DOI:
10.1039/D0RA00100G
(Paper)
RSC Adv., 2020,
10, 7570-7584
Enhancement of ibuprofen solubility and skin permeation by conjugation with L-valine alkyl esters†
Received
4th January 2020
, Accepted 12th February 2020
First published on 21st February 2020
Abstract
New ibuprofen derivatives were made via conjugation with L-valine alkyl esters (ValOR), where R was changed from an ethyl to a hexyl group. The ionic structure was confirmed using NMR and FTIR. Specific rotation, solubility in commonly used solvents, thermal properties including phase transitions temperatures, and thermal stability were also determined. The ionic structure with a protonated amine group on an L-valine ester and melting points below 100 °C allowed inclusion of these ibuprofen derivatives into the pharmaceutically active protic ionic liquids. The ibuprofen salt solubility in deionised water and two buffer solutions at pH 5.4 and 7.4 were established and compared with the parent acid solubility. The octanol/water (buffer) partition coefficient, permeation through porcine skin, and accumulation in the skin were also measured. Ibuprofen pairing with L-valine alkyl esters [ValOR][IBU], caused higher solubility and a greater drug molecule absorption through biological membranes. log
P was lower for ibuprofen salts than for the acid and it increased with a longer L-valine ester cation alkyl chain. In vitro porcine skin tests showed that ibuprofen salts with a propyl or isopropyl ester in L-valine are particularly relevant for topical application. They provide transport for ibuprofen through the skin at much higher rate than the unmodified acid and a higher permeated ibuprofen concentration, which can improve efficacy. Thus, synthesised ibuprofen derivatives could be used as drug carriers in transdermal systems to provide better drug bioavailability, and they can be also be the source of exogenous L-valine.
Introduction
Ibuprofen (IBU) [2-(4-isobutylphenyl)-propionic acid] is a representative non-steroidal anti-inflammatory drug (NSAID), and it is commonly used to treat rheumatoid arthritis and osteoarthritis, pain, and fever. The oral delivery route has been the most widely used. To treat pain and fever, it is desirable to achieve quick relief, which is directly related to the appropriate concentration of ibuprofen in the blood.1
The absorption rate into the blood depends on the ibuprofen dissolution rate. However, ibuprofen, which is a carboxylic acid, has a relatively high lipophilicity and shows poor solubility in an aqueous media. Ibuprofen solubility depends on the pH, and it is especially low in the acidic environment of the stomach; changes are slight within the pH range of 1–4.2–4 An increase in ibuprofen solubility was recorded by various authors as the pKa (e.g. pKa = 4.44),4,5 was achieved and at a pH above the pKa, the solubility increases greatly, which results from ionisation. For example, Potthast et al. showed that solubility of ibuprofen at 37 °C was 0.038 mg cm−3 at pH 1 and it increased two-times (0.084 mg cm−3) and almost 20-times (0.685 mg cm−3) when the pH was changed to 4.5 and 5.5, respectively. However, the experimentally determined solubility at pH 6.8 was already 100-times higher (3.37 mg cm−3).3
The simplest modification of ibuprofen is the formation of its salts. By replacing the acid proton with the sodium cation, solubility in polar solvents, including water, was significantly increased compared to parent acid.6 It had a measurable effect on faster action and earlier pain relief.7–10 The ibuprofen anion can also be paired with organic cations, such as for the amino acids lysine, arginine and polylysine, in which the positive charge is located on the nitrogen atom of the amino group.11–14 Ibuprofen arginine or lysine salts, similar to its sodium salt, showed better solubility and a higher mean maximum plasma or serum ibuprofen concentration in a shorter time.15–18 Additional significant advantages of ibuprofen conjugates with amino acids including providing a source of amino acids, which are nutritional substances and possess marked activity. It was demonstrated that ibuprofen arginate retains key functional NSAID effects with similar or increased potency compared to sodium salt, and it can also decrease the risk of cardiovascular side effects.19,20
Formation of ibuprofen ion-pairs using organic bases was also shown to be an effective approach in topical ibuprofen administration. Dermal delivery overcomes several side effects of systemic use. It confines the therapeutic effects to the affected site and limits systematic absorption to the vascular system,21 thereby avoiding first-pass hepatic metabolism and ibuprofen gastrointestinal toxicity.22,23 However, the highly organised stratum corneum is a crucial barrier, which controls percutaneous absorption, and it thereby limits the achievement of effective therapeutic concentrations. Three penetration routes were considered including intercellular, intracellular, and follicular pathways, and lipid organisation in the intercellular space is important for skin barrier properties.24,25
Sarveiya et al.26 reported increased diffusion of ibuprofen alkylamine salts through the PDMS (polydimethylsiloxane) membrane compared to the sodium salt. Recently, various aromatic, tetra-alkylammonium and tetra-alkylphosphonium counterions were paired with the ibuprofen anion to form ionic liquids. Most of these salts have a markedly improved solubility in water compared to the parent acid. Two of these nine salts, i.e. tetrahexylammonium and didecyldimethylammonium, provided more and faster ibuprofen permeation. All the synthesised ibuprofen ionic liquids showed improved skin permeability compared to the conventional sodium salt.27 Furukawa et al.28 combined ibuprofen with proline ethyl ester as the base to form an ionic liquid, which showed significant enhancement in the cumulative amount of drug in the permeation experiments using pig skin compared to the acid form. Rogers et al.29 revealed that ibuprofen with lidocaine form liquid co-crystals in a deep eutectic form, and both components can be simultaneously transported through the model membrane at much higher rates than the corresponding commercially available crystalline salts, i.e. lidocaine chloride and ibuprofen sodium.
The aim of this study was to synthesise a series of ibuprofen derivatives as ion-pairs with a counterion based on L-valine. In addition to the expected effects for better solubility and permeation of ibuprofen, such derivatives can be the source of L-valine. Valine is an essential exogenous amino acid. It has an anti-catabolic effect, which means that it inhibits muscle-building protein degradation process. It also triggers gluconeogenesis, i.e. the transformation of non-sugar precursors into glucose. Valine is necessary for proper immune and nervous system functions, and it provides the body with energy, reduces fatigue, and has a positive effect on mood. Valine intensifies the secretion of anabolic hormones. It is necessary for the synthesis of pantothenic acid, i.e. vitamin B5.
We designed and characterised ibuprofen salts with L-valine alkyl esters, in which the alkyl group had different lengths from ethyl to hexyl. Physicochemical properties, i.e. thermal stability, specific rotation, solubility in organic solvents, and water, including buffer solutions, partition coefficient, and skin penetration were determined for salts and compared with those of the parent acid.
Experimental
Materials
All reagents were commercially available materials and were used without further purification. (R,S)-Ibuprofen (99%) was obtained from Acros Organics (Geel, Belgium). L-Valine (≥99%) was provided by Carl Roth (Karlsruhe, Germany). Trimethylsilyl chloride (≥99%) (TMSCl) was purchased from Sigma-Aldrich (Steinheim am Albuch, Germany). Methanol (MeOH), ethanol (EtOH), propan-2-ol (iPrOH), propan-1-ol (PrOH), butan-1-ol (BuOH), pentan-1-ol (AmOH), hexan-1-ol (HexOH), acetic acid, potassium chloride, sodium chloride, sodium sulfate anhydrous, orthophosphoric acid (98%), dimethyl sulfoxide, chloroform, ethyl acetate, diethyl ether, toluene and n-hexane were high purity provided by Chempur (Gliwice, Poland). Ammonium hydroxide solution 25% (NH3·H2O) was of analytical grade purchased from StanLab (Lublin, Poland). Acetonitrile (≥99.9%) for HPLC gradient grade and n-octanol (≥99%) were obtained from Sigma-Aldrich (Steinheim am Albuch, Germany). Disodium hydrogen phosphate dihydrate (≥99%) (Na2HPO4·2H2O) was obtained from Fisher Bioreagents (Pittsburgh, Pennsylvania, USA) and sodium dihydrogen phosphate anhydrous (98%) (NaH2PO4) was obtained from Acros Organics (Geel, Belgium). Deuterated chloroform (CDCl3) (99.8%) (+0.03% TMSCl) was obtained from Eurisotop (Cheshire, England). Potassium dihydrogen phosphate anhydrous (99%) was provided from Merck (Darmstadt, Germany).
Porcine skin was used in the permeation experiments due to its similar permeability to human skin.30 The fresh abdominal porcine skin was washed in PBS buffer pH 7.4 several times. After drying, dermatome section skin 0.5 mm thick was prepared (Humby Dermatome, Surtex Instruments, New Malden, England). Then samples of skin were stored frozen at −20 °C not longer than 3 months. This time was safe to keep the barrier properties of skin.31 Prior to the permeation experiments, the skin samples were thawed at room temperature for 30 min. Then the skin was allowed to hydration by phosphate buffer pH 7.4.32–34 Skin integrity was evaluated based on skin electrical impedance measurements (see below). Only undamaged skin pieces were used in the permeation experiments.
Synthesis of ibuprofen derivatives
The three-step synthesis of L-valine alkyl esters ibuprofenates was shown in the Scheme 1. General procedure for L-valine alkyl esters hydrochlorides (ValOR·HCl) synthesis, their neutralization to L-valine alkyl ester (ValOR) together with an identification and properties of these intermediates are available in (ESI, S4–S16† for ValOR·HCl; S17–S29 for ValOR).
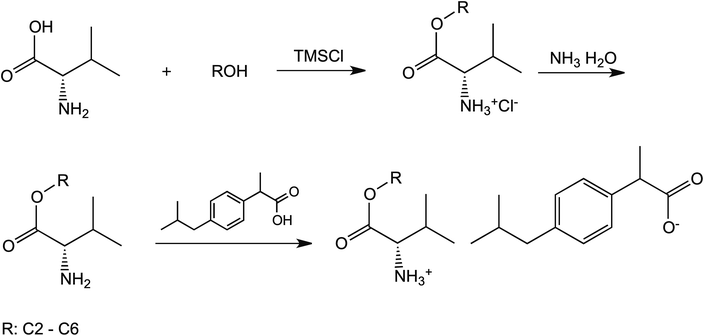 |
| Scheme 1 Synthesis path and structures of ibuprofen salts with L-valine alkyl esters. | |
General procedure for conjugation of ibuprofen with L-valine alkyl esters for preparation of [ValOR][IBU].
The L-valine alkyl ester (ValOR) was dissolved in diethyl ether or chloroform and added into equimolar amount of ibuprofen and stirred thoroughly at room temperature for 20 minutes. Then solvent was removed by evaporation under vacuum at 35 °C. The obtained ibuprofenate was dried in a vacuum oven at 60 °C for 24 h.
1H and 13C NMR spectra, FTIR spectra, TG and DSC curves for [ValOR][IBU] are available in ESI (S30–S47†).
[ValOEt][IBU] – L-valine ethyl ester ibuprofenate
Yield: 99%. 1H NMR (400 MHz, CDCl3) δ in ppm: 7.2 (d, 2H, J5,6 = 8.1 Hz, H5); 7.00 (d, 2H, J6,5 = 8.1 Hz, H6); 5.33 (s, 3H, H15); 4.06–4.13 (m, 2H, H17); 3.54–3.59 (q, 1H, H2); 3.31 (d, 1H, J14,13 = 4.6 Hz, H14); 2.36 (d, 2H, J8,9 = 7.1 Hz, H8); 1.96–2.01 (m, 1H, H13); 1.74–1.80 (m, 1H, H9); 1.38 (d, 3H, J3,2 = 7.1 Hz, H3); 1.18 (t, 3H, J18,17 = 7.1/7.4 Hz, H18); 0.86 (d, 3H, J12,13 = 6.8 Hz, H12); 0.82 (d, 6H, J10,9 = 6.6 Hz, H10); 0.81 (d, 3H, J11,13 = 6.9 Hz, H11); 13C NMR (100 MHz, CDCl3) δ in ppm: 179.25 (C1); 173.92 (C16); 140.25 (C7); 138.35 (C4); 129.21 (C5); 127.26 (C6); 61.05 (C17); 59.00 (C14); 45.62 (C8); 45.06 (C2); 31.51 (C13); 29.71 (C9); 22.41 (C10); 18.79 (C12); 18.50 (C3); 17.29 (C11); 14.23 (C18); FT-IR: ν(ATR): 2958; 2930; 2867; 1741; 1606; 1511; 1464; 1416; 1387; 1359; 1328; 1288; 1259; 1226; 1210; 1175; 1114; 1059; 983; 881; 692 cm−1; elemental analysis: calc. (%) for C20H33NO4 (351.485): C 68.34, H 9.46, N 3.99, O 18.21, found C 68.25, H 9.47, N 3.92, O 18.18.
[ValOPr][IBU] – L-valine propyl ester ibuprofenate
Yield: 95%. 1H NMR (400 MHz, CDCl3) δ in ppm: 7.21 (d, 2H, J5,6 = 8.1 Hz, H5); 7.08 (d, 2H, J6,5 = 8.4 Hz, H6); 5.46 (s, 3H, H15); 4.05–4.10 (m, 2H, H17); 3.63–3.67 (q, 1H, H2); 3.39 (d, 1H, J14,13 = 4.7 Hz, H14); 2.43 (d, 2H, J8,9 = 7.1 Hz, H8); 2.04–2.08 (m, 1H, H13); 1.81–1.87 (m, 1H, H9); 1.63–1.68 (m, 2H, H18); 1.46 (d, 3H, J3,2 = 7.3 Hz, H3); 0.88–0.96 (m, 15H, H10, H11, H12, H19); 13C NMR (100 MHz, CDCl3) δ in ppm: 179.19 (C1); 173.34 (C16); 140.18 (C7); 138.46 (C4); 129.18 (C5); 127.25 (C6); 68.71 (C17); 58.95 (C14); 45.44 (C8); 45.06 (C2); 30.19 (C13); 30.19 (C9); 22.40 (C10); 22.40 (C18); 18.73 (C12); 18.53 (C3); 17.23 (C11); 10.41 (C19); FT-IR: ν(ATR): 2967; 2933; 2868; 2847; 2690; 2363; 2343; 2193; 1744; 1680; 1609; 1548; 1513; 1467; 1414; 1390; 1360; 1329; 1321; 1308; 1290; 1260; 1221; 1176; 1153; 1116; 1061; 929; 883; 693 cm−1; elemental analysis: calc. (%) for C21H35NO4 (365.512): C 69.01, H 9.65, N 3.83, O 17.51, found: C 68.80, H 9.70, N 3.82, O 17.50.
[ValOiPr][IBU] – L-valine isopropyl ester ibuprofenate
Yield: 94%. 1H NMR (400 MHz, CDCl3) δ in ppm: 7.21 (d, 2H, J5,6 = 8.1 Hz, H5); 7.07 (d, 2H, J6,5 = 8.1 Hz, H6); 5.59 (s, 3H, H15); 5.01–5.07 (m, 1H, H17); 3.61–3.66 (q, 1H, H2); 3.36 (d, 1H, J14,13 = 3.9 Hz, H14); 2.43 (d, 2H, J8,9 = 7.3 Hz, H8); 2.04–2.08 (m, 1H, H13); 1.81–1.87 (m, 1H, H9); 1.45 (d, 3H, J3,2 = 7.3 Hz, H3); 1.24 (dd, 6H, J19,17 = 6.2 J18,17 = 6.2 Hz, H18, H19); 0.93 (d, 3H, J12,13 = 7.1 Hz, H12); 0.89 (d, 6H, J10,9 = 6.6 Hz, H10); 0.88 (d, 3H, J11,13 = 6.9 Hz, H11); 13C NMR (100 MHz, CDCl3) δ in ppm: 179.17 (C1); 173.34 (C6); 140.18 (C7); 138.46 (C4); 129.18 (C5); 127.25 (C6); 68.71 (C17); 58.95 (C14); 45.73 (C8); 45.06 (C2); 31.47 (C13); 30.19 (C9); 22.40 (C10); 21.78 (C18, C19); 18.73 (C12); 18.53 (C3); 17.23 (C11); FT-IR: ν(ATR): 2966; 2953; 2925; 2869; 2606; 1742; 1614; 1585; 1509; 1465; 1417; 1386; 1366; 1330; 1288; 1228; 1182; 1146; 1106; 1053; 917; 880; 825; 786; 677; 592 cm−1; elemental analysis: calc. (%) for C21H35NO4 (365.512): C 69.01, H 9.65, N 3.83, O 17.51, found: C 69.23, H 9.65, N 3.86, O 17.45.
[ValOBu][IBU] – L-valine butyl ester ibuprofenate
Yield: 94%. 1H NMR (400 MHz, CDCl3) δ in ppm: 7.22 (d, 2H, J5,6 = 8.1 Hz, H5); 7.08 (d, 2H, J6,5 = 8.0 Hz, H6); 5.09 (s, 3H, H15); 4.08–4.15 (m, 2H, H17); 3.63–3.68 (q, 1H, H2); 3.38 (d, 1H, J14,13 = 4.6 Hz, H14); 2.43 (d, 2H, J8,9 = 7.0 Hz, H8); 2.04–2.08 (m, 1H, H13); 1.80–1.87 (m, 1H, H9); 1.58–1.65 (m, 2H, H18); 1.46 (d, 3H, J3,2 = 7.1 Hz, H3); 1.33–1.42 (m, 2H, H19); 0.88–0.96 (m, 15H, H10, H11, H12, H20); 13C NMR (100 MHz, CDCl3) δ in ppm: 179.16 (C1); 174.44 (C6); 140.36 (C7); 138.21 (C4); 129.24 (C5); 127.25 (C6); 64.88 (C7); 59.20 (C14); 45.45 (C8); 45.07 (C2); 31.68 (C13); 30.63 (C18); 30.18 (C9); 22.41 (C10); 19.13 (C19); 18.91 (C12); 18.43 (C3); 17.24 (C11); 13.06 (C20); FT-IR: ν(ATR): 2962; 2933; 2870; 2848; 2691; 2604; 2349; 2313; 2184; 1743; 1606; 1545; 1511; 1466; 1391; 1361; 1320; 1301; 1288; 1260; 1245; 1215; 1176; 1117; 1062; 881; 727; 694 cm−1; elemental analysis: calc. (%) for C22H37NO4 (379.539): C 69.62, H 9.83, N 3.69, O 16.86, found: C 69.64, H 9.82, N 3.67, O 16.77.
[ValOAm][IBU] – L-valine pentyl ester ibuprofenate
Yield: 95%. 1H NMR (400 MHz, CDCl3) δ in ppm: 7.21 (d, 2H, J5,6 = 8.1 Hz, H5); 7.07 (d, 2H, J6,5 = 8.1 Hz, H6); 5.36 (s, 3H, H15); 4.04–4.14 (m, 2H, H17); 3.62–3.67 (q, 1H, H2); 3.39 (d, 1H, J14,13 = 4.6 Hz, H14); 2.43 (d, 2H, J8,9 = 7.0 Hz, H8); 2.03–2.07 (m, 1H, H13); 1.79–1.86 (m, 1H, H9); 1.61–1.65 (m, 2H, H18); 1.46 (d, 3H, J3,2 = 7.1 Hz, H3); 1.30–1.34 (m, 4H, H19, H20); 0.88–0.95 (m, 15H, H10, H11, H12, H21); 13C NMR (100 MHz, CDCl3) δ in ppm: 179.19 (C1); 174.33 (C16); 140.33 (C7); 138.21 (C4); 129.23 (C5); 127.25 (C6); 65.19 (C17); 59.12 (C14); 45.51 (C8); 45.07 (C2); 31.62 (C13); 30.20 (C9); 28.27 (C18); 28.03 (C19); 22.42 (C10); 22.27 (C20); 18.89 (C12); 18.46 (C3); 17.24 (C11); 13.96 (C21); FT-IR: ν(ATR): 2955; 2930; 2867; 2730; 2694; 2616; 2604; 1739; 1603; 1583; 1541; 1510; 1463; 1417; 1388; 1359; 1321; 1288; 1228; 1228; 1207; 1176; 1168; 1114; 1079; 1057; 971; 881; 692 cm−1; elemental analysis: calc. (%) for C23H39NO4 (393.565): C 70.08, H 9.99, N 3.56, O 16.26, found: C 70.08, H 9.99, N 3.74, O 16.25.
[ValOHex][IBU] – L-valine hexyl ester ibuprofenate
Yield: 96%. 1H NMR (400 MHz, CDCl3) δ in ppm: 7.21 (d, 2H, J5,6 = 7.9 Hz, H5); 7.06 (d, 2H, J6,5 = 8.1 Hz, H6); 6.13 (s, 3H, H15); 4.06–4.14 (m, 2H, H17); 3.60–3.64 (q, 1H, H2); 3.40 (d, 1H, J14,13 = 4.5 Hz, H14); 2.43 (d, 2H, J8,9 = 7.1 Hz, H8); 2.02–2.06 (m, 1H, H13); 1.80–1.87 (m, 1H, H9); 1.58–1.64 (m, 2H, H18); 1.44 (d, 3H, J3,2 = 7.1 Hz, H3); 1.26–1.36 (m, 6H, H19, H20, H21); 0.87–0.93 (m, 15H, H10, H11, H12, H22); 13C NMR (100 MHz, CDCl3) δ in ppm: 179.22 (C1); 173.89 (C16); 140.14 (C7); 138.54 (C4); 129.17 (C5); 127.26 (C6); 65.26 (C17); 58.97 (C14); 45.73 (C8); 45.07 (C2); 31.46 (C13); 31.37 (C18); 30.20 (C9); 28.53 (C19); 25.55 (C20); 22.52 (C21); 22.42 (C10); 18.75 (C12); 18.55 (C3); 17.31 (C11); 14.00 (C22); FT-IR: ν(ATR): 2958; 2930; 2867; 2731; 2165; 1741; 1606; 1511; 1464; 1416; 1387; 1359; 1328; 1288; 1259; 1226; 1210; 1175; 1114; 1059; 983; 881; 692 cm−1; elemental analysis: calc. (%) for C24H41NO4 (405.576): C 70.72, H 10.14, N 3.44, O 15.70, found: C 70.39, H 10.10, N 3.44, O 15.64.
Methods
General analytical methods – NMR, FTIR, UV-VIS, Elemental analysis, Thermogravimetric (TG) analysis, Differential Scanning Calorimetry (DSC), specific rotation, were presented in ESI (S2 and S3†).
A Shimadzu, model Nexera-i, LC-2040C 3D PLUS HPLC system (Kyoto, Japan), equipped with a UV-VIS/DAD detector and Kinetex® F5 column (2.6 μm; 150 × 4.6 mm; Phenomenex, Torrance, CA, USA) was used in solubility and partition coefficient experiments. Analyses were performed at 30 °C under isocratic conditions, with mobile phase consisted of water–acetonitrile mixture (50/50, v/v) and a flow rate of 1 cm3 min−1. Detection wavelength was 210 nm. Data acquisition and processing were performed using a LabSolutions/LC Solution System (software). Injections were repeated at least three times for each sample and the results were averaged. Concentration of ibuprofen and its salts were calculated on peak area measurements using a calibration curve method.
A Knauer (Berlin, German) liquid chromatograph consisting of a WellChrom model K1001 pump, model K2600 UV detector, model EuroChrom 2000 integrator, a Rheodyne model 7125 injector, equipped with UV-VIS detector and Hypersil ODS (C18) column (5 μm; 125 × 4.0 mm I.D.; Thermo Scientific™ Waltham, MA, USA) was used in skin permeation experiments for determination of compounds concentration in acceptor fluid and for measurements of accumulation in the skin. Isocratic elution with mobile phase consisted of acetonitrile – 1% (w/w) aqueous solution of acetic acid – methanol (45/45/10, v/v/v) at 1 cm3 min−1 flow rate was carried out. The signals were monitored at 264 nm and the temperature was 25 °C. Duplicate injections (20 μL) were carried out.
Solubility experiments
Solubility of ibuprofen and salts of ibuprofen with L-valine esters was evaluated in polar and nonpolar solvents by modified Vogel's method35 at the temperature of 25 °C. By this method the compound was classified as soluble, partly soluble and practically insoluble. Dimethyl sulfoxide, ethanol, chloroform, ethyl acetate, diethyl ether, toluene and n-hexane were used as solvents.
Solubility (saturation concentration) defined as a maximum quantity of a substance that may be dissolved at given temperature in unit of solvent volume was determined for ibuprofen and salts of ibuprofen with L-valine alkyl esters in deionised water and phosphate buffers (pH 5.4 and 7.4). An excess of substance was added to 2 cm3 of water or buffer in screwed vial and was stirred vigorously at 25.00 ± 0.05 °C or at 32.00 ± 0.05 °C, for 24 hours. Then mixture was centrifuged at respective temperature, and liquid above the solid was taken, diluted and analysed by the HPLC method to determine the concentration of the substance.
Determination of partition coefficient
The n-octanol/water and n-octanol/phosphate buffer (pH 5.4 and 7.4) partition coefficient was determined for ibuprofen and salts of ibuprofen with L-valine alkyl esters. For this purpose, 10 mg of the substance, weighed with an accuracy of 0.01 mg, was added to 5 cm3 of water (or buffer) saturated with n-octanol and 5 cm3 of n-octanol saturated with water. Mixture was vigorously agitated at 25 °C for 3 hours followed by centrifugation at 7500 rpm, at 25 °C for 10 min for the better phase separation. After centrifugation aqueous layer was decanted and analysed by HPLC to determine the concentration of the compound. The partition coefficient log
Pow was calculated in accordance with the formula:
where cw and coct represents concentration [mg dm−3] of the substance dissolved in aqueous layer (water or phosphate buffer) and octanol respectively.
Concentration of the compound dissolved in octanol was calculated by the formula:
where
c0 is a total concentration [mg dm
−3], calculated based on the mass of compound used in the experiment.
Skin electrical impedance
Skin impedance was measured using an LCR meter 4080 (Voltcraft LCR 4080, Conrad Electronic, Hirschau, Germany), which was operated in parallel mode at an alternating frequency of 120 Hz (error at kΩ values <0.5%). Skin sample was placed in Franz diffusion cell. For the measurement, the tips of measuring probes were immersed in donor and in acceptor chamber, both filled with PBS buffer pH 7.4.36 Skin samples with determined impedance above 3 kΩ were used in the permeation experiments. These values were similar to the electrical resistance of human skin reported by others.37
Skin permeation studies
The permeation experiments were performed in the Franz diffusion cells (SES GmbH Analyse Systeme, Bechenheim, Germany) with a diffusion area of 1 cm2. The volume of the acceptor chamber was 8 cm3 and the volume of the donor chamber was approximately 2 cm3. The acceptor chamber was filled with phosphate buffer pH 7.4 (0.1 M). In each diffusion unit a constant temperature of 32.0 ± 0.5 °C was maintained via thermostat (VEB MLW Prüfgeräte-Werk type of 3280, Leipzig, Germany). The content of the acceptor chamber was stirred with a magnetic stirring bar at the same speed for all cells. The donor chamber was filled with the solution of a tested compound in ethanol 70% (v/v). Concentration of compound was 0.01 g cm−3.
The undamaged skin pieces, prepared as described in the Material section, were placed between donor and acceptor chamber of Franz diffusion cells and integrity has been checked. Following by a 500 μL of the solution of the tested compound was applied to each skin sample.
The experiment was carried for 24 hours. The acceptor fluid was sampled (0.3 cm3) after 0.5 h, 1 h, 2 h, 3 h, 4 h, 5 h, 8 h and 24 h and the acceptor chamber was refilled with the same volume of the fresh buffer. Experiments were carried out in triplicate for each compound. The drug concentration in the acceptor fluid was determined using HPLC method. The cumulative mass (μg cm−2) was calculated based on this concentration. The flux (in μg cm−2 h−1) of the active drug – ibuprofen and its derivatives through the skin into acceptor fluid was determined as the slope of the plot of cumulative mass in the acceptor fluid versus time.
Permeation of ibuprofen was also expressed as percentage of applied dose of ibuprofen in compound.
Accumulation in the skin
After 24 hours permeability tests, the donor chamber was disconnected from Franz diffusion cell and each skin sample was removed, carefully rinsed in PBS solution at 7.4 pH and dried at the room temperature. The skin sample was weighed, diffusion area cut out and minced using scissors. Next skin sample was placed in 2 cm3 methanol and was incubated for 24 h at the temperature of 4 °C. After this time skin sample was homogenised for 3 minutes using a homogenizer (IKA®T18 digital ULTRA TURRAX, Staufen im Breisgau, Germany). The homogenate was centrifuged at 3500 rpm for 5 min. The supernatant was collected and analysed by HPLC.
Accumulation of the ibuprofen in the skin was calculated by dividing the amount of the drug remaining in the skin by a mass of skin sample and was expressed in mass of ibuprofen per mass of the skin (μg g−1). The average and standard deviation of these parameters were calculated from three replicates. Moreover, accumulation of ibuprofen in the skin was expressed as percentage of applied dose of ibuprofen in the compound in the donor chamber.
Statistical analysis
Statistical calculations were done using Statistica 12 PL software (StatSoft, Polska). Results were presented as an arithmetical mean ± standard deviation (SD). The results were evaluated using one-way analysis of variance (ANOVA). All determinations were performed in triplicate (n = 3).
Results and discussion
Synthesis, identification and characterisation of the [ValOR][IBU]
Salts of ibuprofen with L-valine alkyl esters were synthesised using the three-step method (Scheme 1). In the first step, the hydrochlorides of L-valine alkyl esters (L-ValOR·HCl) were prepared from L-valine and the respective alcohol as starting materials in the presence of chlorotrimethylsilane, with a similar method as that used previously for hydrochlorides of amino acid methyl esters.38 The length of the alkyl chain (R) ester group was from C2 to C6, and the C3 group was used as a straight-chain n-propyl and branched-chain isopropyl group. Hydrochlorides were purified from by-products, such as hexamethyldisilane, trimethylsilyl hydroxide, alkyltrimethylsilyl ether and obtained with high yields of 89–95%. Then hydrochlorides were neutralised with excess aqueous ammonia to the respective amino acid esters (ValOR) with yields of 76–84%, and this was followed by pairing the ester with ibuprofen to form [ValOR][IBU]. No by-products were formed in the last synthesis stage and the obtained ibuprofenates were characterized by very high purity. The structure and purity of the products at each stage was checked and confirmed using 1H and 13C-NMR spectroscopy (attached in ESI†). For example, comparison of the three 1H NMR spectra for hydrochloride L-valine ethyl ester and L-valine ethyl ester and its ionic pair with ibuprofen is shown in Fig. 1. The NH3 protons in hydrochloride L-valine alkyl ester appear as a somewhat broadened signal at δ 8.7–8.9 ppm in CDCl3. The broadening is partially caused by a rapid intermolecular exchange. After neutralisation of hydrochloride, the NH2 protons in L-valine alkyl ester appear as a signal at δ 1–2 ppm in CDCl3, which is typical for the primary amine group. The presence of the protonated amine group in ibuprofen salts with L-valine esters was confirmed by strongly downfield-shifted N–H signals, which appeared in CDCl3 at δ 5–6 ppm. Integration of these signals corresponds to three protons. The results of NMR analysis confirm the ionic structure of the synthesised compounds [ValOR][IBU] and the equimolar ratio between both ionic components (ibuprofen anion and L-valine alkyl ester cation). Additionally, evidence for the ionic structure is the carbonyl carbon signal, which is shifted about 2 ppm upfield (to about 179 ppm) in ibuprofen derivatives compared to the chemical shift value for this carbon in the parent ibuprofen (181.16 ppm).39–41
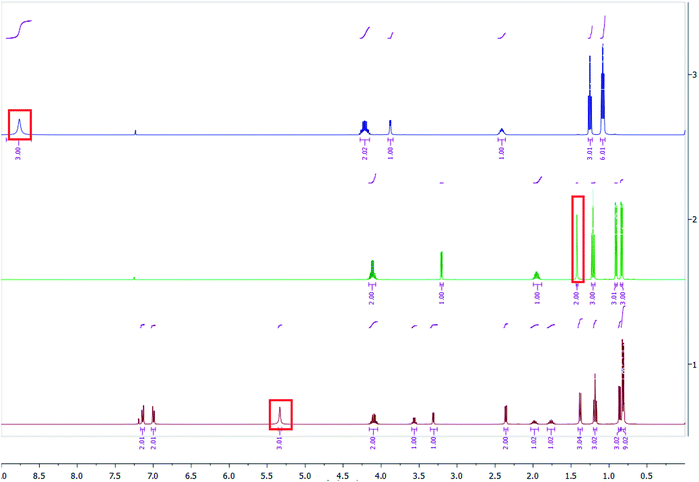 |
| Fig. 1 Comparison of 1H NMR spectra for hydrochloride L-valine ethyl ester [ValOEt][HCl] (dark blue), ethyl ester of L-valine [ValOEt] (green), and its ibuprofen salt [ValOEt][IBU] (red) (the amino and protonated amino group are marked in the red square). | |
The characteristic IR absorption bands for [ValOR][IBU] also confirmed the ionic structure. The FTIR spectra for ibuprofen, L-valine ester, L-valine ester hydrochloride, and ester ibuprofenate are presented for comparison in Fig. 2. In FTIR spectra of all ibuprofen derivatives, the characteristic sharp absorption band was observed in the range of 1739–1744 cm−1, which is attributed to C
O stretching vibrations of the ester carbonyl group in the L-valine ester part. FTIR spectra of L-valine ester and L-valine ester hydrochloride also showed this band and confirmed this finding. For ibuprofen, there was a broad band at 1709 cm−1, which is characteristic of the C
O stretching vibrations of the carboxylic acid group. In ibuprofen derivatives, FTIR spectra of this band were shifted and two bands were observed at approximately 1610 and 1390 cm−1, which are assigned to the asymmetric v(COO−)as and symmetric stretching vibrations v(COO−)sym, respectively. This shift and a difference above 200 cm−1 between the frequency values of these two bands confirmed the presence of the carboxylate anion COO− and the ionic structure of ibuprofen derivatives.42 The differences in the IR bands for the salts of ibuprofen with various L-valine alkyl esters were negligible.
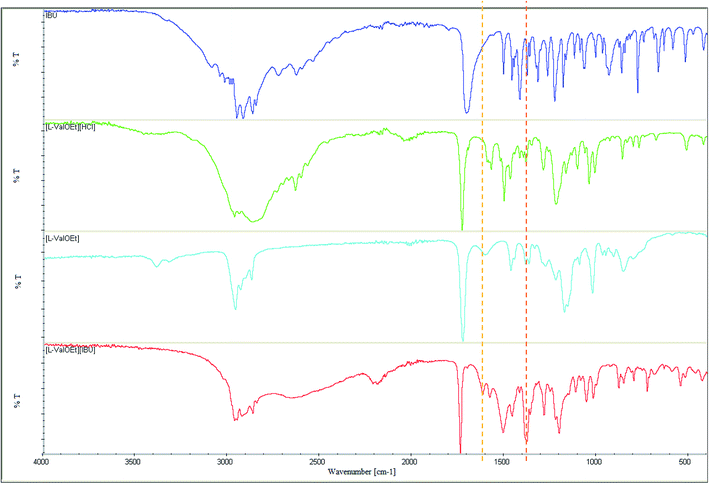 |
| Fig. 2 Comparison of FTIR spectra ibuprofen (dark blue), L-valine ethyl ester hydrochloride (green), L-valine ethyl ester [ValOEt] (light blue), and L-valine ethyl ester ibuprofenate (red) (v(COO−)as and v(COO−)sym are marked with a yellow and orange line, respectively. | |
Elemental analysis confirmed the content of individual elements (C, H, N, O) in synthesised ibuprofen derivatives.
Salts of ibuprofen with L-valine alkyl esters [ValOR][IBU] were white solids at room temperature, which was opposite of the series of ibuprofen salts with counterions, such as phosphonium, imidazolium, ammonium, procaine, lidocaine, or ranitidine that were recently reported by Wu et al.27 which were liquids at ambient temperature. As shown in Table 1, the melting point of [ValOR][IBU], which was determined using the DSC method as a peak temperature, depended on the alkyl chain R in the ester group and it was between 67.4 and 79.8 °C. The lowest melting point was for ibuprofen paired with L-valine hexyl ester and the highest melting point was for L-valine propyl ester. Salts containing butyl and longer chains in the ester group of L-valine showed lower melting points than the parent acid (77.5 °C). Ibuprofen salts give very broad melting peaks compared to the sharp melting peak for ibuprofen, which can be caused by the size distribution of the crystallites. All synthesised compounds resulting from a melting point below 100 °C and the ionic structure can be classified as ionic liquids.43 DSC curves for ibuprofen salts were recorded in a heating–cooling–heating sequence of the sample and are available in ESI.† In DSC analysis, the exothermic peak of crystallisation for each ibuprofen salt, [ValOR][IBU], was observed, while the crystallisation temperature was not determined for the parent ibuprofen (ESI, S50†). Crystallisation peak temperatures were about 30 °C lower than the melting peak temperatures for the respective ibuprofen salt, but the onset of melting and crystallisation was observed at a similar temperature. The evidence that, upon cooling, the sample returns to the same state as before the melting transition, is shown by the melting point temperature that was determined in a second heating mode, which is almost identical to the temperature that was observed at the first heating.
Table 1 The properties of ibuprofen and its L-valine ester derivativesa
Compound |
Colour |
Tm/°C |
Tc/°C |
Tonset/°C |
[α]λT |
Tc, crystallisation peak temperature; Tm, melting point; Tonset, the onset temperature of the thermal degradation. |
rac-IBU |
White |
77.51 |
— |
189.8 |
— |
[ValOEt][IBU] |
White |
77.99 |
55.20 |
89.5 |
+8.867 |
[ValOPr][IBU] |
White |
79.81 |
48.67 |
109.5 |
+9.760 |
[ValOiPr][IBU] |
White |
78.01 |
64.02 |
90.2 |
+11.852 |
[ValOBu][IBU] |
White |
76.80 |
46.22 |
119.9 |
+11.094 |
[ValOAm][IBU] |
White |
73.81 |
41.99 |
129.8 |
+10.076 |
[ValOHex][IBU] |
White |
67.35 |
36.51 |
128.9 |
+8.987 |
Thermal stability of pharmaceutics is a very important useful property that is necessary to control the formulation method of the finished drug. The thermal stability of [ValOR][IBU] was investigated using the TG analysis (Fig. 3). The degradation onset temperature for [ValOR][IBU] was in the range of 89.5–128.9 °C, depending on the alkyl chain in the ester group of L-valine, whereas the value for ibuprofen was 189.8 °C (Table 1). Thus, the synthesised ibuprofen derivatives showed lower thermal stability compared to the acid form of ibuprofen. The thermal stability increased with the length of the alkyl chain. The ionic pair of ibuprofen and L-valine hexyl ester [ValOHex][IBU] had the highest thermal stability, while [ValOEt][IBU] was characterised by the lowest thermal stability (Fig. 3).
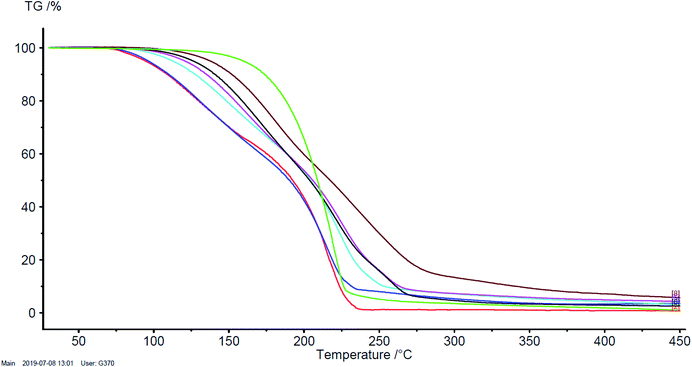 |
| Fig. 3 The TG curves of ibuprofen and its L-valine ester derivatives green, IBU; red [ValOEt][IBU]; dark blue [ValOiPr][IBU]; light blue [ValOPr][IBU]; purple/rose [ValOBu][IBU]; black [ValOAm][IBU]; and brown [ValOHex][IBU]. | |
All obtained ibuprofen derivatives were optical active compounds, which resulted from using optically pure L-valine to synthesise ibuprofen derivatives. Ibuprofen also has the chiral centre, but we started from the ibuprofen racemate. Specific rotation was similar for all ibuprofen salts (Table 1) and it was found to be between +8.867 for [ValOEt][IBU] and +11.852 for [ValOiPr][IBU].
Solubility
Solubility is a very important property in drug discovery and development, and it may be expressed in several ways. We applied Vogel's methodology to determine the solubility of [ValOR][IBU] in solvents with different polarities. Three categories of solubility were created based on the amount of compound which dissolved in 1 cm3 of solvent. The compound was recognised as soluble when more than 100 mg of compound was soluble in 1 cm3, as partly soluble when 33–100 mg of compound dissolved in 1 cm3; as practically insoluble when less than 33 mg of compound was soluble in 1 cm3. The solubility was established and summarised in Table 2. Ibuprofen salts [ValOR][IBU] were soluble or partly soluble in toluene, chloroform, ethanol, dimethyl sulfoxide, diethyl ether, and ethyl acetate. Moreover, the ability of [ValOR][IBU] to dissolve in nonpolar hexane increased with elongation of the alkyl chain R in the L-valine ester group. Ibuprofen and its salts with ethyl to butyl ester of L-valine were practically insoluble in hexane. However, salt with an amyl ester of L-valine [ValOAm][IBU] and with hexyl ester [ValOHex][IBU] were, respectively, partly soluble and soluble in hexane.
Table 2 Solubility of ibuprofen and its L-valine ester derivatives in organic solvents at 25 °Ca
Compound |
Ethanol |
DMSO |
Chloroform |
Ethyl acetate |
Diethyl ether |
Toluene |
n-Hexane |
“+”, soluble >100 mg cm−3; “+/−”, partly soluble 33–100 mg cm−3; “—”, practically insoluble <33 mg cm−3 at 25 °C. |
rac-IBU |
+ |
+ |
+ |
+ |
+ |
+ |
— |
[ValOEt][IBU] |
+ |
+ |
+ |
+ |
+ |
+ |
— |
[ValOPr][IBU] |
+ |
+ |
+ |
+/− |
+/− |
+ |
— |
[ValOiPr][IBU] |
+ |
+ |
+ |
+/− |
+/− |
+ |
— |
[ValOBu][IBU] |
+ |
+ |
+ |
+/− |
+/− |
+ |
— |
[ValOAm][IBU] |
+ |
+ |
+ |
+ |
+ |
+ |
+/− |
[ValOHex][IBU] |
+ |
+ |
+ |
+ |
+ |
+ |
+ |
Considering that the different pharmacological solutions were aqueous solutions, we determined the solubility of the ibuprofen salts in deionised water and two buffer solutions at pH 5.4 and 7.4 and compared with solubility of the parent acid (Table 3). The saturation concentration of the salts in the water and buffers was also expressed as the concentration of ibuprofen and they are presented in Table 3. As was shown, the pairing of ibuprofen with L-valine alkyl esters markedly enhanced ibuprofen's solubility in water and buffer solutions. However, better solubility was found for conjugates that had shorter alkyl chains in the L-valine ester moiety. Lower solubility for longer hydrocarbon chains is typical and caused by their weaker solvation, and more energy is necessary to overcome the strength of intermolecular interaction between more tightly packed molecules.44
Table 3 Solubility of ibuprofen and its L-valine derivatives in water and phosphate buffers (pH 5.4 and 7.4) at 25 °C
Compound |
Solubility in water |
Solubility in phosphate buffer |
pH = 5.4 |
pH = 7.4 |
g dm−3 |
g IBU dm−3 |
g dm−3 |
g IBU dm−3 |
g dm−3 |
g IBU dm−3 |
rac-IBU |
0.076 ± 0.001 |
0.076 ± 0.001 |
0.082 ± 0.001 |
0.082 ± 0.001 |
0.432 ± 0.001 |
0.432 ± 0.001 |
[ValOEt][IBU] |
5.542 ± 0.007 |
3.252 ± 0.007 |
3.969 ± 0.056 |
2.329 ± 0.056 |
5.206 ± 0.044 |
3.055 ± 0.044 |
[ValOPr][IBU] |
4.221 ± 0.033 |
2.382 ± 0.033 |
3.057 ± 0.045 |
1.725 ± 0.045 |
5.367 ± 0.019 |
3.029 ± 0.019 |
[ValOiPr][IBU] |
3.468 ± 0.007 |
1.957 ± 0.007 |
2.350 ± 0.027 |
1.326 ± 0.027 |
4.998 ± 0.018 |
2.821 ± 0.018 |
[ValOBu][IBU] |
3.125 ± 0.007 |
1.699 ± 0.007 |
2.371 ± 0.016 |
1.289 ± 0.016 |
3.089 ± 0.015 |
1.679 ± 0.015 |
[ValOAm][IBU] |
1.798 ± 0.019 |
0.942 ± 0.019 |
1.380 ± 0.007 |
0.723 ± 0.007 |
1.931 ± 0.008 |
1.012 ± 0.008 |
[ValOHex][IBU] |
1.058 ± 0.002 |
0.538 ± 0.002 |
0.759 ± 0.006 |
0.386 ± 0.006 |
1.233 ± 0.001 |
0.627 ± 0.001 |
The obtained salts were slightly less soluble in pH 5.4 buffer than in pH 7.4 buffer. In pH 5.4 buffer, the saturation concentration was 2.329 g IBU dm−3 for [ValOEt][IBU] to 0.386 g IBU dm−3 for [ValOHex][IBU], which was almost up 30-times higher than for the unmodified acidic form (0.082 g dm−3). Solubility in pH 7.4 buffer was found to be between 3.055 g IBU dm−3 for [ValOEt][IBU] and 0.627 g IBU dm−3 for [ValOHex][IBU], and it was up to seven-times higher than that of the parent acid (0.432 g dm−3).
An increase in solubility of salts with increasing pH is in accordance with what is known about sodium ibuprofenate.26
In deionised water, the solubility of salts was similar to the results that were obtained in pH 7.4. It was in the range of 3.252–0.538 g IBU dm−3, and up to 40-times higher than for unmodified ibuprofen (0.076 g dm−3). Moreover, a straight-chain n-propyl in the L-valine ester group resulted in better solubility than a branched-chain isopropyl (Table 3). The solubility in deionised water was 2.382 g IBU dm−3 for [ValOPr][IBU] and 1.957 g IBU dm−3 for [ValOiPr][IBU].
Compared to the water solubility of the known ibuprofen organic salts, the solubility of L-valine ester ibuprofenates was higher than for tetrahexylammonium, trihexyltetradecylphosphonium, and didecyldimetylammonium ibuprofenates, while was close to lidocaine or procaine ibuprofenates but lower than for butylmetylimidazolium or tetrabutylphosphonium salts.27
Partition coefficient
The partition coefficient between n-octanol and water, which is commonly reported as log
Po/w, is a key parameter that is used to predict drug hydrophobicity and partitioning in biological systems. The octanol–water (or buffer) partition coefficients for the acidic form of ibuprofen and its salts with L-valine esters are presented in Table 4. All salts showed a positive log
P, but they were lower compared with that of ibuprofen in the respective system, which indicated that formation of salts reduced hydrophobicity. Reduction of the hydrophobic nature was more significant for the octanol/water and octanol/buffer systems at pH 5.4. For example, log
P octanol/water and octanol/buffer at pH 5.4 for [ValOEt][IBU] was, respectively, 2.5-times and 1.5-times lower than for ibuprofen, for which log
P in two systems were 2.415 and 3.192 respectively. Log
P values for ibuprofen salts in the system octanol/buffer at pH 7.4 were very close to log
P of acid form of ibuprofen (1.284), which can indicate similar ionisation of the acid and salts at a higher pH.
Table 4 The n-octanol/(water or phosphate buffer (pH 5.4 and 7.4) partition coefficient (log
P) of ibuprofen and its L-valine esters derivatives
Compound |
log Pwater |
log PpH=5.4 |
log PpH=7.4 |
rac-IBU (rac) |
2.415 ± 0.001 |
3.192 ± 0.005 |
1.284 ± 0.006 |
[ValOEt][IBU] |
0.992 ± 0.008 |
2.023 ± 0.001 |
1.026 ± 0.001 |
[ValOPr][IBU] |
1.249 ± 0.005 |
2.061 ± 0.001 |
1.197 ± 0.003 |
[ValOiPr][IBU] |
1.154 ± 0.004 |
2.186 ± 0.001 |
1.172 ± 0.001 |
[ValOBu][IBU] |
1.520 ± 0.004 |
2.337 ± 0.001 |
1.237 ± 0.012 |
[ValOAm][IBU] |
1.750 ± 0.003 |
2.421 ± 0.002 |
1.259 ± 0.001 |
[ValOHex][IBU] |
1.833 ± 0.002 |
2.880 ± 0.011 |
1.279 ± 0.001 |
Shorter alkyl chains in the ester group of L-valine provided a decrease in hydrophobicity. The log
P in the octanol/water system for ibuprofen salts decreased from 1.883 to 0.992 when the alkyl chain was changed from hexyl to ethyl. In the octanol/pH 5.4 buffer system, the change in log
P was from 2.880 to 2.023, and in the octanol/pH 7.4 buffer system, log
P changed slightly, from 1.279 to 1.026, with decreasing alkyl chain length in valine ester moiety. The L-valine esters ibuprofenate showed comparable log
P values with known ammonium salts, as follows: [N1,1,10,10][IBU], 1.87; [N6,6,6,6][IBU], 2.47; phosphonium [P4,4,4,4][IBU], 1.54; lidocaine salt, 1.49; and imidazolium [BMIm][IBU], 1.15.27
Skin penetration
Skin penetration of topically applied active compounds is restricted by the stratum corneum, which is a lipophilic skin barrier for exogenous compounds. To determine the possibility of using ibuprofen derivatives in topical and transdermal drug delivery, in vitro skin penetration studies were conducted with using an abdomen porcine skin. Because of difficulties in sourcing human skin as well as ethical issues, the porcine skin is frequently used for preliminary evaluation of percutaneous permeation of topically applied drugs. Numerous histopathological studies confirmed its similarity to human skin.45,46 Thickness of the layers, i.e. basale, spinosum and granulosum as well as the number of cell layers in stratum corneum is comparable for human and porcine skin. In et al.46 compared the thickness of abdomen human and porcine skin. The overall thickness of epidermis was about 60 and 54 μm, thickness of stratum basale – 9.8 and 10 μm, stratum spinosum – 32.9 and 34.4 μm, for human and porcine skin, respectively. Whereas the thickness of stratum granulosum was 3.2 μm for both human and pig skin. The stratum corneum of both species is very similar and consists of dense flatted epithelial cells. The hair follicle structure of porcine skin is also very similar to that of human. As in the structure of human skin, the sebaceous glands of porcine skin are associated with the hair follicle.45,46 It should be added that results of numerous studies suggested that porcine skin as well as skin obtained from other animals (guinea pig, rats) seem to be also useful models to evaluation of percutaneous absorption for topically applied chemicals.47–49 Artificial membranes models are also developed to eliminate natural tissue. Luo et al.50 conducted comparative study of ibuprofen permeation using a skin PAMPA model and silicone membrane with human and porcine tissue. They found the better permeation of ibuprofen through PAMPA than through human or porcine skin. Additionally, the permeation data with using PAMPA were comparable to the results through silicone membrane. Moreover, authors demonstrated that the use of artificial membranes was associated with greater repeatability of experimental results than with porcine and human skin. The variable results for natural tissue are caused by their biological greater complexity but are more relevant to real conditions of using.
In our studies the penetration of new ibuprofen derivatives was compared with the penetration of the parent acid. Permeation through porcine skin at 32 °C was studied using the Franz diffusion cell device. The donor phase was a solution of the compound that was tested in 70% (v/v) ethanol. Ethanol at a concentration of 70% was chosen as the donor solvent because ibuprofen and its salts were freely soluble in it. Moreover, this concentration of ethanol is an optimal vehicle for transepidermal application of the drug.51,52 A higher ethanol concentration, above 70%, caused dehydration of the skin and decreased the drug penetration.53 The acceptor phase was buffer pH 7.4.
The cumulative mass of the ibuprofen in the acceptor phase after 24 h of permeation (Table 5) was higher in application onto the skin the ethanol solution of [ValOPr][IBU] (382.35 μg IBU cm−2) or [ValOiPr][IBU] (341.20 μg IBU cm−2) compared to application of the parent acidic form (302.84 μg IBU cm−2). For [ValOAm][IBU] (308.96 μg IBU cm−2) the cumulative mass was similar to that for ibuprofen and for the other salts was the lower. The lowest cumulative mass was determined for [ValOEt][IBU] (215.19 μg IBU cm−2). However, expressing the permeation as % applied dose of IBU (Table 5), reveals that the application of each salt gives higher part of permeated ibuprofen than the use of unmodified ibuprofen. For the ethanolic solution of ibuprofen salts, between 7.33% and 13.55% of ibuprofen in donor phase was permeated to the acceptor phase, while for acidic form only 6.06% of applied dose.
Table 5 Permeation as cumulative mass of compound or ibuprofen (IBU) and as % applied dose of IBU, after 24 h permeation from ethanolic solution into acceptor phase (mean values ± SD with n = 3)
Compound |
Cumulative mass of compound (μg cm−2) |
Cumulative mass of IBU (μg IBU cm−2) |
% applied dose of IBU |
Value is significantly different from control (ibuprofen) (P < 0.05). Value is significantly different from control (ibuprofen) (P < 0.001). |
rac-IBU |
302.84 ± 3.63 |
302.84 ± 3.63 |
6.06 |
[ValOEt][IBU] |
366.64 ± 8.14a |
215.19 ± 8.14a |
7.33 |
[ValOiPr][IBU] |
604.51 ± 4.87b |
341.20 ± 4.87b |
12.09 |
[ValOPr][IBU] |
677.41 ± 1.05b |
382.35 ± 1.05b |
13.55 |
[ValOBu][IBU] |
533.00 ± 1.05b |
289.74 ± 1.05b |
10.66 |
[ValOAm][IBU] |
589.31 ± 3.77b |
308.96 ± 3.77b |
11.79 |
[ValOHex][IBU] |
490.33 ± 26.06b |
249.46 ± 26.06b |
9.81 |
The flux of ibuprofen and its salts through the skin was determined from the slope of the plot of cumulative mass in the acceptor phase over time (ESI, S51 and S52†). The flux was expressed as the amount of compound or active ibuprofen per skin area and time (μg cm−2 h−1). As shown in Fig. 4, improved rate of permeation of ibuprofen by pairing it with ValOPr, ValOiPr, ValOBu, or ValOAm was achieved. These salts showed the faster permeation of ibuprofen through the skin. For the best salt, [ValOPr][IBU], the flux was 18.555 μg IBU cm−2 h−1 compared to 13.644 μg IBU cm−2 h−1 for unmodified acid. Salts of ibuprofen with ValOHex and ValOEt showed a lower ibuprofen flux. There are some data about the flux for various ibuprofen salts in the literature, but permeation experimental conditions were different than those in this study. For example, Wu et al.27 demonstrated a four–five-fold increase in flux for [N1,1,10,10][IBU] and [N6,6,6,6][IBU] compared to IBU (0.542 μg cm−2 h−1) using Britton–Robinson buffer at pH 7.4 as an acceptor and donor solution. Sarveiya et al.26 measured the steady-state flux through a PDMS membrane with a buffer at pH 7.0 as the acceptor phase for the ibuprofen triethylammonium salt, which was over 15-fold higher than for sodium ibuprofenate. Furukawa et al. reported that the rate of permeation of ibuprofen-ProOEt through pig skin was ten-times higher than for free ibuprofen and variation in skin permeation depends on strong ion-pairing between a cation and an anionic drug.28
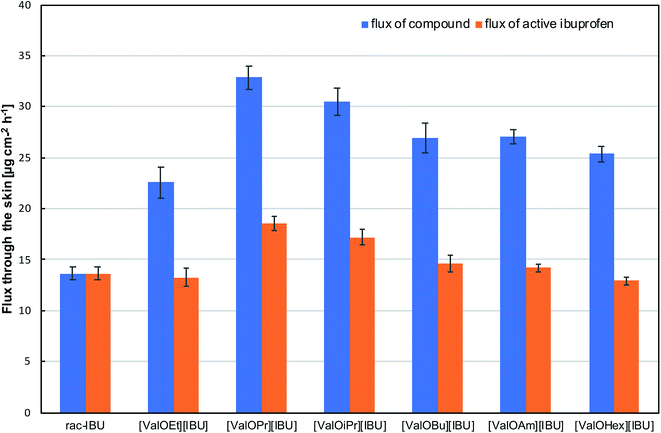 |
| Fig. 4 Flux through the skin for ibuprofen and ibuprofen derivatives (blue bars refer to the flux of compound in μg cm−2 h−1; orange bars refer to flux of active ibuprofen in μg IBU cm−2 h−1). | |
In Fig. 5, the relationship between the flux and log
P in the octanol/buffer 7.4 system for salts of ibuprofen and unmodified ibuprofen is presented. The data in Fig. 5 show that there is a maximum flux for a series of ibuprofen salts when log
P is between 1.172 and 1.197. A similar log
P value (1.18) was found for triethylammonium ibuprofenate, which is characterised by the highest flux from the series of ethylamine salts of ibuprofen.26 Among the obtained salts, the least lipophilic was [ValOEt][IBU], with log
P of 1.026, and the most lipophilic was [ValOHex][IBU], with log
P of 1.279, which provided lower rate of permeation of ibuprofen than the parent acid.
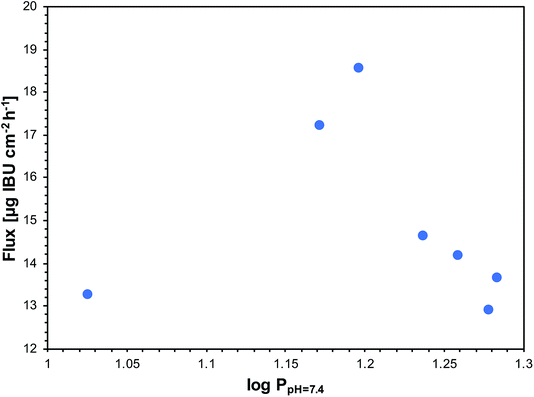 |
| Fig. 5 The relationship between flux and the log P in octanol/pH 7.4 system for ibuprofen and its L-valine ester derivatives. | |
The obtained data for two salts, [ValOPr][IBU] and [ValOiPr][IBU], were the best for topical use. They provided the highest amount of transported ibuprofen and the highest rate of permeation. In practice, this means quickly obtaining the relevant concentration to overcome pain. The salts used were also a source of L-valine, which is an additional advantage of these derivatives.
Skin accumulation
The compound may both penetrate and accumulate in the skin. Table 6 shows the mass of ibuprofen that accumulated in porcine skin in 24 hours, expressed in μg IBU g−1 of skin. In most salts applied in donor phase, a lower mass of ibuprofen in the skin was found compared with applied ibuprofen free acid. The exception was [ValOBu][IBU] for that a slightly higher accumulation of ibuprofen per mass of skin compared to the unmodified acid was measured. However, expressing the accumulation as percentage of applied dose of IBU in form of salt or acid in donor phase, it can be found that all used salts reveal increased skin accumulation of IBU. It was observed the regular increase of IBU accumulation in the range of 3.5% to 7.0% of applied dose of IBU with increase of alkyl chain from ethyl to butyl in valine moiety. Further elongation of alkyl chain resulted in decrease of skin accumulation to about 5.0% applied IBU dose for [ValOAm][IBU] and [ValOHex][IBU]. All used salts reveal increased skin accumulation of IBU compared to the use of parent acid (3.1% of applied dose).
Table 6 Skin accumulation, expressed as μg of IBU per g of skin, and % applied dose of IBU, after 24 h skin permeation of ibuprofen free acid and its salts with L-valine esters from ethanolic solution into acceptor fluid pH 7.4 (mean values ± SD with n = 3)
Compound |
Skin accumulation (μg IBU g−1) |
% applied dose of IBU |
Value is significantly different from control (ibuprofen) (P < 0.05). Value is significantly different from control (ibuprofen) (P < 0.001). |
rac-IBU |
745.69 ± 61.87 |
3.1 |
[ValOEt][IBU] |
412.19 ± 38.45 |
3.5 |
[ValOiPr][IBU] |
630.75 ± 61.87a |
4.9 |
[ValOPr][IBU] |
726.42 ± 113.37a |
5.9 |
[ValOBu][IBU] |
815.14 ± 58.92b |
7.0 |
[ValOAm][IBU] |
565.60 ± 71.09a |
5.0 |
[ValOHex][IBU] |
612.86 ± 129.33a |
4.8 |
Toxicological aspects of new ibuprofen conjugates are very important and studies known from the literature for similar structures allow to predict the tendencies of changes in toxicity of synthesized ibuprofen conjugates with valine alkyl esters.
In general, as the literature data show, pairing a drug with hydrophilic cations, gives combinations with decreased log
P values which results in similar or less toxicity than for the unchanged form of the drug. In contrast, combinations of ibuprofen with lipophilic cations, with increased log
P values, proved to be more toxic than ibuprofen, which was the result of the high cytotoxicity of the cation itself. EC50 values for conjugates with lipophilic cations were two-three orders of magnitude lower than for that with hydrophilic cations.27 Counterions with longer alkyl chains and higher log
P values may interact with cell membrane more extensively and cause more severe cytotoxicity.
Toxicity increases with increasing lipophilicity. Hydrophilic cations reduced the lipophilicity and slightly reduced the cytotoxicity. It has been also shown that pairing of salicylic acid with the ethyl esters of three different amino acids – L-ProOEt, L-AlaOEt and L-AspOEt, proved less cytotoxicity, determined on two mammalian cell lines – L929 and HeLa, than that determined for the native acid. When amino acid cations were incorporated into toxic salicylic acid, the cytotoxicity decreased.54
The alkyl chain length in the ester moiety of L-valine in the presented ibuprofen conjugates may affect the final toxicity. However, taking into account the low values of log
P for all salts (0.992 to 1.883), and lower than for acidic ibuprofen, it seems that the cytotoxicity should not change in the broad range with increase of alkyl chain length. Moshikur et al.54 demonstrated, that among four esters of L-proline (Me, Et, Pr and Bu), methyl and ethyl ester showed similar and the lowest cytotoxicity. Toxicity of the propyl ester was only slightly higher than Et and Me esters, and the highest was for the butyl ester. However, even for butyl ester of L-proline the IC50 values (in HeLa cells test) were only about 3-times lower than for the least toxic ethyl ester.
Moreover, Furukawa et al.28 studies have shown that even at high concentrations of proline ethyl ester, the viability of L929 cell lines decreases only by 20% and the toxicity of proline ester is only slightly higher than that of proline itself.
Therefore, ibuprofen conjugates with valine ester counterions, which have been shown to have lower lipophilicity than native ibuprofen, are expected to have lower toxicity. Due to a number of properties of valine, which has a positive effect on the body, but also repair the skin, they can also reduce the toxicity of ibuprofen.
Conclusion
We demonstrated that new ionic pairs of ibuprofen with the biocompatible counterions of L-valine alkyl ester, where the alkyl chain was between ethyl and hexyl, can enhance ibuprofen transport through biological membranes. They are characterised by better solubility in water and buffer (pH 5.4 and 7.4) solutions and a lower partition coefficient compared to the parent acid, which can have a positive impact on ibuprofen bioavailability with different administration routes. In vitro permeation experiments through porcine skin showed the best ibuprofen permeability for pairs with L-valine propyl and isopropyl ester, in which a higher rate of transport and mass of permeated ibuprofen were achieved. Besides better absorption of drug by penetrating faster and more completely, the presented ibuprofen salts with valine ester can provide dietary supplementation with exogenous L-valine, which is their superiority to the known salts of ibuprofen providing a host of various advantages. L-Valine has a positive effect on the body's functions, including skin repairs effect. It provides the body with energy, reduces fatigue and is necessary for the synthesis of pantothenic acid, which accelerates wound healing.
Conflicts of interest
There are no conflicts of interest to declare.
References
- E. M. Laska, A. Sunshine, I. Marrero, N. Olson, C. Siegel and N. McCormick, The correlation between blood levels of ibuprofen and clinical analgesic response, Clin. Pharmacol. Ther., 1986, 40(1), 1–7, DOI:10.1038/clpt.1986.129
. - K. A. Levis, M. E. Lane and O. I. Corrigan, Effect of buffer media composition on the solubility and effective permeability coefficient of ibuprofen, Int. J. Pharm., 2003, 253, 49–59, DOI:10.1016/s0378-5173(02)00645-2
. - H. Potthast, J. B. Dressman, H. E. Junginger, K. K. Midha, H. Oeser, V. P. Shah, H. Vogelpoel and D. M. Barends, Biowaiver Monographs for Immediate Release Solid Oral Dosage Forms: Ibuprofen, J. Pharm. Sci., 2005, 94(10), 2121–2131, DOI:10.1002/jps.20444
. - L. R. Shaw, W. J. Irwin, T. J. Grattan and B. R. Conway, The effect of selected water-soluble excipients on the dissolution of paracetamol and ibuprofen, Drug Dev. Ind. Pharm., 2005, 31, 515–525, DOI:10.1080/03639040500215784
. - R. M. Watkinson, C. Herkenne, R. H. Guy, J. Hadgraft, G. Oliveira and M. E. Lane, Influence of Ethanol on the Solubility, Ionization and Permeation Characteristics of Ibuprofen in Silicone and Human Skin, Skin Pharmacol. Physiol., 2009, 22, 15–21, DOI:10.1159/000183922
. - P. Bustamante, M. A. Peña and J. Barra, The modified extended Hansen method to determine partial solubility parameters of drugs containing a single hydrogen bonding group and their sodium derivatives: benzoic acid/Na and ibuprofen/Na, Int. J. Pharm., 2000, 194(1), 117–124, DOI:10.1016/s0378-5173(99)00374-9
. - P. Schleier, A. Prochnau, A. M. Schmidt-Westhausen, H. Peters, J. Becker, T. Latz, J. Jackowski, E. U. Peters, G. E. Romanos, B. Zahn, J. Lüdemann, J. Maares and B. Petersen, Ibuprofen sodium dihydrate, an ibuprofen formulation with improved absorption characteristics, provides faster and greater pain relief than ibuprofen acid, Int. J. Clin. Pharmacol. Ther., 2007, 45(2), 89–97, DOI:10.5414/cpp45089
. - P. M. Dewland, S. Reader and P. Berry, Bioavailability of ibuprofen following oral administration of standard ibuprofen, sodium ibuprofen or ibuprofen acid incorporating poloxamer in healthy volunteers, BMC Clin. Pharmacol., 2009, 9, 19, DOI:10.1186/1472-6904-9-19
. - S. E. Nørholt, F. Hallmer, J. Hartlev, L. Pallesen, J. Blomlöf, E. J. Hansen, N. Fernandes, L. Eriksson and E. M. Pinholt, Analgesic efficacy with rapidly absorbed ibuprofen sodium dihydrate in postsurgical dental pain: results from the randomized QUIKK trial, Int. J. Clin. Pharmacol. Ther., 2011, 49, 722–729, DOI:10.5414/cp201553
. - P. Brain, R. Leyva, G. Doyle and D. Kellstein, Onset of analgesia and efficacy of ibuprofen sodium in postsurgical dental pain: a randomized, placebo-controlled study versus standard ibuprofen, Clin. J. Pain, 2015, 31(5), 444–450, DOI:10.1097/ajp.0000000000000142
. - T. Bruzzese and R. Ferrari, Salze von Phenylalkancarbonsäuren mit basischen Aminosäuren, Salts of phenylalkanecarboxylic acids with basic amino acids, Ger. Pat., 2508895, 1975
. - T. Bruzzese and R. Ferrari, Relieving pain and treating inflammatory conditions in warm-blooded animals, US Pat., 4279926, 1981
. - K. C. Kwan, S(+)-ibuprofen-L-amino acid and S(+)-ibuprofen-D-amino acidas onset-hastened enhanced analgesics, US Pat., 5200558, 1993
. - S. Ando and V. J. Stella, Pharmaceutical preparations for taste masking, WO Pat., 03/086293, 2003
. - R. Cristofoletti and J. B. Dressman, Dissolution Methods to Increasing Discriminatory Power of In Vitro Dissolution Testing for Ibuprofen Free Acid and Its Salts, J. Pharmaceut. Sci., 2017, 106, 92–99, DOI:10.1016/j.xphs.2016.06.001
. - R. A. Moore, S. Derry, S. Straube, J. Ireson-Paine and P. J. Wiffen, Faster, higher, stronger? Evidence for formulation and efficacy for ibuprofen in acute pain, Pain, 2014, 155, 14–21, DOI:10.1016/j.pain.2013.08.013
. - J. T. Legg, L. A. Laurent, R. Leyva and D. Kellstein, Ibuprofen Sodium Is Absorbed Faster than Standard Ibuprofen Tablets: Results of Two Open-Label, Randomized, Crossover Pharmacokinetic Studies, Drugs R&D, 2014, 14, 283–290, DOI:10.1007/s40268-014-0070-8
. - P. Desjardins, P. Black, M. Papageorge, T. Norwood, D. D. Shen, L. Norris and A. Ardia, Ibuprofen arginate provides effective relief from postoperative dental pain with a more rapid onset of action than ibuprofen, Eur. J. Clin. Pharmacol., 2002, 58, 387–394, DOI:10.1007/s00228-002-0491-0
. - B. Ahmetaj-Shala, A. Tesfai, C. Constantinou, R. Leszczynski, M. V. Chan, H. Gashaw, G. Galaris, S. Mazi, T. D. Warner and N. S. Kirkby, et al., Pharmacological assessment of on platelet aggregation and colon cancer cell killing, Biochem. Biophys. Res. Commun., 2017, 484(4), 762–766, DOI:10.1016/j.bbrc.2017.01.161
. - N. S. Kirkby, A. Tesfai, B. Ahmetaj-Shala, H. Gashaw, W. Sampaio, G. Etelvino, N. M. Leao, R. A. Santos and J. A. Mitchell, Ibuprofen arginate retains eNOS substrate activity and reverses endothelial dysfunction: implications for the COX-2/ADMA axis, FASEB J., 2016, 30(12), 4172–4179, DOI:10.1096/fj.201600647r
. - I. Tegeder, U. Muth-Selbach, J. Lotsch, G. Rüsing, R. Oelkers, K. Brune, S. Meller, G. R. Kelm, F. Sörgel and G. Geisslinger, Application of microdialysis for the determination of muscle and subcutaneous tissue concentrations after oral and topical ibuprofen administration, Clin. Pharmacol. Ther., 1999, 65(4), 357–368, DOI:10.1016/s0009-9236(99)70128-1
. - A. Patel, M. Bell, C. O'Connor, An. Inchley, J. Wibawa and M. E. Lane, Delivery of ibuprofen to the skin, Int. J. Pharm., 2013, 457, 9–13, DOI:10.1016/j.ijpharm.2013.09.019
. - J. Irvine, A. Afrose and N. Islam, Formulation and delivery strategies of ibuprofen: challenges and opportunities, Drug Dev. Ind. Pharm., 2018, 44(2), 173–183, DOI:10.1080/03639045.2017.1391838
. - M. A. Bolzinger, S. Briancon, J. Pelletier and Y. Chevalier, Penetration of drug through skin, a complex rate-controlling membrane, Curr. Opin. Colloid Interface Sci., 2012, 17, 156–165, DOI:10.1016/j.cocis.2012.02.001
. - A. Baroni, E. Buommino, V. De Gregorio, E. Ruocco, V. Ruocco and R. Wolf, Structure and function of the epidermis related to barrier properties, Clin. Dermatol., 2012, 30, 257–262, DOI:10.1016/j.clindermatol.2011.08.007
. - V. Sarveiya, J. F. Templeton and H. A. E. Benson, Ion-pairs of ibuprofen: increased membrane diffusion, J. Pharm. Pharmacol., 2004, 56, 717–724, DOI:10.1211/0022357023448
. - H. Wu, Z. Deng and B. Zhou, et al., Improved transdermal permeability of ibuprofen by ionic liquid technology: Correlation between counterion structure and the physicochemical and biological properties, J. Mol. Liq., 2019, 283, 399–409, DOI:10.1016/j.molliq.2019.03.046
. - S. Furukawa, G. Hattori and S. Sakai, Highly efficient and low toxic skin penetrants composed of amino acid ionic liquids, RSC Adv., 2016, 6, 87753–87755, 10.1039/c6ra16926k
. - H. Wang, G. Gurau, J. Shamshina, O. A. Cojocaru, J. Janikowski, D. R. MacFarlane, J. H. Davis Jr and R. D. Rogers, Simultaneous membrane transport of two active pharmaceutical ingredients by charge assisted hydrogen bond complex formation, Chem. Sci., 2014, 5, 3449–3456, 10.1039/c4sc01036a
. - B. A. Čuříková, K. Procházková, B. Filková, P. Diblíková, J. Svoboda, A. Kováčik, K. Vávrová and J. Zbytovská, Simplified stratum corneum model membranes for studying the effects of permeation enhancers, Int. J. Pharm., 2017, 534(1–2), 287–296, DOI:10.1016/j.ijpharm.2017.10.038
. - M. M. Badran, J. Kuntsche and A. Fahr, Skin penetration enhancement by a microneedle device (Dermaroller®) in vitro: dependency on needle size and applied formulation, Eur. J. Pharm. Sci., 2009, 36, 511–523, DOI:10.1016/j.ejps.2008.12.008
. - A. Haq and B. Michniak-Kohn, Effects of solvents and penetration enhancers on transdermal delivery of thymoquinone: permeability and skin deposition study, Drug Deliv., 2018, 25(1), 1943–1949, DOI:10.1080/10717544.2018.1523256
. - J. Kuntsche, H. Bunjes, A. Fahr, S. Pappinen, S. Rönkkö, M. Suhonen and A. Urtti, Interaction of lipid nanoparticles with human epidermis and an organotypic cell culture model, Int. J. Pharm., 2008, 354(1–2), 180–195, DOI:10.1016/j.ijpharm.2007.08.028
. - A. Simon, M. I. Amaro, A. M. Healy, L. M. Cabral and V. P. de Sousa, Comparative evaluation of rivastigmine permeation from a transdermal system in the Franz cell using synthetic membranes and pig ear skin with in vivo–in vitro correlation, Int. J. Pharm., 2016, 512(1), 234–241, DOI:10.1016/j.ijpharm.2016.08.052
. - B. H. Furniss, A. J. Hannaford, P. W. G. Smith and A. R. Tatchell, Vogel's Textbook of Practical Organic Chemistry, Longman Scientific & Technical, John Wiley &Sons, Inc., New York, 5th edn, 1989 Search PubMed
. - M. Kopečná, M. Macháček, E. Prchalová, P. Štěpánek, P. Drašar, M. Kotora and K. Vávrová, Galactosyl pentadecene reversibly enhances transdermal and topical drug delivery, Pharm. Res., 2017, 34(10), 2097–2108, DOI:10.1007/s11095-017-2214-3
. - D. J. Davies, R. J. Ward and J. R. Heylings, Multi-species assessment of electrical resistance as a skin integrity marker for in vitro percutaneous absorption studies, Toxicol. In Vitro, 2004, 18(3), 351–358, DOI:10.1016/j.tiv.2003.10.004
. - J. Li and Y. Sha, A Convenient Synthesis of Amino Acid Methyl Esters, Molecules, 2008, 13, 1111–1119, DOI:10.3390/molecules13051111
. - P. Ossowicz, E. Janus, G. Schroeder and Z. Rozwadowski, Spectroscopic Studies of Amino Acid Ionic Liquid-Supported Schiff Bases, Molecules, 2013, 18, 4986–5004, DOI:10.3390/molecules18054986
. - Z. Rozwadowski, Deuterium isotope effects on 13C chemical shifts of lithium salts of Schiff bases amino acids, J. Mol. Struct., 2006, 753, 127–131, DOI:10.1016/j.molstruc.2005.06.005
. - E. Breitmaier and W. Voelter, in Voelter, Monographs in Modern Chemistry, 13C NMR Spectroscopy, ed, H. F. Ebel, Verlag Chemie, Weinheim, 1974 Search PubMed
. - S. Vairam, T. Premkumar and S. Govindarajan, Trimellitate complexes of divalent transition metals with hydrazinium cation, J. Therm. Anal. Calorim., 2010, 100, 955–960, DOI:10.1007/s10973-009-0459-8
. - S. Wilkes, A short history of ionic liquids—from molten salts to neoteric solvents, Green Chem., 2002, 4, 73–80, 10.1039/b110838g
. - S. Forster, G. Buckton and A. E. Beezer, The importance of chain length on the wettability and solubility of organic homologs, Int. J. Pharm., 1991, 72(1), 29–34, DOI:10.1016/0378-5173(91)90377-z
. - U. Jacobi, M. Kaiser, R. Toll, S. Mangelsdorf, H. Audring, N. Otberg, W. Sterry and J. Lademann, Porcine ear skin: an in vitro model for human skin, Skin Res. Technol., 2007, 13, 19–24, DOI:10.1111/j.1600-0846.2006.00179.x
. - M. K. In, K. C. Richardson, A. Loewa, S. Hedtrich, S. Kaessmeyer and J. Plendl, Histological and functional comparisons of four anatomical regions of porcine skin with human abdominal skin, Anat., Histol., Embryol., 2019, 48, 207–217, DOI:10.1111/ahe.12425
. - H. Bando, S. Mohri, F. Yamashita, Y. Takakura and M. Hashida, Effect of skin metabolism on percutaneous penetration of lipophilic drug, J. Pharm. Sci., 1997, 86(6), 759–761, DOI:10.1021/j960408n
. - J. J. Prusakiewicz, C. Ackermann and R. Voorman, Comparison of skin esterase activities from different species, Pharm. Res., 2006, 23(7), 1517–1524, DOI:10.1007/s11095-006-0273-y
. - C. Géniès, E. L. Jamin, L. Debrauwer, D. Zalko, E. N. Person, J. Eilstein, S. Grégoire, A. Schepky, D. Lange, C. Ellison, A. Roe, S. Salhi, R. Cubberley, N. J. Hewitt, H. Rothe, M. Klaric, H. Duplan and C. Jacques-Jamin, Comparison of the metabolism of 10 chemicals in human and pig skin explants, J. Appl. Toxicol., 2019, 39, 385–397, DOI:10.1002/jat.3730
. - L. Luo, A. Patel, B. Sinko, M. Bell, J. Wibawa, J. Hadgraft and M. E. Lane, A comparative study of in vitro permeation of ibuprofen in mammalian skin, the PAMPA model and silicone membrane, Int. J. Pharm., 2016, 505(1–2), 14–19, DOI:10.1016/j.ijpharm.2016.03.043
. - A. Zhang, E. C. Jung, H. Zhu, Y. Zou, X. Hui and H. Maibach, Vehicle effects on human stratum corneum absorption and skin penetration, Toxicol. Ind. Health, 2016, 33(5), 416–425, DOI:10.1177/0748233716656119
. - A. Rashid, E. T. White, T. Howes, J. D. Litster and I. Marziano, Effect of Solvent Composition and Temperature on the Solubility of Ibuprofen in Aqueous Ethanol, J. Chem. Eng. Data, 2014, 59, 2699–2703, DOI:10.1021/je400819z
. - R. M. Watkinson, C. Herkenne, R. H. Guy, J. Hadgraft, G. Oliveira and M. E. Lane, Influence of ethanol on the solubility, ionization and permeation characteristics of ibuprofen in silicone and human skin, Skin Pharmacol. Physiol., 2009, 22, 15–21, DOI:10.1159/000183922
. - R. M. Moshikur, M. R. Chowdhury, R. Wakabayashi, Y. Tahara, M. Moniruzzaman and M. Goto, Characterization and cytotoxicity evaluation of biocompatible amino acid esters used to convert salicylic acid into ionic liquids, Int. J. Pharm., 2018, 546, 31–38, DOI:10.1016/j.ijpharm.2018.05.021
.
Footnote |
† Electronic supplementary information (ESI) available. See DOI: 10.1039/d0ra00100g |
|
This journal is © The Royal Society of Chemistry 2020 |