DOI:
10.1039/D0RA00778A
(Paper)
RSC Adv., 2020,
10, 13021-13028
Direct electrochemical detection of clozapine by RuO2 nanoparticles-modified screen-printed electrode
Received
25th January 2020
, Accepted 16th March 2020
First published on 31st March 2020
Abstract
This study introduces the sensitive electrochemical detection of clozapine with the use of a ruthenium(IV) oxide nanoparticle (RuO2 NP)-modified screen-printed electrode (RuO2 NPs/SPE). The electrochemical behaviors of clozapine at RuO2 NP/SPE have been examined via cyclic voltammetry (CV), differential pulse voltammetry (DPV) and chronoamperometry (CHA). According to the results, the modified electrode has been accompanied by a decreasing over-potential (ca. 170 mV) and enhancement in the peak current (3 times) in comparison with the bare SPE. The results indicated that RuO2 NP/SPE markedly augmented electro-catalytic activities toward clozapine oxidation. In addition, linear responses have been observed in the range between 0.2 and 500.0 μM with a sensitivity of 0.076 μA μM−1 and a limitation of detection of 0.07 μM (3σ). Moreover, the successful application of RuO2 NP/SPE has been seen in detecting clozapine in real samples, which showed satisfied recoveries. Therefore, outputs suggest that RuO2 NP/SPE will be promising for functional utilization.
Introduction
According to the studies, schizophrenia is one of the serious chronic weakening diseases described by positive signs, such as hallucination and delusions, negative signs such as a socially withdrawn behavior and flat emotional expression, and cognitive deficiencies.1 However, upon the introduction of clozapine (8-chloro-11-(4′-methyl-1 piperazinyl)-5H-dibenzo[b,e]-[1,4]-diazepine) that is the parent medicine of the so-called “typical” anti-psychotics, schizophrenia therapy experienced a good advancement. Clozapine is used to treat the negative and positive signs of patients with schizophrenia who do not respond well to the common neuroleptic medicines. Intoxication was evident at a high serum level of clozapine of 1158 ng mL−1 during a trial with a dose of 800 mg d−1.2
With regard to its significance, researchers utilized multiple techniques to detect clozapine in the drug and clinical preparations. The techniques consist of spectrophotometry,3 capillary zone electrophoresis,4 liquid chromatography-mass spectrometry,5 gas chromatography-mass spectrometry,6 high-performance liquid chromatography (HPLC),7 liquid chromatography-tandem mass spectrometry8 and electrochemistry.9–11
Researchers further explored the electrochemical procedures because of benefits such as quicker responses, inexpensive instruments, easy preparation, cost-effectiveness, higher sensitivity, and good selectivity and stability.12–18
In addition, the screen-printed electrodes (SPEs) enjoy highly available and disposable electrochemical sensors, simplified operations and have non-poisonous features. Moreover, replacing traditional electrochemical cells with the SPEs linked to miniaturized potentiostats is one of the major trends in shifting the laboratory electrochemical instrumentations to the hand-held field analyzers. Furthermore, these electrodes are appropriate for operations with micro volumes and decentralized assays (point of care tests), and so forth.19–23
To minimize the oxidation over-potential of numerous electroactive compounds, researchers designed the electro-catalytic procedures for exploitation at the modified electrode surface. Notably, the chemically modified electrodes augment the transfer rate of electrons by declining the over-potential.24–33
Nanoparticle (NP)-based CMEs have been the spotlight because of their increased sensitivity, amplified response signals, and more acceptable reproducibility.34–44
In addition, ruthenium oxide (RuO2) NPs have high potential as one of the electrochemical elements of sensors because of the respective attractive benefits; for example, acceptable electronic conductivity, very good catalytic capability, and higher chemical stability.45,46
Accordingly, this study aimed at developing a RuO2 NR/SPE, lowering the clozapine oxidation potential for its detection with no considerable effects from the background current. Thus, the study prepared an electrochemical sensor achieved by the modification of SPE by RuO2 NPs and its application in detecting clozapine in the real specimens.
Experimental section
Reagents and instrument
We conducted electrochemical tests using an Autolab potentiostat/galvanostat (PGSTAT 302N, Eco Chemie: the Netherlands); then, we monitored the system by a general-purpose electrochemical system software.
It should be noted that SPE (DropSens: DRP-110, Spain) has 3 traditional electrodes called the graphite counter electrode, unmodified graphite working electrode, and a silver pseudo-reference electrode. Moreover, we used a Metrohm 710 pH-meter for measuring pH. Milli-Q® IQ7003/05/10/15 water purification systems were used for preparing deionized water.
Clozapine and all the available reagents were of analytical grade. We chose Merck (Darmstadt: Germany) for purchasing the reagents. Thus, in order to procure the buffers, we applied ortho-phosphoric acid (85%) and respective salts for setting pH ranges between 2.0 and 9.0.
RuO2 NP synthesis
According to the research design, 80 mL of water and 0.8 g of starch were blended and placed in a 60 °C water bath in order to gain a transparent solution. In addition, 1.2 × 10−2 mol of ruthenium chloride salt (99.9%) was added to the above solution. Then, pH was adjusted to 10.0 with a 1.0 M of ammonia solution. Consequently, the solution color changed to be black. Next, the solution was shaken for two hours and filtered. Finally, deionized water was used to wash it; therefore, when a thorough withdrawal of chlorine was ensured, the precipitate was dried and calcined at 450 °C for two hours.
Preparing the electrode
Based on the facile process presented below, the bare SPE was coated. Then, 1 mg of RuO2 NPs were distributed in a 1 mL of aqueous solution over a 45 min ultra-sonication interval. Afterward, 5 μL of the procured suspension was dropped onto the carbon working electrode surface. Finally, it was left at room temperature in order to be dried.
Preparing the real samples
Clozapine tablets (Tehran Chemie Pharmaceutical Co., Iran [labelled value clozapine = 100 mg per tablet]) were purchased. The clozapine pills were thoroughly powdered and homogenized prior to the preparation of 10 mL of a 0.1 M stock solution. In fact, we sonicated the solution for assuring an ideal dissolution. Then, we filtered the solution. Furthermore, we poured the determined volume of the transparent filtrate into an electrochemical cell consisting of 10 mL of 0.1 M PBS (pH 7.0) for recording the differential pulse voltammetry (DPV).
In addition, we kept the urine specimens inside a refrigerator after being collected. Then, 10 mL of the specimens were centrifuged at 2000 rpm for 15 min. In the next stage, we filtered the supernatant through a 0.45 μm filter. Afterward, different contents of the solution were transferred into a 10 mL volumetric flask, and the solution was diluted to the mark with PBS (pH 7.0). No peak current was observed for clozapine in the urine samples. Then, diverse amounts of clozapine were used to spike the diluted urine specimens. Finally, clozapine contents were examined by our technique via the standard addition procedure.
In all two samples, an aliquot of 10 mL of the test solution was placed in the electrochemical cell. The potentials for differential pulse voltammetry (DPV) were controlled between 0.1 and 0.5 V at the scan rate of 50 mV s−1. Anodic peak current (Ipa) was measured at the oxidation potential of clozapine.
Results and discussion
RuO2NP characterization
Fig. 1 displays the scanning electron microscopy (SEM) images of RuO2NPs. The well-arranged NRs further facilitate the contact of samples with the solution.
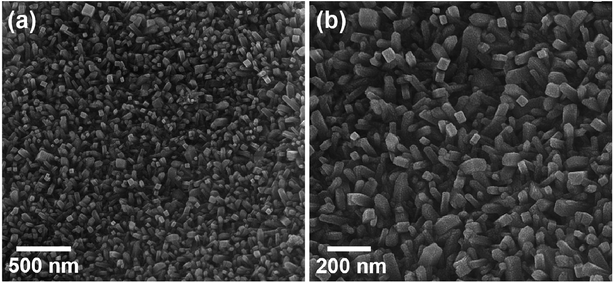 |
| Fig. 1 SEM images of the as-synthesized RuO2NPs. | |
The energy dispersive X-ray analysis (EDX) of RuO2 NPs was performed to qualitatively examine the presence of elements and chemical composition of the as-synthesized RuO2 NPs. As seen in Fig. 2, the EDX spectrum reveals the presence of elemental Ru and O, which approves the higher purity of RuO2 NPs without obvious impurities.
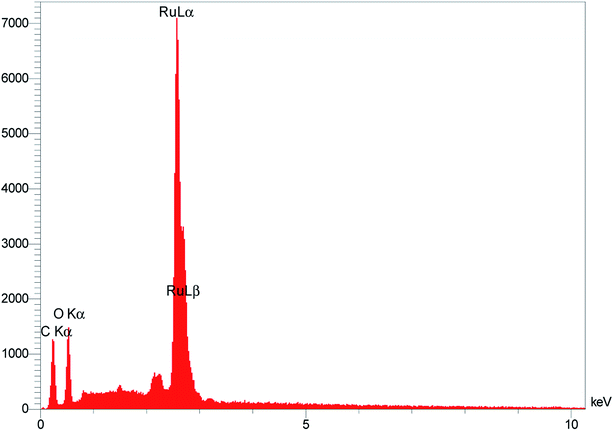 |
| Fig. 2 EDX spectrum of RuO2 NPs. | |
The EDX elemental mapping analysis was performed for RuO2 NPs to check the distribution of elements present in the as-synthesized RuO2 NPs (Fig. 3). Thus, there are O and Ru elements in the as-synthesized RuO2 NPs.
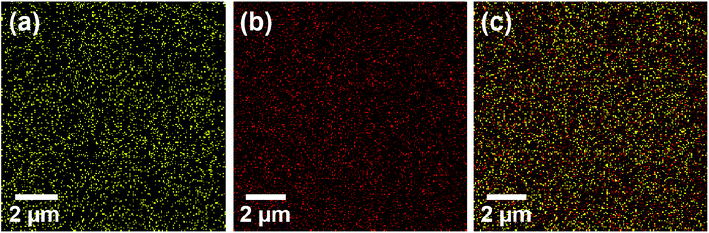 |
| Fig. 3 EDX elemental mapping of the (a) O, (b) Ru, and (c) RuO2. | |
Fig. 4 shows the X-ray diffraction (XRD) patterns of the RuO2NPs synthesized in the present study. In addition, the presence of the miller indices of (110), (101), (200), (211), (220), (002), (310), (112), (301) and (202) in the 2θ range of 28.2, 35.1, 39.7, 54.4, 58.1, 59.4, 65.3, 66.8, 69.1 and 73.8 degree, respectively, confirmed the tetragonal structure for RuO2 NPs (JCPDS 21-1172). The average size of RuO2NPs is calculated using the Scherrer's equation:47
|
d = kλ/β cos θ
| (1) |
where
d is the size of NPs;
k is the Scherrer's constant;
λ is the X-ray wavelength;
β is the width of the XRD peak at half height; and
θ is the Bragg diffraction angle in degree. The average size of RuO
2NPs is calculated to be 35.74 nm.
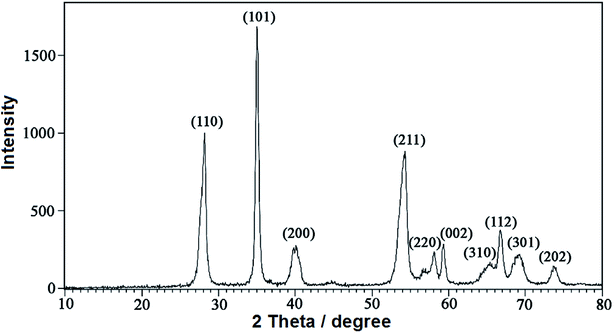 |
| Fig. 4 XRD pattern of RuO2NPs. | |
Fig. 5 displays the transmission electron microscopy (TEM) image of RuO2NPs. The NPs have an average size of ∼20 nm.
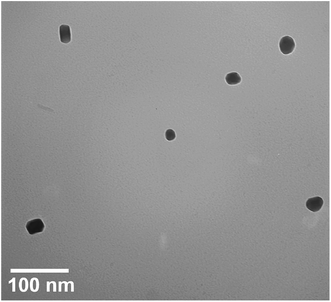 |
| Fig. 5 TEM image of RuO2NPs. | |
Electrochemical behaviour of clozapine on the RuO2 NP/SPE
It is crucial to have an optimal pH-value for studying the electrochemical behaviour of clozapine that is pH-dependent so that we can reach precise outputs. However, we conducted the tests by employing the modified electrodes at distinct pH-values in the range between 2.0 and 9.0 in 0.1 M phosphate buffer saline (PBS). The results indicated the occurrence of the most acceptable outputs for clozapine electro-oxidation at pH equal to 7.0 (Fig. 6).
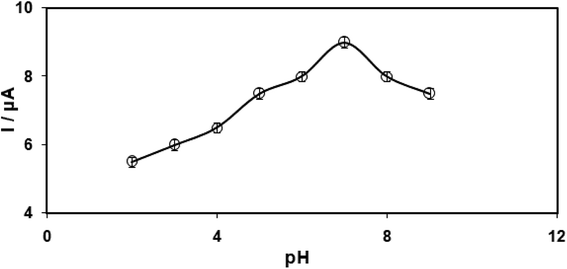 |
| Fig. 6 Plot of Ipa vs. pH of 0.1 M PBS (2.0, 3.0, 4.0, 5.0, 6.0, 7.0, 8.0, and 9.0) at the surface of RuO2 NPs/SPE with 100.0 μM clozapine. | |
Fig. 7 shows the cyclic voltammograms in the presence of 100.0 μM clozapine through the bare SPE (curve b) and the RuO2 NP/SPE (curve c). As seen from the CV results, the greatest level of clozapine oxidation on the RuO2 NP/SPE occurs at 300 mV that is nearly 170 mV more negative in comparison to the unmodified SPE evidencing the reduced polarization owing to the improved conductivity. In addition, CV of the RuO2 NP/SPE in the absence of clozapine shows no peak current (curve a) suggesting the negligible redox activity. Furthermore, there was an increase in the anodic peak current of clozapine at the surface of the RuO2 NP/SPE (curve c) compared with that at bare SPE (curve b) because of the existence of RuO2 NPs at the surface of the electrode, resulting in a higher surface area and electrical conductivity.
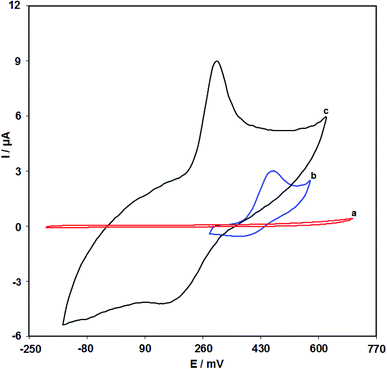 |
| Fig. 7 The cyclic voltammogram of (a) bare SPE in 0.1 M PBS (pH = 7.0) in the absence of clozapine (b) bare SPE and (c) RuO2 NP/SPE in 0.1 M PBS (pH = 7.0) in the presence of 100.0 μM clozapine at a scan rate of 50 mV s−1. | |
Effects of the scan rate on the outputs
It is notable that the increase in the scan rate causes greater oxidation peak currents with regard to outputs gained by examining the effects of potential scan rates on the clozapine oxidation current (Fig. 8). In addition, we found a linear association between Ipa and the square root of the potential scan rate (ν1/2), indicating that diffusion controls the clozapine oxidation process.48
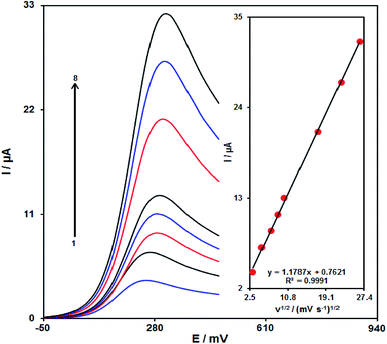 |
| Fig. 8 Linear sweep voltammogram of RuO2 NP/SPE in 0.1 M PBS (pH = 7.0) consisting of 100.0 μM clozapine at different scan rates. Numbering 1–8 refer to 10, 25, 50, 75, 100, 300, 500, and 700 mV s−1. Inset shows the variation of anodic peak current vs. ν1/2. | |
Chronoamperometric analysis
The chronoamperometry analysis for clozapine samples was conducted using the RuO2 NP/SPE at 0.35 V. Fig. 9 shows the chronoamperometric results of different concentrations of clozapine samples in PBS (pH = 7.0). Herein, the Cottrell equation for the chronoamperometry analysis of the electro-active moiety under the mass transfer-limited condition is:48 |
I = nFAD1/2Cbπ−1/2t−1/2
| (2) |
where D refers to the diffusion coefficient (cm2 s−1). Cb is the applied bulk concentration (mol cm−3). Fig. 7a depicts the experimental outputs of I vs. t−1/2 so that the best fit has been obtained for the different concentrations of clozapine. Moreover, Fig. 7b shows the resultant slopes relative to the straight line in Fig. 7a vs. the clozapine concentration. In addition, the mean value of D was calculated to be 1.3 × 10−6 cm2 s−1 based on the Cottrell equation and the final slope of Fig. 7b. This value is comparable with the diffusion coefficient value obtained in a previous research (2.1 × 10−5 cm2 s−1).49
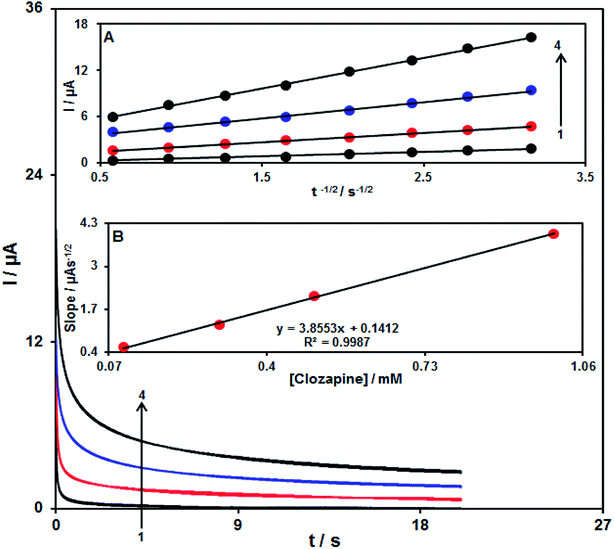 |
| Fig. 9 Chronoamperograms achieved at the RuO2 NP/SPE in 0.1 M PBS (pH = 7.0) for the different concentrations of clozapine. Numbering 1–4 refer to the 0.1, 0.3, 0.5 and 1.0 mM concentrations of clozapine. Insets: (A) I plot vs. t−1/2 achieved from chronoamperograms 1 to 4. (B) The slope plot of straight lines vs. clozapine concentration. | |
Calibration curve
With regard to the clozapine resultant peak currents at the RuO2 NP/SPE, clozapine was quantitatively analysed in water (Fig. 10). We also utilized the modified electrode (RuO2 NP/SPE) as the working electrode within a range of clozapine. DPV, because of its greater sensitivity and more reasonable functions in the analytical utilizations, was used with the following parameters: initial potential (0.1 V), end potential (0.5 V), step potential (10 mV) and modulation amplitude (25 mV). However, outputs implied the linear relationship between peak currents and clozapine concentrations in the concentration range between 0.2 and 500.0 μM, having a correlation coefficient of 0.9991 and a sensitivity of 0.076 μA. μM−1. Finally, the detection limit at 3σ was determined to be 0.07 μM.
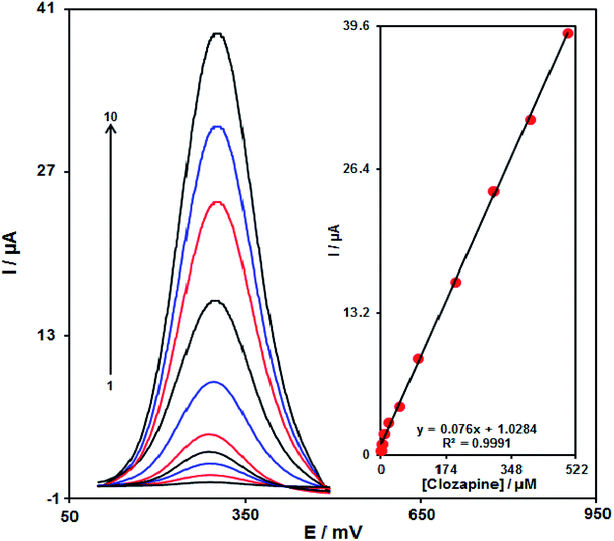 |
| Fig. 10 Differential pulse voltammograms of the RuO2 NP/SPE in the 0.1 M PBS (pH = 7.0) consisting of different concentrations of clozapine. Numbering 1–10 refer to 0.2, 2.5, 7.5, 20.0, 50.0, 100.0, 200.0, 300.0, 400.0, and 500.0 μM of clozapine. Inset: the peak current plot as a function of clozapine concentration ranging between 0.1 and 500.0 μM. | |
Reproducibility and stability of the RuO2 NP/SPE
In order to test the RuO2 NP/SPE stability, we maintained our sensor at pH 7.0 in PBS for 14 days and recorded the cyclic voltammograms of the solution consisting of 30.0 μM of clozapine in order to compare to the cyclic voltammogram achieved prior to the immersion. Results show no change in the clozapine oxidation peak. Moreover, the current declined by approximately 2% in the signals as compared with the initial responses, implying a reasonable stability of the RuO2 NP/SPE.
We analyzed the antifouling feature of the modified SPE toward clozapine oxidation and its products via CV for the modified SPE prior to and following its utilization in the presence of clozapine. Moreover, we recorded cyclic voltammogram in the presence of clozapine following the cycling of the potential fifteen times at a 50 mV s−1. Results showed decline in the currents by approximately 2% and no alteration in the peak potentials.
Analysis of real samples
We used clozapine in the clozapine tablet and the urine samples via the aforementioned procedure in order to assess the proposed modified electrode utility to detect the real samples. Therefore, a standard addition procedure was utilized. Table 1 reports the analysis outputs. The test outcomes showed acceptable recoveries for clozapine. The method reproducibility was investigated via the relative standard deviation (RSD).
Table 1 The application of the RuO2 NP/SPE for the determination of clozapine in real samples (n = 5). All concentrations are in μM
Sample |
Spiked |
Found |
Recovery (%) |
R.S.D. (%) |
Clozapine tablet |
0 |
4.5 |
— |
0.2 |
2.5 |
5.9 |
98.3 |
1.7 |
7.5 |
12.4 |
103.3 |
2.8 |
12.5 |
17.2 |
101.2 |
2.4 |
17.5 |
21.8 |
99.1 |
1.9 |
Urine |
0 |
— |
— |
— |
5.0 |
4.9 |
98.0 |
1.9 |
10.0 |
10.1 |
101 |
2.3 |
15.0 |
14.9 |
99.3 |
2.2 |
20.0 |
20.6 |
103.0 |
3.5 |
Conclusions
The present study measured the detection of clozapine at a RuO2 NP/SPE. According to the results, RuO2 NPs have stability, higher responses, and sensitive detection towards clozapine in comparison with the unmodified SPE. The RuO2 NP/SPE has higher electro-catalytic activities toward clozapine oxidation, indicating 0.076 μA μM−1 sensitivity, a low detection limit of 0.07 μM, and a large linear range of response with the clozapine concentration of 0.2 to 500.0 μM. At the end, this technique ability has been shown in the detection of clozapine in the real specimens with reasonable outputs. Therefore, the RuO2 NP/SPE showed the potential of being constructed as an electrochemical sensor to detect clozapine quantitatively.
Conflicts of interest
There are no conflicts to declare.
Acknowledgements
The authors acknowledge the financial support provided for this project (Project No. 98000791) by Neuroscience Research Center, Kerman University of Medical Sciences, Kerman, Iran. Also, this research was supported by the Future Material Discovery Program (2016M3D1A1027666), Basic Science Research Program (2017R1A2B3009135) through the National Research Foundation of Korea. Also, China Scholarship Council (201808260042) is appreciated.
References
- C. J. Carter, Schizophr. Res., 2006, 86, 1–14 CrossRef PubMed.
- S. Ulrich, R. Wolf and J. Staedt, Serum level of clozapine and relapse, Ther. Drug Monit., 2003, 25, 252–255 CrossRef PubMed.
- A. A. Mohamed and S. M. Al-Ghannam, Farmaco, 2004, 59, 907–911 CrossRef PubMed.
- W. Jin, Q. Xu and W. Li, Electrophoresis, 2000, 21, 1415–1420 CrossRef.
- M. M. Zheng, S. T. Wang, W. K. Hu and Y. Q. Feng, J. Chromatogr. A, 2010, 1217, 7493–7501 CrossRef.
- I. Vardakou, A. Dona, C. Pistos, G. Alevisopoulos, S. Athanaselis, C. Maravelias and C. Spiliopoulou, J. Chromatogr. B: Anal. Technol. Biomed. Life Sci., 2010, 878, 2327–2332 CrossRef.
- G. Zhang, A. V. Terry Jr and M. G. Bartlett, J. Chromatogr. B: Anal. Technol. Biomed. Life Sci., 2007, 856, 20–28 CrossRef.
- A. Wohlfarth, N. Toepfner, M. Hermanns-Clausen and V. Auwärter, Anal. Bioanal. Chem., 2011, 400, 737–746 CrossRef.
- K. Farhadi and A. Karimpour, Anal. Sci., 2007, 23, 479–483 CrossRef CAS.
- M. Arvand and M. G. Shiraz, Electroanalysis, 2012, 24, 683–690 CrossRef CAS.
- A. Shamsi, F. Ahour and B. Sehatnia, J. Solid State Electrochem., 2018, 22, 2681–2689 CrossRef CAS.
- M. Mazloum-Ardakani, H. Beitollahi, M. K. Amini, F. Mirkhalaf, B. F. Mirjalili and A. Akbari, Analyst, 2011, 136, 1965–1970 RSC.
- K. Zhang, T. H. Lee, M. A. Khalilzadeh, R. S. Varma, J.-W. Choi, H. W. Jang and M. Shokouhimehr, ACS Omega, 2020, 5, 1634–1639 CrossRef CAS.
- K. Zhang, T. H. Lee, J. H. Cha, R. S. Varma, J.-W. Choi, H. W. Jang and M. Shokouhimehr, ACS Omega, 2019, 4, 21410–21416 CrossRef CAS.
- K. Zhang, T. H. Lee, J. H. Cha, H. W. Jang, J.-W. Choi, M. Mahmoudi and M. Shokouhimehr, Sci. Rep., 2019, 9, 1–8 CrossRef.
- K. Zhang, T. H. Lee, H. Noh, T. Islamoglu, O. K. Farha, H. W. Jang, J.-W. Choi and M. Shokouhimehr, ACS Appl. Mater. Interfaces, 2019, 11, 31799–31805 CrossRef CAS.
- K. Zhang, T. H. Lee, B. Bubach, M. Ostadhassan, H. W. Jang, J.-W. Choi and M. Shokouhimehr, RSC Adv., 2019, 9, 26668–26675 RSC.
- K. Zhang, T. H. Lee, B. Bubach, M. Ostadhassan, H. W. Jang, J.-W. Choi and M. Shokouhimehr, RSC Adv., 2019, 9, 21363–21370 RSC.
- P. Nicholas, R. Pittson and J. P. Hart, Food Chem., 2018, 241, 122–126 CrossRef CAS.
- M. A. Khalilzadeh, S. Tajik, H. Beitollahi and R. A. Venditti, Ind. Eng. Chem. Res., 2020, 59, 4219–4228 CrossRef CAS.
- S. Srikanta, P. ParmeswaraNaik and G. Krishanamurthy, Asian J. Green Chem., 2019 DOI:10.33945/SAMI/AJGC/2020.2.1.
- P. Bollella, G. Fusco, D. Stevar, L. Gorton, R. Ludwig, S. Ma, H. Boer, A. Koivula, C. Tortolini, G. Favero and R. Antiochia, Sens. Actuators, B, 2018, 256, 921–930 CrossRef CAS.
- M. R. Ganjali, Z. Dourandish, H. Beitollahi, S. Tajik, L. Hajiaghababaei and B. Larijani, Int. J. Electrochem. Sci., 2018, 13, 2448–2461 CrossRef CAS.
- K. Zhang, T. H. Lee, O. K. Farha, H. W. Jang, J.-W. Choi and M. Shokouhimehr, Cryst. Growth Des., 2019, 19, 7385–7395 CrossRef CAS.
- M. A. Khalilzadeh and M. Borzoo, J. Food Drug Anal., 2016, 24, 796–803 CrossRef CAS.
- H. Beitollahi, S. Tajik, M. H. Asadi and P. Biparva, J. Anal. Sci. Technol., 2014, 5, 1–9 Search PubMed.
- K. Zhang, T. H. Lee, M. A. Khalilzadeh, R. S. Varma, J.-W. Choi, H. W. Jang and M. Shokouhimehr, ACS Omega, 2020, 5, 1634–1639 CrossRef CAS.
- K. Zhang, T. H. Lee, J. H. Cha, H. W. Jang, M. Shokouhimehr and J.-W. Choi, Electron. Mater. Lett., 2019, 15, 727–732 CrossRef CAS.
- H. Beitollahi, M. A. Khalilzadeh, S. Tajik, M. Safaei, K. Zhang, H. W. Jang and M. Shokouhimehr, ACS Omega, 2020, 5, 2049–2059 CrossRef CAS.
- K. Zhang, T. H. Lee, J. H. Cha, H. W. Jang, M. Shokouhimehr and J.-W. Choi, Electron. Mater. Lett., 2019, 15, 720–726 CrossRef CAS.
- S. Tajik, M. A. Taher, H. Beitollahi and M. Torkzadeh-Mahani, Talanta, 2015, 134, 60–64 CrossRef CAS.
- K. Zhang, R. S. Varma, H. W. Jang, J.-W. Choi and M. Shokouhimehrb, J. Alloys Compd., 2019, 791, 911–917 CrossRef CAS.
- K. Zhang, T. H. Lee, J. H. Cha, R. S. Varma, J.-W. Choi, H. W. Jang and M. Shokouhimehr, Sci. Rep., 2019, 9, 1–10 CrossRef.
- S. Esfandiari-Baghbamidi, H. Beitollahi, S. Tajik and R. Hosseinzadeh, Int. J. Electrochem. Sci., 2016, 11, 10874–10883 CrossRef.
- N. Rabiee, M. Safarkhani and M. Rabiee, Asian J. Nanosci. Mater., 2018, 1, 63–73 Search PubMed.
- Y. Li, X. Zhai, X. Liu, L. Wang, H. Liu and H. Wang, Talanta, 2016, 148, 362–369 CrossRef CAS.
- M. A. Khalilzadeh and Z. Arab, Curr. Anal. Chem., 2017, 13, 81–86 CrossRef CAS.
- H. Beitollahi, H. Karimi-Maleh and H. Khabazzadeh, Anal. Chem., 2008, 80, 9848–9851 CrossRef CAS.
- D. W. Li, Y. T. Li, W. Song and Y. T. Long, Anal. Methods, 2010, 2, 837–843 RSC.
- Z. Shamsadin-Azad, M. A. Taher, S. Cheraghi and H. Karimi-Maleh, J. Food Meas. Charact., 2019, 13, 1781–1787 CrossRef.
- C. Karuppiah, K. Muthupandi, S. M. Chen, M. A. Ali, S. Palanisamy, A. Rajan, P. Prakash, F. M. A. Al-Hemaid and B. S. Lou, RSC Adv., 2015, 5, 31139–31146 RSC.
- S. Tajik, H. Beitollahi and P. Biparva, J. Serb. Chem. Soc., 2018, 83, 863–874 CrossRef CAS.
- W. H. Elobeid and A. A. Elbashir, Prog. Chem. Biochem. Res., 2019, 2, 24–33 CrossRef.
- Y. Yang, A. M. Asiri, D. Du and Y. Lin, Analyst, 2014, 139, 3055–3060 RSC.
- N. Soin, S. S. Roy, S. K. Mitra, T. Thundat and J. A. Mclaughlin, J. Mater. Chem., 2012, 22, 14944–14950 RSC.
- M. D. Stoller, S. Park, Y. Zhu, J. An and R. S. Ruoff, Nano Lett., 2008, 8, 3498–3502 CrossRef CAS PubMed.
- S. Tajik, H. Mahmoudi-Moghaddam and H. Beitollahi, J. Electrochem. Soc., 2019, 166, B402–B406 CrossRef CAS.
- A. J. Bard and L. R. Faulkner, Electrochemical methods fundamentals and applications, Wiley, New York, 2nd edn, 2001 Search PubMed.
- F. Huang, S. Qu, S. Zhang, B. Liu and J. Kong, Talanta, 2007, 72, 457–462 CrossRef CAS PubMed.
|
This journal is © The Royal Society of Chemistry 2020 |
Click here to see how this site uses Cookies. View our privacy policy here.