156.7 (d, J = 2.8 Hz), 145.3, 139.2, 136.2 (d, J = 5.2 Hz), 132.1 (d, J = 9.1 Hz), 129.5, 128.9, 127.7 (d, J = 10.0 Hz), 127.5, 119.9 (d, J = 25.7 Hz), 119.7, 110.5 (d, J = 21.7 Hz).
7-Fluoro-2-phenylquinoline (3m)25. White solid (38.8 mg, 58%), mp 88–89 °C. 1H-NMR (500 MHz, CDCl3) δ 8.22 (d, J = 8.0 Hz, 1H), 8.16 (d, J = 7.5 Hz, 2H), 7.86–7.80 (m, 3H), 7.56–7.53 (m, 2H), 7.50–7.47 (m, 1H), 7.34–7.30 (m, 1H); 13C-NMR (125 MHz, CDCl3) δ 163.3 (d, J = 249.6 Hz), 158.3, 149.3 (d, J = 12.9 Hz), 139.3, 136.6, 129.6, 129.4 (d, J = 10.0 Hz), 128.9, 127.6, 124.2, 118.3 (d, J = 2.5 Hz), 116.7 (d, J = 25.5 Hz), 113.3 (d, J = 20.2 Hz).
6-Chloro-2-phenylquinoline (3n)26. White solid (40.3 mg, 56%), mp 109–110 °C. 11H-NMR (500 MHz, CDCl3) δ 8.16–8.11 (m, 4H), 7.89 (d, J = 9.0 Hz, 1H), 7.84–7.80 (m, 1H), 7.66 (d, J = 9.0 Hz, 1H), 7.55–7.52 (m, 2H), 7.49–7.47 (m, 1H); 13C-NMR (125 MHz, CDCl3) δ 157.5, 146.6, 139.2, 135.8, 132.0, 131.3, 130.6, 129.6, 128.9, 127.7, 127.5, 126.1, 119.8.
6-Chloro-2-(p-methoxyphenyl)quinoline (3o)27. Pale yellow solid (18.6 mg, 23%), mp 156–158 °C. 1H NMR (500 MHz, CDCl3) δ 8.14 (d, J = 8.5 Hz, 2H), 8.08 (d, J = 8.5 Hz, 2H), 7.85 (d, J = 8.5 Hz, 1H), 7.77 (s, 1H), 7.64 (d, J = 9.0 Hz, 1H), 7.05 (d, J = 8.5 Hz, 2H), 3.89 (s, 3H); 13C NMR (125 MHz, CDCl3) δ 161.1, 157.1, 146.6, 135.7, 131.5, 131.0, 130.5, 128.9, 127.5, 126.1, 119.3, 114.3, 55.4.
7-Chloro-2-phenylquinoline (3p)28. White solid (38.8 mg, 54%), mp 106–107 °C. 1H-NMR (500 MHz, CDCl3) δ 8.21–8.15 (m, 4H), 7.89–7.87 (m, 1H), 7.77–7.75 (m, 1H), 7.56–7.48 (m, 4H); 13C-NMR (125 MHz, CDCl3) δ 158.2, 148.5, 139.0, 136.7, 135.6, 129.7, 128.9, 128.7, 128.6, 127.6, 127.4, 125.5, 119.2.
6-Bromo-2-phenylquinoline (3q)27. White solid (34.9 mg, 41%), mp 113–114 °C. 1H-NMR (500 MHz, CDCl3) δ 8.15 (d, J = 8.0 Hz, 2H), 8.10–8.09 (m, 1H), 8.04 (d, J = 8.0 Hz, 1H), 7.96 (s, 1H), 7.88–7.86 (m, 1H), 7.79–7.77 (m, 1H), 7.55–7.52 (m, 2H), 7.50–7.47 (m, 1H); 13C-NMR (125 MHz, CDCl3) δ 157.6, 146.8, 139.1, 135.8, 133.1, 131.4, 129.6, 129.5, 128.9, 128.2, 127.5, 120.0, 119.7.
2-Phenyl-6-(trifluoromethyl)quinoline (3r)29. White solid (29.5 mg, 36%), mp 118–119 °C. 1H-NMR (500 MHz, CDCl3) δ 8.26 (d, J = 10.0 Hz, 2H), 8.20–8.17 (m, 2H), 8.12 (s, 1H), 7.94 (d, J = 10.0 Hz, 1H), 7.90–7.88 (m, 1H), 7.58–7.51 (m, 3H); 13C-NMR (125 MHz, CDCl3) δ 159.3, 149.3, 138.9, 137.4, 130.9, 130.0, 129.0, 128.2, 127.9, 127.7, 126.1, 125.4 (q, J = 3.8 Hz), 125.3 (q, J = 3.8 Hz), 123.1, 120.0.
2-Phenylquinoline (3s)30. White solid (31.4 mg, 51%), mp 84–85 °C. 1H-NMR (500 MHz, CDCl3) δ 8.24–8.17 (m, 4H), 7.89 (d, J = 10.0 Hz, 1H), 7.84 (d, J = 10.0 Hz, 1H), 7.76–7.72 (m, 1H), 7.56–7.52 (m, 3H), 7.49–7.46 (m, 1H); 13C-NMR (125 MHz, CDCl3) δ 157.4, 148.3, 139.6, 136.8, 129.7, 129.6, 129.4, 128.8, 127.6, 127.4, 127.2, 126.3, 119.0.
4-Ethyl-2-phenylquinoline (3t)20. Yellow oil (49.6 mg, 71%). 1H-NMR (500 MHz, CDCl3) δ 8.22 (d, J = 10.5 Hz, 1H), 8.18–8.17 (m, 2H), 8.04 (d, J = 10.5 Hz, 1H), 7.74–7.70 (m, 2H), 7.56–7.52 (m, 3H), 7.49–7.46 (m, 1H), 3.17 (q, J = 9.5 Hz, 2H), 1.45 (t, J = 9.5 Hz, 3H); 13C-NMR (125 MHz, CDCl3) δ 157.3, 150.4, 148.5, 140.1, 130.6, 129.2, 128.8, 127.6, 126.4, 126.0, 123.2, 117.8, 25.4, 14.2.
4-Isopropyl-2-phenylquinoline (3u)31. Yellow oil (45.9 mg, 62%). 1H-NMR (500 MHz, CDCl3) δ 8.21 (d, J = 10.5 Hz, 1H), 8.17–8.15 (m, 2H), 8.11 (d, J = 10.5 Hz, 1H), 7.79 (s, 1H), 7.73–7.70 (m, 1H), 7.57–7.52 (m, 3H), 7.49–7.45 (m, 1H), 3.84–3.77 (m, 1H), 1.48 (d, J = 9.0 Hz, 6H); 13C-NMR (125 MHz, CDCl3) δ 157.4, 154.9, 148.6, 140.3, 130.7, 129.1, 129.0, 128.8, 127.6, 125.9, 125.8, 122.9, 114.9, 28.6, 23.0.
2,4-Diphenylquinoline (3v)32. White solid (48.1 mg, 57%), mp 104–105 °C. 1H-NMR (500 MHz, CDCl3) δ 8.28 (d, J = 10.5 Hz, 1H), 8.21–8.19 (m, 2H), 7.92 (d, J = 10.5 Hz, 1H), 7.83 (s, 1H), 7.77–7.73 (m, 1H), 7.57–7.55 (m, 4H), 7.54–7.48 (m, 5H); 13C-NMR (125 MHz, CDCl3) δ 156.9, 149.4, 148.7, 139.6, 138.4, 130.0, 129.6, 129.4, 128.8, 128.6, 128.4, 127.6, 126.4, 125.8, 125.7, 119.4.
Conflicts of interest
There are no conflicts of interest to declare.
Acknowledgements
We thank the National Natural Science Foundation of China (No. 21572162) and the Natural Science Foundation of Zhejiang Province (No. LY20B020015) for financial support.
References
- H. Študentová, D. Vitáskováand and B. Melichar, Lenvatinib for the treatment of kidney cancer, Expert Rev. Anticancer Ther., 2018, 18, 511–518 CrossRef PubMed
. - A. Abdelaziz and U. Vaishampayan, Cabozantinib for the treatment of kidney cancer, Expert Rev. Anticancer Ther., 2017, 17, 577–584 CrossRef CAS PubMed
. - Y. Liu, W. Li, S. L. Morris-Natschke, K. Qian, L. Yang, G. Zhu, X. Wu, A. Chen, S. Zhang, X. Nan and K. Lee, Perspectives on biologically active camptothecin derivatives, Med. Res. Rev., 2015, 35, 753–789 CrossRef CAS PubMed
. - For selected reviews, see:
(a) J. Marco-Contelles, E. Perez-Mayoral, A. Samadi, M. C. Carreiras and E. Soriano, Recent advances in the Friedländer reaction, Chem. Rev., 2009, 109, 2652–2671 CrossRef CAS PubMed
;
(b) S. M. Prajapati, K. D. Patel, R. H. Vekariya, S. N. Panchal and H. D. Patel, Recent advances in the synthesis of quinolines: a review, RSC Adv., 2014, 4, 24463–24476 RSC
;
(c) V. Kouznetsov, L. Mendez and C. Gomez, Recent progress in the synthesis of quinolines, Curr. Org. Chem., 2005, 9, 141–161 CrossRef CAS
;
(d) L. M. Nainwal, S. Tasneem, W. Akhtar, G. Verma, M. F. Khan, S. Parvez, M. Shaquiquzzaman, M. Akhter and M. M. Alam, Green recipes to quinoline: a review, Eur. J. Med. Chem., 2019, 164, 121–170 CrossRef CAS PubMed
. -
(a) F. F. Fleming and Q. Wang, Unsaturated nitriles:
conjugate additions of carbon nucleophiles to a recalcitrant class of acceptors, Chem. Rev., 2003, 103, 2035–2078 CrossRef CAS PubMed
;
(b) V. Y. Kukushkin and A. J. L. Pombeiro, Additions to metal-activated organonitriles, Chem. Rev., 2002, 102, 1771–1802 CrossRef CAS PubMed
;
(c) D. Enders and J. P. Shilvock, Some recent applications of α-amino nitrile chemistry, Chem. Soc. Rev., 2000, 29, 359–373 RSC
. - S. F. Rach and F. E. Kühn, Nitrile ligated transition metal complexes with weakly coordinating counteranions and their catalytic applications, Chem. Rev., 2009, 109, 2061–2080 CrossRef CAS PubMed
. -
(a) R. C. Larock, Q. P. Tian and A. A. Pletnev, Carbocycle synthesis via carbopalladation of nitriles, J. Am. Chem. Soc., 1999, 121, 3238–3239 CrossRef CAS
;
(b) C. Zhou and R. C. Larock, Synthesis of aryl ketones by the Pd-catalyzed C–H activation of arenes and intermolecular carbopalladation of nitriles, J. Am. Chem. Soc., 2004, 126, 2302–2303 CrossRef CAS PubMed
;
(c) J. Lindh, P. J. R. Sjöberg and M. Larhed, Synthesis of aryl ketones by palladium(II)-catalyzed decarboxylative addition of benzoic acids to nitriles, Angew. Chem., Int. Ed., 2010, 49, 7733–7737 CrossRef CAS PubMed
;
(d) X. Wang, M. Liu, L. Xu, Q. Wang, J. Chen, J. Ding and H. Wu, Palladium-catalyzed addition of potassium aryltrifluoroborates to aliphatic nitriles: synthesis of alkyl aryl ketones, diketone compounds, and 2-arylbenzo[b]furans, J. Org. Chem., 2013, 78, 5273–5281 CrossRef CAS PubMed
;
(e) J. T. Reeves, C. A. Malapit, F. G. Buono, K. P. Sidhu, M. A. Marsini, C. A. Sader, K. R. Fandrick, C. A. Busacca and C. H. Senanayake, Transnitrilation from dimethylmalononitrile to aryl Grignard and lithium reagents: a practical method for aryl nitrile synthesis, J. Am. Chem. Soc., 2015, 137, 9481–9488 CrossRef CAS PubMed
;
(f) C. A. Malapit, J. T. Reeves, C. A. Busacca, A. R. Howell and C. H. Senanayake, Rhodium-catalyzed transnitrilation of aryl boronic acids with dimethylmalononitrile, Angew. Chem., Int. Ed., 2016, 55, 326–330 CrossRef CAS PubMed
;
(g) C. A. Malapit, D. R. Caldwell, I. K. Luvaga, J. T. Reeves, I. Volchkov, N. C. Gonnella, Z. S. Han, C. A. Busacca, A. R. Howell and C. H. Senanayake, Rhodium-catalyzed addition of aryl boronic acids to 2,2-disubstituted malononitriles, Angew. Chem., Int. Ed., 2017, 56, 6999–7002 CrossRef CAS PubMed
;
(h) M. Meng, L. Yang, K. Cheng and C. Qi, Pd(II)-catalyzed denitrogenative and desulfinative addition of arylsulfonyl hydrazides with nitriles, J. Org. Chem., 2018, 83, 3275–3284 CrossRef CAS PubMed
;
(i) L. R. Mills, J. M. Graham, P. Patel and S. A. L. Rousseaux, Ni-catalyzed reductive cyanation of aryl halides and phenol derivatives via transnitrilation, J. Am. Chem. Soc., 2019, 141, 19257–19262 CrossRef CAS PubMed
. -
(a) L. Qi, R. Li, X. Yao, Q. Zhen, P. Ye, Y. Shao and J. Chen, Syntheses of pyrroles, pyridines, and ketonitriles via catalytic carbopalladation of dinitriles, J. Org. Chem., 2020, 85, 1097–1108 CrossRef CAS PubMed
;
(b) Q. Zhen, R. Li, L. Qi, K. Hu, X. Yao, Y. Shao and J. Chen, Nickel(II)-catalyzed C–C, N–C cascade coupling of ketonitriles into substituted pyrroles and pyridines, Org. Chem. Front., 2020, 7, 286–291 RSC
;
(c) X. Yao, Y. Shao, M. Hu, Y. Xia, T. Cheng and J. Chen, Palladium-catalyzed cascade reaction of o-cyanobiaryls with arylboronic acids: synthesis of 5-arylidene-7-aryl-5H-dibenzo[c,e]azepines, Org. Lett., 2019, 21, 7697–7701 CrossRef CAS PubMed
;
(d) K. Hu, Q. Zhen, J. Gong, T. Cheng, L. Qi, Y. Shao and J. Chen, Palladium-catalyzed three-component tandem process: one-pot assembly of quinazolines, Org. Lett., 2018, 20, 3083–3087 CrossRef CAS PubMed
;
(e) Y. Zhang, Y. Shao, J. Gong, K. Hu, T. Cheng and J. Chen, Palladium-catalyzed tandem reaction of quinazolinone-based nitriles with arylboronic acids: synthesis of 2-(4-arylquinazolin-2-yl)anilines, Adv. Synth. Catal., 2018, 360, 3260–3265 CrossRef CAS
;
(f) K. Hu, L. Qi, S. Yu, T. Cheng, X. Wang, Z. Li, Y. Xia, J. Chen and H. Wu, Efficient synthesis of isoquinolines in water by a Pd-catalyzed tandem reaction of functionalized alkylnitriles with arylboronic acids, Green Chem., 2017, 19, 1740–1750 RSC
;
(g) L. Qi, K. Hu, S. Yu, J. Zhu, T. Cheng, X. Wang, J. Chen and H. Wu, Tandem addition/cyclization for access to isoquinolines and isoquinolones via catalytic carbopalladation of nitriles, Org. Lett., 2017, 19, 218–221 CrossRef CAS PubMed
;
(h) S. Yu, L. Qi, K. Hu, J. Gong, T. Cheng, Q. Wang, J. Chen and H. Wu, The development of a palladium-catalyzed tandem addition/cyclization for the construction of indole skeletons, J. Org. Chem., 2017, 82, 3631–3638 CrossRef CAS PubMed
;
(i) X. Yao, Y. Shao, M. Hu, M. Zhang, S. Li, Y. Xia, T. Cheng and J. Chen, Palladium-catalyzed selective synthesis of dibenzo[c,e]azepin-5-ols and benzo[c]pyrido[2,3-e]azepin-5-ols, Adv. Synth. Catal., 2019, 361, 4707–4713 CrossRef CAS
;
(j) W. Xiong, K. Hu, Y. Lei, Q. Zhen, Z. Zhao, Y. Shao, R. Li, Y. Zhang and J. Chen, Palladium-catalyzed cascade reactions of 2-(cyanomethoxy)chalcones with arylboronic acids: selective synthesis of emissive benzofuro[2,3-c]pyridines, Org. Lett., 2020, 22(4), 1233–1238 CrossRef PubMed
. -
(a) H. Li, S. Zhang, X. Feng, X. Yu, Y. Yamamoto and M. Bao, Rhodium(III)-catalyzed oxidative [3+2] annulation of 2-acetyl-1-arylhydrazines with maleimides: synthesis of pyrrolo[3,4-b]indole-1,3-diones, Org. Lett., 2019, 21, 8563–8567 CrossRef CAS PubMed
;
(b) N. Lv, Z. Chen, Z. Liu and Y. Zhang, Redox-neutral rhodium(III)-catalyzed annulation of arylhydrazines with sulfoxonium ylides to synthesize 2-arylindoles, J. Org. Chem., 2019, 84, 13013–13021 CrossRef CAS PubMed
;
(c) A. S. Pirzer, E.-M. Alvarez, H. Friedrich and M. R. Heinrich, Radical carbofluorination of alkenes with arylhydrazines and selectfluor: additives, mechanistic pathways, and polar effects, Chem.–Eur. J., 2019, 25, 2786–2792 CrossRef CAS PubMed
;
(d) R. Li, X. Chen, S. Wei, K. Sun, L. Fan, Y. Liu, L. Qu, Y. Zhao and B. Yu, A Visible-light-promoted metal-free strategy towards arylphosphonates: organic-dye-catalyzed phosphorylation of arylhydrazines with trialkylphosphites, Adv. Synth. Catal., 2018, 360, 4807–4813 CrossRef
;
(e) Y. Tu, Z. Zhang, T. Wang, J. Ke and J. Zhao, A regioselective approach to trisubstituted pyrazoles via palladium-catalyzed oxidative sonogashira-carbonylation of arylhydrazines, Org. Lett., 2017, 19, 3466–3469 CrossRef CAS PubMed
. -
(a) C. Wang, Z. Zhang, Y. Tu, Y. Li, J. Wu and J. Zhao, Palladium-catalyzed oxidative cross-coupling of arylhydrazines and arenethiols with molecular oxygen as the sole oxidant, J. Org. Chem., 2018, 83, 2389–2394 CrossRef CAS PubMed
;
(b) S. Chang, L. Dong, H. Song and B. Feng, Denitrogenative palladium-catalyzed coupling of aryl halides with arylhydrazines under mild conditions, Org. Biomol. Chem., 2018, 16, 3282–3288 RSC
;
(c) T. Taniguchi, T. Naka, M. Imoto, M. Takeda, T. Nakai, M. Mihara, T. Mizuno, A. Nomoto and A. Ogawa, Transition-metal-free and oxidant-free cross-coupling of arylhydrazines with disulfides: base-promoted synthesis of unsymmetrical aryl sulphides, J. Org. Chem., 2017, 82, 6647–6655 CrossRef CAS PubMed
;
(d) Y. Zhao and Q. Song, Palladium-catalyzed aerobic oxidative cross-coupling of arylhydrazines with terminal alkynes, Chem. Commun., 2015, 51, 13272–13274 RSC
;
(e) M. Ravi, P. Chauhan, R. Kant, S. K. Shukla and P. P. Yadav, Transition-metal-free C-3 arylation of quinoline-4-ones with arylhydrazines, J. Org. Chem., 2015, 80, 5369–5376 CrossRef CAS PubMed
;
(f) Z. Peng, G. Hu, H. Qiao, P. Xu, Y. Gao and Y. Zhao, Palladium-catalyzed suzuki cross-coupling of arylhydrazines via C–N bond cleavage, J. Org. Chem., 2014, 79(6), 2733–2738 CrossRef CAS PubMed
;
(g) J. Hofmann, H. Jasch and M. R. Heinrich, Oxidative radical arylation of anilines with arylhydrazines and dioxygen from air, J. Org. Chem., 2014, 79, 2314–2320 CrossRef CAS PubMed
. - K. Ouyang, W. Hao, W. X. Zhang and Z. Xi, Transition-metal-catalyzed cleavage of C–N single bonds, Chem. Rev., 2015, 115, 12045–12090 CrossRef CAS PubMed
. -
(a) X. Wang, Y. Huang, Y. Xu, X. Tang, W. Wu and H. Jiang, Palladium-catalyzed denitrogenative synthesis of aryl ketones from arylhydrazines and nitriles using O2 as sole oxidant, J. Org. Chem., 2017, 82, 2211–2218 CrossRef CAS PubMed
;
(b) K. Cheng, G. Wang, M. Meng and C. Qi, Acid-promoted denitrogenative Pd-catalyzed addition of arylhydrazines with nitriles at room temperature, Org. Chem. Front., 2017, 4, 398–403 RSC
. -
(a) S. Y. Lee, J. Jeon and C.-H. Cheon, Synthesis of 2-substituted quinolines from 2-aminostyryl ketones using iodide as a catalyst, J. Org. Chem., 2018, 83, 5177–5186 CrossRef CAS PubMed
;
(b) S. Lee and C.-H. Cheon, On-water synthesis of 2-substituted quinolines from 2-aminochalcones using benzylamine as the nucleophilic catalyst, J. Org. Chem., 2018, 83, 13036–13044 CrossRef CAS PubMed
. - T. Xu, Y. Shao, L. Dai, S. Yu, T. Cheng and J. Chen, Pd-catalyzed tandem reaction of 2-aminostyryl nitriles with arylboronic acids: synthesis of 2-arylquinolines, J. Org. Chem., 2019, 84, 13604–13614 CrossRef CAS PubMed
. -
(a) J. G. Harrison, O. Gutierrez, N. Jana, T. G. Driver and D. J. Tantillo, Mechanism of Rh2(II)-catalyzed indole formation the catalyst does not control product selectivity, J. Am. Chem. Soc., 2016, 138, 487–490 CrossRef CAS PubMed
;
(b) L. Garanti and G. Zecchi, Thermochemical behavior of o-azidocinnamonitriles, J. Org. Chem., 1980, 45, 4767–4769 CrossRef CAS
;
(c) J. R. Baker, J. Gilbert, S. Paula, X. Zhu, J. A. Sakoff and A. McCluskey, Dichlorophenylacrylonitriles as AhR ligands that display selective breast cancer cytotoxicity in vitro, ChemMedChem, 2018, 13, 1447–1458 CrossRef CAS PubMed
. - S. Y. Lee and C.-H. Cheon, On-water synthesis of 2-substituted quinolines from 2-aminochalcones using benzylamine as the nucleophilic catalyst, J. Org. Chem., 2018, 83, 13036–13044 CrossRef CAS PubMed
. - B. Gabriele, R. Mancuso, G. Salerno, G. Ruffolo and P. Plastina, Novel and convenient synthesis of substituted quinolines by copper- or palladium-catalyzed cyclodehydration of 1-(2-aminoaryl)-2-yn-1-ols, J. Org. Chem., 2007, 72, 6873–6877 CrossRef CAS PubMed
. - P. Kumar, V. Garg, M. Kumara and A. , K. Verma, Rh(III)-catalyzed alkynylation: synthesis of functionalized quinolines from aminohydrazones, Chem. Commun., 2019, 55, 12168–12171 RSC
. - N. Liu and Z. Wang, Kumada coupling of aryl, heteroaryl, and vinyl chlorides catalyzed by amido pincer nickel complexes, J. Org. Chem., 2011, 76, 10031–10038 CrossRef CAS PubMed
. - W. Liu, X. Yang, Z. Zhou and C. Li, Simple and clean photo-induced methylation of heteroarenes with MeOH, Chem, 2017, 2, 688–702 CAS
. - J. Yuan, L. Yang, P. Mao and L. Qu, AgNO3-catalyzed direct C–H arylation of quinolines by oxidative decarboxylation of aromatic carboxylic acids, Org. Chem. Front., 2017, 4, 545–554 RSC
. - S. S. Palimkar, S. A. Siddiqui, T. Daniel, R. J. Lahoti and K. V. Srinivasan, Ionic liquid-promoted regiospecific Friedlander annulation: novel synthesis of quinolines and fused polycyclic quinolones, J. Org. Chem., 2003, 68, 9371–9378 CrossRef CAS PubMed
. - M. S. Hofmayer, F. H. Lutter, L. Grokenberger, J. M. Hammann and P. Knochel, Practical Ni-catalyzed cross-coupling of unsaturated zinc pivalates with unsaturated nonaflates and triflates, Org. Lett., 2019, 21, 36–39 CrossRef CAS PubMed
. - G. Chakraborty, R. Sikari, S. Das, R. Mondal, S. Sinha, S. Banerjee and N. D. Paul, Dehydrogenative synthesis of quinolines, 2-aminoquinolines, and quinazolines using singlet diradical Ni(II)-catalysts, J. Org. Chem., 2019, 84, 2626–2641 CrossRef CAS PubMed
. - S. Das, D. Maiti and S. D. Sarkar, Synthesis of polysubstituted quinolines from α-2-aminoaryl alcohols via nickel-catalyzed dehydrogenative coupling, J. Org. Chem., 2018, 83, 2309–2316 CrossRef CAS PubMed
. - W. Hu, Y. Zhang, H. Zhu, D. Ye and D. Wang, Unsymmetrical triazolyl-naphthyridinyl-pyridine bridged highly active copper complexes supported on reduced graphene oxide and their application in water, Green Chem., 2019, 21, 5345–5351 RSC
. - M. Maji, K. Chakrabarti, D. Panja and S. Kundu, Sustainable synthesis of N-heterocycles in water using alcohols following the double dehydrogenation strategy, J. Catal., 2019, 373, 93–102 CrossRef CAS
. - S. Das, S. Sinha, D. Samanta, R. Mondal, G. Chakraborty, P. Brandaõ and N. D. Paul, Metal–ligand cooperative approach to achieve dehydrogenative functionalization of alcohols to quinolines and quinazolin-4(3H)-ones under mild aerobic conditions, J. Org. Chem., 2019, 84, 10160–10171 CrossRef CAS PubMed
. - E. J. Cho, T. D. Senecal, T. Kinzel, Y. Zhang, D. A. Watson and S. L. Buchwald, The palladium-catalyzed trifluoromethylation of aryl chlorides, Science, 2010, 328, 1679–1681 CrossRef CAS PubMed
. - M. Tobisu, I. Hyodo and N. Chatani, Nickel-catalyzed reaction of arylzinc reagents with N-aromatic heterocycles: a straightforward approach to C–H bond arylation of electron-deficient heteroaromatic compounds, J. Am. Chem. Soc., 2009, 131, 12070–12071 CrossRef CAS PubMed
. - H. Yan, Z. Hou and H. Xu, Photoelectrochemical C–H alkylation of heteroarenes with organotrifluoroborates, Angew. Chem., Int. Ed., 2019, 58, 4592–4595 CrossRef CAS PubMed
. - J. Horn, S. P. Marsden, A. Nelson, D. House and G. G. Weingarten, Convergent, regiospecific synthesis of quinolines from o-aminophenylboronates, Org. Lett., 2008, 10, 4117–4120 CrossRef CAS PubMed
.
Footnote |
† Electronic supplementary information (ESI) available: 1H and 13C NMR spectra for products. See DOI: 10.1039/d0ra01043j |
|
This journal is © The Royal Society of Chemistry 2020 |