DOI:
10.1039/D0RA00643B
(Paper)
RSC Adv., 2020,
10, 12135-12144
6,N2-Diaryl-1,3,5-triazine-2,4-diamines: synthesis, antiproliferative activity and 3D-QSAR modeling†
Received
21st January 2020
, Accepted 13th March 2020
First published on 25th March 2020
Abstract
A library of 126 compounds with a 6,N2-diaryl-1,3,5-triazine-2,4-diamine scaffold was prepared using a one-pot, microwave-assisted method from readily available cyanoguanidine, aromatic aldehydes and arylamines. The three-component condensation of these reagents in the presence of hydrochloric acid was followed by the treatment with a base, which promoted a rearrangement of the dihydrotriazine ring and its dehydrogenative aromatization. The antiproliferative properties of the prepared compounds were evaluated using three breast cancer cell lines. The most promising results were obtained in the growth inhibition of the triple negative MDA-MB231 breast cancer cells. The active compounds were also selective against cancer cells and did not affect growth of the non-cancerous MCF-10A breast cell line. Analyzing the structure–activity relationship within the series, we built a 3D-QSAR model for the further design of more potent anticancer compounds.
Introduction
The 1,3,5-triazine ring has been extensively explored as a scaffold for the design and construction of molecules with therapeutically useful properties.1 Particular advancements were made in the area of the anticancer agent development.2 Several 1,3,5-triazine based agents have been used for the treatment of various types of cancer, from earlier developed alkylating agents tretamine3,4 and altretamine5 to nucleic acid targeting nucleosides azacitidine6 and decitabine,7,8 and a recently approved inhibitor of isocitrate dehydrogenase 2, enasidenib9,10 (Fig. 1). An intensive exploration of the 1,3,5-triazine scaffold has continued and a number of notable anticancer 1,3,5-triazines have been recently developed, including those undergoing clinical trials gedatolisib inhibiting PI3K and mTOR kinases,11,12 PAK4 inhibitor KY-04031 effective against prostate cancer,13 and HL010183 particularly effective in the inhibition of proliferation and invasion of triple-negative breast cancer cells.14,15
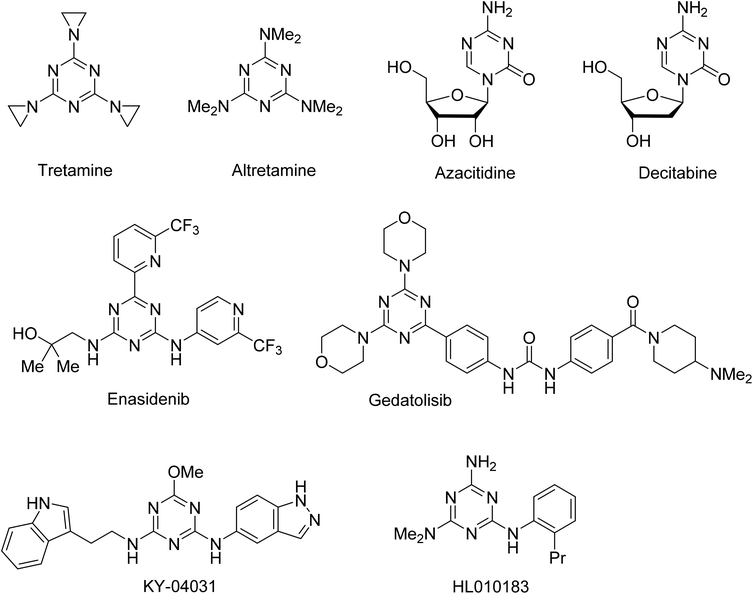 |
| Fig. 1 Selected anticancer 1,3,5-triazines. | |
Recently we reported a new effective synthesis of a library of 6,N2-diaryl-1,3,5-triazine-2,4-diamines, some of which demonstrated promising anticancer properties in preliminary assessment on the DU145 prostate cancer cell line.16 Inspired by these results, we further expanded the initial library to 126 compounds and performed antiproliferative screening of these compounds on three types of breast cancer cell lines. Herein, we report result of this work and attempt to build a QSAR model for the further design of more active compounds.
Results and discussion
Synthesis
The microwave-assisted synthesis has been recognized as a highly valuable approach for the synthesis of 1,3,5-triazines.17 We applied focused microwave irradiation for the synthesis of 6,N2-diaryl-1,3,5-triazine-2,4-diamines using a recently developed one-pot method.16 Initially, a three-component reaction of cyanoguanidine, aromatic aldehydes and arylamines was carried out in the presence of hydrochloric acid under microwave irradiation. Without isolation, intermediates I were treated with a base to give products of the Dimroth rearrangement II, which, at the reaction conditions, underwent a spontaneous dehydrogenation and aromatization affording desired 6,N2-diaryl-1,3,5-triazine-2,4-diamines (1–126) (Scheme 1).
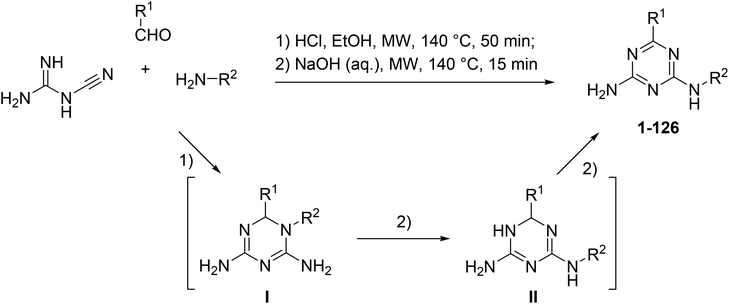 |
| Scheme 1 Synthesis of 6,N2-diaryl-1,3,5-triazine-2,4-diamines (1–126). | |
The developed protocol for the synthesis of 6,N2-diaryl-1,3,5-triazine-2,4-diamines (1–126) was rather general and convenient for the generation of libraries covering a sufficiently broad chemical space for the biological screening.
Cytotoxic evaluation
The prepared compounds 1–126 were tested on three breast cancer cell lines namely, MDA-MB231, SKBR-3 and MCF-7 using MTT assay. SKBR-3 and MCF-7 cell lines are estrogen and progesterone hormone positive cell lines often used as a model for the hormone therapy.18 MDA-MB231 is triple negative breast cancer cell line, which is negative to estrogen and progesterone receptors and human epidermal growth factor receptor 2, a perfect model for chemotherapy.18 Initially, all the prepared compounds 1–126 were tested on these three cancer cell lines at the screening concentration (10 μM) and percentage of cell viability was calculated 72 h after the treatment (Table 1).
Table 1 Antiproliferative screening of 6,N2-diaryl-1,3,5-triazine-2,4-diamines (1–126) at 10 μM
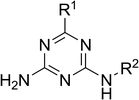
|
Compd |
R1 |
R2 |
Percentage of cell viabilitya |
MDA-MB231 |
SKBR-3 |
MCF-7 |
Mean of three independent experiments. |
1 |
Ph |
Ph |
87 |
81 |
90 |
2 |
Ph |
2-FC6H4 |
82 |
83 |
99 |
3 |
Ph |
4-FC6H4 |
81 |
84 |
100 |
4 |
Ph |
2-ClC6H4 |
72 |
88 |
81 |
5 |
Ph |
4-ClC6H4 |
65 |
94 |
100 |
6 |
Ph |
4-BrC6H4 |
65 |
97 |
100 |
7 |
Ph |
4-MeC6H4 |
98 |
87 |
87 |
8 |
Ph |
2-MeOC6H4 |
78 |
97 |
91 |
9 |
Ph |
4-MeOC6H4 |
98 |
96 |
84 |
10 |
Ph |
4-CF3OC6H4 |
96 |
86 |
92 |
11 |
Ph |
4-iPrC6H4 |
99 |
86 |
99 |
12 |
Ph |
3-Pyridyl |
59 |
78 |
87 |
13 |
3-FC6H4 |
Ph |
100 |
100 |
89 |
14 |
3-FC6H4 |
4-FC6H4 |
50 |
100 |
78 |
15 |
3-FC6H4 |
4-ClC6H4 |
100 |
100 |
89 |
16 |
3-FC6H4 |
4-BrC6H4 |
43 |
89 |
80 |
17 |
3-FC6H4 |
4-MeC6H4 |
29 |
90 |
71 |
18 |
3-FC6H4 |
4-MeOC6H4 |
46 |
74 |
76 |
19 |
3-FC6H4 |
4-CF3OC6H4 |
50 |
100 |
76 |
20 |
3-FC6H4 |
4-iPrC6H4 |
100 |
100 |
92 |
21 |
4-FC6H4 |
Ph |
83 |
94 |
100 |
22 |
4-FC6H4 |
2-FC6H4 |
83 |
85 |
100 |
23 |
4-FC6H4 |
4-FC6H4 |
81 |
100 |
93 |
24 |
4-FC6H4 |
2-ClC6H4 |
75 |
75 |
91 |
25 |
4-FC6H4 |
3-ClC6H4 |
73 |
88 |
95 |
26 |
4-FC6H4 |
4-ClC6H4 |
67 |
98 |
87 |
27 |
4-FC6H4 |
4-BrC6H4 |
96 |
100 |
94 |
28 |
4-FC6H4 |
4-MeC6H4 |
99 |
90 |
88 |
29 |
4-FC6H4 |
2-MeOC6H4 |
60 |
81 |
96 |
30 |
4-FC6H4 |
4-MeOC6H4 |
74 |
90 |
92 |
31 |
4-FC6H4 |
4-CF3OC6H4 |
88 |
92 |
87 |
32 |
4-FC6H4 |
4-iPrC6H4 |
94 |
100 |
84 |
33 |
4-FC6H4 |
3-Pyridyl |
74 |
87 |
85 |
34 |
4-ClC6H4 |
Ph |
81 |
100 |
100 |
35 |
4-ClC6H4 |
2-FC6H4 |
82 |
99 |
93 |
36 |
4-ClC6H4 |
4-FC6H4 |
49 |
98 |
100 |
37 |
4-ClC6H4 |
2-ClC6H4 |
99 |
89 |
99 |
38 |
4-ClC6H4 |
3-ClC6H4 |
99 |
92 |
84 |
39 |
4-ClC6H4 |
4-ClC6H4 |
97 |
100 |
100 |
40 |
4-ClC6H4 |
4-BrC6H4 |
80 |
100 |
92 |
41 |
4-ClC6H4 |
2-MeOC6H4 |
83 |
89 |
100 |
42 |
4-ClC6H4 |
4-MeOC6H4 |
61 |
90 |
85 |
43 |
4-ClC6H4 |
4-CF3OC6H4 |
93 |
83 |
100 |
44 |
4-ClC6H4 |
4-iPrC6H4 |
100 |
97 |
96 |
45 |
4-ClC6H4 |
3-Pyridyl |
99 |
74 |
89 |
46 |
4-BrC6H4 |
2-FC6H4 |
75 |
91 |
100 |
47 |
4-BrC6H4 |
4-FC6H4 |
58 |
98 |
100 |
48 |
4-BrC6H4 |
2-ClC6H4 |
90 |
93 |
100 |
49 |
4-BrC6H4 |
4-ClC6H4 |
96 |
100 |
100 |
50 |
4-BrC6H4 |
4-MeC6H4 |
55 |
93 |
88 |
51 |
4-BrC6H4 |
2-MeOC6H4 |
88 |
89 |
96 |
52 |
4-BrC6H4 |
4-MeOC6H4 |
56 |
91 |
100 |
53 |
3-MeC6H4 |
4-FC6H4 |
100 |
100 |
78 |
54 |
3-MeC6H4 |
4-ClC6H4 |
100 |
93 |
76 |
55 |
3-MeC6H4 |
4-BrC6H4 |
83 |
96 |
75 |
56 |
3-MeC6H4 |
4-MeC6H4 |
12 |
62 |
67 |
57 |
3-MeC6H4 |
4-MeOC6H4 |
91 |
90 |
75 |
58 |
3-MeC6H4 |
4-CF3OC6H4 |
50 |
100 |
73 |
59 |
3-MeC6H4 |
4-iPrC6H4 |
87 |
93 |
76 |
60 |
4-MeC6H4 |
Ph |
84 |
100 |
100 |
61 |
4-MeC6H4 |
2-FC6H4 |
50 |
79 |
73 |
62 |
4-MeC6H4 |
4-FC6H4 |
48 |
78 |
100 |
63 |
4-MeC6H4 |
4-BrC6H4 |
87 |
100 |
93 |
64 |
4-MeC6H4 |
4-MeC6H4 |
59 |
92 |
86 |
65 |
4-MeC6H4 |
2-MeOC6H4 |
97 |
88 |
87 |
66 |
4-MeC6H4 |
4-MeOC6H4 |
83 |
89 |
100 |
67 |
4-MeC6H4 |
4-CF3OC6H4 |
92 |
84 |
93 |
68 |
4-MeC6H4 |
4-iPrC6H4 |
76 |
91 |
100 |
69 |
4-MeC6H4 |
3-Pyridyl |
74 |
79 |
88 |
70 |
4-MeOC6H4 |
2-FC6H4 |
9 |
46 |
63 |
71 |
4-MeOC6H4 |
4-MeOC6H4 |
56 |
93 |
95 |
72 |
4-MeOC6H4 |
4-FC6H4 |
68 |
100 |
100 |
73 |
4-MeOC6H4 |
2-ClC6H4 |
10 |
47 |
84 |
74 |
4-MeOC6H4 |
3-ClC6H4 |
22 |
45 |
86 |
75 |
4-MeOC6H4 |
4-ClC6H4 |
99 |
100 |
100 |
76 |
4-MeOC6H4 |
4-BrC6H4 |
96 |
100 |
91 |
77 |
4-MeOC6H4 |
3-MeC6H4 |
22 |
40 |
79 |
78 |
4-MeOC6H4 |
2-MeOC6H4 |
50 |
66 |
57 |
79 |
4-MeOC6H4 |
4-CF3OC6H4 |
74 |
84 |
95 |
80 |
4-MeOC6H4 |
4-iPrC6H4 |
92 |
86 |
100 |
81 |
4-MeOC6H4 |
3-Pyridyl |
46 |
77 |
91 |
82 |
4-CF3C6H4 |
Ph |
55 |
97 |
96 |
83 |
4-CF3C6H4 |
2-FC6H4 |
55 |
71 |
93 |
84 |
4-CF3C6H4 |
4-FC6H4 |
95 |
98 |
100 |
85 |
4-CF3C6H4 |
4-ClC6H4 |
90 |
77 |
99 |
86 |
4-CF3C6H4 |
4-MeC6H4 |
54 |
95 |
83 |
87 |
4-CF3C6H4 |
2-MeOC6H4 |
63 |
85 |
100 |
88 |
4-CF3C6H4 |
4-MeOC6H4 |
81 |
86 |
100 |
89 |
4-CF3OC6H4 |
Ph |
59 |
90 |
95 |
90 |
4-CF3OC6H4 |
2-FC6H4 |
60 |
80 |
90 |
91 |
4-CF3OC6H4 |
4-FC6H4 |
53 |
86 |
100 |
92 |
4-CF3OC6H4 |
2-ClC6H4 |
74 |
86 |
96 |
93 |
4-CF3OC6H4 |
4-BrC6H4 |
83 |
96 |
90 |
94 |
4-CF3OC6H4 |
4-MeC6H4 |
79 |
87 |
97 |
95 |
4-CF3OC6H4 |
2-MeOC6H4 |
46 |
81 |
88 |
96 |
4-CF3OC6H4 |
4-MeOC6H4 |
69 |
88 |
87 |
97 |
4-CF3OC6H4 |
4-CF3OC6H4 |
92 |
80 |
100 |
98 |
4-CF3OC6H4 |
4-iPrC6H4 |
100 |
90 |
95 |
99 |
4-CF3OC6H4 |
3-Pyridyl |
33 |
81 |
85 |
100 |
4-Me2NC6H4 |
Ph |
21 |
61 |
80 |
101 |
4-Me2NC6H4 |
2-FC6H4 |
28 |
51 |
88 |
102 |
4-Me2NC6H4 |
4-FC6H4 |
32 |
88 |
90 |
103 |
4-Me2NC6H4 |
2-MeOC6H4 |
28 |
48 |
88 |
104 |
4-Me2NC6H4 |
4-iPrC6H4 |
70 |
87 |
100 |
105 |
4-tBuC6H4 |
Ph |
74 |
100 |
96 |
106 |
4-tBuC6H4 |
4-FC6H4 |
97 |
84 |
88 |
107 |
4-tBuC6H4 |
4-ClC6H4 |
100 |
92 |
98 |
108 |
4-tBuC6H4 |
4-BrC6H4 |
59 |
98 |
99 |
109 |
4-tBuC6H4 |
4-MeOC6H4 |
91 |
85 |
93 |
110 |
4-BnOC6H4 |
Ph |
51 |
75 |
100 |
111 |
4-BnOC6H4 |
4-FC6H4 |
69 |
84 |
89 |
112 |
4-BnOC6H4 |
4-BrC6H4 |
82 |
93 |
97 |
113 |
4-BnOC6H4 |
4-MeC6H4 |
82 |
94 |
100 |
114 |
4-BnOC6H4 |
4-CF3OC6H4 |
71 |
87 |
100 |
115 |
2-Thienyl |
Ph |
100 |
87 |
91 |
116 |
2-Thienyl |
2-FC6H4 |
98 |
78 |
90 |
117 |
2-Thienyl |
4-FC6H4 |
100 |
86 |
92 |
118 |
2-Thienyl |
2-ClC6H4 |
100 |
78 |
91 |
119 |
2-Thienyl |
4-ClC6H4 |
100 |
89 |
98 |
120 |
2-Thienyl |
4-BrC6H4 |
46 |
87 |
88 |
121 |
2-Thienyl |
4-MeC6H4 |
49 |
95 |
97 |
122 |
2-Thienyl |
2-MeOC6H4 |
78 |
97 |
91 |
123 |
2-Thienyl |
4-MeOC6H4 |
93 |
88 |
80 |
124 |
2-Thienyl |
4-CF3OC6H4 |
100 |
86 |
85 |
125 |
2-Thienyl |
4-iPrC6H4 |
100 |
86 |
89 |
126 |
2-Thienyl |
3-Pyridyl |
100 |
91 |
86 |
The prepared 6,N2-diaryl-1,3,5-triazine-2,4-diamines selectively inhibited the triple negative breast cancer cells. It was observed that hormone independent cell line (MDA-MB231) was generally more sensitive to the treatment with 6,N2-diaryl-1,3,5-triazine-2,4-diamines, whereas hormone dependent cancer cell lines (SKBR-3 and MCF-7) were more resistant to the treatment with these compounds.
Compounds reducing the growth of the MDA-MB231 cancer cells (at concentration 10 μM) to 50% or less were selected for the evaluation of their 50% growth inhibitory concentrations (GI50). The growth inhibitory effect of the compounds was determined on breast tumor cell line (MDA-MB231, SKBR-3 and MCF-7) at different concentrations with methotrexate and nilotinib as positive controls (Table 2). All the tested compounds were more effective towards the MDA-MB231 cancer cell line than SKBR-3 and MCF-7 cells.
Table 2 Inhibition of cell growth by compounds selected after the initial screening
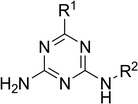
|
Compound |
R1 |
R2 |
GI50a (μM) ± SEMb |
MDA-MB231 |
SKBR-3 |
MCF-7 |
MCF-10A |
Concentration (μM) required to inhibit tumor cell growth by 50%, values are mean of three independent experiments. Standard error of the mean. Positive controls, ND = not determined. |
14 |
3-FC6H4 |
4-FC6H4 |
9.21 ± 0.38 |
>20 |
>20 |
>25 |
16 |
3-FC6H4 |
4-BrC6H4 |
13.13 ± 0.91 |
17.16 ± 1.07 |
>20 |
>25 |
17 |
3-FC6H4 |
4-MeC6H4 |
3.96 ± 0.17 |
>20 |
>20 |
>25 |
18 |
3-FC6H4 |
4-MeOC6H4 |
6.18 ± 0.43 |
16.63 ± 1.38 |
>20 |
>25 |
19 |
3-FC6H4 |
4-CF3OC6H4 |
8.56 ± 0.59 |
>20 |
18.22 ± 1.28 |
>25 |
36 |
4-ClC6H4 |
4-FC6H4 |
14.14 ± 1.52 |
19.40 ± 2.39 |
>20 |
>25 |
56 |
3-MeC6H4 |
4-MeC6H4 |
0.17 ± 0.02 |
1.26 ± 0.18 |
>20 |
>25 |
58 |
3-MeC6H4 |
4-CF3OC6H4 |
15.24 ± 1.01 |
>20 |
>20 |
>25 |
61 |
4-MeC6H4 |
2-FC6H4 |
12.25 ± 1.15 |
>20 |
>20 |
>25 |
62 |
4-MeC6H4 |
4-FC6H4 |
10.68 ± 0.73 |
>20 |
20.15 ± 1.95 |
>25 |
70 |
4-MeOC6H4 |
2-FC6H4 |
0.32 ± 0.04 |
>20 |
>20 |
>25 |
73 |
4-MeOC6H4 |
2-ClC6H4 |
0.23 ± 0.03 |
1.10 ± 0.01 |
>20 |
>25 |
74 |
4-MeOC6H4 |
3-ClC6H4 |
1.33 ± 0.17 |
0.18 ± 0.04 |
18.30 ± 1.11 |
>25 |
77 |
4-MeOC6H4 |
3-MeC6H4 |
0.95 ± 0.04 |
3.38 ± 0.36 |
>20 |
>25 |
78 |
4-MeOC6H4 |
2-MeOC6H4 |
13.25 ± 0.93 |
2.15 ± 0.12 |
>20 |
>25 |
81 |
4-MeOC6H4 |
3-Pyridyl |
14.01 ± 1.33 |
15.58 ± 1.06 |
>20 |
>25 |
95 |
4-CF3OC6H4 |
2-MeOC6H4 |
12.77 ± 0.76 |
>20 |
>20 |
>25 |
99 |
4-CF3OC6H4 |
3-Pyridyl |
11.52 ± 1.51 |
>20 |
>20 |
>25 |
100 |
4-Me2NC6H4 |
Ph |
0.36 ± 0.07 |
4.19 ± 0.37 |
>20 |
>25 |
101 |
4-Me2NC6H4 |
2-FC6H4 |
0.06 ± 0.001 |
0.29 ± 0.04 |
>20 |
>25 |
102 |
4-Me2NC6H4 |
4-FC6H4 |
7.20 ± 0.94 |
>20 |
>20 |
>25 |
103 |
4-Me2NC6H4 |
2-MeOC6H4 |
4.17 ± 0.33 |
3.63 ± 0.23 |
>20 |
>25 |
110 |
4-BnOC6H4 |
Ph |
13.44 ± 1.18 |
>20 |
>20 |
>25 |
120 |
2-Thienyl |
4-BrC6H4 |
11.73 ± 1.39 |
>20 |
>20 |
>25 |
121 |
2-Thienyl |
4-MeC6H4 |
13.88 ± 1.74 |
>20 |
>20 |
>25 |
Methotrexatec |
|
|
0.01 ± 0.001 |
ND |
5.79 ± 0.47 |
ND |
Nilotinibc |
|
|
0.04 ± 0.001 |
9.60 ± 0.51 |
ND |
ND |
Analysis of the structure–activity relationship identified a pattern in types and combinations of R1 and R2 groups associated with the antiproliferative effect. In general, replacement of a phenyl at R1 with the 2-thienyl moiety or a phenyl at R2 with the 3-pyridyl ring demonstrated only slight or no improvement in the cell growth inhibition. Analyzing effect of the substituent at the phenyl rings, we found that antiproliferative properties typically required +M electron-donating groups in para-position of the R1 phenyl ring or a meta-substitution in the same ring. The tolerance of the activity to the R2 substituents depended on the type of the R1 substitution. The combination of a para-substituted phenyl as R1 with the para-substituted phenyl as R2 was detrimental for the activity (except R2 = 4-FC6H4). However, the antiproliferative effect of compounds with a meta-substituted phenyl as R1 was less sensitive to the position of substituents in the R2 moiety. These compounds retained good antiproliferative effect with the para-substituted phenyl as the R2 group. The most efficient for the antiproliferative activity against MDA-MB231 cells were combinations of para-methoxy or para-dimethylamino groups in the R1 phenyl ring and ortho-fluoro- or ortho-chlorophenyl group as R2 (compounds 70, 73, and 101). These compounds were also very active against SKBR-3 cells. Moreover, compound 73 was equipotent in the inhibition of MDA-MB231 and SKBR-3 cell growth. Compound 101 was identified as the most active among the tested compounds in its cytotoxic effect against MDA-MB231 cells with GI50 value of 0.06 μM.
All the compounds, selected for determination of GI50 values against MDA-MB231 cells, were also used for the experiments with MCF-10A normal breast cells. None of the tested compounds applied at concentration of 25 μM showed significant inhibition of the normal breast cell growth. These results indicated that the tested compounds were selectively active towards the breast cancer cells without any substantial effects on the normal breast cells.
3D-QSAR study
To comprehend the structural requirement controlling the antiproliferative activity, a 3D-QSAR model was built applying 3D-QSAR protocol of Discovery Studio v18 (ref. 19) to the experimentally obtained biological data. Twenty-five compounds, having GI50 values against MDA-MB231 breast cancer cell line in the range of 0.06 μM to 15.24 μM, were selected as model data set. The GI50 values of the compounds were converted into corresponding pGI50 values (−log[GI50]) using ‘Prepare dependent values’ protocol in Discovery Studio. The compounds were initially aligned to the minimum energy and then randomly divided into training set (∼80%) and test set (∼20%) by ‘Diverse molecule’ method in Discovery Studio.
The QSAR model was built using ‘Create 3D-QSAR model’ protocol in Discovery Studio. The correlation coefficient r2 between the observed and predicted pGI50 values of the training set was found to be 0.81 proving acceptability of the built QSAR model. RMSE residual error was found to be 0.31 indicating a good ability of the built model to predict the GI50 values. The r2 value > 0.5 and RMSE residual error < 0.5 were considered to represent good model.20,21 Graphically, the model predictive potential is represented by the plot of the experimental pGI50 versus predicted pGI50 values (Fig. 2). The pGI50 values predicted by this QSAR model and the residual errors for all 25 compounds are presented in Table 3.
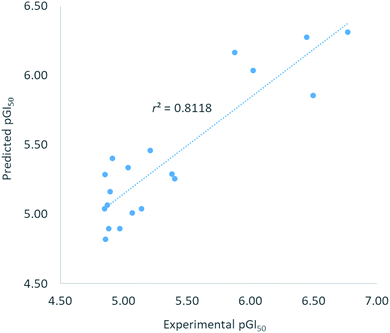 |
| Fig. 2 Plot of experimental versus predicted pGI50 activity of training set. | |
Table 3 Experimental and predicted by 3D-QSAR model inhibitory activities of compoundsa
Compound |
Experimental pGI50 |
Predicted pGI50 |
Residual error |
Underlined compounds were randomly selected for test set. |
14 |
5.04 |
5.33 |
−0.30 |
16 |
4.88 |
4.90 |
−0.01 |
17 |
5.40 |
5.26 |
0.15 |
18 |
5.21 |
5.46 |
−0.25 |
19 |
5.07 |
5.01 |
0.06 |
36 |
4.85 |
5.04 |
−0.19 |
56 |
6.77 |
6.31 |
0.46 |
 |
4.82 |
5.23 |
−0.41 |
61 |
4.91 |
5.40 |
−0.49 |
62 |
4.97 |
4.89 |
0.08 |
70 |
6.49 |
5.85 |
0.64 |
 |
6.64 |
6.10 |
0.54 |
74 |
5.88 |
6.16 |
−0.29 |
77 |
6.02 |
6.03 |
−0.01 |
 |
4.88 |
5.78 |
−0.90 |
71 |
4.85 |
5.29 |
−0.43 |
95 |
4.89 |
5.16 |
−0.27 |
 |
4.94 |
4.28 |
0.66 |
100 |
6.44 |
6.27 |
0.17 |
 |
7.22 |
6.55 |
0.67 |
102 |
5.14 |
5.04 |
0.10 |
103 |
5.38 |
5.29 |
0.09 |
110 |
4.87 |
5.07 |
−0.20 |
 |
4.93 |
4.46 |
0.47 |
121 |
4.86 |
4.82 |
0.04 |
The molecules aligned to the iso-surface of 3D-QSAR model on electrostatic potential grid and van der Waals grid are shown in Fig. 3. Red colour in the electrostatic grid (Fig. 3A) symbolizes that an increase in electron density in this region should increase the activity, while blue colour represents area where lower electron density is expected to be beneficial for the activity. Likewise, green contour in steric map (Fig. 3B) indicates a potential increase in the activity with sterically bulky groups in these regions, while yellow contour shows areas where an increase in the steric bulk would result in a lower activity.21
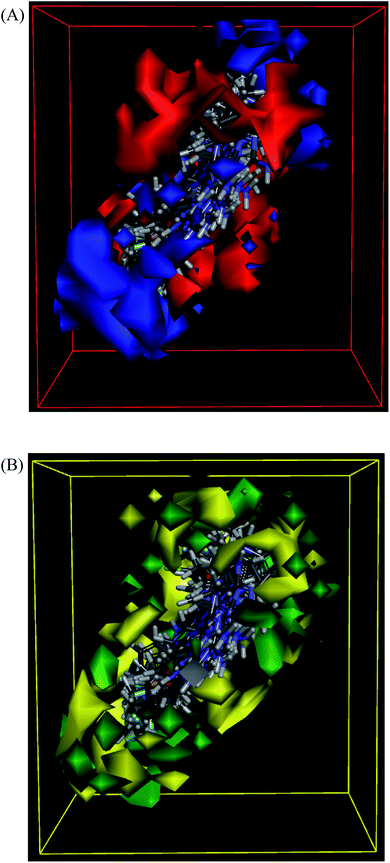 |
| Fig. 3 3D-QSAR model coefficients on electrostatic grid (A) and van der Waals grid (B). | |
The 3D-QSAR map suggested that compounds bearing a high electron density and bulky group at R1 position would show higher activity. Despite the high residual error (0.69) observed for compound 101, this 3D-QSAR map validated its highest activity as the dimethylamino group at the R1 phenyl ring clearly met the above description, particularly on the electrostatic grid. The good antiproliferative activity of compounds bearing a para-methoxy group at the R1 phenyl was also well aligned with the model. The positioning of the most active in the series compound 101 (GI50 = 0.06 μM) in the electrostatic and van der Waals grids is shown in Fig. 4.
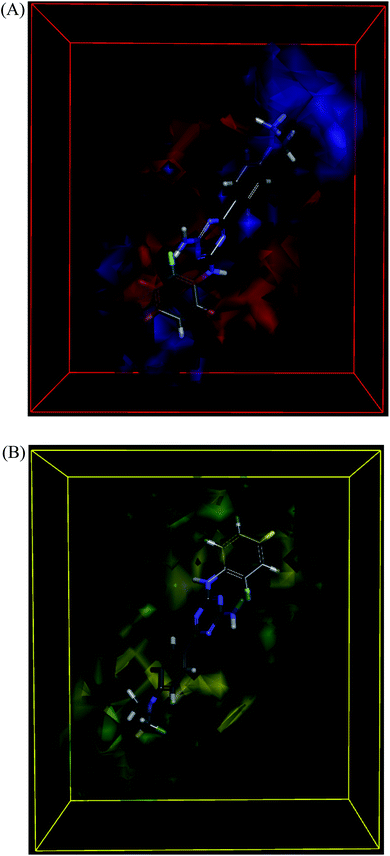 |
| Fig. 4 3D-QSAR model coefficients of compound 101 on electrostatic grid (A) and van der Waals grid (B). | |
Conclusions
We synthesized a library of 6,N2-diaryl-1,3,5-triazine-2,4-diamines and evaluated their antiproliferative properties against three breast cancer cell lines. It was found that MDA-MB231 triple negative breast cancer cells were more sensitive to the prepared compounds than SKBR-3 and MCF-7 cells. The active compounds also demonstrated no inhibition on the growth of non-cancerous MCF-10A breast cells. The 3D-QSAR model constructed using the obtained data could be used for the further design of compoundstargeting triple negative breast cancer cells.
Experimental
General
Melting points (uncorrected) were determined on a Stuart™ SMP40 automatic melting point apparatus. 1H and 13C NMR spectra were recorded on a Bruker Fourier NMR spectrometer (300 MHz) using DMSO-d6 as a solvent and TMS as an internal reference. Microwave-assisted reactions were carried out in the closed vessel focused single mode using a Discover SP microwave synthesizer (CEM, USA) monitoring reaction temperature by the equipped IR sensor. The synthesis of compounds 1, 5–29, 31–33, 35–52, 54–56, 58, 60–64, 66–70, 72–83, 85–90, 92–110, 112–115, 117–121, 124–126 and their characterization were described earlier.16
General method for the synthesis of 6,N2-diaryl-1,3,5-triazine-2,4-diamines (1–126)
To a solution of cyanoguanidine (0.21 g, 2.5 mmol), an aromatic aldehyde (2.5 mmol) and an arylamine (2.5 mmol) in EtOH (2 mL) in a 10 mL seamless pressure vial, conc. HCl (0.21 mL, 2.5 mmol) was added. The reaction mixture was heated at 140 °C for 50 min by irradiation in the Discover SP (CEM) microwave reactor operating at maximal microwave power up to 150 W. Then, an aq. solution of NaOH (5 N, 1 mL) was added to the reaction mixture and heating was continued for another 15 min at 140 °C. After cooling, the precipitated product was filtered, washed with water and recrystallized from a suitable solvent.
N2-(2-Fluorophenyl)-6-phenyl-1,3,5-triazine-2,4-diamine (2)
Yield 0.12 g, 30%. Mp 156–158 °C (EtOH). 1H NMR (300 MHz, DMSO-d6): δ 7.08 (2H, brs, NH2), 7.17–7.30 (3H, m, H-3′′, H-4′′ and H-5′′), 7.46–7.54 (3H, m, H-3′, H-4′ and H-5′), 7.75–7.81 (1H, m, H-6′′), 8.28 (2H, dd, J = 1.6 Hz, J = 8.1 Hz, H-2′ and H-6′), 9.02 (1H, s, NH); 13C NMR (75 MHz, DMSO-d6): δ 115.5 (d, 2JCF = 19.4 Hz, C-3′′), 124.0 (d, 3JCF = 3.7 Hz, C-6′′), 125.5 (d, 3JCF = 7.5 Hz, C-4′′), 126.6 (d, 4JCF = 1.5 Hz, C-5′′), 126.7 (d, 2JCF = 12.0 Hz, C-1′′), 127.7 (C-3′ and C-5′), 128.2 (C-2′ and C-6′), 131.3 (C-4′), 136.6 (C-1′), 155.4 (d, 1JCF = 245.9 Hz, C-2′′), 165.2 (C-4), 167.3 (C-6), 170.2 (C-2). Anal. calcd for C15H12FN5: C, 64.05; H, 4.30; N, 24.90. Found: C, 63.96; H, 4.41; N, 24.77.
N2-(4-Fluorophenyl)-6-phenyl-1,3,5-triazine-2,4-diamine (3)
Yield 0.25 g, 37%. Mp 172–174 °C (EtOH). 1H NMR (300 MHz, DMSO-d6): δ 7.13 (2H, brs, NH2), 7.15 (2H, dd, 3JHF = 8.8 Hz, 3JHH = 9.2 Hz, H-3′′ and H-5′′), 7.48–7.56 (3H, m, H-3′, H-4′ and H-5′), 7.85 (2H, dd, 4JHF = 5.0 Hz, 3JHH = 9.2 Hz, H-2′′ and H-6′′), 8.32 (2H, dd, J = 1.7 Hz, J = 8.1 Hz, H-2′ and H-6′), 9.57 (1H, s, NH); 13C NMR (75 MHz, DMSO-d6): δ 114.9 (d, 2JCF = 22.0 Hz, C-3′′ and C-5′′), 121.6 (d, 3JCF = 8.2 Hz, C-2′′ and C-6′′), 127.7 (C-3′ and C-5′), 128.2 (C-2′ and C-6′), 131.3 (C-4′), 136.2 (d, 4JCF = 2.2 Hz, C-1′′), 136.7 (C-1′), 157.4 (d, 1JCF = 238.6 Hz, C-4′′), 164.5 (C-4), 167.1 (C-6), 170.2 (C-2). Anal. calcd for C15H12FN5: C, 64.05; H, 4.30; N, 24.90. Found: C, 63.97; H, 4.38; N, 24.81.
N2-(2-Chlorophenyl)-6-phenyl-1,3,5-triazine-2,4-diamine (4)
Yield 0.26 g, 35%. Mp 82–84 °C (EtOH/H2O). 1H NMR (300 MHz, DMSO-d6): δ 7.10 (2H, brs, NH2), 7.20 (1H, ddd, J = 1.6 Hz, J = 7.5 Hz, J = 7.9 Hz, H-4′′), 7.37 (1H, ddd, J = 1.5 Hz, J = 7.5 Hz, J = 8.0 Hz, H-5′′), 7.46–7.54 (4H, m, H-3′, H-5′, H-4′ and H-3′′), 7.84 (1H, dd, J = 1.6 Hz, J = 8.0 Hz, H-6′′), 8.26 (2H, dd, J = 1.6 Hz, J = 6.7 Hz, H-2′ and H-6′), 8.75 (1H, s, NH); 13C NMR (75 MHz, DMSO-d6): δ 125.9 (C-6′′), 127.1 (C-4′′), 127.3 (C-2′′), 127.7 (C-3′ and C-5′), 128.0 (C-5′′), 128.2 (C-2′ and C-6′), 129.3 (C-3′′), 131.3 (C-4′), 135.7 (C-1′′), 136.5 (C-1′), 165.1 (C-4), 167.2 (C-6), 170.2 (C-2). Anal. calcd for C15H12ClN5: C, 60.51; H, 4.06; N, 23.52. Found: C, 60.39; H, 4.15; N, 23.44.
6-(4-Fluorophenyl)-N2-(4-methoxyphenyl)-1,3,5-triazine-2,4-diamine (30)
Yield 0.20 g, 30%. Mp 158–160 °C (MeCN). 1H NMR (300 MHz, DMSO-d6): δ 3.75 (3H, s, OCH3), 6.91 (2H, d, J = 9.0 Hz, H-3′′ and H-5′′), 7.06 (2H, brs, NH2), 7.33 (2H, dd, 3JHF = 9.0 Hz, 3JHH = 8.8 Hz, H-3′ and H-5′), 7.70 (2H, d, J = 8.9 Hz, H-2′′ and H-6′′), 8.36 (2H, dd, 4JHF = 5.8 Hz, 3JHH = 8.8 Hz, H-2′ and H-6′), 9.37 (1H, s, NH); 13C NMR (75 MHz, DMSO-d6): δ 55.1 (OCH3), 113.6 (C-3′′ and C-5′′), 115.1 (d, 2JCF = 21.7 Hz, C-3′ and C-5′), 121.8 (C-2′′ and C-6′′), 130.1 (d, 3JCF = 8.9 Hz, C-2′ and C-6′), 132.8 (C-1′′), 133.3 (d, 4JCF = 2.7 Hz, C-1′), 154.7 (C-4′′), 164.1 (d, 1JCF = 248.3 Hz, C-4′), 164.4 (C-4), 167.1 (C-6), 169.1 (C-2). Anal. calcd for C16H14FN5O: C, 61.73; H, 4.53; N, 22.50. Found: C, 61.60; H, 4.65; N, 22.34.
6-(4-Chlorophenyl)-N2-phenyl-1,3,5-triazine-2,4-diamine (34)
Yield 0.25 g, 33%. Mp 154–156 °C (EtOH). 1H NMR (300 MHz, DMSO-d6): δ 7.01 (1H, t, J = 7.9 Hz, H-4′′), 7.19 (2H, brs, NH2), 7.32 (2H, t, J = 7.9 Hz, H-3′′ and H-5′′), 7.60 (2H, d, J = 8.6 Hz, H-3′ and H-5′), 7.85 (2H, d, J = 7.9 Hz, H-2′′ and H-6′′), 8.33 (2H, d, J = 8.7 Hz, H-2′ and H-6′), 9.58 (1H, s, NH); 13C NMR (75 MHz, DMSO-d6): δ 120.0 (C-2′′ and C-6′′), 122.0 (C-1′′), 128.4 (C-3′, C-5′, C-3′′ and C-5′′), 129.5 (C-2′ and C-6′), 135.6 (C-1′), 136.1 (C-4′), 139.8 (C-4′′), 164.5 (C-4), 167.1 (C-6), 169.2 (C-2). Anal. calcd for C15H12ClN5: C, 60.51; H, 4.06; N, 23.52. Found: C, 60.46; H, 4.20; N, 23.36.
N2-(4-Fluorophenyl)-6-(3-methylphenyl)-1,3,5-triazine-2,4-diamine (53)
Yield 0.25 g, 35%. Mp 138–139 °C (EtOH/H2O). 1H NMR (300 MHz, DMSO-d6): δ 2.40 (3H, s, CH3), 7.13 (2H, brs, NH2), 7.15 (2H, dd, 3JHF = 8.9 Hz, 3JHH = 8.9 Hz, H-3′′ and H-5′′), 7.37–7.42 (2H, m, H-4′ and H-5′), 7.85 (2H, dd, 4JHF = 5.0 Hz, 3JHH = 9.1 Hz, H-2′′ and H-6′′), 8.10–8.16 (2H, m, H-2′ and H-6′), 9.56 (1H, s, NH); 13C NMR (75 MHz, DMSO-d6): δ 21.0 (CH3), 114.8 (d, 2JCF = 21.6 Hz, C-2′′ and C-6′′), 121.5 (d, 3JCF = 7.5 Hz, C-3′′ and C-5′′), 124.9 (C-2′), 128.1 (C-6′), 128.3 (C-5′), 131.9 (C-4′), 136.3 (d, 4JCF = 3.0 Hz, C-1′′), 136.7 (C-3′), 137.3 (C-1′), 157.4 (d, 1JCF = 238.4 Hz, C-4′′), 164.4 (C-2), 167.1 (C-4), 170.2 (C-6). Anal. calcd for C16H14FN5: C, 65.07; H, 4.78; N, 23.71. Found: C, 64.95; H, 4.91; N, 23.58.
N2-(4-Methoxyphenyl)-6-(3-methylphenyl)-1,3,5-triazine-2,4-diamine (57)
Yield 0.22 g, 30%. Mp 150–152 °C (EtOH/H2O). 1H NMR (300 MHz, DMSO-d6): δ 2.39 (3H, s, CH3), 3.74 (OCH3), 6.89 (2H, d, J = 9.0 Hz, H-3′′ and H-5′′), 7.02 (2H, brs, NH2), 7.34–7.41 (2H, m, H-4′ and H-5′), 7.71 (2H, d, J = 9.1 Hz, H-2′′ and H-6′′), 8.09–8.15 (2H, m, H-2′ and H-6′), 9.34 (1H, s, NH); 13C NMR (75 MHz, DMSO-d6): δ 21.0 (CH3), 55.1 (OCH3), 113.6 (C-3′′ and C-5′′), 121.6 (C-2′′ and C-6′′), 124.9 (C-2′), 128.1 (C-6′), 128.3 (C-5′), 131.8 (C-1′′), 132.9 (C-4′), 136.8 (C-3′), 137.2 (C-1′), 154.5 (C-4′′), 164.4 (C-2), 167.1 (C-4), 170.1 (C-6). Anal. calcd for C17H17N5O: C, 66.43; H, 5.58; N, 22.79. Found: C, 66.34; H, 5.70; N, 22.64.
N2-(4-Isopropylphenyl)-6-(3-methylphenyl)-1,3,5-triazine-2,4-diamine (59)
Yield 0.36 g, 45%. Mp 134–136 °C (EtOH/H2O). 1H NMR (300 MHz, DMSO-d6): δ 1.20 (6H, d, J = 6.9 Hz, (CH3)2), 2.40 (3H, s, CH3), 2.85 (1H, m, J = 7.0 Hz, CH), 7.08 (2H, brs, NH2), 7.17 (2H, d, J = 8.5 Hz, H-3′′ and H-5′′), 7.34–7.42 (2H, m, H-4′ and H-5′), 7.75 (2H, d, J = 8.6 Hz, H-2′′ and H-6′′), 8.12–8.18 (2H, m, H-2′ and H-6′), 9.44 (1H, s, NH); 13C NMR (75 MHz, DMSO-d6): δ 21.0 (CH3), 24.0 ((CH3)2), 32.8 (CH), 120.1 (C-2′′ and C-6′′), 125.0 (C-2′), 126.0 (C-3′′ and C-5′′), 128.1 (C-6′), 128.3 (C-5′), 131.9 (C-4′), 136.8 (C-3′), 137.2 (C-1′), 137.6 (C-1′′), 142.0 (C-4′′), 164.5 (C-2), 167.1 (C-4), 170.2 (C-6). Anal. calcd for C19H21N5: C, 71.45; H, 6.63; N, 21.93. Found: C, 71.38; H, 6.69; N, 21.85.
N2-(4-Methoxyphenyl)-6-(4-methylphenyl)-1,3,5-triazine-2,4-diamine (65)
Yield 0.45 g, 60%. Mp 168–170 °C (EtOH). 1H NMR (300 MHz, DMSO-d6): δ 2.38 (3H, s, CH3), 3.74 (3H, s, OCH3), 6.90 (2H, d, J = 9.0 Hz, H-3′′ and H-5′′), 7.00 (2H, brs, NH2), 7.30 (2H, d, J = 8.2 Hz, H-3′ and H-5′), 7.71 (2H, d, J = 9.0 Hz, H-2′′ and H-6′′), 8.21 (2H, d, J = 8.1 Hz, H-2′ and H-6′), 9.32 (1H, s, NH); 13C NMR (75 MHz, DMSO-d6): δ 21.0 (CH3), 55.1 (OCH3), 113.6 (C-3′′ and C-5′′), 121.6 (C-2′′ and C-6′′), 127.7 (C-3′ and C-5′), 128.8 (C-2′ and C-6′), 132.9 (C-1′′), 134.1 (C-1′), 141.1 (C-4′), 154.5 (C-4′′), 164.5 (C-4), 167.1 (C-6), 170.0 (C-2). Anal. calcd for C17H17N5O: C, 66.43; H, 5.58; N, 22.79. Found: C, 66.34; H, 5.66; N, 22.70.
N2,6-Bis(4-methoxyphenyl)-1,3,5-triazine-2,4-diamine (71)
Yield 0.30 g, 38%. Mp 163–165 °C (MeCN). 1H NMR (300 MHz, DMSO-d6): δ 3.74 (3H, s, OCH3), 3.84 (3H, s, OCH3), 6.90 (2H, d, J = 9.0 Hz, H-3′′ and H-5′′), 6.94 (2H, brs, NH2), 7.05 (2H, d, J = 8.9 Hz, H-3′ and H-5′), 7.71 (2H, d, J = 9.0 Hz, H-2′′ and H-6′′), 8.28 (2H, d, J = 8.9 Hz, H-2′ and H-6′), 9.28 (1H, s, NH); 13C NMR (75 MHz, DMSO-d6): δ 55.1 (OCH3), 55.2 (OCH3), 113.5 (C-3′′ and C-5′′), 113.6 (C-3′ and C-5′), 121.6 (C-2′′ and C-6′′), 129.1 (C-1′), 129.5 (C-2′ and C-6′), 133.0 (C-1′′), 154.5 (C-4′), 161.8 (C-4′′), 164.4 (C-4), 167.0 (C-6), 169.6 (C-2). Anal. calcd for C17H17N5O2: C, 63.15; H, 5.30; N, 21.66. Found: C, 63.02; H, 5.41; N, 21.57.
N2-(4-Fluorophenyl)-6-(4-(trifluoromethyl)phenyl)-1,3,5-triazine-2,4-diamine (84)
Yield 0.21 g, 28%. Mp 150–152 °C (EtOH). 1H NMR (300 MHz, DMSO-d6): δ 7.16 (2H, dd, 3JHF = 8.9 Hz, 3JHH = 8.9 Hz, H-3′′ and H-5′′), 7.29 (2H, brs, NH2), 7.85 (2H, dd, 4JHF = 5.1 Hz, 3JHH = 9.1 Hz, H-2′′ and H-6′′), 7.90 (2H, d, 3J = 8.6 Hz, H-3′ and H-5′), 8.50 (2H, d, J = 8.6 Hz, H-2′ and H-6′), 9.70 (1H, s, NH); 13C NMR (75 MHz, DMSO-d6): δ 114.9 (d, 2JCF = 22.5 Hz, C-3′′ and C-5′′), 121.8 (d, 3JCF = 7.5 Hz, C-2′′ and C-6′′), 124.1 (q, 1JCF = 271.5 Hz, CF3), 125.3 (q, 3JCF = 3.7 Hz, C-3′ and C-5′), 128.4 (C-1′), 131.1 (q, 2JCF = 31.6 Hz, C-4′), 136.1 (d, 4JCF = 2.2 Hz, C-1′′), 140.6 (q, 4JCF = 1.5 Hz, C-2′ and C-6′), 157.6 (d, 1JCF = 238.8 Hz, C-4′′), 164.5 (C-4), 167.1 (C-6), 169.0 (C-2). Anal. calcd for C16H11F4N5: C, 55.02; H, 3.17; N, 20.05. Found: C, 54.88; H, 3.26; N, 19.90.
N2-(4-Fluorophenyl)-6-(4-(trifluoromethoxy)phenyl)-1,3,5-triazine-2,4-diamine (91)
Yield 0.32 g, 58%. Mp 139–140 °C (EtOH). 1H NMR (300 MHz, DMSO-d6): δ 7.16 (2H, dd, 3JHF = 8.9 Hz, 3JHH = 8.9 Hz, H-3′′ and H-5′′), 7.22 (2H, brs, NH2), 7.51 (2H, d, J = 8.0 Hz, H-3′ and H-5′), 7.85 (2H, dd, 4JHF = 5.0 Hz, 3JHH = 9.0 Hz, H-2′′ and H-6′′), 8.42 (2H, d, J = 8.9 Hz, H-2′ and H-6′), 9.64 (1H, s, NH); 13C NMR (75 MHz, DMSO-d6): δ 114.9 (d, 2JCF = 22.1 Hz, C-3′′ and C-5′′), 119.4 (q, 1JCF = 154.2 Hz, OCF3), 120.5 (C-3′ and C-5′), 121.7 (d, 3JCF = 7.4 Hz, C-2′′ and C-6′′), 129.8 (C-2′ and C-6′), 135.8 (C-1′), 136.1 (d, 4JCF = 2.2 Hz, C-1′′), 150.6 (q, 3JCF = 1.5 Hz, C-4′), 157.5 (d, 1JCF = 239.0 Hz, C-4′′), 164.5 (C-4), 167.1 (C-6), 169.0 (C-2). Anal. calcd for C16H11F4N5O: C, 52.61; H, 3.04; N, 19.17. Found: C, 52.55; H, 3.21; N, 18.98.
6-(4-(Benzyloxy)phenyl)-N2-(4-fluorophenyl)-1,3,5-triazine-2,4-diamine (111)
Yield 0.53 g, 55%. Mp 177–179 °C (EtOH). 1H NMR (300 MHz, DMSO-d6): δ 5.19 (2H, s, CH2), 7.07 (2H, brs, NH2), 7.12–7.18 (4H, m, H-3′, H-5′, H-3′′ and H-5′′), 7.35–7.50 (5H, m, Ph), 7.86 (2H, dd, 4JHF = 5.0 Hz, 3JHH = 9.1 Hz, H-2′′ and H-6′′), 8.29 (2H, d, J = 8.9 Hz, H-2′ and H-6′), 9.52 (1H, s, NH); 13C NMR (75 MHz, DMSO-d6): δ 69.3 (CH2), 114.4 (C-3′ and C-5′), 114.8 (d, 2JCF = 22.3 Hz, C-3′′ and C-5′′), 121.5 (d, 3JCF = 7.4 Hz, C-2′′ and C-6′′), 127.7 (C-2′′′ and C-6′′′), 127.9 (C-4′′′), 128.4 (C-3′′′ and C-5′′′), 129.2 (C-1′), 129.5 (C-2′ and C-6′), 136.4 (d, 4JCF = 2.2 Hz, C-1′′), 136.7 (C-1′′′), 157.3 (d, 1JCF = 238.5 Hz, C-4′′) 161.0 (C-4′), 164.4 (C-4), 167.0 (C-6), 169.8 (C-2). Anal. calcd for C22H18FN5O: C, 68.21; H, 4.68; N, 18.08. Found: C, 68.08; H, 4.80; N, 17.96.
N2-(2-Fluorophenyl)-6-(thiophen-2-yl)-1,3,5-triazine-2,4-diamine (116)
Yield 0.32 g, 45%. Mp 165–167 °C (EtOH/H2O). 1H NMR (300 MHz, DMSO-d6): δ 7.09 (2H, brs, NH2), 7.15–7.27 (4H, m, H-4′, H-3′′, H-4′′ and H-5′′), 7.72–7.78 (2H, m, H-5′ and H-6′′), 7.87 (1H, dd, J = 1.1 Hz, J = 3.6 Hz, H-3′), 9.04 (1H, s, NH); 13C NMR (75 MHz, DMSO-d6): δ 115.5 (d, 2JCF = 19.4 Hz, C-3′′), 124.0 (d, 3JCF = 3.7 Hz, C-6′′), 125.5 (d, 3JCF = 7.6 Hz, C-4′′), 126.5 (d, 2JCF = 11.9 Hz, C-1′′), 126.6 (d, 4JCF = 2.2 Hz, C-5′′), 128.0 (C-4′), 129.2 (C-5′), 130.9 (C-3′), 142.4 (C-1′), 155.4 (d, 1JCF = 246.3 Hz, C-2′′), 164.8 (C-4), 166.6 (C-6), 166.9 (C-2). Anal. calcd for C13H10FN5S: C, 54.35; H, 3.51; N, 24.38. Found: C, 54.22; H, 3.75; N, 24.26.
N2-(2-Methoxyphenyl)-6-(thiophen-2-yl)-1,3,5-triazine-2,4-diamine (122)
Yield 0.43 g, 58%. Mp 165–167 °C (MeCN). 1H NMR (300 MHz, DMSO-d6): δ 3.87 (3H, s, OCH3), 6.94–7.00 (1H, m, H-3′′), 7.04–7.08 (2H, m, H-4′′ and H-5′′), 7.18 (2H, brs, NH2), 7.21 (1H, dd, J = 3.7 Hz, J = 5.0 Hz, H-4′), 7.77 (1H, dd, J = 1.2 Hz, J = 5.0 Hz, H-5′), 7.92 (1H, dd, J = 1.3 Hz, J = 3.7 Hz, H-3′), 7.97 (1H, s, NH), 8.21 (1H, d, J = 7.3 Hz, H-6′′); 13C NMR (75 MHz, DMSO-d6): δ 55.7 (OCH3), 110.9 (C-3′′), 120.3 (C-5′′), 121.8 (C-6′′), 123.5 (C-4′′), 127.7 (C-1′′), 128.1 (C-4′), 129.3 (C-5′), 131.0 (C-3′), 142.3 (C-1′), 149.6 (C-2′′), 164.2 (C-4), 166.6 (C-6), 166.9 (C-2). Anal. calcd for C14H13N5OS: C, 56.17; H, 4.38; N, 23.40. Found: C, 56.08; H, 4.50; N, 23.26.
N2-(4-Methoxyphenyl)-6-(thiophen-2-yl)-1,3,5-triazine-2,4-diamine (123)
Yield 0.52 g, 70%. Mp 139–141 °C (EtOH/H2O). 1H NMR (300 MHz, DMSO-d6): δ 3.74 (3H, s, OCH3), 6.88 (2H, d, J = 9.0 Hz, H-3′′ and H-5′′), 7.06 (2H, brs, NH2), 7.20 (1H, dd, J = 3.7 Hz, J = 5.0 Hz, H-4′), 7.70 (2H, d, J = 9.1 Hz, H-2′′ and H-6′′), 7.76 (1H, dd, J = 1.3 Hz, J = 5.0 Hz, H-5′), 7.89 (1H, dd, J = 1.3 Hz, J = 3.7 Hz, H-3′), 9.35 (1H, s, NH); 13C NMR (75 MHz, DMSO-d6): δ 55.1 (OCH3), 113.5 (C-3′′ and C-5′′), 121.6 (C-2′′ and C-6′′), 128.0 (C-4′), 129.0 (C-5′), 130.7 (C-3′), 132.8 (C-1′′), 142.6 (C-1′), 154.5 (C-4′′), 164.0 (C-4), 166.3 (C-6), 166.7 (C-2). Anal. calcd for C14H13N5OS: C, 56.17; H, 4.38; N, 23.40. Found: C, 56.05; H, 4.54; N, 23.23.
Cytotoxicity evaluation
The cytotoxic activity of 6,N2-diaryl-1,3,5-triazine-2,4-diamines (1–126) was evaluated against three breast carcinoma cell lines (MDA-MB231, SKBR-3, and MCF-7) and normal breast cell line (MCF-10A) by MTT assay.22 All cells were obtained from the American Type Culture Collection and were grown in 10% fetal bovine serum and 1% pen-strep antibiotic supplemented media (DMEM for MDA-MB231 and SKBR-3, RPMI for MCF-7, and MEGM for MCF-10A). For MTT assay, 20
000 to 75
000 cells per mL (based on the doubling time for each cell line) were seeded in 96 well plates and incubated for 24 h at 37 °C in 5% CO2 incubator. Then, compounds at different concentrations were added followed by the incubation at 37 °C for 72 h. After that, MTT solution (0.5 mg mL−1) was added and the plates were incubated for another 4 h. The supernatant was then discarded and 100 μL of DMSO was added to each well. The plates were then read by Tecan NanoQuant (model: infinite m200 pro) plate reader and absorbance was measured at 570 nm. GI50 values were calculated using sigmoidal concentration–response curves (see ESI†) generated using the GraphPad Prism 7 program. Three independent experiments were carried out and the data were represented as mean of the three experiments.
Building 3D-QSAR model
Out of 25 compounds, 19 compounds were utilized as a training set for building QSAR model. To assess reliability of the prepared model, an external validation was performed using remaining 6 compounds as a test set. The compounds were randomly divided into training and test set via ‘Generate training and test set’ module in Discover Studio v18. The selected test set included compounds 58, 73, 78, 99, 101, and 120.
For the model construction, the GI50 values of the compounds on MDA-MB231 were converted to the negative logarithmic scale (pGI50). The compounds were aligned to the minimum energy using the ‘Align small molecules’ protocol in the Discovery Studio. Steric (50%) and electrostatic (50%) fields were used to align the compounds.
In Discovery Studio, the CHARMm force field was used and the electrostatic potential and the van der Waals potential were treated as separate terms. A +1e point charge was used as the electrostatic potential probe and distance-dependent dielectric constant was used to mimic the solvation effect. For the van der Waals potential, a carbon atom with a 1.5 Å radius was used as a probe. The truncation for both the steric and electrostatic energies was set to 30 kcal mol−1. The standard parameters implemented in Discovery Studio v18 were used. A Partial Least-Square (PLS) model was built using energy grids as descriptors. The QSAR model was built using the created 3D-QSAR protocol of Discovery Studio v18.
Conflicts of interest
There are no conflicts to declare.
Acknowledgements
This work is supported by the Ministry of Higher Education, Malaysia under the Fundamental Research Grant Scheme, grant number FRGS/1/2018/STG01/MUSM/02/2. The work is also partially supported by the Bridging Grant from the School of Pharmacy, Monash University Malaysia.
References
- P. Singla, V. Luxami and K. Paul, Triazine as a promising scaffold for its versatile biological behavior, Eur. J. Med. Chem., 2015, 102, 39–57 CrossRef CAS PubMed.
- S. Cascioferro, B. Parrino, V. Spano, A. Carbone, A. Montalbano, P. Barraja, P. Diana and G. Cirrincione, 1,3,5-Triazines: a promising scaffold for anticancer drugs development, Eur. J. Med. Chem., 2017, 142, 523–549 CrossRef CAS PubMed.
- J. H. Silverberg and W. Dameshek, Use of triethylene melamine in treatment of leukemia and leukosarcoma, JAMA, 1952, 148, 1015–1021 CrossRef CAS PubMed.
- J. R. Walsh, P. T. Pratt, W. E. Graham and H. J. Zimmerman, Treatment of leukemia and lymphomata with triethylene melamine, Acta Haematol., 1954, 11, 329–338 CrossRef CAS PubMed.
- P. G. Dyment, D. J. Fernbach and W. W. Sutow, Hexamethylmelamine (NSC-13875) for acute leukemia and solid tumors in children, J. Clin. Pharmacol. New Drugs, 1973, 13, 111–113 CrossRef CAS PubMed.
- M. Karon, L. Sieger, S. Leimbrock, J. Z. Finklestein, M. E. Nesbit and J. J. Swaney, 5-Azacytidine: a new active agent for the treatment of acute leukemia, Blood, 1973, 42, 359–365 CrossRef CAS PubMed.
- A. Aribi, G. Borthakur, F. Ravandi, J. Shan, J. Davisson, J. Cortes and H. Kantarjian, Activity of decitabine, a hypomethylating agent, in chronic myelomonocytic leukemia, Cancer, 2007, 109, 713–717 CrossRef CAS PubMed.
- E. Jabbour, J.-P. Issa, G. Garcia-Manero and H. Kantarjian, Evolution of decitabine development: accomplishments, ongoing investigations, and future strategies, Cancer, 2008, 112, 2341–2351 CrossRef CAS PubMed.
- E. S. Kim, Enasidenib: First global approval, Drugs, 2017, 77, 1705–1711 CrossRef CAS PubMed.
- E. M. Stein, Enasidenib, a targeted inhibitor of mutant IDH2 proteins for treatment of relapsed or refractory acute myeloid leukemia, Future Oncol., 2018, 14, 23–40 CrossRef CAS PubMed.
- A. M. Venkatesan, C. M. Dehnhardt, E. Delos Santos, Z. Chen, O. Dos Santos, S. Ayral-Kaloustian, G. Khafizova, N. Brooijmans, R. Mallon, I. Hollander, L. Feldberg, J. Lucas, K. Yu, J. Gibbons, R. T. Abraham, I. Chaudhary and T. S. Mansour, Bis(morpholino-1,3,5-triazine) Derivatives: Potent Adenosine 5′-Triphosphate Competitive Phosphatidylinositol-3-kinase/Mammalian Target of Rapamycin Inhibitors: Discovery of Compound 26 (PKI-587), a Highly Efficacious Dual Inhibitor, J. Med. Chem., 2010, 53, 2636–2645 CrossRef CAS PubMed.
- M. Robert, R. F. Larry, L. Judy, C. Inder, D. Christoph, D. S. Efren, C. Zecheng, D. S. Osvaldo, A. K. Semiramis, V. Aranapakam and H. Irwin, Antitumor efficacy of PKI-587, a highly potent dual PI3K/mTOR kinase inhibitor, Clin. Cancer Res., 2011, 17, 3193–3203 CrossRef PubMed.
- B. J. Rhy, S. Kim, B. Min, K. Y. Kim, J. S. Lee, W. J. Park, H. Lee, S. H. Kim and S. Y. Park, Discovery and the structural basis of a novel p21-activated kinase 4 inhibitor, Cancer Lett., 2014, 349, 45–50 CrossRef PubMed.
- M. Koh, J. C. Lee, C. Min and A. Moon, A novel metformin derivative, HL010183, inhibited proliferation and invasion of triple negative breast cancer cells, Bioorg. Med. Chem., 2013, 8, 2305–2313 CrossRef PubMed.
- G. Miao, B. Liu, X. Guo, X. Zhang and G. Liu, Reduction behavior induced by HL010183, a metformin derivative against the growth of cutaneous squamous cell carcinoma, Int. J. Clin. Exp. Pathol., 2015, 1, 287–297 Search PubMed.
- A. Junaid, F. P. L. Lim, E. R. T. Tiekink and A. V. Dolzhenko, New one-pot
synthesis of 1,3,5-triazines: three-component condensation, Dimroth rearrangement and dehydrogenative aromatization, ACS Comb. Sci., 2019, 21, 548–555 CrossRef CAS PubMed.
- A. Junaid and A. V. Dolzhenko, Microwave-assisted synthesis of 1,3,5-triazines: efficient approaches to therapeutically valuable scaffold, Heterocycles, 2019, 98, 1678–1706 CrossRef.
- D. L. Holliday and V. Speirs, Choosing the right cell line for breast cancer research, Breast Cancer Res., 2011, 4, 215 CrossRef PubMed.
- Discovery Studio Modeling Environment, Biovia DS, Dassault Systems, San Diego, 2017 Search PubMed.
- M. Awasthi, S. Singh, V. P. Pandey and U. N. Dwivedi, Molecular docking and 3D-QSAR-based virtual screening of flavonoids as potential aromatase inhibitors against estrogen-dependent breast cancer, J. Biomol. Struct. Dyn., 2015, 33, 804–819 CrossRef CAS PubMed.
- B. Yang, Y. S. Yang, N. Yang, G. Li and H. L. Zhu, Design, biological evaluation and 3D QSAR studies of novel dioxin-containing pyrazoline derivatives with thiourea skeleton as selective HER-2 inhibitors, Sci. Rep., 2016, 6, 27571 CrossRef CAS PubMed.
- D. A. Scudiero, R. H. Shoemaker, K. D. Paull, A. Monks, S. Tierney, T. H. Nofziger, M. J. Currens, D. Seniff and M. R. Boyd, Evaluation of a Soluble Tetrazolium/formazan Assay for Cell Growth and Drug Sensitivity in Culture Using Human and Other Tumor Cell Lines, Cancer Res., 1988, 48, 4827–4833 CAS.
Footnote |
† Electronic supplementary information (ESI) available: Copies of 1H and 13C NMR spectra of new compounds and concentration–response plots used for the calculation GI50 values. See DOI: 10.1039/d0ra00643b |
|
This journal is © The Royal Society of Chemistry 2020 |