DOI:
10.1039/D0RA00184H
(Paper)
RSC Adv., 2020,
10, 34928-34937
Study on mechanism of elemene reversing tumor multidrug resistance based on luminescence pharmacokinetics in tumor cells in vitro and in vivo
Received
8th January 2020
, Accepted 28th June 2020
First published on 21st September 2020
Abstract
While elemene (ELE) can reverse tumor multidrug resistance (MDR), the mechanisms for ELE reversing MDR remain unclear. Numerous studies have suggested that the efflux functionality of ATP-binding cassette (ABC) transporters, not their quantity, is more relevant to tumor MDR. However, no appropriate methods exist for real-time detection of the intracellular drug efflux caused by ABC transporters in vitro, especially in vivo, which hinders the examination of MDR reversal mechanisms. This study directly investigates the correlation between efflux functionality of ABC transporters and MDR reversal via ELE, using D-luciferin potassium salt (D-luc) as the chemotherapeutic substitute to study the intracellular drug efflux. Here, a luciferase reporter assay system combined with bioluminescence imaging confirmed that the efflux of D-luc from MCF-7/DOXFluc cells in vitro and in vivo was significantly reduced by ELE and when combined with Doxorubicin (DOX), ELE showed a synergistically anti-tumor effect in vitro and in vivo. Additionally, the luminescence pharmacokinetics of D-luc in MCF-7/DOXFluc cells and pharmacodynamics of the combined ELE and DOX in vivo showed a great correlation, implying that D-luc might be used as a probe to study ABC transporters-mediated efflux in order to explore mechanisms of traditional Chinese medicines reversing MDR.
1. Introduction
Cancer is regarded as one of the most serious diseases, and chemotherapy remains a key treatment method for different stages and many types of cancer. However, the efficiency of clinical therapy remains poor and unsatisfactory.1,2 One of the most prominent obstacles to universally effective chemotherapy remains tumor multidrug resistance (MDR), which is the intrinsic or acquired resistance of tumor cells to a wide range of drugs with diverse structures and functions.3,4
Numerous studies have validated ATP-binding cassette (ABC) transporters-such as ABCB1 (also called P-glycoprotein), ABCC1 (also called MRP1), and ABCG2 (also called BCRP)-as the main players for MDR to distinct anti-tumor drugs.5–9 Chemical MDR reversal agents-such as verapamil,10 cyclosporin A,11 and tamoxifen12 have limited application because of their high toxicities, side effects, and unobvious reversal effect when combined with chemotherapies.13 Comparatively, some traditional Chinese medicines (TCMs) have shown a significant MDR reversal effect with fewer side effects.14–16 In our former studies, we found that several TCMs-such as elemene (ELE), a noncytotoxic broad-spectrum anti-tumor drug extracted from Curcuma zedoaria Roscoe have great reversal effect in MDR cells. However, these TCMs do not always reduce the expression of MDR-related ABC transporters.17 Interestingly, some TCMs decrease the expression of ABC transporters in tumor cells in vitro but have less or no effect in vivo or vice versa. That means there may be no direct correlation between the quantity of ABC transporters in vitro and in vivo. Do these TCMs directly affect the functionality of ABC transporters and then changing the drug efflux from tumor cells? Is there a direct correlation between the functionality of ABC transporters in vitro and in vivo? Regretfully, the mechanisms of these TCMs reversing MDR remain unclear today. Thus, identifying a method to examine the functionality of ABC transporters in vitro, especially in vivo, is of particular importance to reversing MDR.
In this paper, D-luciferin potassium salt (D-luc), a specific substrate of ABC transporters, served as the substitute for chemotherapeutics in studying the effect of intracellular drug efflux treated by ELE. As both D-luc and most small molecule chemotherapeutics are substrates of ABC transporters, their uptake into or efflux from tumor cells should be very similar.18 A luciferase reporter assay system combined with bioluminescence imaging (BLI) technology was applied to examine the uptake or efflux fluorescence kinetics of D-luc in tumor cells in real time. When D-luc enters tumor cells, it is catalyzed by luciferases based on energy-dependent reactions in form of visible lights emitting photons under the specific wavelengths, which can be quantitatively monitored in real-time by BLI.19 A BLI-based assay also allows for noninvasive real-time monitoring of biological processes in many disease models, such as cancers.20 Additionally, the assay's high signal-to-noise and low background allow for accurately examining cells that stably express a small amount of luciferase, requiring as little as 10−19 mol of luciferase.21 Furthermore, the factors that determine the BLI intensity involve the oxygen and substrate concentration, the presence of magnesium ions, and the expression level of luciferase.22 Several papers have confirmed that ABC transporters impact readout intensity of BLI in intact living cells by greater efflux of substrates such as D-luc, which is a vital aspect of several potential confusing factors affecting BLI values.23 Since the luciferase reporter is only constructed in tumor cells,24,25 the readout should only show the D-luc in tumor cells, even in vivo. Combining pharmacokinetics (PK) modeling with BLI, a more exact set of PK parameters would be obtained, which has been confirmed in the former study.26 Thus, the fluorescence PK of D-luc in vitro and in vivo should be highly related and could be used as an effective probe to reflect drug efflux from tumor cells.
Ultimately, this research aimed to study the mechanisms of ELE reversing breast cancer MDR via evaluating the functionality of ABC transporters based on the PK of D-luc in vitro and in vivo in MCF/DOXFluc cells. Then, ELE combined with DOX was injected into on MCF/DOXFluc cells and tumor-bearing nude mice to study the effects. Finally, the following relationships were analyzed by Pearson correlation analysis: (1) between the PK of D-luc in vitro and in vivo, (2) between the PK of D-luc in vitro and the PD of the combined ELE and DOX in vitro, and (3) between the PK of D-luc in vivo and the PD of the combined ELE and DOX in vivo.
2. Materials and methods
2.1 Materials
D-luc was provided by Thermo Fisher Scientific (USA). ELE (purity ≥ 98%) was obtained from Dalian Holley Kingkong Pharmaceutical Company (Dalian, China). Doxorubicin (DOX) was obtained from Hisun Pharmaceutical Company (Hangzhou, China). The BCA protein quantitation kit and RIPA lysis buffer were provided by Beyotime Biotechnology (Shanghai, China). Verapamil and Ko143 were obtained from Apexbio (Houston, USA). Fetal bovine serum (FBS) was provided by Bioind (Israel). Phosphate-buffered saline (PBS) was purchased from Northend Company (Hangzhou, China). Penicillin/streptomycin (P/S), Dulbecco's Modified Eagle's Medium (DMEM), and 0.25% trypsin–EDTA were obtained from Gibco (USA). All cell culture supplies (culture flask, Petri dish, well plates) were purchased from Corning Incorporated (USA). Matrigel was provided by BD Biosciences (USA). Thiazolyl blue tetrazolium bromide (MTT) was obtained from Sigma-Aldrich (USA). All chemicals for the experiment were analytical reagent grade.
2.2 Cell line and experimental animals
The MCF-7/DOX cells (DOX-resistant human breast cancer cells) were kindly presented by Sichuan University (Sichuan, China) and routinely grew in DMEM medium containing 1% P/S and 10% FBS and incubated at 5% CO2 and 37 °C. The MCF-7/DOXFluc cell line, which stably expresses the firefly luciferase reporter gene, was constructed by lentivirus infection in our former studies.26
The nude mice (aged 4–5 weeks, female, BALB/c) were provided by Slake Laboratory Animal Company (Shanghai, China) and bred by Zhejiang Chinese Medical University Laboratory Animal Research Center (Hangzhou, China). Then, to establish the xenograft models, 6 × 107 of MCF-7/DOXFluc cells and Matrigel at a ratio of 1
:
1 suspended in 200 μL of PBS were inoculated subcutaneously into the right flank of mice. Briefly, all animal experiments were performed in accordance with the National Institutes of Health Guidelines on Laboratory Research and approved by the Animal Care Committee at Zhejiang Chinese Medical University.
2.3 In vitro cytotoxicity studies
The cytotoxicity of MCF-7/DOXFluc cells and the effect of ELE reversing MDR were evaluated using a standard MTT assay. MCF-7/DOXFluc cells were seeded in 96-well plates at the density of 7 × 103 cells per well for 24 h. Subsequently, cells were incubated with different samples, which were either exposed to DOX alone or in combination with ELE at a ratio of 1
:
3, to check the cell viability and proliferation. After the treating period and washing the cells with PBS, fresh medium containing MTT (0.5 mg mL−1) was added to each well, and the plates were maintained at 37 °C for another 4 h. Briefly, the MTT solution was completely replaced by 150 μL of DMSO to dissolve the formed formazan salt. The absorbance of each well was detected at 570 nm by a Bio-Tek microplate reader. Each concentration was tested for at least 3 wells, and the data were performed as mean ± SD. Then, the half maximal inhibitory concentration (IC50) values were presented by GraphPad Prism V6.01 software. Each sample was repeated in triplicate. Cell viability (%) was presented using the following equation: [(ODsample − ODblank control)/(ODpositive control − ODblank control)] × 100. Then, the combination index (CI) analysis of combination ELE and DOX was examined by CompuSyn software.
2.4 In vivo anti-tumor efficacy and systemic toxicity
Xenografts of human breast cancer were used as described in Section 2.2. MCF/DOXFluc tumor-bearing nude mice were used to examine tumor inhibition efficacy of ELE at different concentrations in combination with DOX. The mice were divided randomly into 6 groups (n = 6) and treated with saline, ELE (10 mg kg−1, 25 mg kg−1, i.p.), DOX (5 mg kg−1, i.v.), and DOX (5 mg kg−1, i.v.) combined with ELE (10 mg kg−1, 25 mg kg−1, i.p., given 1 h before DOX treatment). The DOX was given twice a week for 4 weeks, and ELE was given every other day. Additionally, body weights were weighed and recorded twice a week. The tumor volume was recorded twice a week and then calculated using the following equation: volume (V) = length (L) × width2 (W2) × 1/2. At the end of treatment, the major organs and tumors were collected for hematoxylin and eosin (H&E) staining to evaluate specific toxicity.
2.5 In vitro fluorescence kinetics of D-luc in MCF-7/DOXFluc cells analysis
To investigate the effect of ELE on the functionality of ABC transporters in vitro, the efflux kinetic of D-luc from MCF-7/DOXFluc cells was noninvasively monitored, and the change of luminescent intensity was quantitatively measured by BLI in real time. MCF-7/DOXFluc cells were seeded in 96-well solid black plate at the density of 7 × 103 cells per well. After cells attached for 24 h, Ko143 (inhibitors of ABCG2, 5 μmol L−1) and verapamil (inhibitors of ABCB1, 10 μmol L−1) alone were used as control or treated with ELE at varying concentrations (5 μg mL−1 and 20 μg mL−1), and incubated for different time (24 h, 48 h, and 72 h). Then D-luc was put into each well and immediately kinetically imaged using an in vivo imaging system (IVIS) (Xenogen, USA). The luminescent intensity values of intracellular photon signals were caught every 5 min for a total of 115 min to acquire the efflux kinetics of D-luc. Relative BLI (BLIrel) meant the photon signal intensity of each group normalized via total protein content to eliminate the confounding influence of increasing cell populations of photon signaling intensity. The data was calculated to obtain BLIrel versus time curves. Via the non-compartment model method, the dynamic parameters (AUC, MRT) of D-fluorescein potassium in cells were calculated.
2.6 In vivo fluorescence kinetics of D-luc in MCF-7/DOXFluc cells analysis
Different concentrations of D-luc directly affects the luminescent intensity. To detect optimal luminescent intensity, the appropriate concentration is crucial for the trials. The mice were intraperitoneally injected with different concentrations of D-luc (50 mg kg−1, 75 mg kg−1, and 100 mg kg−1). To obtain the efflux kinetics of D-luc in vivo, the BLI signals of each time point were quantitatively recorded within 180 min. The photon signaling intensity of each time point was normalized by the volume of tumors in each treatment group and considered as BLIrel. Subsequently, the acceptable concentration of D-luc could be applied in the following studies.
To acquire the efflux kinetics of D-luc under the intervention of ELE at different concentrations (10 mg kg−1, 25 mg kg−1), the BLI signals were taken at every time point for 0–115 min by using the IVIS kinetic imaging system after treating with ELE at a series of time points (7 d, 14 d, and 21 d). The BLI signal was also normalized as above. Via the non-compartment model method, the dynamic parameters-area under the curve (AUC) and mean residence time (MRT) of D-luc were calculated.
2.7 In vivo and in vitro correlation analysis
The correlation between the PK of D-luc and the PD of combined efficiency of DOX with ELE was explored to evaluate fluorescence efflux dynamics using D-luc, which served as a probe. The PK of D-luc used dynamic parameters (AUC, MRT) as the main indicators which respectively represented the intracellular accumulation and efflux rate of D-luc. The viability rate of MCF-7/DOXFluc cells and the tumor inhibition rate, respectively, were used as the main indexes for the PD of the combined ELE and DOX in vitro and in vivo. Three following relationships were analyzed using Pearson correlation analysis: (1) between the PK of D-luc in vitro and in vivo, (2) between the PK of D-luc in vitro and the PD of the combined ELE and DOX in vitro, and (3) between the PK of D-luc in vivo and the PD of the combined ELE and DOX in vivo.
2.8 Statistical analysis
Data was shown as mean ± SD (n > 3). Combining SPSS 20.0 software, the statistical analysis of data was determined using the Student t-test. The statistically significant difference was defined as *P < 0.05, **P < 0.01, and ***P < 0.001.
3. Results
3.1 ELE inhibited the viability of MCF-7/DOXFluc cells in vitro
The effects on the viability of MCF-7/DOXFluc cells after treating with ELE and DOX alone or in combination at different time points were evaluated (Fig. 1A–C). The results showed that the ELE, DOX, and ELE combined with DOX inhibited the viability of MCF-7/DOXFluc cells, and ELE was revealed to enhance the cytotoxicity of DOX on MCF-7/DOXFluc cells. More narrowly, the IC50 values of DOX at different time points (48 h, 72 h, and 96 h) were 57.55 μg mL−1, 21.02 μg mL−1, and 18.80 μg mL−1, respectively, demonstrating that the increased cytotoxicity of DOX on MCF-7/DOXFluc cells was time-dependent. The CIs of ELE and DOX were calculated by CompuSyn software, and the result indicated that ELE and DOX had a synergistical effect to reduce the cytotoxicity of DOX to MCF-7/DOXFluc cells (Fig. 1D).
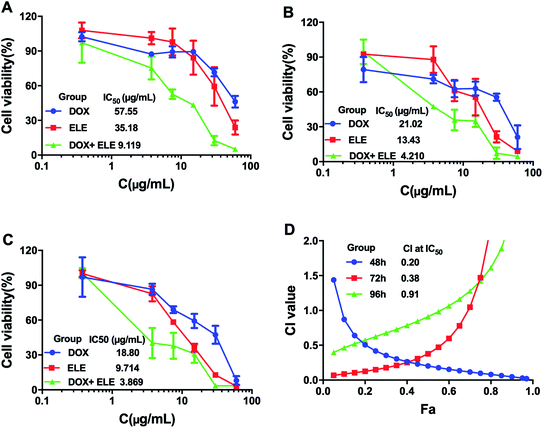 |
| Fig. 1 Cell viability of MCF-7/DOXFluc. MCF-7/DOXFluc cells were treated with ELE and DOX alone or in combination for (A) 48 h, (B) 72 h, and (C) 96 h. (D) Synergistic index curve of combination group treating on MCF-7/DOXFluc cells for 48 h, 72 h, and 96 h. Data are mean ± SD (n = 3). | |
3.2 ELE enhanced the inhibition of the MCF-7/DOXFluc breast cancer xenogeneic model in vivo
The tumor inhibition efficiency is shown in Fig. 2A–D. Comparing with the PBS group, the group treated with ELE (10 mg kg−1, i.p.) or DOX (5 mg kg−1, i.v.) alone exhibited mild reductions in the growth rate of tumors, while the combined DOX and ELE produced significant tumor growth inhibition effects (p < 0.05). The decrement of tumor weight (Fig. 2D) in mice treated by DOX combined with ELE was significantly lower than in the DOX group. Besides, H&E-stained tumor sections revealed that the internal tumor tissues were almost completely necrotic in the combined group (Fig. 2E). Additionally, the H&E staining showed a significant decrease in renal toxicities and slightly decreased cardiotoxicity in the combined group. This result demonstrated that ELE enhanced the anti-tumor efficacy and decrease the toxicity of DOX.
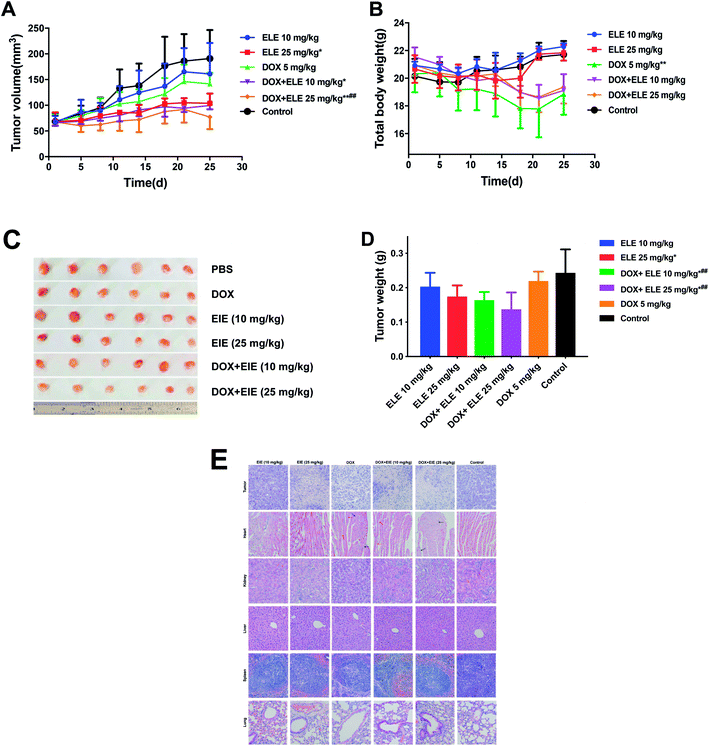 |
| Fig. 2 Tumor inhibition effects and systemic toxicity of DOX, ELE, and DOX in combination with ELE on MCF-7/DOXFluc tumor-bearing nude mice. (A) The nude mice were treated with DOX (5 mg mL−1, i.v.) and ELE (10 mg mL−1 and 25 mg mL−1, i.p.) or the combination of both drugs. The tumor volumes were measured twice a week. *P < 0.05; **P < 0.01 vs. control; #P < 0.05, ##P < 0.01 vs. DOX group. (B) Effect of different drugs on body weight of tumor-bearing nude mice. The body weights were measured twice a week. *P < 0.05; **P < 0.01 vs. control. (C) Visual observations of tumor size in per treatment group at the end time point. (D) Tumor mean (with SD) weights were measured 24 h after the last injection. *P < 0.05 vs. control group; ##P < 0.01 vs. the DOX group. (E) H&E staining of major organs and tumor tissues from tumor-bearing nude mice that received last treatment (20×). Data are mean ± SD (n = 6). | |
3.3 ELE inhibited the functionality of ABC transporters in MCF-7/DOXFluc cells in vitro
The efflux kinetic of D-luc in MCF-7/DOXFluc cells was noninvasively monitored. The luminescent intensity change was measured using IVIS after treating MCF-7/DOXFluc cells with the ABCG2/BCRP inhibitor Ko143 and the ABCB1 inhibitor verapamil (Fig. 3A), or with the ELE with varying concentrations for 24 h, 48 h, and 72 h (Fig. 3B–D). The result showed that ELE might weaken the elimination of intracellular D-luc, which caused the same trend as ABC transporter inhibitors Ko143 or verapamil. Compared with the control, the ABCB1 inhibitor verapamil (Table 1), and ELE at 20 μg mL−1 (Table 2) significantly increased the AUC, Ko143, verapamil and ELE suppressed MRT significantly (P < 0.05). These findings meant that Ko143 and verapamil could, to some extent, inhibit the functionality of ABC transporters, and verified that ELE could obviously increase the intracellular accumulation of D-luc to reduce its efflux by affecting the functionality of ABC transporters.
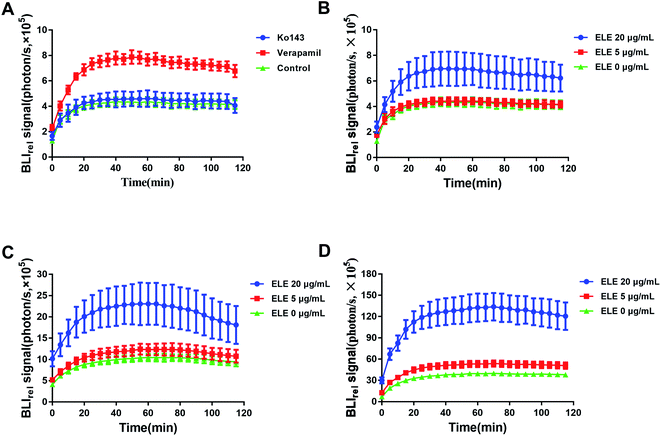 |
| Fig. 3 BLIrel signal photon–time curve of MCF-7/DOXFluc at different treatments in vitro. (A) Treated with verapamil and Ko143 for 24 h (x ± SD, n = 6). Treated with ELE at different concentrations for (B) 24 h, (C) 48 h, and (D) 72 h. Data are mean ± SD (n = 6). | |
Table 1 Pharmacokinetic parameters of BLIrel data (n = 6, mean ± SD)a
Parameters |
Verapamil (μg mL−1) |
Ko143 (μg mL−1) |
Control |
**P < 0.01, ***P < 0.001 vs. control. |
AUC (×107) |
6.7 ± 1.5** |
5.0 ± 0.6 |
4.5 ± 0.4 |
MRT |
625.3 ± 177.8*** |
359.4 ± 147.3*** |
1211.0 ± 270.6 |
Table 2 Pharmacokinetic parameters of BLIrel data in vitro (n = 6, mean ± SD)a
Concentration (μg mL−1) |
AUCluc (×107) |
MRTluc |
24 h |
48 h |
72 h |
24 h |
48 h |
72 h |
*P < 0.05, **P < 0.01, ***P < 0.001 vs. control. |
0 |
4.5 ± 0.3 |
10.5 ± 0.8 |
39.4 ± 1.9 |
1030.8 ± 466.2 |
305.8 ± 50.5 |
971.8 ± 163.9 |
5 |
4.6 ± 0.3 |
12.41 ± 1.2** |
53.4 ± 4.1** |
789.8 ± 93.6 |
263.3 ± 48.2 |
909.5 ± 108.3 |
20 |
7.1 ± 1.2*** |
23.0 ± 4.4*** |
131.8 ± 17.6*** |
469.4 ± 198.7* |
186.2 ± 46.3** |
554.9 ± 241.7* |
3.4 ELE inhibited the functionality of ABC transporters in MCF-7/DOXFluc cells in vivo
The luminescent intensity in tumor cells significantly enhanced with increasing concentration of D-luc (Fig. 5A). Since the concentration of 75 mg kg−1 D-luc was unsaturated while detected, it was chosen for the subsequent studies of BLI signal detection. BLI signals of the MCF-7/DOXFluc cells could be detected after i.p. injection of D-luc (Fig. 4). Tumor-bearing nude mice were i.p.-injected with 100 μL of D-luc after pretreatment of ELE (10 mg kg−1 and 25 mg kg−1, respectively) for different time points (7 d, 14 d, and 21 d) and then used for BLI. As shown in Fig. 5B–D, the BLIrel was gradually increased over time. Additionally, the parameters were calculated according to the non-compartmental model. The AUC of ELE-treated groups was higher than that of control group after 7 d, 14 d, and 21 d, whereas the MRT of ELE-treated groups was lower than for the control group (Table 3). This finding meant that, with the time-growth of ELE treatments, the intracellular efflux rate of D-luc in MCF/DOXFluc cells in vivo was reduced, while the intracellular accumulation was increased and concentration-dependent.
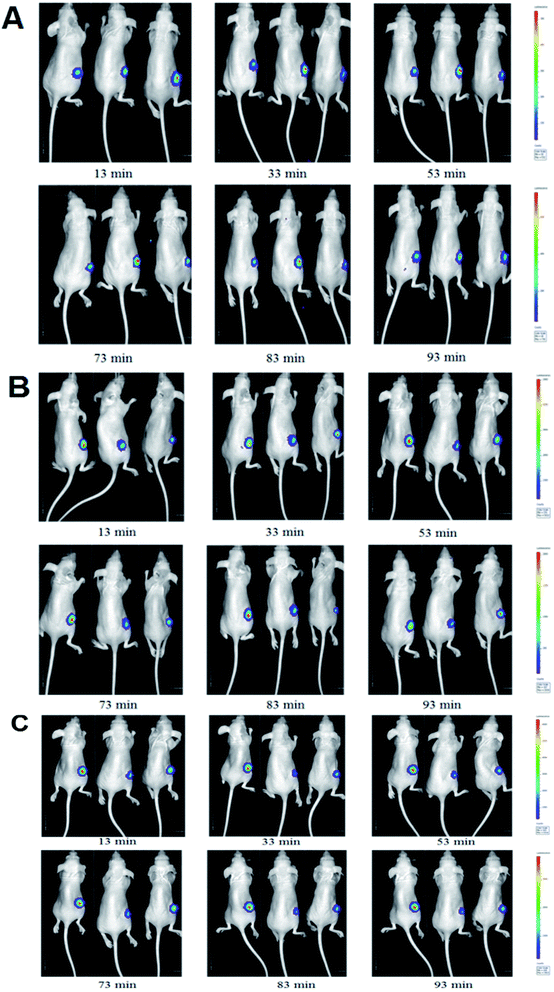 |
| Fig. 4 BLI of MCF-7/DOXFluc tumor-bearing nude mice at different time points within 115 min after intraperitoneal injected D-luc with a dose of 100 mg kg−1 (n = 3). (A) PBS group (i.p., QD). (B) The low ELE concentration group (ELE 10 mg kg−1, i.p., QD). (C) The high ELE concentration group (ELE 25 mg kg−1, i.p., QD). | |
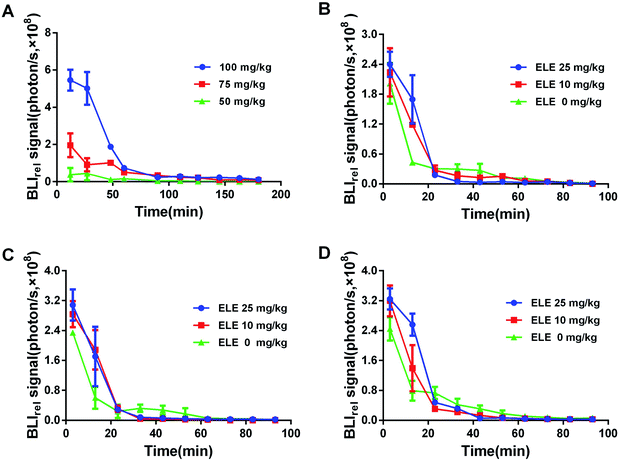 |
| Fig. 5 BLIrel signal photon–time curve of MCF-7/DOXFluc tumor-bearing nude mice at different treatments. (A) Bioluminescence response of D-luc at different concentrations. Treated with different ELE concentrations for (B) 7 d, (C) 14 d, and (D) 21 d. Data are mean ± SD (n = 3). The data are normalized to the 115 min time point. | |
Table 3 Pharmacokinetic parameters of BLIrel data in vivo (n = 6, mean ± SD)a
Concentration (mg kg−1) |
AUCluc (×109) |
MRTluc |
7 d |
14 d |
21 d |
7 d |
14 d |
21 d |
*P < 0.05, **P < 0.01 vs. control. |
0 |
3.1 ± 0.4 |
3.6 ± 0.1 |
4.5 ± 0.2 |
20.6 ± 0.7 |
17.9 ± 0.7 |
25.2 ± 5.1 |
10 |
3.6 ± 0.1 |
4.9 ± 0.6 |
5.4 ± 0.1 |
15.9 ± 1.6 |
14.5 ± 3.5 |
15.6 ± 0.3 |
25 |
3.7 ± 0.5 |
5.1 ± 0.8 |
5.7 ± 0.6 |
11.2 ± 0.7** |
13.3 ± 0.6* |
13.9 ± 0.2 |
3.5 A correlation existed between the PK of D-luc in MCF-7/DOXFluc cells in vitro and in vivo
After treating MCF-7/DOXFluc cells with ELE with varying concentrations (5 μg mL−1, and 20 μg mL−1) at different time points (24 h, 48 h, and 72 h), the AUC of D-luc in MCF-7/DOXFluc cells in vivo and in vitro was analyzed using Pearson correlation analysis. The results indicated that the AUC in vitro was positively correlated with the AUC in vivo in all ELE groups, and the Pearson correlation coefficient values were more than 0.7 (P < 0.05) (Table 4).
Table 4 Correlation of AUC (PK) in vitro and in vivo after ELE treatment
Group |
Pearson coefficient |
P |
ELE 5 mg kg−1 |
0.755 |
0.041 |
ELE 20 mg kg−1 |
0.881 |
0.02 |
3.6 A correlation existed between the PK of D-luc in MCF-7/DOXFluc cells and PD of the combined ELE and DOX in vitro
The viability of MCF-7/DOXFluc cells after being treated with ELE (5 μg mL−1, and 20 μg mL−1) and the AUC in MCF-7/DOXFluc cells at different time points (24 h, 48 h, and 72 h) were analyzed using Pearson correlation analysis. The AUC was negatively correlated with the cell viability in all ELE groups, which is shown in Table 5. The absolute Pearson correlation coefficient values for ELE groups (5 μg mL−1 and 20 μg mL−1) were 0.971 (P < 0.01) and 0.753 (P < 0.05), respectively.
Table 5 Correlation between cell viability (PD) and AUC (PK) in vitro after ELE treatment
Group |
Pearson coefficient |
P |
ELE 5 μg mL−1 |
−0.971 |
0.001 |
ELE 20 μg mL−1 |
−0.753 |
0.042 |
3.7 A correlation existed between the PK of D-luc in MCF-7/DOXFluc cells and PD of the combined ELE and DOX in vivo
The tumor inhibition on MCF/DOXFluc tumor-bearing nude mice was obtained by combined ELE (5 mg kg−1 and 10 mg kg−1) with DOX for 7 d, 14 d, and 21 d. The correlation between the AUC of D-luc in vivo and tumor inhibition was analyzed using Pearson correlation analysis. The results, listed in Table 6, showed a strong correlation between AUC and PD in vivo. The Pearson correlation coefficient values were 0.965 (P < 0.01) and 0.89 (P < 0.05), respectively.
Table 6 Correlation between tumor inhibition rate (PD) and AUC (PK) in vivo after ELE treatment
Group |
Pearson coefficient |
P |
ELE 5 mg kg−1 |
0.965 |
0.002 |
ELE 20 mg kg−1 |
0.890 |
0.017 |
4. Discussions
MDR reversing in cancer is a challenging task because it can be caused by many complex mechanisms, such as increased efflux of drugs, disordered cell apoptosis pathway, impaired DNA replication and repair capacity, altered drug target, and so on.27–32 Following a general number of studies, it has been widely recognized that the primary reason of MDR is the increased efflux of anti-tumor drugs via overexpression or abnormal functionality of membrane-embedded ABC transporters.33–35 Studies have shown that the drug efflux are not necessarily linked to the quantity while the functionality of ABC transporters. Now fluorescent dye rhodamine 123 (P-gp substrate) was already applied to determine the functional activity of ABC transporters in MDR cells quantitatively,35 but it failed to dynamically detect the luminescence pharmacokinetics in cell in real time, which must be subsequently analyzed by flow cytometry or high-performance liquid chromatography to determine the cellular content of rhodamine 123. Furthermore, it cannot evaluate the functionality of ABC transporters in live animals in vivo.
In our study, by combining firefly luciferase with BLI technology, the MDR reversing mechanisms of ELE were validated by directly exploring the functionality of ABC transporters through the real time altering of the cellular efflux of D-luc (substitute for chemotherapeutics) in vitro, especially in vivo. BLI detects the generated light by luciferase-catalyzed reactions of substrates which is especially suitable for observing biological processes, such as in vitro and in vivo when monitoring kinetic profiles of carriers or drugs.36 Several studies found a correlation between BLI dynamics and D-luc uptake and efflux mechanisms.37,38 In this paper, our findings revealed that the reversing MDR mechanism on human breast cancer of ELE was to inhibit the drug efflux by weakening the functionality of ABC transporters. Since D-luc efflux from the cell could be detected both in vitro and in vivo in real time, a good correlation between the efflux PK of D-luc in vitro and in vivo were also established. This finding offers a possible molecular mechanism to highlight the cellular uptake kinetics of D-luc so that it might serve as an effective probe for reflecting the drug efflux in tumor cells. It also suggests that firefly luciferase reporter assay system combined with BLI is useful for studying ABC transporters-mediated efflux by TCMs which are of higher efficacy and lower toxicity.
5. Conclusions
At present, many studies have found that the combination of traditional Chinese medicine and chemotherapeutic drugs showed a good reversal MDR effect. However, the reversal MDR mechanism is not very clear and it is difficult to establish an effective correlation between the reversal effect of TCM in vitro and in vivo. In this paper, we used BLI technology combined with luciferase reporter gene technology to achieve a non-injury, real-time, dynamic and quantitative characterization of ABC transporter substrate D-luc effluxing from live tumor cells in vitro and in vivo. This method could be used to evaluate the functionality of ABC transporters affected by MDR reversal agent and make it possible to establish a positive correlation between the reversal effect in vivo and in vitro.
Conflicts of interest
There are no conflicts to declare.
Acknowledgements
All work was financially supported by the National Natural Science Foundation of China (No. 81774011, No. 81473434, No. 81703713), by the Health Science and Technology Plan General (B) Project of Hangzhou, Zhejiang of China (No. OO20190902), by internal support from Zhejiang Chinese Medical University and by Opening Project of Zhejiang Provincial Preponderant and Characteristic Subject of Key University (Traditional Chinese Pharmacology), Zhejiang Chinese Medical University (No. ZYAOXZD2019003), and by Zhejiang Provincial Science Foundation of China (No. LY16H280007).
References
- R. L. Siegel, K. D. Miller and A. Jemal, Cancer statistics, 2018, Ca-Cancer J. Clin., 2018, 60(5), 7–30 CrossRef.
- W. Chen, R. Zheng, P. D. Baade, S. Zhang, H. Zeng and F. Bray, et al., Cancer statistics in China, 2015, Ca-Cancer J. Clin., 2016, 66(2), 115–132 CrossRef.
- W. Li, H. Zhang, Y. G. Assaraf, K. Zhao, X. Xu and J. Xie, et al., Overcoming ABC transporter-mediated multidrug resistance: molecular mechanisms and novel therapeutic drug strategies, Drug Resist. Updates, 2016, 27, 14–29 CrossRef.
- Y. Zhang, Z. Wu, H. Yu, H. Wang, G. Liu and S. Wang, et al., Chinese Herbal Medicine Wenxia Changfu Formula Reverses Cell Adhesion-Mediated Drug Resistance via the Integrin β1-PI3K-AKT Pathway in Lung Cancer, J. Cancer, 2019, 10(2), 293–304 CrossRef CAS.
- S. Ranjbar, R. Khonkarn, A. Moreno, H. Baubichon-Cortay, R. Miri and M. Khoshneviszadeh, et al., 5-Oxo-hexahydroquinoline derivatives as modulators of P-gp, MRP1 and BCRP transporters to overcome multidrug resistance in cancer cells, Toxicol. Appl. Pharmacol., 2019, 362, 136–149 CrossRef CAS.
- R. W. Robey, K. M. Pluchino, M. D. Hall, A. T. Fojo, S. E. Bates and M. M. Gottesman, Revisiting the role of ABC transporters in multidrug-resistant cancer, Nat. Rev. Cancer, 2018, 18(7), 452–464 CrossRef CAS.
- Y. F. Fan, W. Zhang, L. Zeng, Z. N. Lei, C. Y. Cai and P. Gupta, et al., Dacomitinib antagonizes multidrug resistance (MDR) in cancer cells by inhibiting the efflux activity of ABCB1 and ABCG2 transporters, Cancer Lett., 2018, 421, 186–198 CrossRef CAS.
- I. Genovese, A. Ilari, Y. G. Assaraf, F. Fazi and G. Colotti, Not only P-glycoprotein: amplification of the ABCB1-containing chromosome region 7q21 confers multidrug resistance upon cancer cells by coordinated overexpression of an assortment of resistance-related proteins, Drug Resist. Updates, 2017, 32, 23–46 CrossRef.
- W. K. Lee and F. Thevenod, Oncogenic PITX2 facilitates tumor cell drug resistance by inverse regulation of hOCT3/SLC22A3 and ABC drug transporters in colon and kidney cancers, Cancer Lett., 2019, 449, 237–251 CrossRef CAS.
- R. Hu, J. Gao, R. Rozimamat and H. A. Aisa, Jatrophane diterpenoids from Euphorbia sororia as potent modulators against P-glycoprotein-based multidrug resistance, Eur. J. Med. Chem., 2018, 146, 57–170 CrossRef.
- S. Ding, N. Patel and H. J. Zhang, Ciclosporin A as a Reversal Agent Against Concurrent Multidrug Resistance in Tumors with Nanobubbles, J. Biomed. Nanotechnol., 2018, 14(1), 190–197 CrossRef CAS.
- K. Jin, S. Park, W. W. Teo, P. Korangath, S. S. Cho and T. Yoshida, et al., HOXB7 Is an ERalpha Cofactor in the Activation of HER2 and Multiple ER Target Genes Leading to Endocrine Resistance, Cancer Discovery, 2015, 5(9), 944–959 CrossRef CAS.
- P. Caetano-Pinto, J. Jansen, Y. G. Assaraf and R. Masereeuw, The importance of breast cancer resistance protein to the kidneys excretory function and chemotherapeutic resistance, Drug Resist. Updates, 2017, 30, 15–27 CrossRef.
- X. Ma, M. Hu, H. Wang and J. Li, Discovery of traditional Chinese medicine monomers and their synthetic intermediates, analogs or derivatives for battling P-gp-mediated multi-drug resistance, Eur. J. Med. Chem., 2018, 159, 381–392 CrossRef CAS.
- J. Yang, B. Zhang, Z. Qin, S. Li, J. Xu and Z. Yao, et al., Efflux excretion of bisdemethoxycurcumin-O-glucuronide in UGT1A1-overexpressing HeLa cells: identification of breast cancer resistance protein (BCRP) and multidrug resistance-associated proteins 1 (MRP1) as the glucuronide transporters, Biofactors, 2018, 44(6), 558–569 CrossRef CAS.
- Y. Xiao, L. Xin, L. Li, G. Li, X. Shi and G. Ji, et al., Quercetin and kaempferol increase the intestinal absorption of isorhamnetin coexisting in Elaeagnus rhamnoides (L.) A. Nelson (Elaeagnaceae) extracts via regulating multidrug resistance-associated protein 2, Phytomedicine, 2019, 53, 154–162 CrossRef CAS.
- B. Zhai, Y. Zeng, Z. Zeng, N. Zhang, C. Li and Y. Zeng, et al., Drug delivery systems for elemene, its main active ingredient beta-elemene, and its derivatives in cancer therapy, Int. J. Nanomed., 2018, 13, 6279–6296 CrossRef CAS.
- Y. Zhang, J. P. Bressler, J. Neal, B. Lal, H. E. Bhang and J. Laterra, et al., ABCG2/BCRP expression modulates D-luciferin based bioluminescence imaging, Cancer Res., 2007, 67(19), 9389–9397 CrossRef CAS.
- S. Lwano, M. Sugiyama, H. Hama, A. Watakabe, N. Hasegawa and T. Kuchimaru, et al., Single-cell bioluminescence imaging of deep tissue in freely moving animals, Science, 2018, 359(6378), 935–939 CrossRef.
- J. Li, L. Chen, L. Du and M. Li, Cage the firefly luciferin!-a strategy for developing bioluminescent probes, Chem. Soc. Rev., 2013, 42(2), 662–676 RSC.
- R. Huang, J. Vider, I. Serganova and R. G. Blasberg, ATP-binding cassette transporters modulate both coelenterazine- and D-luciferin-based bioluminescence imaging, Mol. Imaging, 2011, 10(3), 215–226 CrossRef CAS.
- K. Sasaki, M. Tachikawa, Y. Uchida, S. Hirano, F. Kadowaki and M. Watanabe, et al., ATP-Binding Cassette Transporter A Subfamily 8 is a Sinusoidal Efflux Transporter for Cholesterol and Taurocholate in Mouse and Human Liver, Mol. Pharm., 2018, 15(2), 343–355 CrossRef CAS.
- Y. Zhang, M. Pullambhatla, J. Laterra and M. G. Pomper, Influence of Bioluminescence Imaging Dynamics by D-Luciferin Uptake and Efflux Mechanisms, Mol. Imaging, 2012, 11(6), 499–506 CrossRef CAS.
- Y. Zhang, Y. Byun, Y. R. Ren, J. O. Liu, J. Laterra and M. G. Pomper, Identification of inhibitors of ABCG2 by a bioluminescence imaging-based high-throughput assay, Cancer Res., 2009, 69(14), 5867–5875 CrossRef CAS.
- A. Pichler, J. L. Prior and D. Piwnica-Worms, Imaging reversal of multidrug resistance in living mice with bioluminescence: MDR1 P-glycoprotein transports coelenterazine, Proc. Natl. Acad. Sci. U. S. A., 2004, 101(6), 1702–1707 CrossRef CAS.
- C. Y. Tang, L. X. Zhu, J. D. Yu, Z. Chen, M. C. Gu and C. F. Mu, et al., Effect of beta-elemene on the kinetics of intracellular transport of D-luciferin potassium salt (ABC substrate) in doxorubicin-resistant breast cancer cells and the associated molecular mechanism, Eur. J. Pharm. Sci., 2018, 120, 20–29 CrossRef CAS.
- G. L. Beretta, G. Cassinelli, M. Pennati, V. Zuco and L. Gatti, Overcoming ABC transporter-mediated multidrug resistance: the dual role of tyrosine kinase inhibitors as multitargeting agents, Eur. J. Med. Chem., 2017, 142, 271–289 CrossRef CAS.
- V. GolerF-Baron and Y. G. Assaraf, Structure and function of ABCG2-rich extracellular vesicles mediating multidrug resistance, PLoS One, 2011, 6(1), e16007 CrossRef.
- Y. Miao, W. Zheng, N. Li, Z. Su, L. Zhao and H. Zhou, et al., MicroRNA-130b targets PTEN to mediate drug resistance and proliferation of breast cancer cells via the PI3K/Akt signaling pathway, Sci. Rep., 2017, 7, 41942 CrossRef CAS.
- S. Mallappa, P. K. Neeli, S. Karnewar and S. Kotamraju, Doxorubicin induces prostate cancer drug resistance by upregulation of ABCG4 through GSH depletion and CREB activation: relevance of statins in chemosensitization, Mol. Carcinog., 2019, e22996 Search PubMed.
- S. M. Stefan and M. Wiese, Small-molecule inhibitors of multidrug resistance-associated protein 1 and related processes: a historic approach and recent advances, Med. Res. Rev., 2019, 39(1), 176–264 CrossRef CAS.
- J. Zhang, Li Xiang and L. Huang, Non-viral nanocarriers for siRNA delivery in breast cancer, J. Controlled
Release, 2014, 190, 440–450 CrossRef CAS.
- Z. Chen, T. Shi, L. Zhang, P. Zhu, M. Deng and C. Huang, et al., Mammalian drug efflux transporters of the ATP binding cassette (ABC) family in multidrug resistance: a review of the past decade, Cancer Lett., 2016, 370(1), 153–164 CrossRef CAS.
- A. Dlugosz and A. Janecka, ABC Transporters in the Development of Multidrug Resistance in Cancer Therapy, Curr. Pharm. Des., 2016, 22(30), 4705–4716 CrossRef CAS.
- M. Z. El-Readi, S. Eid, A. A. Abdelghany, H. S. Al-Amoudi, T. Efferth and M. Wink, Resveratrol mediated cancer cell apoptosis, and modulation of multidrug resistance proteins and metabolic enzymes, Phytomedicine, 2019, 55, 269–281 CrossRef CAS.
- S. M. Thompson, M. R. Callstrom, B. E. Knudsen, J. L. Anderson, S. L. Sutor and K. A. Butters, et al., Molecular bioluminescence imaging as a noninvasive tool for monitoring tumor growth and therapeutic response to MRI-guided laser ablation in a rat model of hepatocellular carcinoma, Invest. Radiol., 2013, 48(6), 413–421 CrossRef CAS.
- B. D. Furmanski, S. Hu, K. I. Fujita, L. Li, A. A. Gibson and L. J. Janke, et al., Contribution of ABCC4-mediated gastric transport to the absorption and efficacy of dasatinib, Clin. Cancer Res., 2013, 19(16), 4359–4370 CrossRef CAS.
- J. Bakhsheshian, B. R. Wei, K. E. Chang, S. Shukla, S. V. Ambudkar and R. M. Simpson, et al., Bioluminescent imaging of drug efflux at the blood-brain barrier mediated by the transporter ABCG2, Proc. Natl. Acad. Sci. U. S. A., 2013, 110(51), 20801–20806 CrossRef CAS.
Footnote |
† These authors made equal contributions to this work. |
|
This journal is © The Royal Society of Chemistry 2020 |
Click here to see how this site uses Cookies. View our privacy policy here.