DOI:
10.1039/D0RA00227E
(Paper)
RSC Adv., 2020,
10, 9924-9933
Synthesis of caffeic acid sulfonamide derivatives and their protective effect against H2O2 induced oxidative damage in A549 cells†
Received
9th January 2020
, Accepted 27th February 2020
First published on 9th March 2020
Abstract
Exogenous antioxidants are considered as important therapeutic tools for oxidative stress associated disorders as they can regulate the redox state, which is associated with cell and organ function. Inspired by natural polyphenols, six new caffeic acid sulfonamide derivatives were synthesized by coupling sulfonamides to the backbone of caffeic acid with good yields. Their structure and lipophilicity were characterized by 1H nuclear magnetic resonance (NMR), 13C{1H} NMR, infrared spectroscopy (IR) and oil–water partition coefficient assay. Their free radical scavenging activity and antioxidant activity were assessed by DPPH assay and hydrogen peroxide (H2O2) induced oxidative stress in human lung carcinoma A549 cells. The oil–water partition coefficient results indicate that the conjugation of sulfonamides increases the lipophilicity of caffeic acid. The CASMD, CASDZ and CASN results show higher free radical scavenging effects compared with vitamin C. The derivatives do not show any inhibitory effect on the proliferation of A549 cells up to a concentration of 200 μM, except CASDZ which significantly inhibits the growth of A549 cells at a concentration of 200 μM. In addition, the obtained derivatives markedly attenuate H2O2 induced decrease of cell viability, inhibit the production of ROS and MDA, and promote the activities of superoxide dismutase (SOD), catalase (CAT) and glutathione peroxidase (GSH-Px). Besides, treatment of H2O2 stimulated A549 cells with caffeic acid sulfonamide derivatives further increases mRNA expression of NF-E2-related factor 2 (Nrf2) and its target genes, including heme oxygenase-1 (HO-1), NAD(P)H quinone dehydrogenase 1 (NQO1) and thioredoxin reductase 1 (TXNRD1). These results suggest that these new caffeic acid sulfonamide derivatives have higher lipophilicity and better antioxidant activities than the parent caffeic acid, and they might be able to control the antioxidant response in cells via the Nrf2 pathway.
1. Introduction
Reactive oxidants produced in normal cells in a controlled manner have many important physiological functions, such as fighting against infected pathogens, regulation of cell division or autophagy, controlling stress response and inflammation reaction. Nevertheless, bacterial or viral infections, heavy metal exposure, the use of cancer drugs or antibiotics, and excessive exercise can induce uncontrolled production of oxidants and disrupt the dynamic equilibrium between the production and elimination of reactive oxidants in the body.1 Excessive oxidants can oxidize cell membranes, enzymes, proteins, DNA and other substances; induce cell apoptosis or necrosis and cause damage to cells, tissues and organs.2,3 Perturbation of reactive oxidants equilibrium is considered an important factor that promotes the pathological development of diseases, such as cancer, arteriosclerosis, diabetes, hypertension, and Alzheimer's disease.4,5 In addition, the free radical senility theory proposes that aging is also caused by the accumulation of free radicals in cells and excessive oxidative damage in tissues.6,7 Numerous studies have shown that dietary supplements of antioxidants enhance the body's defence and help maintain oxidative dynamic equilibrium.8–10 Therefore, the development of free radical scavenging functional foods and drugs has been increasingly emphasized.
Caffeic acid and its derivatives, such as caffeic acid amides, caffeic acid esters, caffeic acid sugars and caffeic acid glycoside derivatives, are frequently found in various fruits and vegetables.11,12 Previous studies have suggested that caffeic acid and its derivatives are promising natural antioxidants and their antioxidant activity is mainly based on free radical scavenging, metal ion chelation, and inhibiting the oxidation of lipid peroxidation, catalase and low-density lipoprotein.13–15 Studies on the structure–activity relationships of a series of synthetic caffeic acid amides and caffeic acid esters found that the antioxidant activity of caffeic acid derivatives increased with the number of hydroxyl groups or catechol moieties.13 The presence of other hydrogen donor groups such as –NH or –SH can further enhance their antioxidant activity.13 Isolation of caffeic acid and its derivatives from the plant kingdom to fulfil the social requirement of food and medical antioxidant is inefficient and costly, thus great efforts have been focused on chemical synthesis of antioxidants with negligible toxicity.
Hydrogen peroxide (H2O2) is an important active oxygen molecule with relatively stable properties and is often used as a model chemical to induce oxidative stress injury in vitro. In order to explore new antioxidants and systematically evaluate their antioxidant activity, sulfonamides were coupled to the backbone of caffeic acid for the synthesis of new caffeic acid derivatives. The regulatory effect of obtained caffeic acid derivatives on the H2O2 induced oxidative stress were evaluated by cell survival rate, reactive oxygen species (ROS) and malondialdehyde (MDA) production, antioxidant enzyme activities and the messenger RNA (mRNA) levels of NF-E2-related factor 2 (Nrf2) pathway associated genes.
2. Results
2.1 Synthesis and characterization
Six new caffeic acid sulfonamide derivatives were obtained by coupling sulfanilamide (SN), sulfamethoxydiazine (SMD) and sulfadiazine (SDZ) to the backbone of caffeic acid with three step reactions according to the synthetic scheme in Fig. 1. After the fully characterized by melting points (mp) test, electrospray ionization mass spectrometry (EIS-MS) (Fig. S1–S6†), Fourier transfer-infrared spectrometry (FT-IR) spectrometry (Fig. S7–S12†), 1H nuclear magnetic resonance (NMR) (Fig. S13–S18†) and 13C{1H} NMR (Fig. S19–S24†) spectrometry, the structures of these six derivatives were confirmed and named as ACASMD, CASMD, ACASDZ, CASDZ, ACASN and CASN according to their molecule composition. Their characteristic information are listed below.
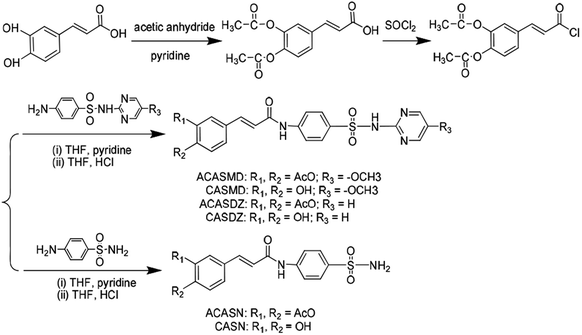 |
| Fig. 1 Synthesis route of caffeic acid sulfonamide derivatives. | |
ACASMD.
(Z)-4-(3-(4-(N-(5-Methoxypyrimidin-2yl)sulfamoyl)phenylamino)-3-oxoprop-1-enyl)-1,2-phenylene diacetate, white powder, ESI-MS: 525 [M − H]−; mp: 266–267 °C; 1H-NMR (300 MHz, dimethyl sulfoxide (DMSO)-d6): δ 11.43 (s, 1H, SO2–NH), 10.62 (s, 1H, –CO–NH), 8.30 (s, 2H, N–CH), 7.84 (d, J = 12.4 Hz, 4H, 4× Ar–H), 7.65 (d, J = 15.47 Hz, 1H, CO–CH), 7.57 (d, J = 8.42 Hz, 2H, Ar–H), 7.35 (d, J = 8.41 Hz, 1H, Ar–H), 6.80 (d, J = 15.7 Hz, 1H, Ar–CH), 3.79 (s, 3H, OCH3), 2.31 (d, J = 2.5 Hz, 6H, –CO–CH3). 13C{1H} NMR (75 MHz, DMSO) δ 168.69, 164.24, 151.45, 150.09, 145.09, 143.57, 142.81, 139.90, 134.93, 133.82, 129.26, 126.69, 124.74, 123.26, 119.11, 56.71, 20.81. IR (KBr, cm−1): 3058.72 (w, νN–H), 1771.47 (s, νC
O), 1683.65 (s, νRCH
CHR), 1635.01 (s, νAr), 1589.28 (s, νAr), 1506.06 (s, νAr).
CASMD.
(Z)-3-(3,4-Dihydroxyphenyl)-N-(4-(N-(5-methoxypyrimidin-2-yl)sulfamoyl)phenyl)acrylamide, light yellow powder, ESI-MS: 441 [M − H]−; mp: 274–276 °C; 1H-NMR (300 MHz, DMSO-d6): δ 11.40 (s, 1H, SO2–NH), 10.46 (s, 1H, –CO–NH), 9.55 (s, 1H, Ar–OH), 9.25 (s, 1H, Ar–OH), 8.30 (s, 2H, N–CH), 7.96–7.84 (d, J = 8.9 Hz, 4H, 4× Ar–H), 7.49 (d, J = 15.5 Hz, 1H, CO–CH), 7.04 (d, J = 1.7 Hz, 2H, Ar–H), 6.78 (d, J = 8.1 Hz, 1H, Ar–H), 6.53 (d, J = 15.5 Hz, 1H, Ar–CH), 3.47 (s, 3H, –CO–CH3). 13C{1H} NMR (75 MHz, DMSO) δ 165.03, 151.45, 150.08, 145.10, 143.77, 142.35, 134.49, 129.24, 126.44, 121.65, 118.92, 114.51, 56.70, 49.07. IR (KBr, cm−1): 3592.84 (s, νO–H), 3454.57 (s, νN–H), 1687.38 (s, νC
O), 1607.9 (m, νRCH=CHR), 1588.33 (s, νAr), 1538.48 (s, νAr), 1514.31 (w, νAr).
ACASDZ.
(Z)-4-(3-Oxo-3-(4-(N-pyrimidin-2ylsulfamoyl)phenylamino) prop-1-enyl)-1,2-phenylene diacetate, light yellow powder, ESI-MS: 495 [M − H]−; mp: 230–232 °C; 1H-NMR (300 MHz, DMSO-d6): δ 11.75 (s, 1H, SO2–NH), 10.63 (s, 1H, –CO–NH), 8.50 (d, J = 4.9 Hz, 2H, N–CH), 8.01–7.87 (d, J = 8.9 Hz, 4H, 4× Ar–H), 7.66 (d, J = 15.67 Hz, 1H, CO–CH), 7.60–7.57 (d, J = 8.9 Hz, 2H, Ar–H), 7.37 (d, J = 4.9 Hz, 1H, Ar–H), 7.03 (t, J = 4.9 Hz, 1H, CH–CH), 6.86 (d, J = 15.7 Hz, 1H, Ar–CH), 2.45 (d, J = 2.9 Hz, 6H, –CO–CH3). 13C{1H} NMR (75 MHz, DMSO) δ 168.68, 164.27, 158.80, 157.40, 143.57, 142.82, 139.93, 134.71, 133.82, 129.44, 126.68, 124.72, 123.24, 123.14, 119.10, 116.23, 20.77. IR data (KBr pellets, cm−1): 3043 (m, νN–H), 1771 (s, νC
O), 1683 (s, νC
O), 1635 (m, νRCH
CHR), 1590 (s, νAr), 1527 (m, νAr), 1505 (m, νAr).
CASDZ.
(Z)-3-(3,4-Dihydroxyphenyl)-N-(4-(N-pyrimidin-2-ylsulfamoyl) phenyl)acrylamide, light yellow powder, ESI-MS: 411 [M − H]−; mp: 289–291 °C; 1H-NMR (300 MHz, DMSO-d6): δ 10.48 (s, 1H, –CO–NH), 8.50 (s, 2H, Ar–OH), 7.94 (d, J = 7.4 Hz, 2H, N–CH), 7.87 (d, J = 8.9 Hz, 4H, 4× Ar–H), 7.43 (d, J = 15.5 Hz, 1H, CO–CH), 7.04 (d, J = 4.8 Hz, 2H, Ar–H), 6.93 (d, J = 4.5 Hz, 1H, Ar–H), 6.80 (d, J = 18.1 Hz, 1H, CH–CH), 6.53 (d, J = 15.5 Hz, 1H, Ar–CH). 13C{1H} NMR (75 MHz, DMSO) δ 165.01, 158.81, 157.43, 148.49, 146.10, 143.86, 142.33, 134.30, 129.38, 126.43, 121.63, 118.86, 118.17, 116.30, 114.50. IR (KBr, cm−1): 3486.34 (s, νO–H), 3386.56 (s, νN–H), 1664.28 (m, νC
O), 1593.05 (s, νAr), 1559.25 (s, νAr), 1534.83 (s, νAr).
ACASN.
(Z)-4-(3-Oxo-3-(4-sulfamoylphenylamino)prop-1-enyl)-1,2-phenylene diacetate, white powder, ESI-MS: 417 [M − H]−; melting point (mp): 242–244 °C; 1H-NMR (300 MHz, DMSO-d6): δ 10.59 (s, 1H, –CO–NH), 7.88–7.79 (d, J = 9.0 Hz, 4H, 4× Ar–H), 7.66 (d, J = 15.7 Hz, 1H, Ar–CH), 7.62 (d, J = 8.13 Hz, 2H, Ar–H), 7.58 (d, J = 8.1 Hz, 1H, Ar–H), 7.38 (d, J = 8.1 Hz, s, 2H, SO2–NH2), 6.86 (d, J = 15.7 Hz, 1H, CO–CH), 2.32 (d, J = 2.9 Hz, 6H, –CO–CH3). 13C{1H} NMR (75 MHz, DMSO) δ 168.70, 164.17, 143.54, 142.82, 139.76, 133.88, 126.65, 124.75, 123.37, 123.15, 20.82. IR (KBr, cm−1): 3328.82 (s, νN–H) (s), 3208.64 (s, νC
O), 1776.91 (s, νC
O), 1677.25 (m, νRCH
CHR), 1628.14 (s, νAr), 1536.42 (s, νAr), 1502.11 (s, νAr).
CASN.
(Z)-3-(3,4-Dihydroxyphenyl)-N-(4-sulfamoylphenyl)acrylamide, yellow powder, ESI-MS: 333 [M − H]−; mp: 298–300 °C; 1H-NMR (300 MHz, DMSO-d6): δ 10.42 (s, 1H, –CO–NH), 9.53 (s, 1H, Ar–OH), 9.24 (s, 1H, Ar–OH), 7.87–7.77 (d, J = 8.9 Hz, 4H, Ar–H), 7.49 (d, J = 15.5 Hz, 1H, Ar–CH), 7.26 (s, 2H, SO2–NH2), 6.04 (2H, Ar–H), 6.78 (d, J = 8.1 Hz, 1H, Ar–H), 6.54 (d, J = 15.6 Hz, 1H, –CO–CH). 13C{1H} NMR (75 MHz, DMSO) δ 164.94, 148.44, 146.10, 142.86, 142.17, 138.55, 126.48, 121.60, 118.29, 116.30, 114.48. IR (KBr, cm−1): 3333.94 (s, νO–H), 3326.56 (s, νN–H), 1670.83 (s, νC
O), 1576.84 (s, νAr).
2.2 Lipophilicity of caffeic acid sulfonamide derivatives
The ultraviolet (UV) spectrum of caffeic acid sulfonamide derivatives in n-octanol saturated PBS (0.3 μM) is shown in Fig. 2A. Two absorption peaks were observed from the spectrums of CASMD, CASDZ and CASN, while only one peak was observed from the spectrums of ACASMD, ACASDZ and ACASN. Protection of two hydroxyl groups on the benzene ring with acetyl groups resulted in the disappearance of the absorption peak at ∼340 nm but the increase of peak values at the wavelength of ∼316 nm. The maximum absorption wavelengths of tested compounds were determined as 316, 338, 316, 342, 316, 340 and 324 nm for ACASMD, CASMD, ACASDZ, CASDZ, ACASN, CASN and caffeic acid, respectively.
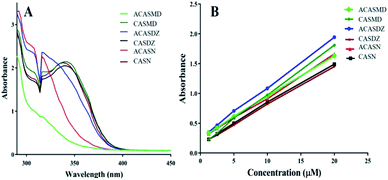 |
| Fig. 2 UV spectrum of caffeic acid sulfonamide derivatives (A) and standard curves prepared by measuring the absorbance at peak wavelength of series concentration of these derivatives in n-octanol saturated PBS (B). | |
Standard curves were prepared by measuring the UV absorbance of series concentrations of caffeic acid sulfonamide derivatives in the n-octanol saturated PBS at predefined maximum wavelength (Fig. 2B). A good linear relationship between the UV absorbance and their concentrations at a range of 0.312 μM to 5 μM for caffeic acid and a range of 1.25 μM to 20 μM for caffeic acid sulfonamide derivatives were obtained. The oil–water distribution coefficient was analyzed according to the standard curves and shown in Table 1. It was found that the conjugation of sulfonamides to the backbone of caffeic acid significantly increased its lipophilicity. ACASMD exhibited the strongest lipophilicity with an oil–water distribution coefficient (log
P) of 1.3109, followed by CASMD, ACASDZ and CASDZ showing coefficients higher than 0.5. Additional acetyl group increased the lipophilicity, as the lipophilicity of ACASMD, ACASDZ and ACASN was larger than respective derivatives without acetyl group protection. However, the parent caffeic acid exhibits a negative coefficient of −1.4171.
Table 1 Standard curves and distribution coefficients of caffeic acid sulfonamide derivatives
Compound name |
Slope |
Y-Intercept |
R
2
|
log P |
ACASMD |
0.06901 |
0.2571 |
0.9997 |
1.3109 |
CASMD |
0.08401 |
0.1343 |
0.999 |
0.7835 |
ACASDZ |
0.08415 |
0.2577 |
0.9993 |
0.7023 |
CASDZ |
0.06723 |
0.1587 |
0.9991 |
0.5910 |
ACASN |
0.07105 |
0.2304 |
0.999 |
0.1985 |
CASN |
0.06554 |
0.1469 |
0.9994 |
0.09379 |
Caffeic acid |
0.03432 |
0.1773 |
0.9994 |
−1.4171 |
2.3 DPPH scavenging capacity
The radical scavenging capacity of caffeic acid sulfonamide derivatives was evaluated by 1,1-diphenyl-2-picrylhydrazyl radical 2,2-diphenyl-1-(2,4,6-trinitrophenyl)hydrazyl (DPPH) assay and results are shown in Fig. 3 and Table 2. The radical scavenging rate increased with the increase in concentration of caffeic acid derivatives, exhibiting a dose-dependent manner (Fig. 3). Table 2 shows that the IC50 of these compounds are ranging from 39.37 μM to 798.00 μM. CASMD exhibited the lowest IC50 among tested chemicals, showing a value of 39.37 μM, which was lower than caffeic acid and vitamin C. The IC50 of CASMD, CASDZ and CASN was much lower than that of respective compounds with additional acetyl groups.
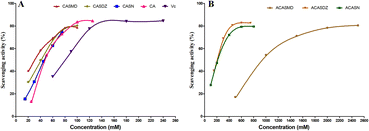 |
| Fig. 3 Radical scavenging capacity of caffeic acid sulfonamide derivatives, caffeic acid and vitamin C using DPPH assay. | |
Table 2 Radical scavenging capacity of caffeic acid sulfonamide derivatives
Compound name |
IC50 |
R
2
|
ACASMD |
798.00 |
0.9986 |
CASMD |
39.37 |
0.9895 |
ACASDZ |
259.7 |
0.9915 |
CASDZ |
45.55 |
0.9917 |
ACASN |
223.4 |
0.9957 |
CASN |
50.30 |
0.9983 |
Caffeic acid |
40.91 |
0.9955 |
Vitamin C |
91.04 |
0.9930 |
2.4
In vitro cytotoxicity of caffeic acid sulfonamide derivatives against A549 cells
The cytotoxicity of caffeic acid sulfonamide derivatives against human lung carcinoma cell line (A549) cells was evaluated using 3-(4,5-dimethyl-2-thiazolyl)-2,5-diphenyl-2-H-tetrazoliumbromide (MTT) assay and results are shown in Fig. 4. The viability of A549 cells was not significantly decreased when they were administrated with ACASMD, CASMD, ACASDZ, CASDZ, ACASN and CASN at the concentration of ≤200 μM or treated with CASMD at the concentration of ≤100 μM (Fig. 4B).
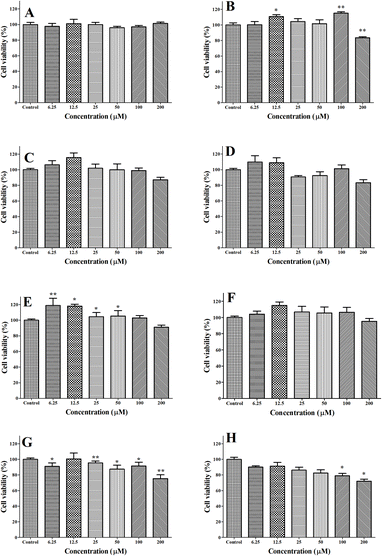 |
| Fig. 4 Cytotoxicity against A549 cells. (A) ACASMD, (B) CASMD, (C) ACASDZ, D) CASDZ, (E) ACASN, (F) CASN, (G) caffeic acid, (H) vitamin C. *, ** and *** indicate statistically different from control in a significant level of P < 0.05, P < 0.01, P < 0.001. (n = 4). | |
2.5 Effect of caffeic acid sulfonamide derivatives on H2O2 induced oxidative stress in A549 cells
2.5.1 Caffeic acid sulfonamide derivatives reduce H2O2 induced cell death.
H2O2 treatment induced a decrease in the viability of A549 cells in a dose-dependent manner (data not shown). 4 h exposure of cell to 800 μM H2O2 resulted in 40% decrease of the cell viability compared to control and this concentration was utilized for further experiments. The viability of cells treated with H2O2 and caffeic acid sulfonamide derivatives are shown in Fig. 5. 4 h treatment with 800 μM H2O2 resulted in around 40% drop of cell viability, while pretreatment with CASMD (≤200 μM), CASDZ (≤200 μM), CASN (≤200 μM), ACASMD (≤100 μM), ACASDZ (≤50 μM) and ACASN (≤50 μM) markedly attenuated the H2O2-induced decrease in cell viability (p < 0.05). However, caffeic acid and vitamin C at a concentration range of 3.125–100 μM could not prevent 800 μM H2O2 induced decrease in cell viability. Among the test concentrations, 6.25 μM of all caffeic acid sulfonamide derivatives exhibited promising effect on the inhibitory of H2O2-induced decrease in cell viability and this concentration was used for the following experiments.
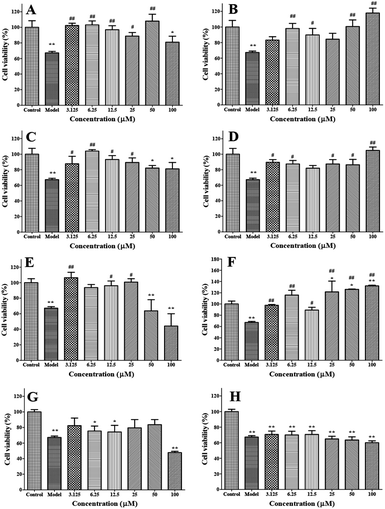 |
| Fig. 5 Viability of A549 cells pretreated with series concentration of caffeic acid sulfonamide derivatives for 24 h and then administrated with 800 μM of H2O2 for 4 h. (A) ACASMD, (B) CASMD, (C) ACASDZ, (D) CASDZ, (E) ACASN, (F) CASN, (G) caffeic acid, (H) vitamin C. * and # indicate statistical difference from control and H2O2 group, respectively (P < 0.05); ** and ## indicate statistical difference from control and H2O2 group, respectively (P < 0.01); *** and ### indicate statistical difference from control and H2O2 group, respectively (P < 0.001). (n = 4). | |
2.5.2 Caffeic acid sulfonamide derivatives inhibit ROS generation.
The levels of ROS in A549 cells were analyzed using dichloro-dihydro-fluorescein diacetate (DCF-DA) labels. From Fig. 6, bright green color and less cells was observed comparing to control group, which is consistent with the result of cell viability. The flow cytometry results indicated that H2O2-treatment induced the generation of ROS, showing a significantly higher level of ROS content comparing to that of control. Caffeic acid sulfonamide derivatives treatment strongly inhibited H2O2 induced increase of ROS levels (Fig. 6 and 7A).
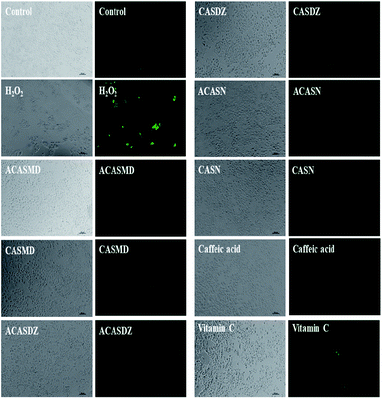 |
| Fig. 6 Optical and fluorescent microscopic images of A549 cells pretreated with 6.25 μM of caffeic acid sulfonamide derivatives for 24 h and then administrated with 800 μM of H2O2 for 4 h. | |
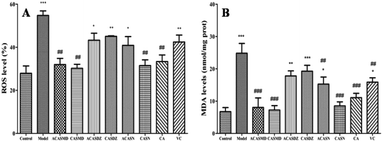 |
| Fig. 7 Effect of caffeic acid sulfonamide derivatives on the production of ROS (A) and MDA (B) in A549 cells pretreated with 6.25 μM of caffeic acid sulfonamide derivatives for 24 h and then administrated with 800 μM of H2O2 for 4 h. * and # indicate statistical difference from control and H2O2 group, respectively (P < 0.05); ** and ## indicate statistical difference from control and H2O2 group, respectively (P < 0.01); *** and ### indicate statistical difference from control and H2O2 group, respectively (P < 0.001). (n = 3). | |
2.5.3 Caffeic acid sulfonamide derivatives decrease MDA production.
The contents of MDA within the A549 cells were also examined. It was found that the levels of MDA were significantly elevated in H2O2-exposed cells, while caffeic acid sulfonamide derivatives at a concentration of 6.25 μM markedly decreased the generation of MDA (Fig. 7B).
2.5.4 Caffeic acid sulfonamide derivatives increase activities of anti-oxidative enzymes.
The effects of caffeic acid sulfonamide derivatives on the activities of endogenous antioxidant enzymes are shown in Fig. 8. It can be seen that the enzyme activities of superoxide dismutase (SOD), catalase (CAT) and glutathione peroxidase (GSH-Px) were significantly decreased after administrated with 800 μM H2O2 comparing to those of control. Caffeic acid sulfonamide derivatives pretreatment was able to enhance their activities, and except ACASDZ, all tested compounds significantly increase the activities of SOD (P < 0.05 or P < 0.001). In addition, CASN strongly upregulated the activities of CAT (P < 0.05), while CASMD dramatically enhanced the activity of GSH-Px (P < 0.05).
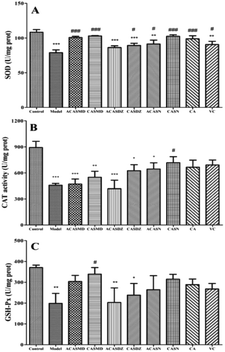 |
| Fig. 8 Effect of caffeic acid sulfonamide derivatives on the activities of endogenous antioxidant enzymes in A549 cells pretreated with 6.25 μM of caffeic acid sulfonamide derivatives for 24 h and then administrated with 800 μM of H2O2 for 4 h. (A) SOD, (B) CAT and (C) GSH-Px. * and # indicate statistical difference from control and H2O2 group, respectively (P < 0.05); ** and ## indicate statistical difference from control and H2O2 group, respectively (P < 0.01); *** and ### indicate statistical difference from control and H2O2 group, respectively (P < 0.001). (n = 3). | |
2.5.5 Caffeic acid sulfonamide derivatives upregulate critical gene expressions.
The mRNA expression of Nrf2 and Nrf2 target genes were further examined by reversed quantitative real time polymerase chain reaction (qRT-PCR) using primer sequences listed in Table 3 in order to find out the potential mechanisms accounting for the protective effects of caffeic acid sulfonamide derivatives against H2O2-induced oxidative stress. It was found that H2O2 activates the transcription of Nrf2 and Nrf2 target genes, including heme oxygenase-1 (HO-1), NAD(P)H quinone dehydrogenase 1 (NQO1) and thioredoxin reductase 1 (TXNRD1), and caffeic acid sulfonamide derivatives pretreatment further upregulated the mRNA expression of these genes in H2O2 treated A549 cells (Fig. 9).
Table 3 Sequence of the primers for qRT-PCR
Gene |
Forward primer (5′ → 3′) |
Reverse primer (5′ → 3′) |
Nrf2 |
GAGACAGGTGAATTTCTCCCAAT |
GGGAATGTGGGCAACCTGGG |
NQO1 |
AAGAAAGGATGGGAGGTGGT |
GCTTCTTTTGTTCAGCCACA |
TXNRD1 |
GGAACTAGATGGGGTCTCGG |
TCTTGCAGGGCTTGTCCTAA |
HO-1 |
AAGACTGCGTTCCTGCTCAAC |
AAAGCCCTACAGCAACTGTCG |
β-Actin |
GATCATTGCTCCTCCTGAGC |
ACTCCGCTTGCTGATCCAC |
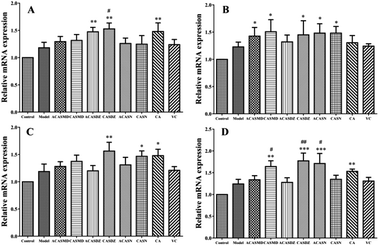 |
| Fig. 9 Relative mRNA expression levels of Nrf2 (A), HO-1 (B), NQO1 (C) and TXNRD1 (D) in A549 cells pretreated with 6.25 μM of caffeic acid sulfonamide derivatives for 24 h and then administrated with 800 μM of H2O2 for 4 h. * and # indicate statistical difference from control and H2O2 group, respectively (P < 0.05); ** and ## indicate statistical difference from control and H2O2 group, respectively (P < 0.01); *** and ### indicate statistical difference from control and H2O2 group, respectively (P < 0.001). (n = 3). | |
3. Discussion
Under pathological conditions, production of ROS is far more than their clearance by endogenic antioxidase, leading to oxidative stress. Excess ROS will induce oxidative damage to nucleic acid, protein and biomembrane of cells and cause cell injury and cell death. Free radicals, such as ROS, have been implicated in the pathogenesis of inflammation, cardiovascular diseases, cancer and ageing.16 Caffeic acid, an important phenolic acid extensively distributed in various plants, has the functions of anti-inflammation, anti-oxidation, promoting blood circulation and stopping bleeding, and delaying senility. Good antioxidative activities, potent analgesic activity, antimicrobial activities have been reported from many synthesized caffeic acid amide analogues and ester analogues.17–19 In this study, six new caffeic acid sulfonamide derivatives were synthesized by coupling sulfonamides including SN, SMD and SDZ, to the backbone of caffeic acid with or without the protection of hydroxyl groups with acetyl groups. The obtained derivatives exhibited stronger hydrophilicity than the parent form, which might help their penetration across biomembranes and thus easier to be absorbed into the circulation system and to function in target cells. This result is consistent with Ates's study on amide derivative of N-acetylcyteine (NACA).20
DPPH˙ assay is the simplest method to measure the ability of antioxidants to intercept free radicals. In this study, the DPPH˙ scavenging ability of caffeic acid sulfonamide derivatives decreased in the following order according to their hydrogen-donating ability: CASMD > caffeic acid > CASDZ > CASN > vitamin C > ACASN > ACASDZ > ACASMD. Increasing the number of hydroxyl or catechol groups can enhance the radical scavenging activity of chemicals, as the scavenging activity of CSAN, CASMD and CASDZ were much stronger than those of respective compounds with additional acetyl group (ACSAN, ACASMD and ACASDZ), this result is consistent with previous reports.13
H2O2 can directly oxidize the proteins and lipids in cell membrane. It is also capable of diffusing throughout the mitochondria and across cell membranes and produce many types of cellular injury, thus it has been considered as one of the most important stimulators for the establishment of oxidative stress cell models.21,22 Exogenous antioxidants can tackle the oxidative damage to protect cell and organ functions.23 In this study, oxidative stress was established in A549 cells using H2O2 stimulation to evaluate the antioxidative activities of synthesized caffeic acid sulfonamide derivatives. It was found that the proliferation of A549 cells was significantly inhibited by H2O2, but the inhibition of H2O2 on the survival rate of A549 cells was significantly reduced by the treatment of caffeic acid sulfonamide derivatives, suggesting that caffeic acid sulfonamide derivatives was able to alleviate H2O2 induced cell damage.
Pathogen infection and exposure to xenobiotics, drugs, heavy metals and ionizing radiation usually generate large amount of ROS, and these radicals induced oxidative stress may profoundly affect the survival and evolution of all living organisms.1 Upon oxidative stress, oxygen radicals attack unsaturated fat in biofilms, triggering lipid peroxidation reactions and producing lipid peroxidation products such as MDA and keto groups. Lipid peroxidation not only converts reactive oxygen species into reactive chemicals, which are not free radical like decomposition products, but also intensify cell damages via chain or branched chain reactions. Antioxidants can protect against free radicals, and free radical-induced lipid peroxidation and DNA damage.24 In this study, H2O2 stimulation induced oxidative stress in A549 cells, representing with significantly increased ROS levels and MDA production. Caffeic acid sulfonamide derivatives treatment inhibited the production of ROS and MDA, suggesting that they were potential candidates to protect cells from free radicals.
Reactive oxidants are counterbalanced by intricate antioxidants including reduced glutathione (GSH), vitamins C and E, and urate, and endogenous antioxidant enzymes such as SOD, CAT and GSH-Px.16 SOD catalyze the disproportionation reaction of peroxo-anion to scavenge free radicals. CAT, which accounts for about 40% of the total catalase system, catalyze the decomposition of hydrogen peroxide to produce water and oxygen. GSH-Px, an important peroxide enzyme that widely exists in the body, catalyze the reaction of GSH with peroxide to produce nontoxic hydroxyl compounds and oxidized glutathione (GSSG).16 The activity of antioxidant enzymes in the body is a direct reflection of the level of dynamic equilibrium regulation in cells under oxidative stress. When the stimulation of external oxidative stress source is stronger than the self-regulated range, damage of cells and tissues by oxidative stress will occur. In order to resist the injury from ROS and restore the redox balance, endogenous antioxidant enzymes (SOD, CAT and GSH-Px) are consumed in large quantities. As a result, the oxidative defence system is haywire. Hence, when A549 cells were stimulated by H2O2, the whole antioxidant defence system was severely damaged, and corresponding activities of SOD, CAT and GSH-Px in A549 cells significantly reduced. However, the activities of antioxidant enzymes were considerably increased after 24 hours incubation with caffeic acid sulfonamide derivatives, indicating that these compounds could reduce the consumption of endogenous antioxidant enzymes by H2O2 and alleviate the injury of oxidative stress. This above observation was consistent with the previous finding that antioxidants attenuate H2O2-induced cell damage by reducing lipid peroxidation products and elevating the level of GSH-Px and SOD activities.25 Further, the antioxidative ability of rats was significantly improved after oral administration of grape seed polyphenol, which was superior to vitamin C and other common antioxidants.26
Besides radical scavenging, oxidative stress and antioxidant action also includes the modulation of cell signaling pathway and expression of genes associated with cellular antioxidative capacity.27 Nrf2-mediated antioxidative response pathway controls the expression of antioxidative response element-dependent genes, including HO-1, NQO1 and TXNRD1, three important antioxidation and detoxification enzymes.28 This pathway has been considered as the primary cellular defence mechanism against the cytotoxic effects of oxidative stress, and the knockout of Nrf2 genes induce higher susceptibility to chemical toxicity and disease conditions associated with oxidative pathology.29,30 Activation of Nrf2 pathway and increased mRNA levels of HO-1, NQO1 and TXNRD1 are frequently reported under oxidative stress conditions.31,32 Antioxidant treatment significantly increased the cell viability, SOD activity and GSH level, which may function via the modulation of Nrf2 pathway.33–35 In this study, the mRNA expressions of Nrf2 and associated antioxidation and detoxification enzymes (HO-1, NQO1 and TXNRD1) were upregulated upon H2O2 stimulation, while their expression were further upregulated by caffeic acid sulfonamide derivative pretreatment.
4. Materials and methods
4.1 Chemicals
Caffeic acid, DPPH and MTT were obtained from Sigma-Aldrich, USA. SN, SMD and SDZ were purchased from Shenzhen Remote Technology Development Co. High glucose Dulbecco's Modified Eagle's Medium (DMEM), fetal bovine serum (FBS), penicillin sodium and streptomycin (PS) were purchased from Gibco, USA. Other chemicals and reagents were supplied by Xilong Scientific Co. All chemicals were commercially available and used as received.
4.2 Synthesis and characterization of caffeic acid sulfonamide derivatives
Caffeic acid sulfonamide derivatives were prepared by coupling SN, SMD or SDZ to the backbone of caffeic acid according to the synthetic scheme in Fig. 1. Firstly, the hydroxyl groups on caffeic acid were protected by acyl groups by reacting with acetic anhydride and sulfoxide chloride (SOCl2) to obtain the intermediate of (E)-4-(3-chloro-3-oxoprop-1-en-1-yl)-1-2-phenlene diacetate (CPD). Briefly, 17.9 g of caffeic acid and 4 mL of pyridine were added into 80 mL acetic anhydride and stirred at room temperature for 24 h. The mixture was then concentrated by rotary evaporation to obtain primrose yellow oily product which were then mixed with 100 mL distilled water and placed in room temperature for 12 h to produce large amount of white precipitate. The precipitate was vacuum filtered and ∼21.2 g diacetyl caffeic acid was obtained. The diacetyl caffeic acid (5.0 g) was reacted with 10 mL of SOCl2 by refluxing at 60 °C for 5 h. Excess SOCl2 was removed by distillation at 50 °C under reduced pressure and the intermediate of (E)-4-(3-chloro-3-oxoprop-1-en-1-yl)-1-2-phenlene diacetate (CPD) was obtained. Obtained CPD was dissolved in 25 mL of tetrahydrofuran (THF) and excess SN, SMD or SDZ and 5 mL of pyridine was added to the above mixture. The mixture was stirred in ice-bath for 2 hours and then at room temperature for another 24 hours. Upon completion, excess THF and pyridine was removed by vacuum evaporation. The obtained crude product was washed by distilled water and purified by recrystallization in a THF–methanol system. Finally, one kind of light yellow powder and two kinds of white powders denoted as ACASMD, ACASN and ACASDZ were obtained. Acetyl groups protecting hydroxyl groups on the benzene ring of ACASN, ACASMD and ACASDZ (5 g) were removed by reacting with hydrochloric acid (6 mL, 38%) in THF (20 mL) at 55 °C for 4 hours to obtain CASN, CASMD and CASDZ. The purity of final products was higher than 99.0% with the yield of 58%–89%.
The melting points of obtained caffeic acid sulfonamide derivatives were measured by XT-4 micro melting apparatus. EIS-MS was recorded by Shimadzu LC-MS 2010A. 1H-NMR and 13C{1H} NMR spectrums were recorded using a Bruker Advance III 300 at 25 °C with frequencies of 400 and 125 MHz, respectively. FT-IR spectrums were obtained by a Nicolet 380 spectrophotometer. The UV absorption spectrums were determined on an UV-2600 (Shimadzu, Japan).
4.3 Determination of the lipophilicity of caffeic acid sulfonamide derivatives
The lipophilicity of caffeic acid derivatives was calculated by n-octanol–water distribution coefficients. Briefly, series concentrations of caffeic acid sulfonamide derivatives in PBS (10 mM, pH 7.4) saturated n-octanol were prepared and their UV absorbance were measured at the pre-determined wavelength to plot the standard curves. 8 μL of caffeic acid sulfonamide derivatives (5 μM in DMSO) was mixed with 2 mL of n-octanol saturated PBS and PBS saturated n-octanol. The mixture was thoroughly mixed. The obtained emulsion was centrifuged at 4000 rpm for 5 min to separate the aqueous and organic phases. The UV absorption of caffeic acid sulfonamide derivatives in the aqueous and organic phases were measured and their concentrations were calculated using obtained standard curves. log
P was defined as the logarithm of the ratio between the concentration of the compound in the organic and aqueous phases, and calculated as log(Corganic/Caqueous).36
4.4 Quenching of DPPH radical
All tested chemicals were dissolved in DMSO and diluted with ethanol. To evaluate their DPPH scavenging capacity, 3.94 mg DPPH was dissolved in 100 mL of anhydrous ethanol and 2 mL of this solution was mixed with series concentrations of caffeic acid derivatives. Vitamin C was used as control. The mixture was kept at room temperature in dark for 30 min and the absorbance was measured at 519 nm using UV spectrophotometer. The DPPH scavenging activity was calculated as percentage of (Acontrol − Asample)/Acontrol.
4.5
In vitro cytotoxicity assay
Human lung carcinoma A549 cells were purchased from the cell bank of the Chinese Academy of Science (Shanghai, China). Cells were cultured in completed DMEM medium supplemented with 10% fetal bovine serum, 100 U mL−1 of penicillin and 100 U mL−1 streptomycin, and maintained in a humidified atmosphere at 37 °C with 5% CO2.
The cytotoxicity of synthesized compounds against A549 cells were tested using MTT assay. Briefly, cells were seeded in 96-well plates with 5 × 103 cells per well and incubated overnight. Then cells were administrated with series concentrations of caffeic acid sulfonamide derivatives ranging from 6.25–200 μM. 48 h later, 100 μL of MTT (0.5 mg mL−1 in DMEM) was added into each well and incubated for another 4 h. The supernatant was carefully removed, and the purple crystals were dissolved in 100 μL DMSO. The absorbance at 490 nm were measured using a multi-functional microplate reader and cell viability was calculated as percentage of (ODtest − ODblank)/(ODcontrol − ODblank).
4.6 Effect of caffeic acid sulfonamide derivatives on H2O2 induced oxidative stress
A549 cells were divided into control group (cells without drug and H2O2 treatment), H2O2 group (cells exposed to 800 μM H2O2 for 4 h) and tested groups (cells pretreated with caffeic acid sulfonamide derivatives for 24 h and then exposed to 800 μM H2O2 for 4 h). Cells were subjected to different treatments and then serial analysis were carried out, including cell viability, cell morphology, oxidative product production, anti-oxidative enzyme activities, and mRNA expressions of oxidative reaction associated genes.
4.6.1 Cell viability.
Viability of A549 cells treated with/without H2O2 and caffeic acid sulfonamide derivatives was measured following the same procedure as 4.5.
4.6.2 ROS generation.
After cell treatment in 24-well plates, the supernatant was discarded and cells were carefully washed with PBS (pH 7.2). Then cells were harvested, transferred to a 2.0 mL centrifuge tube, and centrifuged at 1500 rpm for 5 min. The precipitate was washed with serum-free DMEM twice and incubated with 10 μM of DCFH-DA (Sigma, USA) in serum free DMEM at 37 °C for 30 min in dark. Cells were further washed with serum-free DMEM twice and resuspended in serum-free DMEM for flow cytometry detection on a FACSAria II flow cytometer (BD, USA). In addition, cells cultured in 48-well plates labeled with DCFH-DA were imaged with an inverted microscope (Nikion, Japan).
4.6.3 MDA production.
After H2O2 and caffeic acid sulfonamide derivatives treatment, cells were harvested and ultrasonicated on ice to release MDA from the cells. After centrifugation at 3000 rpm for 10 min at 4 °C, the MDA levels in the supernatant were tested using commercial assay kits (Jiancheng Bioengineering Institute, China).
4.6.4 Intracellular anti-oxidative enzyme activities.
Cells treated in accordance with the experimental design in 6-well plates were harvested and disrupted ultrasonically on ice. After centrifugation at 3000 rpm for 10 min at 4 °C, the supernatants were used for the measurement of SOD, GSH-Px and CAT levels using commercial assay kits (Jiancheng Bioengineering Institute, China) according to the manufacturer's instructions.
4.6.5 Quantitative real time polymerase chain reaction analysis of gene activation.
Total RNA was extracted from treated A549 cells using Total RNA Extraction Reagent (Vazyme, China) and was reverse transcribed into cDNA using the HiScript III RT SuperMix for qPCR kit (Vazyme, China) following the manufacturer's instructions. A SYBR-Green I PCR kit (Vazyme, China) was used to amplify cDNA using the following conditions: initial denaturation at 95 °C for 30 s, followed by 35 amplification cycles at 95 °C for 10 s, 55 °C for 10 s, 72 °C for 15 s, and a final extension at 72 °C for 10 min. β-Actin was used as an internal reference gene. Relative values for mRNA levels were calculated using the following formula: fold change = 2−ΔΔCq. The primers used for each gene were listed in Table 3.
4.7 Statistical analysis
Data were presented as means ± SD. Statistical significance was determined using one way analysis of variance (ANOVA) followed by LSD post hoc test.
5. Conclusions
In conclusion, six new caffeic acid sulfonamide derivatives have been synthesized with simple reactions and high yield under mild conditions. They were fully characterized by various physicochemical and spectroscopic methods. The results of oil–water partition coefficient and DPPH scavenging assays indicate that these derivatives have high lipophilicity and strong radical scavenging activities. In vitro studies using H2O2 induced oxidative stress cell models suggest that the caffeic acid sulfonamide derivatives are able to protect cells from H2O2 induced oxidative stress possibly by regulating the Nrf2 pathway. However, further confirmation of the Nrf2 translocation from the cytoplasm to the cell nucleus by western blot would convince the regulation of Nrf2 signaling pathway. In vivo studies on the protective effect of the derivatives with strong antioxidative capability screened from this in vitro study are required to evaluate their potential biomedical applications on the treatment of oxidative stress associated disease.
Funding
This work was supported by the Guangxi Natural Science Foundation [grant number 2016GXNSFBA380053]; and the National Natural Science Foundation of China [grant number 21362001].
Conflicts of interest
The authors declare no competing financial interest.
Acknowledgements
We thank Dr Palanisamy Kannan for the language help on the preparation of this manuscript.
References
- J. W. Hamilton and K. E. Wetterhahn, Mol. Carcinog., 1989, 2, 274–286 CrossRef CAS PubMed.
- Y. C. Shi and T.-M. Pan, Appl. Microbiol. Biotechnol., 2012, 94, 47–55 CrossRef CAS PubMed.
- V. Sosa, T. Moline, R. Somoza, R. Paciucci, H. Kondoh and L. L. ME, Ageing Res. Rev., 2013, 12, 376–390 CrossRef CAS PubMed.
- M. O. Parat, T. T. T. Nguyen, P. N. Shaw and A. K. Hewavitharana, Mol. Nutr. Food Res., 2013, 57(1), 153–164 CrossRef PubMed.
- C. Y. Chang, C.-Y. Shen, C.-K. Kang, Y.-P. Sher, W. H.-H. Sheu, C.-C. Chang and T.-H. Lee, Toxicol. Appl. Pharmacol., 2014, 279, 351–363 CrossRef CAS PubMed.
- V. K. Koltover, Curr. Aging Sci., 2017, 10, 12–17 CrossRef CAS PubMed.
- M. V. Clement and L. Luo, Proteomics, 2019, e1800400, DOI:10.1002/pmic.201800400.
- Q. Song, Y. Zhao, Q. Li, X. Han and J. Duan, Biomed. Pharmacother., 2019, 122, 109690 CrossRef PubMed.
- Y. Fu, L. Jiang, W. Zhao, X.-N. Meng, S. Huang, J. Yang, T. Hu and H. Chen, Sci. Rep., 2017, 7, 8676 CrossRef PubMed.
- H. Chen, J. Yang, Y. Fu, X. Meng, W. Zhao and T. Hu, BMC Complementary Altern. Med., 2017, 17, 244 CrossRef PubMed.
- A. Colonnello, G. Aguilera-Portillo, L. C. Rubio-Lopez, B. Robles-Banuelos, E. Rangel-Lopez, S. Cortez-Nunez, Y. Evaristo-Priego, A. Silva-Palacios, S. Galvan-Arzate, R. Garcia-Contreras, I. Tunez, P. Chen, M. Ashner and A. Santamaria, Neurotoxic. Res., 2019, 37, 326–337 CrossRef PubMed.
- K. D. Pantoja Pulido, A. J. Colmenares Dulcey and J. H. Isaza Martinez, Food Chem. Toxicol., 2017, 109, 1079–1085 CrossRef CAS PubMed.
- S. Son and B. A. Lewis, J. Agric. Food Chem., 2002, 50, 468–472 CrossRef CAS PubMed.
- C. C. Hung, W. Tsai, L. Y. Kuo and Y. Kuo, Bioorg. Med. Chem., 2005, 13, 1791–1797 CrossRef CAS PubMed.
- Y. Li, Y. Zhu, R. Liang and C. Yang, Int. J. Cosmet. Sci., 2017, 39, 402–410 CrossRef CAS PubMed.
- S. Reuter, S. C. Gupta, M. M. Chaturvedi and B. B. Aggarwal, Free Radicals Biol. Med., 2010, 49, 1603–1616 CrossRef CAS PubMed.
- P. Rajan, I. Vedernikova, P. Cos, D. Vanden Berghe, K. Augustyns and A. Haemers, Bioorg. Med. Chem. Lett., 2001, 11, 215–217 CrossRef CAS PubMed.
- Y. Jung, T. Kang, J. Yoon, B. Joe, H. Lim, C. Seong, W. Park, J. Kong, J. Cho and N. Park, Bioorg. Med. Chem. Lett., 2002, 12, 2599–2602 CrossRef CAS PubMed.
- J. Fu, K. Cheng, Z.-m. Zhang, R.-q. Fang and H.-l. Zhu, Eur. J. Med. Chem., 2010, 45, 2638–2643 CrossRef CAS PubMed.
- B. Ates, L. Abraham and N. Ercal, Free Radical Res., 2008, 42, 372–377 CrossRef CAS PubMed.
- D. Chavarria, C. Fernandes, B. Aguiar, T. Silva, J. Garrido, F. Remiao, P. J. Oliveira, E. Uriarte and F. Borges, Molecules, 2019, 24, 1–16 CrossRef PubMed.
- W. Jin, X. Zhu, F. Yao, X. Xu, X. Chen, Z. Luo, D. Zhao, X. Li, X. Leng and L. Sun, Biomed. Pharmacother., 2020, 121, 109676 CrossRef CAS PubMed.
- B. E. Bilgic, S. Ayla, G. Tunali, K. Sofuoglu, A. A. Ozdemir, G. Tanriverdi, S. Ozdemir, B. C. Soner, B. Ozturk, S. Karahuseyinoglu, E. G. Aslan and I. Seckin, Acta Histochem., 2018, 120, 117–121 CrossRef PubMed.
- D. Bagchi, M. Bagchi, S. Stohs, D. Das, S. Ray, C. Kuszynski, S. Joshi and H. Pruess, Toxicology, 2000, 148, 187–197 CrossRef CAS PubMed.
- H. Hong and G. Liu, Planta Med., 2004, 70, 427–431 CrossRef CAS PubMed.
- T. Koga, K. Moro, K. Nakamori, J. Yamakoshi, H. Hosoyama, S. Kataoka and T. Ariga, J. Agric. Food Chem., 1999, 47, 1892–1897 CrossRef CAS PubMed.
- F. Nadia, V. Giuseppa, S. Antonella, C. Santa, L. G. Enrico, C. Pietro, D. P. Angela and N. Michele, J. Evidence-Based Complementary Altern. Med., 2015, 1–14 Search PubMed.
- K. Eri, S. Takafumi and Y. Masayuki, Oxid. Med. Cell. Longevity, 2013, 2013, 1–7 Search PubMed.
- Q. Ma and X. He, Pharmacol. Rev., 2012, 64, 1055–1081 CrossRef CAS PubMed.
- H. Motohashi and M. Yamamoto, Trends Mol. Med., 2004, 10, 549–557 CrossRef CAS PubMed.
- G. Szklarz, Annu. Rev. Pharmacol. Toxicol., 2013, 53, 401 CrossRef PubMed.
- P. K. Leong, P. Y. Chiu, N. Chen, H. Leung and K. M. Ko, Free Radical Res., 2011, 45, 483–495 CrossRef CAS PubMed.
- W. Ying, Z. Li, Y. qing, L. Mai and T. Mei, Appl. Physiol., Nutr., Metab., 2018, 44, 1–6 Search PubMed.
- E. Jiang, H. Li, C. Yu, C. Yu, S. Jing, H. Sun, C. Wang, X. Fan, J. Chen and S. Wang, Neuroreport, 2016, 26, 360–366 CrossRef PubMed.
- R. Jia, H. Zhang, Z. Yang, H. Zhao, F. Liu, H. Wang, M. Miao, Q. Wang and Y. Liu, Int. Immunopharmacol., 2017, 53, 11–16 CrossRef CAS PubMed.
-
D. E. K. Li, ADME to Toxicity Optimization, 2016, DOI:10.1080/02103702.1987.10822155.
Footnote |
† Electronic supplementary information (ESI) available. See DOI: 10.1039/d0ra00227e |
|
This journal is © The Royal Society of Chemistry 2020 |
Click here to see how this site uses Cookies. View our privacy policy here.