DOI:
10.1039/D0RA00615G
(Paper)
RSC Adv., 2020,
10, 12953-12961
Nitrogen-containing switchable solvents for separation of hydrocarbons and their derivatives
Received
20th January 2020
, Accepted 17th March 2020
First published on 31st March 2020
Abstract
Solvent extraction is commonly used to separate mixtures of hydrocarbons and their derivatives, and solvent choice is strongly influenced by the affinity to the target component, cost and safety. Nitrogen-containing switchable solvents can switch from water-immiscible form to water-miscible bicarbonate salts when CO2 is injected and back to water-immiscible form when N2 is injected. Switchable solvents, as a type of recyclable green solvent, can be used not only to separate such mixtures but also to reduce process costs. Herein, four switchable solvents, namely, dipropylamine, di-sec-butylamine, N,N-dimethylcyclohexylamine (CyNMe2), and N,N,N′,N′-tetraethyl-1,3-propanediamine (TEPDA), were employed to separate benzene/cyclohexane, ethyl acetate/acetonitrile, and ethyl acetate/n-heptane mixtures, and the corresponding partition and selectivity coefficients were determined. In all cases, separation selectivity increased in the order of dipropylamine < di-sec-butylamine < CyNMe2 < TEPDA, i.e., TEPDA was best suited for the separation of hydrocarbons and their derivatives. In addition, cycling experiments revealed that the TEPDA can be re-used at least 15 times and was well suited for industrial applications. In the end, the mechanism of the separation was put forward.
1. Introduction
The effective separation of mixtures of hydrocarbons and their derivatives, which are important petroleum components, can make petroleum resource utilisation more efficient. At present, the above separation is widely realised using solvent extraction, with much attention directed at the investigation of traditional solvents such as sulfolanes, esters, alcohols, and ionic liquids (ILs). However, as the toxicity, volatility, and flammability of organic solvents pose potential hazards during industrial-scale separation, new, highly efficient, and environmentally friendly solvents for hydrocarbon mixture separation are highly sought.1–4
As a type of recyclable green solvents, switchable solvents are soluble in water under a CO2 atmosphere and insoluble in the absence of CO2.1–7 The polarity, hydrophobic–hydrophilic and other properties of switchable solvents can be reversibly changed in the presence of triggers. Taking advantage of this property, a switchable solvent can be used to extract the product first, and then, a trigger is added to separate the product from the solvent through a phase transition process, and the solvent can be recovered after removal of the trigger, all without any distillation step, which greatly simplifies the process of solvent recovery. However, most switchable solvents are amines, as these compounds are protonated in aqueous media in an atmosphere of CO2 and deprotonated under N2;8 however, not all amines are switchable solvents.9,10 Nitrogen-containing switchable solvents have been widely used for extraction,11–15,17 e.g., Holland et al. used N,N-dimethylcyclohexylamine (CyNMe2) to extract bitumen, achieving bitumen recoveries of 94–97%.18 Besides, the above amine was also used to extract lipids from Botryococcus braunii microalgae with an extraction efficiency of 22%.11 Wang et al. studied the physicochemical properties of switchable-hydrophilicity solvent systems,19 and Bediako et al. used CyNMe2 as a switchable solvent in their novel method of producing fine silica aerogel powder from silica hydrogel.20 In the past few years, switchable solvent microextraction has been extensively studied.16,21–26 These findings indicate that nitrogen-containing switchable solvents can be effectively applied in extraction-based separation.
Hydrocarbon mixtures are generally difficult to separate using conventional solvents, e.g., benzene/cyclohexane mixtures can only be separated by extractive distillation in view of the similar volatilities of these hydrocarbons.27–30 Li et al. showed that ILs can be used as entrainers to facilitate the separation of benzene and cyclohexane by extractive distillation,27 which is also the only method allowing the separation of ethyl acetate/acetonitrile and ethyl acetate/n-heptane mixtures31–33 and is characterised by high energy consumption and equipment cost.33 Although many researchers have applied switchable solvents to the separation of mixtures of hydrocarbons and their derivatives, the corresponding studies involving nitrogen-containing switchable solvents are few.
Accordingly, we make use of the variable ability of nitrogen-containing switchable solvents to separate different hydrocarbons and their derivatives to design a novel procedure for the separation of hydrocarbons and their derivatives, employing benzene/cyclohexane, ethyl acetate/acetonitrile, and ethyl acetate/n-heptane as model mixtures and dipropylamine, di-sec-butylamine, CyNMe2, and N,N,N′,N′-tetraethyl-1,3-propanediamine (TEPDA) as model solvents. In addition, process parameters are optimised, and the effects of switchable solvent and mixture component identities on separation selectivity and solvent recyclability are revealed.
2. Materials and methods
2.1. Materials
Chemicals, including dipropylamine, di-sec-butylamine, CyNMe2, TEPDA, benzene, cyclohexane, ethyl acetate, acetonitrile, and n-heptane were of analytical grade and were purchased from Aladdin Reagent Company Co., Ltd., China. The chemical structures of nitrogen-containing switchable solvents are shown in Fig. 1. CO2 and N2 were used at a purity of 99.9%. Milli-Q water (18.2 MΩ cm at 25 °C) was used throughout.
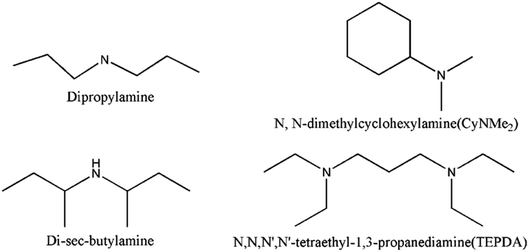 |
| Fig. 1 Chemical structures of nitrogen-containing switchable solvents. | |
2.2. Separation of benzene/cyclohexane, ethyl acetate/acetonitrile, and ethyl acetate/n-heptane
Details of the separation procedure are provided in Fig. 2. Typically, a benzene/cyclohexane mixture (2 mL of each component) was treated with dipropylamine (16 mL), and the resulting homogenous solution was transferred into a 100 mL beaker held at a constant temperature of 45 °C in a water bath. Then, CO2 (300 mL) was injected into the mixture over 30 min, which was followed by the injection of deionised water (16 mL) to induce separation into upper (cyclohexane) phase and lower (benzene + dipropylamine) phases. The concentrations of cyclohexane and benzene were determined by gas chromatography (GC) and used to calculate the cyclohexane partition coefficient, the benzene partition coefficient, and the benzene selectivity coefficient, with the benzene selectivity coefficient employed to assess separation efficiency. Subsequently, N2 was injected into the lower phase to induce separation into the upper dipropylamine phase and the lower benzene phase, and then the upper phase and the lower phase was phase separation, and then the amine could be recycling use. In the next experiment, the amine was used for the separation again, and then the separation effect was calculated. In addition, using different switchable solvents (CyNMe2, di-sec-butylamine and TEPDA) to separate ethyl acetate/acetonitrile and ethyl acetate/n-heptane mixtures according to the method described above under the same operating conditions. The detailed device diagram of separating benzene and cyclohexane was shown in Fig. 3, and other hydrocarbons and their derivatives separation was similar. The selectivity coefficient was determined according to eqn (1). Dbenzene and Dcyclohexane were the partition coefficient of benzene and cyclohexane, which were calculated by the eqn (2). In the eqn (2), ym and yn represent the mole fractions of components in the m and n phases, respectively, Vm and Vn represent the volumes of the m and n phases, respectively. |
 | (1) |
|
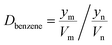 | (2) |
|
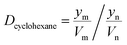 | (3) |
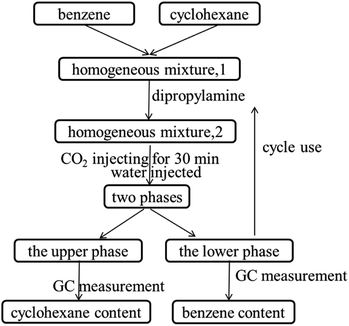 |
| Fig. 2 Separation of benzene/cyclohexane based on the use of dipropylamine. | |
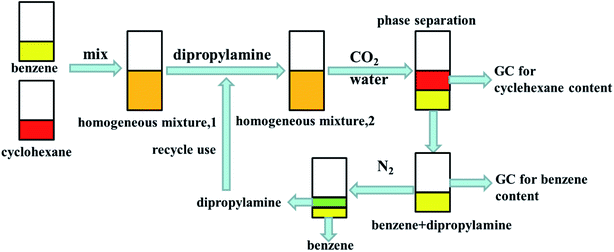 |
| Fig. 3 Experimental steps followed in this work to separate hydrocarbons and their derivatives. | |
All the experimental data were repeated at least three times to obtain a reliable result. The error bars value of experimental data was calculated according to the following eqn (4). The error bars were the standard deviation. In eqn (4), the N represents the total measurement times, and the xi represents every measurement value, and the
represents the average value of every measurement.
|
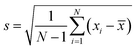 | (4) |
2.3. Optimisation of the separation process and cycling experiments
The performances of the four switchable solvents for the separation of benzene/cyclohexane, ethyl acetate/acetonitrile, and ethyl acetate/n-heptane mixtures were optimised using the setup in Fig. 2 by varying (1) the CO2 injection rate (100, 200, 300, 400, and 500 mL min−1), (2) temperature (25, 35, 45, 55, and 65 °C), and (3) solvent amount (4, 8, 12, 16, and 20 mL).
Cycling experiments were performed to evaluate the suitability of the developed method for industrial applications. After N2 injection into the lower phase, the solvents were recovered and re-used, and selectivity coefficients were calculated after each cycle.
3. Results and discussion
3.1. Benzene/cyclohexane separation
For all solvents and mixtures, a homogeneous phase was formed after CO2 injection into amine–water mixtures because of amine protonation (amine + H2O + CO2 → amineH+ + HCO3−), whereas subsequent N2 injection resulted in separation into two phases due to the reverse process (i.e., decomposition into the amine and CO2).
Fig. 4 presents the effects of the three parameters on the benzene selectivity coefficient, revealing that TEPDA showed the best performance among the four solvents. The benzene selectivity coefficient increased with CO2 injection rate (Fig. 3(a)), as high injection rates favoured switchable intermediate formation, reaching saturation at 300 mL min−1, which indicated the establishment of equilibrium. Due to the CO2 injecting rate influence the formation of switchable hydrophilic solvent salts,8 and when the switchable hydrophilic salts formed, then the phase separation between benzene and cyclohexane was clear. With the higher CO2 injecting rate, the switchable hydrophilic solvent salts would form faster, and then the benzene selectivity coefficient would increase. A similar trend was observed for temperature, with saturation observed at 45 °C in cases of dipropylamine, di-sec-butylamine, and TEPDA (Fig. 3(b)). In the case of CyNMe2, the benzene selectivity coefficient decreased at temperatures above 45 °C because of the concomitant decrease of CyNMe2 switchability. Therefore, the optimal temperature was identified as 45 °C. Finally, the benzene selectivity coefficient increased with solvent amount (Fig. 3(c)), saturating at 16 mL, which was therefore selected as the optimal solvent volume. Among the four solvents, TEPDA was most influenced by the three to-be-optimised parameters, and this high sensitivity was ascribed to the relatively slow protonation attributable to the presence of two amine groups.
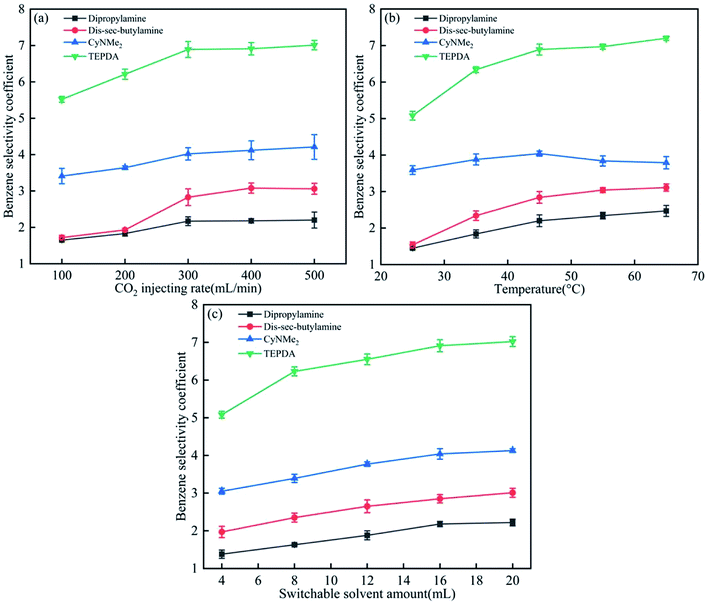 |
| Fig. 4 Effects of (a) CO2 injection rate, (b) temperature, and (c) switchable solvent amount on the benzene selectivity coefficient. | |
3.2. Ethyl acetate/acetonitrile separation
As shown in Fig. 5(a), the ethyl acetate selectivity coefficient increased with increasing CO2 injection rate, saturating above 400 mL min−1, which was therefore chosen as the optimal injection rate. Temperature and solvent amount had similar effects (Fig. 5(b) and (c)), with optimal values determined as 45 °C and 16 mL, respectively.
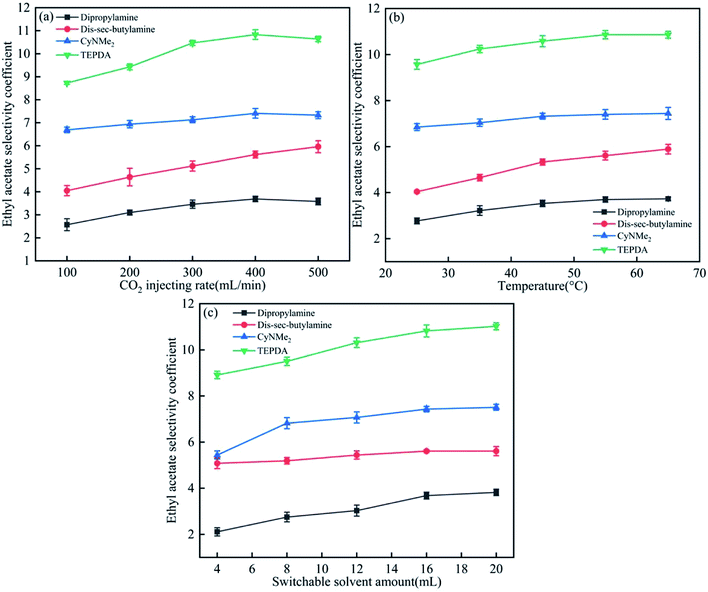 |
| Fig. 5 Effects of (a) CO2 injection rate, (b) temperature, and (c) switchable solvent amount on the ethyl acetate selectivity coefficient. | |
3.3. Ethyl acetate/n-heptane separation
As shown in Fig. 6(a), the ethyl acetate selectivity coefficient remained almost unchanged with increasing CO2 injection rate, and the smallest rate of 100 mL min−1 was therefore chosen as optimal. The above coefficient increased with increasing temperature and solvent amount (Fig. 6(b) and (c)), with saturation-marking values of 55 °C and 8 mL chosen as optimal.
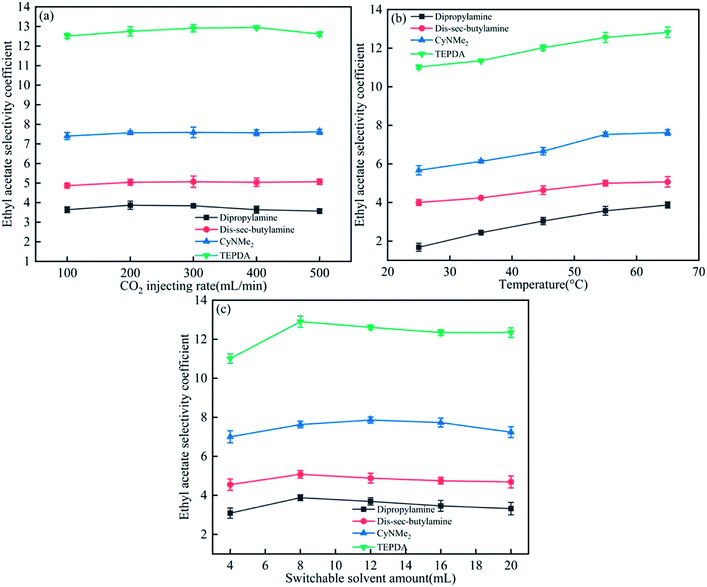 |
| Fig. 6 Effects of (a) CO2 injection rate, (b) temperature, and (c) switchable solvent amount on the ethyl acetate selectivity coefficient. | |
3.4. Partition and selectivity coefficients
As shown in Fig. 7, the benzene partition coefficient increased in the order of dipropylamine (0.85) < di-sec-butylamine (0.97) < CyNMe2 (1.13) < TEPDA (1.45), and the cyclohexane partition coefficient decreased in the order of dipropylamine (0.39) > di-sec-butylamine (0.34) > CyNMe2 (0.28) > TEPDA (0.21), i.e., separation efficiency increased in the order of dipropylamine < di-sec-butylamine < CyNMe2 < TEPDA. The same behaviour was observed for the ethyl acetate partition coefficient in cases of ethyl acetate/acetonitrile and ethyl acetate/n-heptane separation, which was ascribed to the increase of amine steric hindrance (and, hence, of separation efficiency) in the order of dipropylamine < di-sec-butylamine < CyNMe2 < TEPDA. The enhanced performance of TEPDA was ascribed to its diamine nature. Fig. 8 indicates that all selectivity coefficients increased in the order of dipropylamine < di-sec-butylamine < CyNMe2 < TEPDA, in agreement with the results presented in Fig. 6. For the same switchable solvent, the selectivity coefficient increased in the order of benzene < ethyl acetate (in acetonitrile mixture) < ethyl acetate (in n-heptane mixture), which was ascribed to the concomitant decrease of structural similarity between mixture components. The chosen switchable solvents were an organic solvent which could be used to dissolve the hydrocarbons and their derivatives for separation. Take the TEPDA for example, after the separation of hydrocarbons and their derivatives, the switchable solvents could be separated from the mixed hydrocarbons by the addition of water and CO2 injection. This protonated TEPDA would be switched back to TEPDA by heating or N2 bubbling to remove the CO2. However, when the water was added to the TEPDA in the separation system, things become more complex. The TEPDA would play several different roles in this aqueous/non-aqueous hybrid process. Most of the TEPDA still acted as a solvent in its non-ionic state to soften and dissolve the mixed hydrocarbons and their derivatives. The dissolution of mixture in the TEPDA allowed the solubility between the mixed hydrocarbons. At the same time, a small amount of TEPDA was ionized in water. The TEPDA ions (i.e., (C2H5)2N–(CH2)3–NH+(C2H5)2) in the water solution acts like a surfactant in enhancing the mixed hydrocarbons and their derivatives separation. The accumulation of TEPDA ions at the interface reduces the mixed hydrocarbons and their derivatives–water interfacial tension, resulting in the separation.
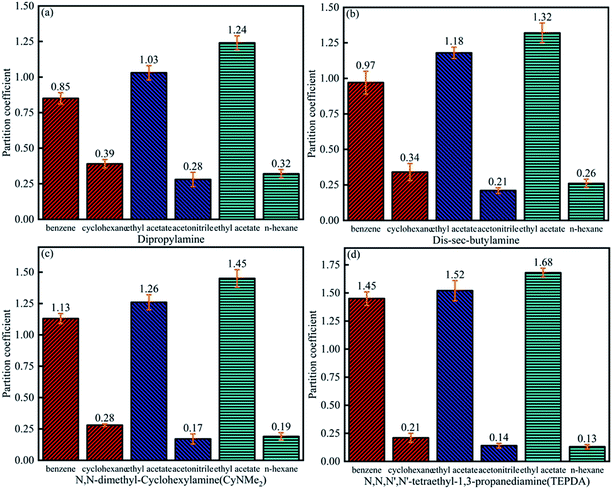 |
| Fig. 7 Partition coefficients obtained for benzene/cyclohexane, ethyl acetate/acetonitrile, and ethyl acetate/n-heptane separations using (a) dipropylamine, (b) di-sec-butylamine, (c) CyNMe2, and (d) TEPDA. | |
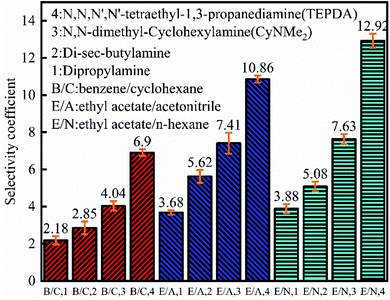 |
| Fig. 8 Selectivity coefficients obtained for benzene/cyclohexane, ethyl acetate/acetonitrile, and ethyl acetate/n-heptane separations. | |
3.5. Recyclability of nitrogen-containing switchable solvents
Fig. 9 summarises the effects of solvent recycling on separation performance. For dipropylamine and di-sec-butylamine, selectivity coefficients decreased from the first cycle to the tenth cycle, subsequently stabilising. For CyNMe2 and TEPDA, stabilisation was observed after the seventh and fifth cycles, respectively. In general, the employed solvents could be recycled for at least 15 times, and the corresponding decrease of separation efficiency was acceptable for industrial applications. In addition, the switchable solvent (TEPDA) was applied to oil–solid separation. Xingang Li et al. used TEPDA to separate heavy oil from a carbonate solid surface, resulting in over 10% additional oil recovery.34
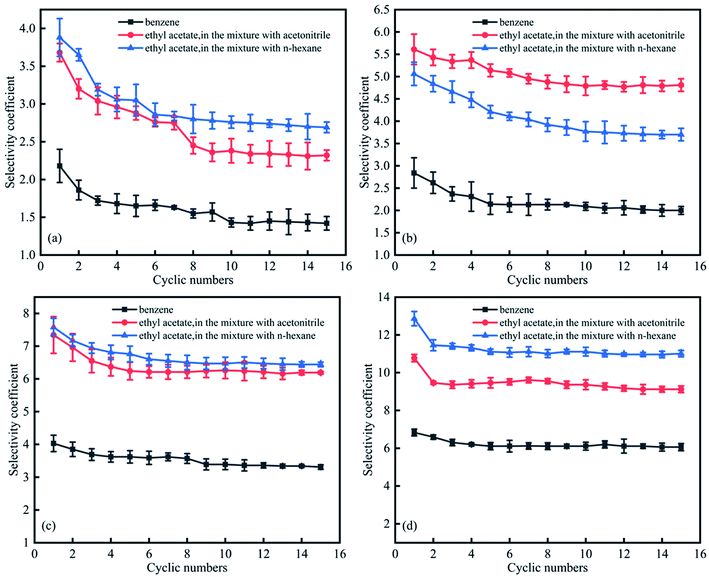 |
| Fig. 9 Effect of cycle number on selectivity coefficients for (a) dipropylamine, (b) di-sec-butylamine, (c) CyNMe2, and (d) TEPDA. | |
3.6. The mutual solubility of switchable solvents in the products
The four different switchable solvents (dipropylamine, di-sec-butylamine, CyNMe2 and TEPDA) solubility and recovery were shown in Table 1. The mutual solubility of switchable solvents in the products were low, and the switchable solvents recovery were high.
Table 1 The four switchable solvents (dipropylamine, di-sec-butylamine, CyNMe2, TEPDA) in the solubility in the products and recovery
Switchable solvent |
Separation products |
Solubility (g g−1) |
Recovery (%) |
Dipropylamine |
Benzene/cyclohexane |
0.002 |
99.63 |
Ethyl acetate/acetonitrile |
0.021 |
99.83 |
Ethyl acetate/n-heptane |
0.006 |
98.97 |
Di-sec-butylamine |
Benzene/cyclohexane |
0.012 |
98.67 |
Ethyl acetate/acetonitrile |
0.015 |
99.32 |
Ethyl acetate/n-heptane |
0.023 |
99.58 |
CyNMe2 |
Benzene/cyclohexane |
0.008 |
99.67 |
Ethyl acetate/acetonitrile |
0.041 |
99.63 |
Ethyl acetate/n-heptane |
0.032 |
99.32 |
TEPDA |
Benzene/cyclohexane |
0.009 |
99.21 |
Ethyl acetate/acetonitrile |
0.013 |
99.72 |
Ethyl acetate/n-heptane |
0.021 |
98.67 |
4. Conclusions
Four nitrogen-containing switchable solvents (dipropylamine, di-sec-butylamine, CyNMe2, and TEPDA) were used to separate benzene/cyclohexane, ethyl acetate/acetonitrile, and ethyl acetate/n-heptane mixtures, and the following conclusions were drawn.
(1) Optimal separation conditions depended on the chosen mixture.
(2) All selectivity coefficients increased in the order of dipropylamine < di-sec-butylamine < CyNMe2 < TEPDA. For the same solvent and operating conditions, the selectivity coefficient increased in the order of benzene < ethyl acetate (in acetonitrile mixture) < ethyl acetate (in n-heptane mixture).
(3) The tested solvents could be recycled for at least 15 times, thus being well suited for industrial-scale separation of hydrocarbons and their derivatives.
Funding
This work was financially supported by the 13th Five-Year Plan National Key Project of China (No. 2017ZX05005005-006), the National Major Science and Technology Project of China (No. 2017ZX05032-004-005), the CNOOC Project (2019KJ-ZC02) and the National Natural Science Foundation of China (No. 51974354 and No. 51704322).
Conflicts of interest
The authors declare no conflict of interests.
References
- P. G. Jessop, S. M. Mercer and D. J. Heldebrant, CO2-Triggered Switchable Solvents, Surfactants, and Other Materials, Energy Environ. Sci., 2012, 5, 7240–7253 RSC.
- G. J. Philip, L. Phan, A. Carrier, S. Robinson, J. D. Christoph and R. H. Jitendra, A solvent having switchable hydrophilicity, Green Chem., 2010, 12, 809–814 RSC.
- P. G. Jessop, C. A. Eckert, C. L. Liotta and D. J. Heldebrant, Switchable solvents and methods of use thereof, US Pat., 2008/005849A1, United States Patent Application Publication, 2008.
- J. Durelle, J. R. Vanderveen, Yi Quan, C. B. Chalifoux, J. Kostin and P. G. Jessop, Extending the range of switchable-hydrophilicity solvents, Phys. Chem. Chem. Phys., 2015, 17, 5308–5313 RSC.
- C. Samorì, L. Pezzolesi, D. López Barreiro, A. Quintavalla, A. Pasteris and E. Tagliavini, Synthesis of new polyethoxylated tertiary amines and their use as Switchable Hydrophilicity Solvents, RSC Adv., 2014, 4, 5999–6008 RSC.
- P. Pollet, C. A. Eckert and C. L. Liotta, Switchable solvents, Chem. Sci., 2011, 2, 609–614 RSC.
- A. D. Wilson and F. F. Stewart, Structure–function study of tertiary amines as switchable polarity solvents, RSC Adv., 2014, 4, 11039–11049 RSC.
- Y. Kazemzadeh, I. Ismail, H. Rezvani, M. Sharifi and M. Riazi, Experimental investigation of stability of water in oil emulsions at reservoir conditions: Effect of ion type, ion concentration, and system pressure, Fuel, 2019, 243, 15–27 CrossRef CAS.
- P. G. Jessop, J. R. Vanderveen and J. Durelle, Design and evaluation of switchable-hydrophilicity solvents, Green Chem., 2014, 16, 1187–1197 RSC.
- J. Durelle, J. R. Vanderveen and G. Philip, Jessop, Modelling the behaviour of switchable-hydrophilicity solvents, Phys. Chem. Chem. Phys., 2014, 16, 5270–5275 RSC.
- A. R. Boyd, P. Champagne, P. J. McGinn, K. M. MacDougall, J. E. Melanson and P. G. Jessop, Switchable hydrophilicity solvents for lipid extraction from microalgae for biofuel production, Bioresour. Technol., 2012, 118, 628–632 CrossRef CAS PubMed.
- P. Lam, H. Brown, J. F. White, A. Hodgson and P. G. Jessop, Soybean oil extraction and separation using switchable or expanded solvents, Green Chem., 2009, 11, 53–59 RSC.
- C. Samorì, D. López Barreiro, R. Vet, L. Pezzolesi, W. Brilman, P. Galletti and E. Tagliavini, Effective lipid extraction from algae cultures using switchable solvents, Green Chem., 2013, 15, 353–356 RSC.
- Y. Du, B. Schuur, R. Sascha, A. Kersten and D. W. F. Brilman, Opportunities for switchable solvents for lipid extraction from wet algal biomass: An energy evaluation, Algal Res., 2015, 11, 271–283 CrossRef.
- N. R. Carty, J. A. Kornfield and J. Lal, Using the “Switchable” Quality of Liquid Crystal Solvents To Mediate Segregation between Coil and Liquid Crystalline Polymers, Macromolecules, 2006, 39, 3921–3926 CrossRef.
- M. Khan and S. Mustafa, Switchable solvent based liquid phase microextraction of mercury from environmental samples: a green aspect, RSC Adv., 2016, 6, 10–1039 Search PubMed.
- E. Yilmaz and M. Soylak, Switchable solvent based liquid phase microextraction of copper(II): optimization and application to environmental samples, J. Anal. At. Spectrom., 2015, 30, 1629–1635 RSC.
- A. Holland, D. Wechsler, A. Patel, B. M. Molloy, A. R. Boyd and P. G. Jessop, Separation of bitumen from oil sands using a switchable hydrophilicity solvent, Can. J. Chem., 2012, 90, 805–810 CrossRef CAS.
- J. Wang, Y. Du, C. Du, A. Xu, G. Yao, H. Zhao, X. Zhu and X. Guo, Physicochemical properties of switchable-hydrophilicity solvent systems: N,N-Dimethylcyclohexylamine, water and carbon dioxide, J. Chem. Thermodyn., 2019, 133, 1–9 CrossRef CAS.
- A. Bediako, B. Bernard, P. Zhou, R. Benoit, Q. Liu, Y. Su, H. Wang and J. Li, A switchable hydrophilicity solvent mediated process to prepare fine silica aerogel powder as an excellent flatting agent, Adv. Powder Technol., 2019, 30, 565–571 CrossRef.
- Y. Xu, F. Wang, Q. Hou, Y. Zhao, G. Ding and X. Xu, Strategy for Synthesizing Novel Acetamidines as CO2-Triggered Switchable Surfactants via Acetimidates, Trans. Tianjin Univ., 2019, 25, 237–244 CrossRef CAS.
- G. Lasarte-Aragonés, R. Lucena, S. Cárdenas and M. Valcárcel, Use of switchable solvents in the microextraction context, Talanta, 2015, 131, 645–649 CrossRef PubMed.
- G. Lasarte-Aragonés, R. Lucena, S. Cárdenas and M. Valcárcel, Use of switchable hydrophilicity solvents for the homogeneous liquid–liquid microextraction of triazine herbicides from environmental water samples, J. Sep. Sci., 2015, 38, 990–995 CrossRef PubMed.
- R. Jamileh, K. S. Hasheminasab, A. Laleh and A. Hamid, Switchable-hydrophilicity solvent-based microextraction combined with gas chromatography for the determination of nitroaromatic compounds in water samples, J. Sep. Sci., 2017, 40, 3114–3119 CrossRef PubMed.
- M. Reclo, E. Yilmaz, Y. Bazel and M. Soylak, Switchable solvent based liquid phase microextraction of palladium coupled with determination by flame atomic absorption spectrometry, Int. J. Environ. Anal. Chem., 2017, 97, 1315–1327 CrossRef CAS.
- S. Mustafa, M. Khan and E. Yilmaz, Switchable solvent based liquid phase microextraction of uranium in environmental samples: A green approach, Anal. Methods, 2016, 8, 979–986 RSC.
- W. Li, B. Xu, Z. Lei and C. Dai, Separation of benzene and cyclohexane by extractive distillation intensified with ionic liquid, Chem. Eng. Process., 2018, 126, 81–89 CrossRef CAS.
- L. Li, Y. Tu, L. Guo, L. Sun and Y. Tian, Optimization and Control of Extractive Distillation with Heat Integration for Separating Benzene/Cyclohexane Mixtures, China Pet. Process. Petrochem. Technol., 2016, 18, 117–127 Search PubMed.
- J. Qin, Q. Ye, X. Xiong and Li Ning, Control of Benzene-Cyclohexane Separation System via Extractive Distillation Using Sulfolane as Entrainer, Ind. Eng. Chem. Res., 2013, 52, 10754–10766 CrossRef CAS.
- Y. Liu, Z. Qiu, H. Zhong, Z. Nie, Li Jia, W. Huang and X. Zhao, Bitumen Recovery from Crude Bitumen Samples from Halfaya Oilfield by Single and Composite Solvents-Process, Parameters, and Mechanism, Materials, 2019, 12, 2656 CrossRef CAS PubMed.
- W. Ma, J. Sun, W. Hongpu and R. Wang, Study on separation of acetonitrile and ethyl acetate mixture by extractive distillation, Appl. Chem. Ind., 2010, 39, 781–782 CAS.
- W. Kang, M. Li, Y. Wu, J. Cheng and R. Gao, Separation of ethyl acetate and n-heptane by Extractive Distillation, Mod. Chem. Ind., 2017, 37, 167–170 Search PubMed.
- J. Gu, X. You, C. Tao, J. Li, W. Shen and J. Li, Improved design and optimization for separating tetrahydrofuran–water azeotrope through extractive distillation with and without heat integration by varying pressure, Chem. Eng. Res. Des., 2018, 133, 303–313 CrossRef CAS.
- X. Li, Z. Yang, H. Sui, A. Jain and L. He, A hybrid process for oil-solid separation by a novel multifunctional switchable solvent, Fuel, 2018, 221, 303–310 CrossRef CAS.
|
This journal is © The Royal Society of Chemistry 2020 |