DOI:
10.1039/D0RA00735H
(Review Article)
RSC Adv., 2020,
10, 14040-14049
Microneedles: a potential strategy in transdermal delivery and application in the management of psoriasis
Received
23rd January 2020
, Accepted 26th March 2020
First published on 7th April 2020
Abstract
The skin is an absorptive organ, targeted in the transdermal delivery system. The microneedle method is an updated approach to deliver compounds through the skin in an efficient way. A microneedle patch is composed of dozens to hundreds of micron-sized needles with various structures and advantages benefiting from their special and smart designs. The microneedles method is much more superior to those traditional transdermal delivery ways because of advantages such as minimally invasive, painless, convenience, and improved patient compliance. Up to now, the microneedles field has developed rapidly and is divided into four major types by researchers: solid microneedles (SMNs), hollow microneedles (HMNs), dissolving microneedles (DMNs), and coated microneedles (CMNs). Every type of these microneedles has different advantages according to their unique properties and designs. Psoriasis is a polygenic chronic dermatological disease with no cure, and the treatment is based on symptom control. The traditional therapeutic method is diverse, embracing topical drugs, systemic drugs, physical therapy, etc. With the limitation of present drug delivery, the demand for new delivery methods for psoriasis is in the spotlight. Thus, this review paper aims to provide a comprehensive statement about the microneedles, the progress of the microneedle application in the management of psoriasis and the clinical challenges.
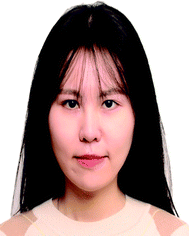 Zihan Zhao | Zihan Zhao is presently a postgraduate student in Tongji University and her research interests are inflammatory skin diseases especially psoriasis, microneedles, and nano-carrier. She has published 3 papers in SCI journals. |
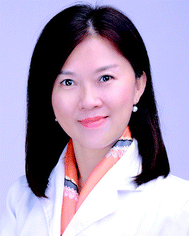 Yuling Shi | Prof. Yuling Shi is the professor, chief physician, doctoral supervisor of Dermatology and Venereology. She is the director of the Institute of Psoriasis, Tongji University School of Medicine who has been involved in basic and clinical research on inflammatory skin diseases, such as psoriasis for long term. She graduated from the Second Military Medical University with a Ph.D. in Dermatology and has conducted postdoctoral research at the Henry Ford Health System in the United States. She has published more than 70 professional papers in this field, including more than 40 papers in SCI journals. |
1. Introduction
The skin is the largest organ in the human body in terms of body weight and it plays an important role, not only in its shield-like external defense function but also in the regulation of temperature and water.1,2 Being the outermost layer of the body, the presence of skin protects the rest of the body from direct exposure to the various microorganisms and pathogenic factors present in the external environment. The architecture of skin has been recognized as three layers perpendicular to the skin surface: epidermis, dermis and subcutis (Fig. 1). The epidermis and dermis layers bind tightly to each other on the surface of the skin, forming a complete envelope structure, and are separated from deeper tissue by the subcutis.
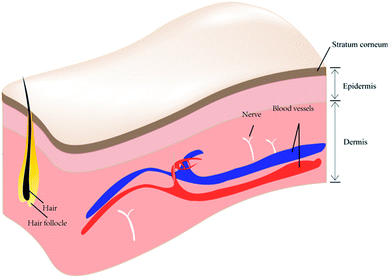 |
| Fig. 1 Skin anatomy. | |
The epidermis is the most superficial and biologically active of these layers, as the basal layer of the epithelium (stratum basale) is constantly renewing.3 The stratum corneum (SC) also forms part of the epidermis and its normal thickness is approximately 100 μm. The SC is a major anatomical component of the skin's barrier function and of its regulation of water. In some immune skin diseases, the skin is the target tissue of the immune response attack. Moreover, the human skin itself forms a “skin immune system”, which consists of many different types of cell including Langerhans cells, T lymphocytes, keratinocytes, macrophages, and dendritic cells. One of the basic functions of human skin is absorption, and the realization of this function mainly depends on the existence of the SC. In dermatologic conditions, the absorption function of the skin is the principle method by which topical drugs are used to treat diseases. However, the absorption function of the skin is limited, and is an obstacle to topical drug absorption by the skin. It must be mentioned here that there is an easily overlooked function of skin – aesthetic appreciation. A particularly interesting claim is that people “wear their health” on their skin.4 The smoothness, integrity, and evenness of human skin can greatly influence a person's appearance from a visual perspective. There is no wonder why people care about skin conditions and how skin problems are solved in numerous ways. Therefore, the optimization of skin treatment is particularly important.
With skin being the largest organ of the human body, skin diseases are experienced by a wide range of patients globally. Taking psoriasis as an example, 2–3% of the world's population suffers from this condition, 80–90% of whom have plaque psoriasis; more than one-third of them present with the moderate-severe form of the disease.5 Psoriasis has a significant impact on quality of life; visible plaques, desquamation, and itching are the most common and concerning symptoms and signs. The current treatment for psoriasis, although effective, remains unsatisfactory in the long term and treatment challenges still exist.
Drug administration through the gastrointestinal tract or circulatory system sometimes increases drug consumption or reduces local drug concentration per unit area of skin. Topical cream spreads only on the skin surface. It is reported that only 10–20% or less of total drug loaded in cream is permeated through the skin.6 To solve this problem, dermatologists are increasingly turning their attention to transdermal drug delivery systems (TDDSs). In this field, emerging TDDSs using microneedles have developed rapidly in recent decades. Microneedle drug delivery systems have been developed and are considered to be hybrids of the problematic hypodermic needle and transdermal patch.7 Microneedle systems comprise a base plate with microneedles arrays perpendicular to it. The microneedles patch (MNP) is a more accurate definition of microneedles loaded with drugs. Once the microneedles pierce the skin, the loaded drug has greater opportunity to directly contact the epidermis or dermis without the challenge of a barrier. The procedure makes drug delivery much easier, bypassing the main obstacle in a “smart” way. As a convenient invasive delivery device, the properties of microneedle treatment make it an excellent choice, with the capacity to transport various drugs or other substances suitable for this method to the human body. This review aims to describe the characteristics and advantages of several accepted microneedle types and their potential in psoriasis treatment, with particular attention to the microneedle patch (MNP).
2. History, mechanism, types and advantages of microneedles
In the past decades, the probability of microneedles strategy in drug delivery through the skin has been broadly demonstrated. Researchers facilitate a leap from scratch of the application of microneedles by developing different types of microneedles and using them to treat a variety of diseases. Due to the factors such as differences in structure and relationship between cargoes and the substrate, various operation mechanisms are listed. Naturally, multifarious types of microneedles may have distinctive advantages. Common strengths and the difference are described separately below.
2.1 History of microneedles
Hypodermic needles are common medical devices that draw or inject gas or liquid with a procedure piercing the skin. They are one of the most used devices worldwide with application in subcutaneous injection, intramuscular injection, intravenous injection, vein blood collection, and various types of puncture procedure. Their use is generally associated with pain and/or discomfort. However, the limited absorption capacity of the SC and the size and other properties of drug molecules has meant that the application of many drugs through the alternative method of traditional TDDS such as transdermal patches is restricted. The dosage of drugs may need to be increased to achieve a more satisfactory concentration of local skin, or smarter methods, such as using microneedles, may be required. Gerstel and Martin from Alza Corporation first raised the concept of “microneedles” in 1976 as a way of conquering the pain issue in drug administration.8 This is undoubtedly an extremely forward-looking sketch that laid the foundation for the initial goal of microneedle development as a solution to the pain problem. But not until the 1990s, with vast improvement in techniques in the microfabrication industry, were microneedles developed with more precise structure and better function.
In 1998, a novel approach to transdermal drug delivery that dramatically enhanced the transport of molecules across skin was presented.9 Microneedles microfabricated by etching arrays of micron-sized needles into silicon were shown to be capable of dramatically increasing permeability to calcein more than 1000-fold when inserted into the skin. This indicated that the application of microneedles could potentially be improved greatly and has promoted researchers' enthusiasm to explore the field of microneedles. A few years later, Matriano et al. reported that a type of commercial microprojection array patch (Macroflux@) coated with ovalbumin (OVA) at 1 and 5 μg doses resulted in immune responses up to 50-fold greater than observed after the same cutaneous or intramuscular dose.10 This result was of interest because of the vital role the immune response plays in many diseases. The use of the MNP became more prominent with an increasing amount of research into this smart device.
2.2 Mechanism of microneedles
Microneedles comprise two parts: invasive and supporting components. The invasive component is an array formed by hundreds of needles with length from 25 to 2000 microns.8 The supporting part is a base plate that offers an appropriate, uniform mechanical support for the sharp needle tips to enable them to pierce through the SC (Fig. 2). These two parts can be fabricated from the same kind of material or fabricated using separate raw materials before they are tightly bonded with each other. The most important purposes of an MNP is to create a micron-sized channel on the skin layer and to work as a delivery system. MNP function is influenced by MNP design and requires sufficient hardness, suitable mechanical strength, and sufficient toughness. Signs of failure of an MNP are needle tips that bend, deflect or fracture. These demands make choosing applicable materials and types of MNP critical.
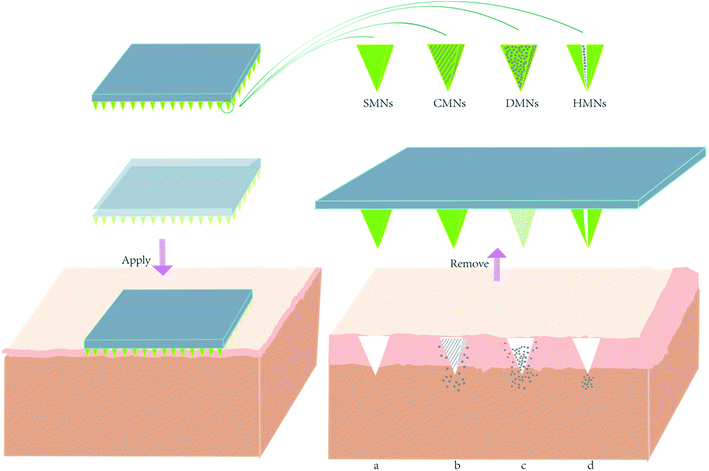 |
| Fig. 2 The schematic diagram of compounds delivery mechanism of four different types of microneedles patches. When the microneedle was removed from the surface of the skin, (a) SMNs left pores in the skin; (b) CMNs deliver compounds from the outer coated layer containing compounds; (c) DMNs' needle tips dissolve and release compounds; (d) HMNs deliver compounds through the hollow hole in the needle. | |
2.3 Types of microneedles
With research validated and implemented, the application of microneedles has been expanded. Microneedles are now generally divided into four types depending on transportation method: solid microneedles (SMNs), hollow microneedles (HMNs), dissolving microneedles (DMNs), and coated microneedles (CMNs).
2.3.1 Solid microneedles. While this review focuses more on the MNP, SMNs for pretreatment will be described for the completeness of classification. To some degree, all other types of microneedles are upgraded optimized designs of SMNs. SMNs are regarded as device pretreatment for the delivery of compounds via the skin. By simply inserting and pulling out SMNs there will be micron-sized channels left on the surface of the skin. The shape, depth, and diameter of the channel are determined by the SMN design. The array of SMNs ranges from 5 × 5 to 20 × 20.11 After the application of SMNs, tiny pores across the epidermis and dermis enable faster and more efficient delivery of a drug from a patch. The relatively simple “poke and patch” procedure exemplifies the SMN mechanism,12 representing an early stage in microneedle development. Later devices have tended to integrate microneedles with patches.Mikszta et al. reported a meaningful attempt at topical gene transfer.13 In this study, devices known as microenhancer arrays (MEAs) were used, microfabricated with silicon projections that function as SMNs to achieve penetration of the skin barrier. MEA geometry was characterized by projection heights ranging from 50 to 200 microns and the interval between each projection was twice the height. MEAs were designed to be placed on a hand-held applicator and used to deliver naked plasmid DNA encoding firefly luciferase topically to untreated mice skin, resulting in mean luciferase activity using this lateral pass method over 1000-fold higher than that in topical controls, after six applications. The same research also demonstrated that DNA encoding hepatitis B surface antigen delivered by MEAs induced a powerful immune response. Additionally, 100% seroconversion was achieved by MEA delivery after two immunizations compared with 40% achieved via intramuscular injection and 50% via intradermal injection. This study prompted interest in the potential of SMNs in the vaccine field and researchers turned their focus to the application of vaccines with microneedles.14 In 2004 it was reported that the use of SMNs improved the efficacy of insulin transdermal transport and reduced blood glucose levels by as much as 80%.15 Martanto and co-workers fabricated SMNs with stainless steel, finally fabricating arrays with 7 rows of 15 needles each, giving a total of 105 needles. Each needle had a tapering tip 1000 microns in length. These SMNs were inserted and removed from the surface of hairless mice skin, after which the insulin solution was kept in contact with the rat's skin for 4 hours. The researchers found that the strategy performed surprisingly better than the injection method of 0.05 U insulin and in comparison to negative controls in which the rat's skin was not pretreated. They then measured plasma insulin and found that shorter needle insertion time resulted in larger plasma insulin levels. For delivery of compounds, SMNs undermine the skin barrier and provide good penetration.
2.3.2 Hollow microneedles. The structure and mechanical principles of HMNs are similar to those of hypodermic needles with a channel; the center of the needle tubes are hollow and there are holes at the needle tips. The compounds flow through the cavity of the needle, actuated by pressure equipment. The flow speed of compounds stored in the needle into the body can be completely controlled by specially designed devices, increasing personalized design for different drug delivery needs. Based on previous research, HMNs are used for high molecular weight compounds such as proteins, vaccines, and oligonucleotides.16Niu et al. found that intradermal delivery by hollow microneedle array creates a sustained release depot in the skin and can create a burst transit through draining lymph nodes.17 Strong support to the application value of HMNs was given when researchers found that HMN array transfer resulted in a significantly higher immune response compared with the intramuscular injection method. A case study in 2018 reported that use of the HMN strategy resulted in a “steady state” of insulin plasma concentration that mimicked a multiple-dose regimen.18 In an in vivo experiment in the same study, the HMN array (13 × 13 mm2) was designed with 100 microneedles (220 μm high, 130 μm outer diameter, and 50 μm-inner diameter) with a special microneedle array carrier and a prestretching belt through which significant variables could be controlled. Once the microneedle array was attached to the skin surface with the aid of a fixative, a preset syringe pump began delivering liquid compounds stored in the needle space in a continuous manner. The use of HMNs is suggested for sample extraction,19 because of their special design and structure.
2.3.3 Coated microneedles and dissolving microneedles. CMNs and DMNs are introduced together in this section due to similarities in both their fabrication material and the manner of delivering compounds. In these two designs, the compounds have close contact with the body of the needle. In CMNs, a coating of drug solution is applied to the surface of the needle, while a biodegradable polymer with drugs dissolved in it is used in DMNs. Neither of them need a subsequent procedure for transdermal drug delivery.Generally speaking, the coated drug layer stays water soluble so that the drug can be released from the outer layer after the insertion of CMNs into the surface of the skin and with sufficient exposure to the watery environment of the tissue. In the research of Lee et al., bleomycin-coated microneedles were developed to complete the delivery of bleomycin into the subepidermal skin layer, providing an effective treatment for warts without causing pain or fear in patients.20 The application of CMNs in the treatment of peanut allergies has been recently studied.21 The researchers found that a CMN group treated with peanut protein extract had significant improvement in clinical symptoms and an upregulation of Th1 cytokines compared with an untreated group, suggesting the use of a CMN strategy successfully accelerated immune regulation involving the Th1 pathway. In the treatment of ocular disease, CMNs also play an important role; a more detailed introduction can be found elsewhere.22
As mentioned previously, DMN devices are fabricated with biodegradable polymers containing the compounds to be delivered. The choice of materials is crucial, not only to fulfill various physical parameters such as mechanical strength and toughness, but also for bio-friendliness – the tips of the needle will dissolve, circulate and metabolize in the human body. The speed of drug release is decided by the speed at which the polymer material dissolves.
Despite the strict requirements for efficacy, DMNs have a broad research scope and application value. Dillon et al. have worked on encapsulating active drug peptides in a microneedle matrix.23,24 They presented a DMN system composed of polyvinylpyrrolidone (PVP) and trehalose to encapsulate active pharmaceutical peptides within the microneedles. In their design, the drug remains active after 10 weeks of storage at room temperature. Colin and coworkers also proved that micron channels in the skin surface will help the absorption of compounds in the initial stage of the application. A recent study reported that DMNs can be used as powerful tools in clinical photodynamic therapy.25 Use of DMNs loaded with 5-ALA, a widely used prodrug in photodynamic therapy, resulted in high activity and achieved greater inhibition of tumors at a lower dose with a shorter application time than conventional methods.
2.4 Advantages of microneedles
While the concept of “microneedles” was put forward in 1976, it was a strategy conquering the pain issue in drug administration. With the development of structure industrial technology and the gathering of wisdom, the advantages of microneedles have been rapidly expanded. Microneedles exhibit superiority in the safety, convince, sample extraction and so forth. At the same time, different kinds of microneedles have been developed with unique applications and dominances.
2.4.1 Advantages across microneedle types.
2.4.1.1 Minimally invasive and pain. Among the advantages of microneedles, the minimal degree of invasiveness is the most powerful when compared with traditional hypodermic needles. With needle length and width at the level of microns, the damage to the skin caused by each needle is slight. This brings additional benefits: recovery is quick, microbial organisms are less able to enter the body through the holes created by needles, and the risk of bleeding is lower. Wound healing is closely related to wound size and risk of infection. The smaller the wound, the shallower the depth and the quicker the recovery. Hundreds of microchannels from the epidermis to dermis layers lead to tiny controlled injuries that create a relatively short active inflammation period in the skin tissue wound healing procedure.26 The minimally invasive tiny openings between the body and the external environment avoid large amounts of microorganisms invading the body. Theoretically, the shallower depth of puncture brings about a lower risk of contact between needle tip and large blood vessels. Additionally, although fainting is experienced by less than 1% of blood donors according to a large-scale study of donation records,27 this smart approach is also friendly to people who react negatively to the sight of blood.In terms of pain, needles of greater length and diameter can go deeper into the skin layers in which nerves are located, causing pain and possible nerve damage.28 For some patients suffering from needle phobia, the pain and discomfort caused by venipuncture are the main source of distress.29 A tiny MNP provides a method of successfully delivering compounds via the skin without visualizing a long metal needle. This helps patients relax, ensuring smooth treatment.
2.4.1.2 Convenience. The MNP is more convenient than other drug delivery methods. This advantage is convincingly described from the following perspectives: (1) patient self-administration via MNP is possible, as well as administration by a family member, whereas hypodermic needles should only be used by health workers with a professional license.30 MNP self-administration avoids the anxiety, incomprehension and fear associated with the medical environment, especially for pediatric patients. Patients and their families can self-inject by MNP without difficulty, after being taught the applicable methods in a short space of time. (2) Self-medication as described in the previous point is uncomplicated and reduces the risk of self-medication errors. (3) The treatment schedule for self-administration, under the advice and time frame of the treating doctors, can be more flexible than the schedule of specific medical facilities or medical personnel. This saves waiting time for patients for some medical conditions, with the doctor's appropriate advice and guidance. (4) An MNP method lowers economic costs for individuals. Since there is no need for these patients to have professional treatment in a hospital or clinic, spending on transportation, medical services, and medical supplies is reduced. Considering the low threshold for storage needs of microneedles,31 logistical costs may be reduced, saving the patient on healthcare costs.
2.4.1.3 Improved patient compliance. Better patient compliance is a generally accepted advantage of the use of TDDSs.32,33 One compliance issue is that of deliberate noncompliance, where patients arrive at the (often rational) decision not to engage in treatment as directed.34 Poor compliance has been indicated as the most common reason for nonresponse to medication or low efficacy.35 Topical treatments may have limitations such as causing tingling, itching, or sticky sensations, which may contribute to poor patient compliance. Poor compliance with medical procedures associated with needles also occurs. Considering these points, the MNP is a drug delivery system with greater focus on patients; it may be more popular among patients thus improving compliance. It has been indicated that by avoiding the first-pass metabolism effect of drugs with poor bioavailability, the dosing schedule can be reduced and as a result patient compliance can also be improved.36
2.4.1.4 Lighter medical burden. For some conditions, the medical burden is reflected by the workload of healthcare staff. A smaller workload is necessary for effective and efficient medical behavior. Several steps are required for a patient to receive a simple injection treatment, including making an appointment, arranging and buying drugs, paying for medical services and related supplies, waiting in line, and finally receiving their injection. Every procedure in this long interlocking chain needs the participation of healthcare staff. Overloaded situations may occur, affecting the enthusiasm and concentration of personnel, and risking a higher rate of medical error. The use of an MNP involves the once-off cost of teaching self-administration, and a long period of autonomous treatment is guaranteed if no complications occur. Compared to methods such as subcutaneous injection, using this smart device reduces duplication of effort and frees up healthcare staff to focus on more complex interventions.
2.4.1.5 Reduction of risk of accidental exposure. Healthcare staff may be affected by accidental injury or accidental occupational exposure despite professional diagnosis, treatment, and nursing care. The most invasive procedures provide opportunities for contact with the patient's blood, body fluids and secretions, and with medical waste, increasing the risk of sharp instrument injury or exposure to exposed skin and mucous membrane. Needle injury is the most common exposure injury in healthcare staff in the course of diagnosis and treatment. Nursing staff experience the highest needle injury incidence in the workplace. Most needles used are solid and hollow, such as hypodermic needles. Intravenous puncture, injection, blood drawing, and other procedures may easily cause needle injury. The self-injected use of an MNP potentially reduces medical risk exposure by less reliance on traditional needle use.
2.4.1.6 Other advantages. The MNP method is more cost-effective in terms of production. As mentioned previously, MNPs are highly stable because a drying procedure is required in their fabrication, resulting in higher stability. Indirectly, this removes the need for cold chain storage and specialized transport systems, which has considerable impact in developing regions where cold chain storage facilities may be scarce.31 Additionally, the reuse of hypodermic needles is an ongoing concern in developing regions. Most MNPs are disposable medical products without sharp biohazardous waste, and lower medical pollution is expected.
2.4.2 Unique advantages of different types of microneedles.
2.4.2.1 Stability provided by CMNs and DMNs. Differing from the other two types of MNP, in CMNs and DMNs the compounds are dissolved inside or covered. The compounds delivered to the skin may be the result of a complex fabrication procedure in combination with the initial materials, since they compose an integrated patch. In one design of IgG-loaded hyaluronan-based dissolving microneedles, the stability of IgG was tested using intrinsic fluorescence spectroscopy.37 The results of the same peak location indicated that the tertiary structure of the IgG load was not altered during the DMN fabrication process. The research also proved that there was no complexation of hyaluronan and IgG in the DMN. Another team developed a type of DMN to deliver human growth hormone (hGH) and found that the hGH remained complete after encapsulation and retained most of its activity after storage for up to 15 months at room temperature and in humidity.38 This is excellent stability – many drugs that require oral and intravenous administration in clinical practice do not stay stable for such a long period. For CMNs, excellent stability was showed when recombinant human erythropoietin alfa (EPO) was coated on a titanium microneedle.39 The ZP-EPO patch was stable at room temperature for at least 3 months with no significant change observed in terms of percentage aggregates, isoforms, or potency.
2.4.2.2 Sample extraction function of HMNs. An extraction function is provided by the special structure of HMNs. As early as 2005, the concept of using MNPs for biological sampling was proposed.40 A following qualitative study focusing on schoolchildren aged 10 to 14 years showed that HMNs had an improved acceptance in comparison to traditional blood sampling among the children.41 An interstitial fluid (ISF) extraction function has also been expanded.42 In their study, Samant and coworkers designed an MNP to sample ISF from the skin using suction as the driving force. HMNs have potential to serve as new technology to monitor biochemical indexes.
3. Application of microneedles in psoriasis
Psoriasis is a polygenic inflammatory chronic disorder disease of the skin. It is immune-mediated and relapse is common.43 Psoriasis reportedly affects approximately 2% of the world's population,5 negatively influencing the life quality of patients. The exact cause of psoriasis is still unclear. Despite being studied for a long period, and with some achievements, no firm conclusions on the etiology and pathogenesis of this disease have been reached so far. The immune system is involved in psoriasis development and the key pathogenic factor including but not limited to TNF- α, IL-17A, IL-23 as it's been widely acknowledged. Psoriasis lesions typically feature erythema, scales, red papules, and thickened skin. Itching, desquamation, and visible plaques are the most concerning common symptoms and signs of psoriasis and may have a serious impact on the quality of life; that there is no cure for psoriasis so far is regrettable. Local and systemic drugs have been widely used for a long time in dermatologic disease treatment. Topical drugs, physical therapy, and systemic therapy are used in the treatment of psoriasis to avoid progressive damage to health. However, the topical treatment is time-consuming and messy, with the oiliness and stickiness of topical treatments commonly reported problems. The physical therapy, constituting a significant part in the psoriasis treatment, has a relatively strict requirement of treating frequency and time. Systemic therapy is often associated with side effects.34 It is been reported that 74% of drugs delivered orally are not as effective as desired.44 An alternative injection choice is desirable when poor efficiency arises, which is usually associated with pain and fear. In the systemic therapy, the first-line drugs in the treatment of psoriasis include methotrexate (MTX), cyclosporine A (CyA), and retinoic acid.5 These drugs are effective but bring about harmful side effects such as hepatotoxicity, impaired renal function, and hypertension. Traditional oral, intramuscular, or intravenous drips may also be administered. With the advent of targeted biologics, the efficacy of the targeted attack on pathogenic factors is more significant than that of traditional drugs. The high cost of biologics is an obstacle to extensive promotion.
Because of limitations of the existing treating method such as relatively poor penetration, low efficacy and side effects as mentioned previously, researchers are committed to performing innovative research into drug delivery methods. MNP strategies for psoriasis are of particular focus here. A summary of basic information is listed in Table 1.
Table 1 The application of microneedles in the treatment for psoriasis
Delivered compounds |
Matrix material |
Microneedles type |
Microneedles design |
Transdermal delivering efficacy in vivo |
Ref. |
MTX |
Soluble maltose |
SMNs |
28 (8 × 2) needles with height of 500μm |
— |
45 |
MTX |
Poly lactic-co-glycolic acid (PLGA) |
SMNs |
100 (10 × 10) needles with height of 500μm |
— |
46 |
MTX |
Hyaluronic acid, (HA) |
DMNs |
100 (10 × 10) needles with height of 650 ± 19 μm |
About 90% maximum |
47 |
CyA |
Hydroxypropyl cellulose |
DMNs |
100 (10 × 10) needles with a height of 600μm |
— |
50 |
Anti-TNF-α Ab |
Sodium carboxymethyl cellulose |
DMNs |
100 (10 × 10) needles with height of 700μm |
Greater than 75% maximum |
52 |
Anti-TNF-α Ab |
Sodium carboxymethyl cellulose |
DMNs |
100 (10 × 10) needles with height of 700μm |
70% maximum |
53 |
Calcipotriol/betamethasone ointment |
Hyaluronic acid, (HA) |
SMNs |
76 circular cone shaped microneedles with height of 650μm |
— |
54 |
Researchers have developed MNPs to improve the MTX treatment efficacy for psoriasis and reduced the side effects caused by microneedles.45–47 Ajay K. Banga and teammates' works have reported that when MTX is applied under MNP-pretreated skin, the delivery of MTX is more effective than with control methods.45,46 In 2008, their team developed a MNP fabricated by soluble maltose, consisting of 56 (28 × 2) needles with a height of 500 μm. In this study,45 the MNP was used before the methotrexate (MTX) gel's application they claimed that the MNP enhanced MTX delivery over passive delivery. A decade later, Banga and his team designed another MNP with 100 (10 × 10) needles with a height of 500 μm.46 The matrix material is Poly Lactic-co-Glycolic Acid (PLGA) which experienced a high temperature or 180 °C for 30 min. What's more, they proved that the PLGA is a superior material to fabricate microneedles with mechanically uniform by using SEM imaging and commercial Parafilm M®. Although they focused more on the combined application of PLGA microneedles and laser, the single application of PLGA microneedles showed the ability in the improvements of transdermal transport efficacy. Du and coworkers developed a dissolving MNP made with hyaluronic acid loaded with MTX to improve efficacy of psoriasis treatment.47 In their design, the hyaluronic acid was chosen as the matrix material for the MNP, considering its excellent biological properties of biodegradability and non-immunogenicity. The MNP with MTX loaded in the needle tips was fabricated using a two-step micromolding process. It consisted of 100 (10 × 10) needles with a height of 650 ± 19 μm, base width of 220 ± 7 μm, and center space of adjacent needles of 500 ± 12 μm. The MTX spread quickly to the skin after its insertion in vitro and the release percentage reached 52% within 10 minutes, 76% and 87% at 30 minutes and 1 hour, respectively. The author points out that the initial burst release may be associated with the water solubility of hyaluronic acid. In vivo, the MNP completely dissolved within 10 minutes with a reduction in ear thickness and downregulation of the IL23/IL17 axis in the MTX-MNP group. What's more, these results indicated that the MTX loaded by DMN was more efficient in ameliorating IMQ-induced skin inflammation than taking it orally from both the epidermal thickness and expression level of Ki67 aspects (Fig. 3). This research, demonstrating a new method based on a dissolving hyaluronic-acid MNP to deliver MTX from the skin, showed a strengthened therapeutic effect in comparison with current methods.
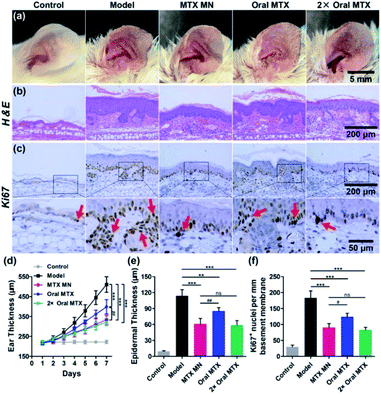 |
| Fig. 3 Therapeutic effects of MTX-loaded MNs (13.8 μg), and oral administration of the same dose (13.8 μg) and a double dose (27.6 μg) of MTX on IMQ-induced psoriasis-like skin inflammation. Representative photographs of (a) left ear lesions, (b) H&E staining, and (c) Ki67 (pointed by the red arrows) IHC staining of skin sections on day 7. The scale bars in the last images can be applied to the others in the same line. (d) Variation of ear thickness in different groups. Quantitative analysis of (e) epidermal thickness and (f) the counts of Ki67 + nuclei per millimeter of basement membrane. All experiments were performed in triplicate. Data are presented as mean ± SD (n = 5) (ns, p > 0.05; **, p < 0.01; ***, p < 0.001; #, p < 0.05; ##, p < 0.01). Reprinted with permission from ref. 47. Copyright(2019) American Chemical Society. | |
CyA is another effective immunosuppressive medication used frequently in clinical work, just as mentioned above. With its large molecular size and hydrophobic properties, transdermal delivery of this compound has been difficult. Several new transdermal therapies have been explored, bringing hope to the development of topical application of CyA.48,49 The expanded application of MNPs in the delivery of CyA has been reported by Jeong et al.50 In their research, hydroxypropyl cellulose and CyA were dissolved in methanol, forming a solution that was then poured into a special mold to fabricate a dissolving MNP containing CyA. After being pushed into porcine skin and shaved rat skin at a specified force and time with the help of a band-aid and a clip, the MNP was removed. Their results showed that mechanical strength and brittleness were in relation to the CyA content – the higher the CyA content, the lower the mechanical strength, and the more brittle the MNP. They indicated that this dissolving MNP performed well with a longer period of therapeutic effect locally compared with oral administration, and as a result, the systemic toxicity was reduced.
The horizon has broadened in recent years for new biologic therapies in psoriasis treatment, especially for moderate to severe psoriasis. Despite biologic therapies bringing new hope with good efficacy and safety, the possibility of relapse remains a weakness.43 Tumor necrosis factor-alpha specific antibody (anti-TNF-α Ab) is a biological agent inhibiting the activation of the TNF-α receptor, reducing inflammation in psoriasis. Subcutaneous and intramuscular injection are the delivery methods for this compound, and the satisfying topical concentrations reached with a relatively high dose of systemic injection,51 which may bring about non-specific immune suppression. Korkmaz et al. developed a kind of DMN loaded with anti-TNF-α Ab in the tips of the needles and found that this method demonstrated powerful delivery efficacy and anti-inflammation function in BLAB/c mice with psoriatic lesions induced by imiquimod.52 The MNP, fabricated using low viscosity sodium carboxymethyl cellulose, has a complete appearance and completely dissolved in 30 minutes with the help of an applicator. In a murine psoriasis model, the MNP was observed to have the ability to reduce inflammation from the aspects of epidermal thickness, cellular infiltrate, and IL-1β mRNA expression. These results proved that the antibody remains the necessary protein structure after the designed fabrication procedure (Fig. 4) of microneedles and supported the efficient delivery by the microneedle method. More information about anti-TNF-α Ab delivered by MNP is available in the related publication.53 These researches provide new directions for the use of biologic therapies in psoriasis treatment.
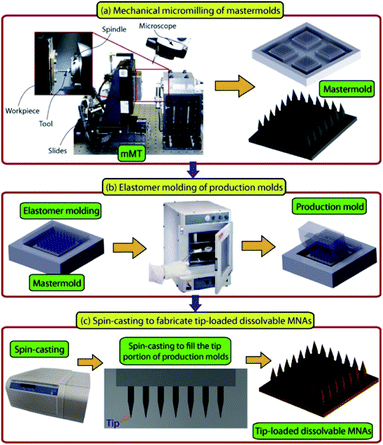 |
| Fig. 4 Description of the micromilling/spin-casting based fabrication approach used for creating tip-loaded dissolvable microneedle arrays. Three-steps: (a) mechanical micromilling of mastermolds. (b) Elastomer molding of production molds. (c) Spin-casting to localize anti-TNF-α Abs in the apex of obelisk microneedles and fabricate tip-loaded dissolvable MNAs. Images are reproduced from ref. 52. Copyright(2015) Acta Materialia Inc. | |
In 2018, an open pilot trial in Korea that included 10 patients, assessed the enhanced role of a hyaluronic acid-based MNP as a topical therapy for psoriasis.54 After a topical application of calcipotriol/betamethasone ointment (Daivobet®) daily for at least 4 weeks, resistant psoriasis plaques were selected to be the target sites of the MNP. The MNP remained on the surface of the Daivobet® pretreated plaques overnight and clinical improvement was assessed at week 0 and week 1 (Fig. 5). This clinical study reported an obvious clinical effect from the MNP on the psoriasis plaques but whether the enhancement was caused by this strategy or the hyaluronic acid was unclear, considering the lack of control.
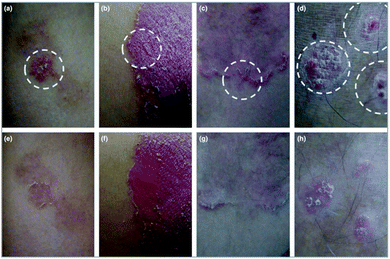 |
| Fig. 5 Psoriatic plaques improved significantly after 1 week of microneedle patch application. The circle indicated by the white dotted line is a plaque treated with a microneedle patch. The modified psoriasis area and severity index decreased from 7 to 1 in patient 1 (a and e), from 9 to 3 in patient 5 (b and f), from 5 to 0 in patient 6 (c and g) and from 6 to 2 in patient 9 (d and h). These four patients were very satisfied with the additional application of the microneedle patch. Images are reproduced from ref. 54. Copyright(2017) British Association of Dermatologists. | |
Psoriasis is characterized by excessive proliferation of keratinocytes, and excessive and incomplete keratinization could be seen. The turnover from basal cell to horny cell to exfoliation, which normally takes 28 days, takes only 4 to 7 days in psoriasis condition, because of the enhanced proliferation of epidermal cells. The thickened SC makes absorption of drugs locally difficult. As one of the most important treatment strategies of dermatological disease is topical treatment, the way it works in the case of psoriasis is restricted. The existing limitations of other current therapies as mentioned above also highlight the need for better method. The MNP, as a new strategy for the treatment of psoriasis, is playing its role in both basic and clinical research. Better efficacy, more convenient application, lower adverse reactions, faster skin barrier recovering brought from MNP therapy are the tip of the iceberg. With the number of studies and targeting drugs in the MNP field still limited, we look forward to more meaningful studies exploring an MNP approach to psoriasis treatment.
4. Challenges and prospects
There is a growing focus on MNPs as transdermal patches to deliver compounds and traditional drugs in academia, and the MNP strategy is a powerful approach to the management of dermatological conditions.55–60 Psoriasis, as a complex immune-mediated disease manifests with chronic inflammatory lesions in the skin and systemic manifestations, its treatment ladder usually including topical therapy, phototherapy, and finally systemic medication. The properties of the drugs and delivery ways bring about complicated drug-taking procedures, low efficacy or high cost, etc. The prominent plaques caused by keratinocytes hyper proliferation undoubtedly add another difficulty in the transdermal drug delivery in psoriasis. The MNP strategy is a potential method for psoriasis management, taking the researches limited but of high quality mentioned above.
Although microneedles have huge advantages in drug delivery in comparison to traditional transdermal methods, it has to be pointed out that the MNP remains a novel technology and many procedures in model design and production are not optimal. The performance of matrix material varies in different conditions and needs further investigation. Many factors have an influence on final commercial prices. MNP is an invasive technique applied to the skin and the possibility of skin irritation should be considered. Further studies on the application of MNPs are needed to overcome existing challenges and expand the scope of application.
An increase focus on the development of MNPs brings hope that existing challenges will be overcome and that more evidence on the feasibility of this smart method in clinical work will be found. A revolutionary impact on the drug delivery field from the development and use of microneedles is envisioned.
Conflicts of interest
There are no conflicts to declare.
Acknowledgements
This work was supported by grants from National Natural Science Foundation of China (No. 81673050, 81872522), Innovation Program of Shanghai Municipal Education Commission (No. 2019-01-07-00-07-E00046), the Program of Science and Technology Commission of Shanghai Municipality (No. 18140901800), Excellent Subject Leader Program of Shanghai Municipal Commission of Health and Family Planning (No. 2018BR30), Clinical Research Program of Shanghai Hospital Development Center (No. SHDC12018X06).
References
- M. Rauma, A. Boman and G. Johanson, Adv. Drug Delivery Rev., 2013, 65, 306–314 CrossRef CAS PubMed.
- A. K. Dąbrowska, F. Spano, S. Derler, C. Adlhart, N. D. Spencer and R. M. Rossi, Skin Res. Technol., 2018, 24, 165–174 CrossRef PubMed.
- R. Wong, S. Geyer, W. Weninger, J. C. Guimberteau and J. K. Wong, Exp. Dermatol., 2016, 25, 92–98 CrossRef PubMed.
- L. Gravitz, Nature, 2018, 563(7732), S83 CrossRef CAS PubMed.
- A. Menter, A. Gottlieb, S. R. Feldman, A. S. Van Voorhees, C. L. Leonardi, K. B. Gordon, M. Lebwohl, J. Y. Koo, C. A. Elmets, N. J. Korman, K. R. Beutner and R. Bhushan, J. Am. Acad. Dermatol., 2008, 58, 826–850 CrossRef PubMed.
- M. R. Prausnitz and R. Langer, Nat. Biotechnol., 2008, 26, 1261–1268 CrossRef CAS PubMed.
- T. Waghule, G. Singhvi, S. K. Dubey, M. M. Pandey, G. Gupta, M. Singh and K. Dua, Biomed. Pharmacother., 2019, 109, 1249–1258 CrossRef CAS PubMed.
- R. F. Donnelly, T. R. Raj Singh and A. D. Woolfson, Drug Deliv, 2010, 17, 187–207 CrossRef CAS PubMed.
- S. Henry, D. V. Mcallister, M. G. Allen and M. R. Prausnitz, J. Pharm. Sci., 1998, 87, 922–925 CrossRef CAS PubMed.
- J. A. Matriano, M. Cormier, J. Johnson, W. A. Young, M. Buttery, K. Nyam and P. E. Daddona, Pharm. Res., 2002, 19, 63–70 CrossRef CAS PubMed.
- A. H. Sabri, Y. Kim, M. Marlow, D. J. Scurr, J. Segal, A. K. Banga, L. Kagan and J. B. Lee, Adv. Drug Delivery Rev., 2019 DOI:10.1016/j.addr.2019.10.004.
- G. Ma and C. Wu, J. Controlled Release, 2017, 251, 11–23 CrossRef CAS PubMed.
- J. A. Mikszta, J. B. Alarcon, J. M. Brittingham, D. E. Sutter, R. J. Pettis and N. G. Harvey, Nat. Med., 2002, 8, 415–419 CrossRef CAS PubMed.
- J. Arya and M. R. Prausnitz, J. Controlled Release, 2016, 240, 135–141 CrossRef CAS PubMed.
- W. Martanto, S. P. Davis, N. R. Holiday, J. Wang, H. S. Gill and M. R. Prausnitz, Pharm. Res., 2004, 21, 947–952 CrossRef CAS PubMed.
- K. Ita, Pharmaceutics, 2015, 7, 90–105 CrossRef CAS PubMed.
- L. Niu, L. Y. Chu, S. A. Burton, K. J. Hansen and J. Panyam, J. Controlled Release, 2019, 294, 268–278 CrossRef CAS PubMed.
- D. Resnik, M. Možek, B. Pečar, A. Janež, V. Urbančič, C. Iliescu and D. Vrtačnik, Micromachines, 2018, 9, 40 CrossRef PubMed.
- P. R. Miller, R. M. Taylor, B. Q. Tran, G. Boyd, T. Glaros, V. H. Chavez, R. Krishnakumar, A. Sinha, K. Poorey, K. P. Williams, S. S. Branda, J. T. Baca and R. Polsky, Commun. Biol., 2018, 1, 173 CrossRef PubMed.
- H. S. Lee, H. R. Ryu, J. Y. Roh and J. H. Park, Pharm. Res., 2017, 34, 101–112 CrossRef CAS PubMed.
- A. K. Shakya, R. S. J. Ingrole, G. Joshi, M. J. Uddin, S. Anvari, C. M. Davis and H. S. Gill, J. Controlled Release, 2019, 314, 38–47 CrossRef CAS PubMed.
- P. Gupta and K. S. Yadav, Life Sci., 2019, 237, 116907 CrossRef PubMed.
- C. Dillon, H. Hughes, N. J. O'Reilly and P. McLoughlin, Int. J. Pharm., 2017, 526, 125–136 CrossRef CAS PubMed.
- C. Dillon, H. Hughes, N. J. O'Reilly, C. J. Allender, D. A. Barrow and P. McLoughlin, Int. J. Pharm., 2019, 565, 9–19 CrossRef CAS PubMed.
- J. Zhu, L. Dong, H. Du, J. Mao, Y. Xie, H. Wang, J. Lan, Y. Lou, Y. Fu, J. Wen, B. Jiang, Y. Li, J. Zhu and J. Tao, Adv. Healthcare Mater., 2019, 8, e1900896 CrossRef PubMed.
- A. Buganza Tepole and E. Kuhl, Pediatr. Res., 2013, 73, 553–563 CrossRef CAS PubMed.
- C. R. France and J. L. France, Transfusion, 2018, 58, 2360–2364 CrossRef PubMed.
- A. C. Williams and B. W. Barry, Adv. Drug Delivery Rev., 2004, 56, 603–618 CrossRef CAS PubMed.
- L. S. Cook, J. Infus. Nurs., 2016, 39, 273–279 CrossRef PubMed.
- D. Brambilla, S. T. Proulx, P. Marschalkova, M. Detmar and J. C. Leroux, Small, 2016, 12, 1053–1061 CrossRef CAS PubMed.
- A. H. Sabri, J. Ogilvie, K. Abdulhamid, V. Shpadaruk, J. McKenna, J. Segal, D. J. Scurr and M. Marlow, Eur. J. Pharm. Biopharm., 2019, 140, 121–140 CrossRef PubMed.
- Y. Song, C. Xiao, R. Mendelsohn, T. Zheng, L. Strekowski and B. Michniak, Pharm. Res., 2005, 22, 1918–1925 CrossRef CAS PubMed.
- A. Tfayli, O. Piot, F. Pitre and M. Manfait, Eur. Biophys. J., 2007, 36, 1049–1058 CrossRef CAS PubMed.
- H. L. Richards, D. G. Fortune, T. M. O'Sullivan, C. J. Main and C. E. M. Griffiths, J. Am. Acad. Dermatol., 1999, 41, 581–583 CAS.
- J. Murphy and G. Coster, Drugs, 1997, 54(6), 797–800 CrossRef CAS PubMed.
- B. J. Thomas and B. C. Finnin, Drug Discovery Today, 2004, 9, 697–703 CrossRef CAS.
- J. Mönkäre, M. R. Nejadnik, K. Baccouche, S. Romeijn, W. Jiskoot and J. A. Bouwstra, J. Controlled Release, 2015, 218, 53–62 CrossRef PubMed.
- J. W. Lee, S. O. Choi, E. I. Felner and M. R. Prausnitz, Small, 2011, 7, 531–539 CrossRef CAS PubMed.
- E. E. Peters, M. Ameri, X. Wang, Y.-F. Maa and P. E. Daddona, Pharm. Res., 2012, 29, 1618–1626 CrossRef CAS PubMed.
- P. Zhang and G. Jullien, The 2005 international conference on MEMS, NANO, and smart systems, Banff, Canada, 2005, pp. 392–395 Search PubMed.
- K. Mooney, J. C. McElnay and R. F. Donnelly, Int. J. Pharm. Pract., 2014, 22, 335–344 CrossRef PubMed.
- P. P. Samant and M. R. Prausnitz, Proc. Natl. Acad. Sci. U. S. A., 2018, 115, 4583–4588 CrossRef PubMed.
- P. de la Cueva Dobao, J. Notario, C. Ferrandiz, J. L. Lopez Estebaranz, I. Alarcon, S. Sulleiro, J. Borras, E. Dauden, J. M. Carrascosa, J. L. Sanchez Carazo, E. Monte Boquet and L. Puig, J. Eur. Acad. Dermatol. Venereol., 2019, 33, 1214–1223 CrossRef CAS PubMed.
- H. Marwah, T. Garg, A. K. Goyal and G. Rath, Drug Deliv, 2016, 23, 564–578 CrossRef CAS PubMed.
- V. Vemulapalli, Y. Yang, P. M. Friden and A. K. Banga, J. Pharm. Pharmacol., 2008, 60, 27–33 CrossRef CAS PubMed.
- H. X. Nguyen and A. K. Banga, Pharm. Res., 2018, 35, 68 CrossRef PubMed.
- H. Du, P. Liu, J. Zhu, J. Lan, Y. Li, L. Zhang, J. Zhu and J. Tao, ACS Appl. Mater. Inter., 2019, 11(46), 43588–43598 CrossRef CAS PubMed.
- J. Kristof, H. Miyamoto, A. N. Tran, M. Blajan and K. Shimizu, Biointerphases, 2017, 12, 02B402 CrossRef PubMed.
- J. W. So, H. H. Park, S. S. Lee, D. C. Kim, S. C. Shin and C. W. Cho, Drug Deliv, 2009, 16, 52–56 CrossRef CAS PubMed.
- H. R. Jeong, J. Y. Kim, S. N. Kim and J. H. Park, Eur. J. Pharm. Biopharm., 2018, 127, 237–243 CrossRef CAS PubMed.
- K. S. Paudel, M. Milewski, C. L. Swadley, N. K. Brogden, P. Ghosh and A. L. Stinchcomb, Therapeutic Deliv, 2010, 1, 109–131 CrossRef CAS PubMed.
- E. Korkmaz, E. E. Friedrich, M. H. Ramadan, G. Erdos, A. R. Mathers, O. Burak Ozdoganlar, N. R. Washburn and L. D. Falo, Acta Biomater., 2015, 24, 96–105 CrossRef CAS PubMed.
- E. Korkmaz, E. E. Friedrich, M. H. Ramadan, G. Erdos, A. R. Mathers, O. B. Ozdoganlar, N. R. Washburn and L. D. Falo, J. Pharm. Sci., 2016, 105, 3453–3457 CrossRef CAS PubMed.
- J. H. Lee, Y. S. Jung, G. M. Kim and J. M. Bae, Br. J. Dermatol., 2018, 178, e24–e25 CAS.
- C. Wang, Y. Ye, G. M. Hochu, H. Sadeghifar and Z. Gu, Nano Lett., 2016, 16, 2334–2340 CrossRef CAS PubMed.
- H. P. Tham, K. Xu, W. Q. Lim, H. Chen, M. Zheng, T. G. S. Thng, S. S. Venkatraman, C. Xu and Y. Zhao, ACS Nano, 2018, 12, 11936–11948 CrossRef CAS PubMed.
- A. K. Jain, C. H. Lee and H. S. Gill, J. Controlled Release, 2016, 239, 72–81 CrossRef CAS PubMed.
- H. T. T. Duong, Y. Yin, T. Thambi, T. L. Nguyen, V. H. Giang Phan, M. S. Lee, J. E. Lee, J. Kim, J. H. Jeong and D. S. Lee, Biomaterials, 2018, 185, 13–24 CrossRef CAS PubMed.
- R. Paleco, S. R. Vučen, A. M. Crean, A. Moore and S. Scalia, Int. J. Pharm., 2014, 472, 206–213 CrossRef CAS PubMed.
- J. H. Kim, J. U. Shin, S. H. Kim, J. Y. Noh, H. R. Kim, J. Lee, H. Chu, K. Y. Jeong, K. H. Park, J. D. Kim, H. K. Kim, D. H. Jeong, T. S. Yong, J. W. Park and K. H. Lee, Biomaterials, 2018, 150, 38–48 CrossRef CAS PubMed.
|
This journal is © The Royal Society of Chemistry 2020 |