DOI:
10.1039/D0RA00001A
(Review Article)
RSC Adv., 2020,
10, 12554-12572
Anammox and partial denitrification coupling: a review
Received
1st January 2020
, Accepted 12th March 2020
First published on 27th March 2020
Abstract
As a new wastewater biological nitrogen removal process, anammox and partial denitrification coupling not only plays a significant role in the nitrogen cycle, but also holds high engineering application value. Because anammox and some denitrifying bacteria are coupled under harsh living conditions, certain operating conditions and mechanisms of the coupling process are not clear; thus, it is more difficult to control the process, which is why the process has not been widely applied. This paper analyzes the research focusing on the coupling process in recent years, including anammox and partial denitrification coupling process inhibitors such as nitrogen (NH4+, NO2−), organics (toxic and non-toxic organics), and salts. The mechanism of substrate removal in anammox and partial denitrification coupling nitrogen removal is described in detail. Due to the differences in process methods, experimental conditions, and sludge choices between the rapid start-up and stable operation stages of the reactor, there are significant differences in substrate inhibition. Multiple process parameters (such as pH, temperature, dissolved oxygen, redox potential, carbon-to-nitrogen ratio, and sludge) can be adjusted to improve the coupling of anammox and partial denitrification to modify nitrogen removal performance.
1 Introduction
With the development of the economy and public awareness of environmental protection, the pollution of water environments has become the central topic of academic discussion. The deterioration of water quality caused by the accumulation of inorganic nitrogen is a typical and serious pollution source, and it can influence human and animal health directly or indirectly.1,2 In recent years, high nitrogen removal has been a focus point in water treatment. NO3−, NO2− and NH4+ are the main living style of the cycle network of inorganic nitrogen in the biosphere (Fig. 1) in nature. Nitrogen removal in sewage treatment means that different forms of nitrogen are converted into nitrogen and discharged into air. Familiar nitrogen removal processes include nitrification and denitrification, partial nitrification and denitrification, the anammox process, and the anammox and partial denitrification coupling process. In these processes, anammox and partial denitrification coupling is a clean and environment-friendly approach, which can remove ammonia nitrogen, nitrate nitrogen and nitrous nitrogen at the same time. In one case, a novel partial denitrification anammox coupling process achieved a high total nitrogen (TN) removal rate of 97.8%.3 The development of the anammox and partial denitrification coupling technology shows great significance for the establishment of a relatively complete denitrification mechanism and a new nitrogen cycle system.4 The coupling mechanism is shown in Fig. 2: (1) Denitrification provides carbon sources and substrates for anammox. During the denitrification process, NO3−, as an electron acceptor, generates NO2− under enzymatic action and inhibits NO2− reductase to reduce N2O production, where organic matter is consumed and CO2 is generated.2,5 (2) Partial denitrification consumes dissolved oxygen (DO) during the consumption of organic matter to provide a low-oxygen environment for anammox;6 CO2 from denitrification is used as a carbon source for anammox. (3) Anammox consumes the substrate NO2−, reducing the effects of NO2− accumulation on denitrification and avoiding the further reduction of NO2− to N2O. Anammox bacteria use NO2− and NH4+ as substrates that are reduced and oxidized to N2, and the released energy is used for CO2 synthesis of organic matter and life activities.7,8
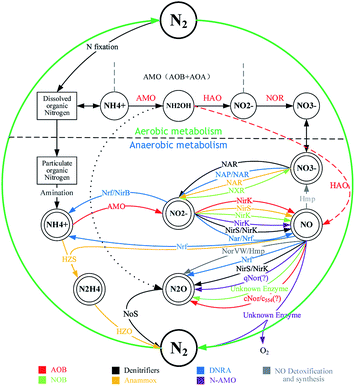 |
| Fig. 1 Nitrogen biosphere cycle network. | |
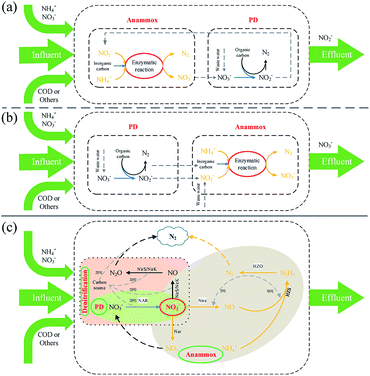 |
| Fig. 2 Anammox and partial denitrification coupling nitrogen removal matrix flow direction. | |
In 1998, Strous et al.9 cultivated anammox bacteria in a sequencing batch reactor and proposed a stoichiometric formula for the anammox metabolism (eqn (1)). Although many quantitative methods of anammox metabolism have been proposed in recent years, such as that by Lotti et al.,10 the growth mechanism of suspension of anammox bacteria and the study of the kinetics of eqn (2) and (1) have a wide range of applications and are readily accepted by scholars.
|
NH4+ + 1.32NO2− + 0.66HCO3− + 0.13H+ → 0.066CH2O0.5N0.15 + 1.02N2 + 0.26NO3− + 2.03H2O
| (1) |
|
NH4+ + 1.146NO2− + 0.071HCO3− + 0.057H+ → 0.071CH1.74O0.31N0.20 + 0.986N2 + 0.161NO3−+2.0002H2O
| (2) |
|
NO3− + 0.083C6H12O6 → NO2− + 0.5CO2 + 0.5H2O
| (3) |
As shown by eqn (1) and (2), NO3− produces about 11.2% of the total nitrogen after consuming 1 mol of NH4+. Although nitrogen in sewage cannot be eliminated, it supplements the consumption of NO3− in the denitrification process.6 Therefore, the operating costs of wastewater treatment plants (WWTPs) are reduced.11–14 Through the anammox and partial denitrification coupling process, the NO3−–N removal efficiency can reach 95.8%, while the NH4+–N removal rate reaches 92.8% and the anammox contribution to TN removal is 78.9%.13 The process meets the requirements of smaller space, simple control and environmental friendliness; furthermore, it creates no secondary pollution.7
2 Anammox and partial denitrification coupling process and application
Anammox and partial denitrification coupling nitrogen removal is divided into separated and combined processes (Table 1).
Table 1 Experimental studies on separated and combined processes
Process |
Reactor |
Operating parameter |
Nitrogen removal effect |
Reference |
C/N |
Carbon source |
Influent |
T/°C |
Effluent TN/mg L−1 |
NO3−–N removal rate/% |
NTR |
Anammox denitrification contribution rate/% |
TN removal rate/% |
Separated process |
PD (SBR) |
3.0/2.8/2.6 |
Sewage |
NO3−–N = 40 |
18.7–27.8 |
4 |
97.9 |
85.6 |
78.2 |
— |
7 |
Anammox (UASB) |
NH4+–N = 59.3 |
PD (SBR) |
Low |
Sewage |
NH4+–N = 166 |
12.9–15.1 |
8 |
89.5 |
55.1 |
70 |
— |
12 |
Anammox (UASB) |
NO3−–N = 50 |
PD (SBR) |
0.5–1.7 |
Septic tank |
NH4+–N = 40 |
14.8–28.2 |
11 |
95.8 |
80 |
78.9 |
— |
13 |
Anammox (UASB) |
NO3−–N = 125 |
PD (SBR) |
3.0 |
Sodium acetate |
NO3−–N = 30–400 |
23.6–28.8 |
— |
92.5 |
83.3 |
— |
— |
30 |
Anammox (USB) |
|
PD (SBR) |
2.9 |
Sodium acetate |
NH4+–N = 15 |
25 ± 3 |
5.8 |
— |
80 |
49.8 |
90 |
17 |
Anammox (UASB) |
NO3−–N = 20 |
PD (SBR) |
3.0 |
Septic tank |
NH4+–N = 70.1 |
28 |
≤20 |
— |
80 |
— |
97.8 |
15 |
Anammox (UASB) |
NO3−–N = 20 |
Combined process |
UASB |
3.0/3.4 |
Sodium acetate |
NH4+–N = 30 |
17.3–28.8 |
10 |
89.5 |
97.1 |
9.23 |
93.2 |
25 |
NO3−–N = 30 |
SBR |
3.0 |
Landfill leachate |
NH4+–N = 47.5 |
30 ± 1 |
≤20 |
— |
75.6 |
— |
84.8 |
21 |
NO3−–N = 93.7 |
UASB. |
1.6 |
Septic tank |
NH4+–N = 67.7 |
26 ± 2 |
<10 |
83.3 |
— |
95.6 |
93.1 |
27 |
NO3−–N = 70–180 |
SBR |
3.0 |
Acetate solution |
NH4+–N = 50 |
12.7–29.2 |
15 |
91.5 |
87 |
95 |
93.6 |
18 |
NO3−–N = 50 |
SBBR |
2.0–2.5 |
Sodium acetate |
NH4+–N = 151 |
25–35 |
12.5 |
>90 |
— |
— |
93 |
19 |
NO3−–N = 165 |
UASB |
1.1 |
Sodium acetate |
NH4+–N = 280 |
34–36 |
9.3 |
— |
97.4 |
85.8 |
96.2 |
20 |
NO3−–N = 280 |
2.1 Separated process
The separated process of anammox and partial denitrification coupling is inoculated with denitrifying bacteria and anammox with absolute advantages in two mutually independent reactors and simultaneously generates NO2−–N and nitrogen removal processes, which can effectively avoid space competition. Organic matter is fully utilized by denitrifying bacteria in the denitrification reactor, reducing the concentration of organic matter flowing to the anammox reactor. Wastewater containing nitrate (such as municipal wastewater, industrial wastewater, and aquaculture wastewater) first flowed into the partial denitrification reactor to reduce nitrate to NO2−–N while consuming organic matter as the electron donor. Then, the wastewater NO2− was adjusted before flowing into the anammox reactor (NO2−–N/NH4+–N = 1.32) and was used to remove nitrogen, and part of the effluent returned to the partial denitrification reactor for deep nitrogen removal.13
Cao et al.15 used a separated reactor to treat high-concentration nitrogen-containing wastewater (NO3−–N = 820 mg L−1) in which the TN in the effluent was less than 20 mg L−1, and the TN removal rate was as high as 97.8%. It is demonstrated that the removal rate of NH4+–N peaks at 96.7% when treating domestic sewage, and this process can effectively solve the problem of nitrogen residues in sewage. Du et al.13 optimized the mixed volume ratio of influent NO3−–N and sewage and the amount of organic matter to treat high-nitrogen wastewater (NO3−–N = 1000 mg L−1); the TN in the effluent was less than 11 mg L−1, and the removal rate of TN was as high as 95.8%. Meanwhile, the contribution rate of anammox to TN nitrogen removal was 78.9%. In addition, Wang et al.16 proposed a new process, EPDPR (endogenous partial denitrification and denitrifying phosphorus removal), in which the removal rate of phosphorus is as high as 92.3% and the output of 79.2% of NO2−–N is the best for the post-anammox process. The suitable substrate ratio (NO2−–N/NH4+–N = 1.32) and high purification capacity (TN < 6 mg L−1, TP < 0.4 mg L−1) provide new ideas for removing nitrogen, phosphorus and other nutrients from sewage. A mainstream literature search showed that the reason why the separated process is stable and the TN removal rate is high is that anammox makes a greater contribution to the TN removal rate.7,12,13,17
2.2 Combined process
The combined process of anammox and partial denitrification coupling is started by inoculating the optimal partial denitrification floc sludge and anammox granular sludge,18–20 and it has also been reported that the inoculated partial denitrification sludge is acclimated from PN sludge.21
Zhang et al.20 reported a new process called completely anaerobic ammonium removal over nitrite (CAARON); they compared the simultaneous addition of acetate at low C/N with that of asynchronous addition and confirmed that the asynchronous addition of acetate optimized the reactor. The distribution of the bacterial nitrate-to-nitrite transformation ratio (NTR) was 97.4%, and the anammox nitrogen removal contribution rate was 85.8%. Also, Du et al.22 carried out the DEAMOX reaction in an SBR reactor, and supplying acetate to the reactor at different periods also achieved a nitrogen removal rate of up to 95.8%. Zhang et al.23 studied the engineering feasibility, economic feasibility, and potential challenges of the PN/AMX and PDN/AMX processes. The researchers noted that bacteria showed good capture of soluble COD; this is another way to control the effects of organic matter on anammox bacteria. Qin et al.24 focused on the effects of glucose on DEAMXO nitrogen removal performance in an upstream continuous consideration reactor and confirmed that dissolved COD had a significant effect on the biological communities. When the concentration of glucose was 56.4 mg L−1, the system TN removal rate was 98.6%.
2.3 Reactor management
The method of managing the reactor in the separated and combined processes is as follows:
(1) Selection of reactor type and seed sludge. Given that the processing has low carbon demand and minimal sludge production, researchers usually use SBR or UASB as the anammox and partial denitrification coupling starting reactor.18,20,21,25 Anammox granular sludge and partial denitrification floc sludge are inoculated as seed sludge, or anammox sludge is domesticated to obtain the target strain.
(2) Optimization of hydraulic retention time (HRT) and proper ventilation. Properly increasing wastewater HRT in the reactor can afford higher TN and COD removal rates, and even difficult-to-degrade COD will be consumed in a higher HRT. This method effectively enriches a sufficient amount of partial denitrification and anammox functional microorganisms.13,19,25 Optimizing aeration can avoid excessive production of extracellular polymers (EPS), which is beneficial to the stability of the microbial community and nitrogen removal.25
(3) Selection of organic matter feeding methods and addition of microbial carriers. Organic matter feeding methods are distributed continuously and intermittently. The richness and metabolism of continuous feeding of organic matter anammox bacteria will be limited, reducing the nitrogen removal ability of the reactor. Intermittent feeding is widely used in the combined process and performs well, with a high TN load nitrogen removal effect.20,22 Partial denitrification and anammox bacteria can be effectively retained on the microbial carrier, expand the specific surface area of the microorganism, increase the mass transfer rate, and enhance the nitrogen removal effect.26,27
2.4 Engineering application
Research of the anammox and partial denitrification coupling process is still in its infancy. The process has not been popularized or applied to nitrogen removal for municipal wastewater treatment; therefore, it has been rarely reported to date. Although the engineering application of anammox has been increasing gradually in the past 10 years, the development of anammox and some denitrification coupling processes have lagged, and laboratory-scale research7,13,25,28 has a positive effect on engineering applications.
Wang et al.29 reported simultaneous partial nitrification, anaerobic ammonium oxidation and denitrification (SNAD) treatment of landfill leachate in a full-scale (192 m3) sewage treatment plant. The TN removal was around 83.9%, which confirms that mass balance of the system is the best approach for the evaluation of anammox and nitration processes and that molecular tools and tracer studies with labeled nitrogen are useful approaches for the evaluation of anammox. Based on an AAO process, Li et al.26 added carriers to the reactor to enrich anammox bacteria in the anoxic zone, forming the An-AAO process. Anammox species enrichment in situ increases the nitrogen removal rate, and it has been proven that anammox has a high nitrogen removal contribution rate and is the key to the stability and engineering application of the denitrification coupling process, which was successfully used in urban sewage treatment plants.
3 Inhibition factors of the coupling process
3.1 Nitrogen inhibition
NH4+, NO2− and NO3− are important monitoring indicators of wastewater (including domestic sewage, landfill leachate, mustard wastewater, aquaculture wastewater and printing and dyeing wastewater). NO2− and NO3− act as key raw materials and inhibitors in the anammox and partial denitrification coupling process. Their inhibition mechanism and concentration will affect the coupling process, operating conditions and operating strategies; therefore, it is imperative to understand the nitrogen inhibitory concentration and mechanism.
3.1.1 Free ammonia inhibition. One study found that a high concentration of NH4+ has no influence on the anammox process; Strous et al.31 noted that the anammox process showed no inhibition when treating high-concentration ammonia nitrogen wastewater at 1000 mg L−1 NH4+. Isaka et al.32 also mentioned that a high concentration of ammonia (NH4+, 690 mg L−1) shows no significant inhibition of the anammox process during the process of ammonia plant wastewater treatment. However, with deep research into anammox and partial denitrification coupling,33–35 it was found that the above situation is likely caused by the fact that the content of FA (free ammonia, NH3) in the sewage is less than the requirement of the inhibition value; therefore, the inhibitory effect on the anammox process is FA instead of NH4+.Compared with NH4+, FA can diffuse more easily into biological cells through the lipid membrane. In the mechanism of FA inhibition, the FA changes the pH (as eqn (4)) of the intracellular environment after it enters the cells6,35 (intracellular pH < extracellular pH). High or low pH destroys the transmembrane potential and changes the substance exchange selectivity between the cells and the external environment; this will interfere with the synthesis of enzymes, leading to cell inactivation or even death.
|
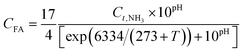 | (4) |
Fernández et al.36 analyzed the short-term and long-term effects of FA on the anammox process. As a result, with short-term exposure, as the concentration of FA increased from 38 to 100 mg L−1, the metabolic activity was inhibited by 50% to 80%; these inhibition concentrations (ICs) were recorded as the IC50 and IC80, respectively. However, in their study of long-term effects, anammox showed decreased nitrogen removal performance when the FA concentration was only 35 to 40 mg L−1. Summarized from many related research studies (Table 2), the activity threshold (initiation inhibition concentration) of FA on anammox is 20 to 25 mg L−1, and FA < 5 mg L−1 can afford higher nitrogen removal efficiency.
Table 2 Research on the inhibitory threshold concentration of FA for anammox and denitrifying bacteria
Reactor |
T/°C |
pH |
HRT/d |
Sludge source |
NH4+/(mg L−1) |
Time/d |
Threshold |
Reference |
UASB |
33–35 |
— |
0.375–0.5 |
Nitrification/denitrification 1 : 1 |
50–400 |
187 |
29.65 mg L−1 |
42 |
SBR |
29 |
— |
1.35–2.57 |
ELAN granular sludge |
500–1000 |
175 |
23.55 mg L−1 |
43 |
MBR |
25 ± 1 |
8.0 |
0.5 |
Anammox reactor |
100 |
800 |
16–23 mg L−1 |
44 |
MBBR |
34 ± 0.8 |
— |
2 |
Laboratory MBBR |
700 ± 100 |
275 |
25.5 mg L−1 |
45 |
SBR |
32–35 |
7.9 |
0.5 |
EGSB reactor |
429–513 |
220 |
40 mg L−1 |
46 |
SBR |
33/30 |
7.8 |
— |
SBR reactor |
70–2800 |
200 |
35–40 mg L−1 |
36 |
SBR |
30–35 |
7.8 |
— |
UASB reactor |
1615 ± 68.5 |
131 |
77 mg L−1 |
47 |
EGSB |
32 |
7.7 |
— |
— |
84–420 |
70 |
>50 mg L−1 |
48 |
The inhibition concentration of FA for denitrifying bacteria was only 7.5 mg L−1 as reported by Vadivelu et al.37 However, Nair et al.151 and Waki et al.38 both noted that a certain concentration of FA can enhance the activity of microorganisms, especially in the denitrification process. Higher concentrations of FA (exceeding the activity threshold) will inhibit biological activity, and long-term loading of denitrifying bacteria and anammox strains will cause large-scale deaths. It can be concluded that FA mainly affects biological activity (inhibition of reversibility) by affecting the biome structure.39,40 FA inhibition can be reduced by changing sewage operating conditions, such as pH, HRT, sludge type and sludge status (particles, biofilm or flocculation). He et al.41 stated that the safe concentration of FA in the reactor increased to 15 mg L−1 (IC10) after pH elevation. Compared with a granular sludge reactor, Yang et al.42 added a composite support to the anammox reactor to increase reactor stability and FA tolerance and proved that nitrogen removal performance could be properly restored by reducing the NH4+ concentration and increasing the hydraulic retention time (HRT). In addition, it is possible to domesticate bacteria under high concentrations of FA by applying restrictive conditions to the bacteria and obtain highly active anammox bacteria.
3.1.2 Free nitrous acid inhibition. In the coupling process of anammox and partial denitrification, both anammox and denitrifying bacteria were inhibited under a certain concentration of NO2−, and the inhibition of anammox was more obvious.11 Numerous studies49–52 have shown that NO2− inhibition is actually derived from the toxic effects of free nitrous acid (FNA), and the FNA concentration is affected by temperature, pH and NO2− concentration (eqn (5)).53 The FNA inhibition rate is about 3 times that of FA; Zhang et al.54 proved that FNA is the largest inhibitor of the coupling process, and the long-term stable operating concentration of the reactor should be controlled to less than 1.5 μg L−1. |
 | (5) |
In the mechanism of FNA inhibition, NO2− acts as a decoupling agent to diffuse into the membrane of prokaryotic cells; the permeability expansion of the cell membrane affects the enzymes involved in electron transport and proton transfer, thereby affecting the inhibition of the synthesis of adenosine triphosphate (ATP).55,56 Finally, it will affect the ATPase-catalyzed reaction and reduce biological activity. Studies have shown that in order to ensure safe and stable operation of the reactor, the IC10 value can usually be used as a safe value,54,57,58 and the FNA concentration should be controlled between 1.5 and 5 μg L−1. In this research, the coupling nitrogen removal efficiency could be improved with proper heating (>25 °C) and low pH (<5.6), which could reduce the effects of the FNA concentration to ensure high activity of the strains.59
FNA inhibits the reduction of N2O to cause partial denitrification and accumulate NO2−. According to the research by Alinsafi et al.,60 the amount of N2O released during wastewater treatment is positively correlated with the concentration of NO2−, which indirectly proves that an increase in FNA concentration can promote N2O reduction. Among the studies of FNA inhibition on denitrifying poly-phosphate accumulating organisms, Zhou et al.61 considered FNA as the main reason for the effects on N2O produced by the denitrification process, and they found that its inhibitory concentration was 0.7 to 1.0 μg L−1. A low concentration of FNA provides mild coupling conditions for denitrification and anammox.
Niu et al.62 proposed that the IC50 of FNA for anammox inhibition was in the range of 1.08–2.81 μg L−1, and it showed a higher nitrogen removal rate under FNA <2 μg L−1. The inhibition of FNA can be reduced by changing the spatial structure (filler or carrier) of the biome, sludge state and operating conditions. Yang et al.42 used shale ceramic particles and suspended spheres as carrier-loaded functional bacteria; their research showed that the initial value of FNA inhibition was as low as 77.02 μg L−1 and the anti-toxic performance was much lower than the anammox granular sludge IC50 (117 μg L−1), which matched the research results of Puyol et al.52 He et al.55 observed that the inhibition concentrations of anammox and some denitrifying bacteria communities ranged from 1.5 to 213 μg L−1 (Table 3).
Table 3 Research on the effects of FNA on anammox and denitrifying bacteria
Reactor |
T/°C |
pH |
HRT/h |
NO2−–N mg L−1 |
FNA/μg L−1 |
Sludge |
Effect |
Time/d |
Reference |
UASB |
35 ± 1 |
7.5–7.8 |
12–1.5 |
130–260 |
0.67/2.81 |
Granule sludge |
IC10/IC50 |
900 |
62 |
EGSB |
35 ± 1 |
7.1–7.3 |
1.5–3 |
117.8–710 |
15 |
Digested sludge |
IC10 |
400 |
41 |
UASB |
35 ± 1 |
7.5–7.9 |
1.5–3 |
229.7 |
17 |
Granule sludge |
IC10 |
700 |
63 |
AAFEB |
35 |
7.5–8.5 |
3 |
533.8 |
12–19 |
Biofilm/granule |
IC10 |
300 |
64 |
CSTAn |
25 ± 3 |
7.0–7.3 |
12–24 |
300–400 |
70 ± 10 |
Digested sludge |
Restrain |
180 |
65 |
EGSB |
30 ± 2 |
6.5–7.3 |
— |
160 |
19 |
Granule sludge |
IC10 |
— |
52 |
UASB |
35 ± 1 |
7.5–8.2 |
4 |
210–420 |
6 |
Anammox |
No |
600 |
66 |
SBR1/2 |
30/33 |
7.8 |
24 |
70–420 |
11 |
Biofilm |
IC50 |
200 |
36 |
3.2 Organic inhibition
Organic matter is of great significance to anaerobic and denitrifying bacteria. It acts as a carbon source for the denitrification process and as an inhibitor of the anammox process. There are numerous organic substances in sewage; these can be roughly classified into concentration inhibition type and toxicity inhibition type according to their inhibition mechanisms. Studies on the concentration and inhibition mechanisms of organics on the coupling process will help researchers to adjust the operating conditions of denitrification processes and improve their efficiency.
3.2.1 Concentration inhibition. There are two separate explanations for the mechanism of concentration-inhibiting organic inhibition. The first one is the inhibition of space competition. Within the inhibition of space competition, the organic matter is used by denitrifying bacteria as the carbon source, causing the proliferation rate of denitrifying bacteria to become much higher than that of anammox bacteria. Because the anammox bacteria have reduced living space, the number of bacteria and the nitrogen removal efficiency of anammox experience great reductions.67–69 The second explanation is the inhibition of metabolic pathway diversity. Scholars consider anammox to be the dominant strain in the system under a high concentration of organic matter; however, the metabolic mode can change. In the process, anammox bacteria uses organic matter as a substrate instead of NO2− and NH4+, which reflects the diversity of anammox metabolism.6,67,70 The two processes of anammox and organic oxidation are independent, and the anammox process can be re-expressed under inorganic-dominated conditions.67As shown in many studies, high concentrations of organic matter can inhibit the coupling nitrogen removal process, while lower concentrations can promote the growth and coupling of biomes.67,70,71 However, because the lack of a carbon source will inhibit the growth of denitrifying bacteria, the proper concentration of organic matter is vital to the anammox and coupling denitrification process; the effects of concentration on the anammox and denitrifying bacteria are shown in Table 4. Du et al.13 calculated the contribution rates of several nitrogen removal processes. It was found that the anammox nitrogen removal contribution rate was 78.9%, and the domination of the anammox process was the real reason for the coupling and stable operation. When treating high-concentration organic wastewater (landfill leachate, mustard wastewater, etc.), it is possible to conclude that the appropriate addition of non-toxic organic substances (such as acetate, glucose and skim milk powder) can improve the activity of anammox and denitrifying bacteria and improve their coupling effects.16,72,73 The largest contribution rate of anammox during treatment of landfill leachate was only 23.4%, recorded by Wei et al.28 The main reason is that functional COD in sewage is beneficial to the growth of denitrifying bacteria and inhibits the development of the anammox community. Continuing to inoculate sludge and optimize the influent parameters can promote the growth and reproduction of anammox bacteria and shorten the reactor startup time. Feng et al.74 studied the metabolism of anammox bacteria and found that under the condition of higher organic concentration, more energy consumption was required to produce EPS and amino acids accumulated in the cells, which reduced the contribution rate of anammox to nitrogen removal.
Table 4 Research on the inhibitory effects of the organic matter concentration on anammox and denitrifying bacteria
Reactor |
T/°C |
pH |
HRT/d |
Sludge |
Carbon source |
c (mg L−1) |
Effect |
Time/d |
Reference |
MBfR |
22 ± 2 |
7.0–8.0 |
1.1 |
Biofilm |
Methane |
0.24–1.21 atm |
Increased activity |
70 |
75 |
UASB |
35 |
7.8–8 |
1.5 |
Granule sludge |
Skim milk powder |
0–400 |
C/N threshold value 3.1 |
30 |
76 |
— |
20 |
7.4 |
2.6 |
Activated sludge |
Pig wastewater |
2200/6.4 |
No |
25 |
38 |
USB |
28–35 |
7–8 |
— |
Mixed sludge |
Landfill leachate |
1400–2000 |
Restraint |
90 |
14 |
UASB |
25–32 |
7.9 |
0.67 |
Activated sludge |
Livestock wastewater |
254 ± 115 |
No |
190 |
68 |
EGSB |
35 ± 1 |
— |
— |
Anammox sludge |
Glucose |
C/N: 1.6 |
Increased activity |
91 |
77 |
— |
30 ± 1 |
7.2 |
1–2 |
Granule sludge |
Sodium acetate |
0–40 |
Increased activity |
400 |
73 |
SBR |
35 |
7.5 |
— |
Biofilm |
Methane |
Enough |
Restraint |
200 |
78 |
3.2.2 Toxicity inhibition. Inhibition of toxic organics occurs due to irreversible poisoning of microorganisms or inactivation of key enzymes. Several common toxic organics are alcohols, aldehydes, phenols and antibiotics.
(1) Alcohols, aldehydes and phenols. The main toxic alcohols are methanol and ethanol. The inhibition mechanisms are that methanol will be converted to aldehydes by methanol dehydrogenase, and ethanol will be converted to acetyl coenzyme before being oxidized to acetaldehyde.79 As noted in previous research, there was no accumulation of NO2− in a reactor with added methanol; the contribution rate of anammox denitrification continuously decreased, and the coupling system was seriously inhibited. Peng et al.80 analyzed the methanol in the reactor experimentally; they found that anammox had no activity at 3 to 4 mmol methanol, and the inhibition was irreversible.Phenols originate from manufacturing wastewater (such as paper, textile and pharmaceutical); also, there are small amounts of phenols in urban sewage. Lyu et al.81 stated that when the phenol concentration was set at 400 mg L−1 and 1000 mg L−1 in intermittent experiments, the anammox removal rate decreased by 56% and 76%, respectively. Through inhibiting the activity of anammox strains and changing the microbial community structure, the experiment reduced the nitrogen removal rate. Studies by Peng et al.80 pointed out that the inhibitory strength of benzenes is related to their substituent groups, and the relationships between them are as follows: –COOH < –SO3–H < –H < –OH < –CH3 < –CHO < –OCH3 < –Cl. When benzene, toluene, phenol and benzoic acid are taken as carbon sources, their IC50s are about 9.21 (7.80 to 10.62), 35.28 (30.16 to 40.40), 124.55 (113.51 to 135.59) and 3833.12 (3481.57 to 4184.67) mg L−1, respectively.
(2) Antibiotics. Antibiotics are biopharmaceuticals and are found in wastewater, such as aquaculture wastewater, livestock wastewater and surface water. Because of their antibiotic actions, microorganisms are inhibited or killed, and antibiotics have obvious inhibitory effects on anammox and denitrifying bacteria in sewage treatment (Table 5). Ding et al.82 studied the effects of different antibiotics on anammox and found that the order of toxicity of various antibiotics was as follows: polymyxin B > chloramphenicol > penicillin G-Na > kanamycin sulfate. The IC50 inhibition concentrations of these antibiotics were 5114.4 (4946.4 to 5282.4), 10.2 (1.8 to 18.6), 409.9 (333.7 to 486.1) and 5254.1 (3934.4 to 6573.8) mg L−1, respectively; moreover, the simultaneous toxicity of multi-component antibiotics shows synergism. According to the research by Zhang et al.83 on the effects of erythromycin on the coupling process, transient inhibition occurred at low concentration (expressed as low-dose addition inhibition), but the activity could be restored in a short time; however, the inhibitory effect was irreversible when a higher concentration was added.
Table 5 Inhibition of anammox and denitrification by antibiotics
Type |
Antibiotic |
T/°C |
pH |
c (mg L−1) |
Effect |
Reference |
β-Lactam |
Penicillin G-Na |
20 ± 1 |
7.0 ± 0.05 |
4946.4–5282.4 |
−50% |
82 |
G-Antibiotic |
Polymyxin B sulfate |
1.8–18.6 |
−50% |
Amide alcohol |
Chloramphenicol |
333.7–486.1 |
−50% |
Aminoglycosides |
Kanamycin sulfate |
3934.4–6573.8 |
−50% |
Macrolides |
Erythromycin |
25 ± 1 |
7.8 ± 0.1 |
20 |
−50.6% |
83 |
Quinolones |
Norfloxacin |
24 ± 1.9 |
8.2 ± 0.07 |
0.001 |
−39.6% |
84 |
Macrolides |
Erythromycin |
24 ± 1.3 |
8.1 ± 0.04 |
0.001 |
A few |
|
Tetracycline |
Oxytetracycline |
35 ± 1 |
8.4 ± 0.11 |
2 |
−81.3% |
85 |
3.3 Salt inhibition
Salt will affect the infiltration of the inside and outside environments of microbial cells. This process creates an osmotic pressure difference that will cause cell death or cleft of microorganisms. This phenomenon exists in some wastewater, such as seafood processing wastewater, mustard production wastewater, dyeing wastewater, and petroleum production wastewater.86 Salt-tolerant microorganisms can be obtained by high salinity acclimation or directly from the ocean.
For example, Giustinianovich et al.43 found that the activity of anammox was inversely proportional to the salt concentration in the treatment of canned fish wastewater. When the concentration of NaCl increased from 1.75 to 18 g L−1, the activity of anammox decreased by 94%, while the coupling nitrogen removal rate of anaerobic ammonia nitrogen was reduced to 30%. However, direct exposure of anammox to 18 g L−1 NaCl for acclimation resulted in higher activity of anammox bacteria and a higher denitrification rate. In another study, the microbial community was subjected to short-term high load salinity (3.4 to 12.2 g L−1),87 and it was found that anammox and denitrifying bacteria were inhibited when the salt was added; however, they could restore their activity within a short time and maintained stable operation in wastewater. Additionally, the influences of the concentration values of different salts are dissimilar; Dapena et al.88 stated that the IC50s of Na2SO4, NaCl, and KCl were 11.36, 13.46, and 14.9 g L−1, respectively (Table 6).
Table 6 Research on the effects of salinity on anammox and denitrifying bacteria
Reactor |
HRT/d |
Salt type |
c (g L−1) |
Effect |
Time/d |
References |
SBR |
1.4–2.6 |
NaCl |
1.75–18.33 |
Anammox activity −94%, nitrogen removal rate −70% |
215 |
43 |
SBR |
0.9–1.3 |
NaCl |
3.4–12.2 |
Nitrogen removal rate −60% |
450 |
89 |
SBR |
— |
Seawater |
3.5–35 |
Short-term 30 g L−1 activity −30%, long-term 35 g L−1 activity −42% |
3 year |
90 |
SBBGRs |
2 |
NaCl |
0–5% |
Activity −40% |
241 |
91 |
PN-An |
0.1 |
NaCl/KCl/Na2SO4 = 1 : 1 : 1 |
0.11–2.4% |
25 g L−1 activity −60%, >24.1 g L−1 nitrification rate −50% |
150 |
92 |
Batch |
— |
NaCl |
25–125 |
100 g L−1 AOB, no effect, 125 g L−1 start suppression |
— |
93 |
EGSB |
— |
NaCl |
5–12 |
Increased heat resistance |
2 year |
94 |
— |
0.25–0.5 |
NaCl |
0/3/25/45 |
Inhibition 0/23.43/48.89/67.94 |
120 |
95 |
3.4 Other inhibition
3.4.1 Metal inhibition. Metals can inhibit microbial metabolism in wastewater treatment processes, especially heavy metals. They affect the microbial activity, cell structure, biological species and population structure by changing cell membrane permeability, synthesizing specific expressed proteins and small organic acids, and forming poorly soluble inorganic substances. Research on heavy metals has achieved abundant outcomes (Table 7); most metals have inhibitory effects on microorganisms. Val del Río et al.87 studied the IC50 values of Cu2+, Cr2+, Pb2+, Zn2+, Ni2+, Cd2+ and Mn2+ on anammox bacteria, and the respective results were as follows: 19.3, 26.9, 45.6, 59.1, 69.2, 174.6, and 175.8 mg L−1. Kalkan et al.96 also focused on the short-term impact of anammox IC50. However, Chen et al.97 added some metal ions to the reactor, namely Fe3+ (6.61 mg L−1), Cu2+ (1.18 mg L−1), and Ni2+ (1.11 mg L−1); it was found that the anammox nitrogen removal rate increased by 50%, and this phenomenon showed that metal ions may have positive influences on anammox bacteria.98 Studies have found that low concentrations of Cu2+ (0.05 to 0.56 mg L−1) will inhibit the activity of denitrifying bacteria, while Zn2+ will promote the growth and reproduction of denitrifying bacteria at lower concentrations.66 Moreover, higher concentrations (>30 mg L−1) will produce inhibition,99 and when two kinds of metal ions impact denitrifying bacteria, the superimposed effect on denitrifying bacteria is slightly weaker.
Table 7 Inhibitory concentrations of metal ions on anammox bacteria
Experimental conditions |
IC50 (mg L−1) |
Reference |
Sludge |
pH |
T/°C |
Domestication time (h) |
Main strain |
Experiment type |
Cu2+ |
Cr2+ |
Pb2+ |
Zn2+ |
Ni2+ |
Cd2+ |
Mn2+ |
PD/An granule 3.2 mm |
7.8 |
30 |
12 |
Brocadia Fulgida |
Gas |
19.3 |
26.9 |
45.6 |
59.1 |
69.2 |
174.6 |
175.8 |
87 |
Mixed sludge |
7.9 ± 0.1 |
35 ± 0.5 |
24 |
Candidatus Brocadia |
Liquid |
— |
— |
— |
15.1 |
6.0 |
— |
— |
96 |
An granule 2.6 ± 0.6 mm |
7.8 ± 0.12 |
35 ± 1 |
0 |
— |
Liquid |
— |
— |
— |
— |
— |
— |
7.33 |
100 |
Anammox granule |
7.5 ± 1 |
30 |
2 |
Brocadia |
Liquid |
4.2 |
— |
— |
7.6 |
48.6 |
11.2 |
— |
101 |
Anammox sludge |
7.0 ± 0.2 |
37 ± 1 |
— |
Planctomyces |
Liquid |
— |
9.84 |
2.3 |
As3+: 10.4 |
7.0 |
— |
— |
102 |
Anammox sludge |
7.5 ± 0.1 |
35 ± 0.5 |
1.8 |
Candidatus Kuenenia |
Liquid |
— |
— |
— |
— |
14.6 |
— |
— |
103 |
Anammox granule |
8.1 ± 0.1 |
35 ± 1 |
0.8 |
— |
Liquid |
12.9 |
— |
— |
— |
— |
— |
— |
104 |
KSU-1 bacteria |
7.0 ± 0.2 |
35 ± 1 |
24 |
KSU-1 |
Liquid |
Hg2+: 60.4 ± 2.5 |
Ag+: 11.5 ± 0.5 |
— |
11.7 ± 0.4 |
— |
— |
— |
105 |
3.4.2 Sulfide and phosphate inhibition. Sulfides and phosphides are common stepless inhibitors of the anammox and denitrification process. In the coupling denitrification process, SO42− and NO2− are simultaneously reduced by anammox bacteria, which function as electron acceptors to produce H2S and N2. The produced sulfide (H2S) will inhibit the anammox process and may be the dominant species (sludge inoculation). There are differences between the operating conditions and the inhibition effects in each study.88,106,107 Zhang et al.108 studied the effects of phosphate on different morphologies of sludge (anammox particles and flocs) through batch experiments and found that phosphate can reduce the abundance of bacteria in the sludge but has less effect on the dominant strain (Candidatus Kuenenia stuttgartiensis). Moreover, according to the study, the IC50 values of the phosphate medium for anammox particles and flocs were 1664.7 ± 114.7 and 1205.9 ± 7.5 mg L−1, respectively. In studies of the effects of sulfides and phosphates on anammox and denitrification, the bacterial strains used are relatively few, and other bacteria should be studied in the future.
4 Operational regulation of the coupling process
In order to operate the coupling process efficiently and economically, the coupling parameters should be adjusted to increase the denitrification rate and reduce the inhibition effect. The adjusting methods include: (1) Mechanical treatment (sedimentation or filtration). (2) Operating parameter control (pH, DO or T). (3) Sludge control (sludge selection, domestication or residence time). (4) Modification of the reactor operation strategy.
4.1 Parameter control
4.1.1 pH and T. pH is the main factor affecting the concentration of FA and FNA; it has independent effects on FA and FNA inhibition. Low pH will increase FNA concentration (eqn (5)), and high pH will increase FA concentration (eqn (4)). By adjusting to the appropriate pH, FA and FNA inhibition can be reduced. According to eqn (1) and (3), the anammox and partial denitrification coupling nitrogen removal process will consume H+ (alkali production process) and the pH will increase.109 In order to ensure better metabolic and propagation conditions and a higher nitrogen removal rate, the pH of the inlet water should be adjusted to neutral or weakly acidic. If the amount of alkali production is uncertain, slightly alkaline inlet water is more suitable.110,111 It is suitable for slightly alkaline inlet water.According to the study by Strous et al.,9 the pH should be controlled within the range of 7.0 to 8.0; the optimal physiological pH range of anammox in a single system is 6.7 to 8.3, while the optimal growth pH of denitrifying bacteria is 6 to 9 and the optimal coupling pH is 8.0. Similar studies111 have found that a suitable range for anammox bacteria growth is pH 7.0 to 8.5; denitrifying bacteria have little effect at pH 7.0 to 8.0, while the optimal pH load for anammox and partial denitrification coupling is 7.0 to 8.0. If the pH is lower (<5.06) or higher (>9.00), it will affect the concentration of substrates and inhibitors in the water environment, influencing the growth and reproduction of anammox and denitrifying bacteria. Thereby, the electrolyte balance in the cell is destroyed to achieve the purpose of inhibiting the bacteria.112 Puyol et al.52 confirmed that high pH is more serious than FA inhibition, and maintaining an appropriate pH value is crucial for the stability of the bacterial community structure. Strous et al.9 pointed out that adding HCO3− to water is an effective method to regulate pH, and proper pH conditions will enrich the bacterial community and lead to a higher nitrogen removal rate.
The survival temperature of anammox bacteria is between 20 °C and 40 °C, and the optimal temperature range for nitrogen removal is 30 °C to 40 °C.111,113 Higher temperature (>45 °C) will irreversibly inhibit the activity of anammox bacteria, and the life activity of bacteria at lower temperature (<10 °C) is completely stopped.111 The optimal growth temperature range of denitrifying bacteria is 20 °C to 35 °C; excessively high or low temperature will cause changes in the physical structures of cell membranes (lipids and proteins).113 Much research has shown that maintaining the temperature at 30 °C to 35 °C is optimum for anammox and partial denitrification coupling.45,55,114
Although the bacteria can be inactivated at high temperature, the demand and application of high temperature denitrification cannot be hindered. Zhang et al.94 increased the salinity appropriately to improve the heat resistance of anaerobic microorganisms (anammox). The optimum temperature is extended to 40 °C to 50 °C. In recent years, low and medium temperature coupling technology for denitrification has become a research focus point. Cheng et al.115 studied urban river sludge and found that the anammox rate is higher in winter than in summer, while the denitrification rate is higher in summer than in winter; this phenomenon provides an opportunity for the application of coupled nitrogen removal technology. The studies by Gilbert et al.116 showed that the temperature effect is closely related to the shape of the biomass. It was also found that the activities of the granular and carrier biofilm species (anammox) can be maintained at a temperature below 13 °C; however, the activity of suspended species begins to be suppressed at temperatures below 15 °C. The comparison shows that the thicker the biofilm in the low temperature environment, the higher the environmental adaptability and the stronger the resistance to temperature impact. According to the studies by Lotti et al.,117 the anammox and partial denitrification coupling process can work well at low and medium temperatures (15 °C to 25 °C). Anammox bacteria that are biologically active in high or low temperature environments (85 °Cand −2 °C) are also found in nature,118,119 which provides the possibility of denitrification in wastewater at different temperatures. However, the anammox and denitrifying bacteria that survive at higher or lower temperatures cannot meet the optimal temperature of enzyme activity. Further exploration of the optimal temperature requirement of enzyme activity coupling with anammox and denitrification is needed. In addition, the temperature affects the FA and FNA concentrations. As shown in eqn (4) and (5), temperature is an important parameter for stable operation control of the reactor.
4.1.2 DO and ORP. Dissolved oxygen (DO) is one of the key parameters for coupling control of anammox and partial denitrification. The living environment of DO for anammox bacteria is usually controlled at 0.6 to 0.8 mg L−1. Generally, lower DO (<2% air saturation) will inhibit the life activity of anammox bacteria (reversible), while higher DO (>18 air saturation) will irreversibly inhibit the bacteria.120 Anammox bacteria will die when exposed to an excessive DO environment, and the living environment of DO for denitrifying bacteria is close to zero; therefore, the coupling of anammox and some denitrification bacteria needs to occur in a low oxygen environment.58 Due to the mutual difference between oxidase and nitrite reductase, higher DO will inhibit nitrate reduction.106 When anammox and partial denitrification are coupled, the DO should be controlled below 0.7 mg L−1 to facilitate the coupling.Oxidation–reduction potential (ORP) is used to characterize the macroscopic redox properties exhibited by substances in aqueous solution, and the ORP values are related to DO, pH, and organic matter concentration.121,122 It has been noted that when anammox is coupled with partial denitrification, an ORP between −50 and +50 mV is beneficial for denitrification.106 ORP is widely used for online monitoring and parameter control of anammox, nitrification, and denitrification processes. A crowd of studies have shown that compared with other parameters (DO or pH), ORP is a more consistent process control parameter.123 The ORP provides reliable control operating parameters for the stable operation of the anammox and partial denitrification coupling process.124
4.1.3 C/N and NO2−/NH4+. C/N is the key to start an anammox and partial denitrification coupling reactor, and it is also an important control parameter to maintain stable reactor operation and efficient nitrogen removal.19,30,125 The presence of organic matter is generally believed to have adverse effects on the anammox process because the heterotrophic denitrifying bacteria grow at a much higher rate and with a higher cellular synthesis growth yield compared to autotrophic anammox bacteria.106Anammox bacteria are autotrophic bacteria, and the participation of organic carbon will inhibit bacterial growth. Sheng et al.77 studied the effects of different organics and C/N on the SAD process; although the biological community believed that in a low COD environment, the anammox bacteria were dominant (C/N = 0.8), they found that the denitrifying bacteria grew faster under a high carbon–nitrogen ratio (C/N = 1.2). Wang et al.129 performed batch tests and indicated that anammox collaborates well with denitrification at low C/N (C/N = 1.0). Miao et al.126 found that when the C/N of the reactor inlet water was adjusted from 1.1 to 2.5, the increase of C/N promoted the growth of denitrifying bacteria and inhibited the growth rate of anammox bacteria (the abundance and activity of anammox bacteria remained stable). Influent C/N directly affects the competition between autotrophic and heterotrophic microorganisms, and most denitrifying bacteria are heterotrophic microorganisms; therefore, a higher influent C/N is beneficial to the growth of denitrifying bacteria. Miao et al.126 reported that C/N low-load wastewater was adjusted as the C/N increased from 1.0 to 2.5; the contribution of denitrification to TN removal gradually increased, and denitrifying bacteria also accumulated. Cao et al.12 reported that the contribution of denitrification to TN removal is directly proportional to C/N, confirming the effects of C/N on denitrifying bacteria.
Studies have shown126 that the C/N the reactor can accept is related to the thickness of the biofilm. Thicker biofilms can maintain a good nitrogen removal rate even with high C/N (C/N = 10). When the biofilm is thin and sensitive, low C/N (C/N = 2) can also inactivate microorganisms. C/N has a significant effect on the accumulation of NO2−–N. Therefore, in the treatment of sewage with less organic matter, an additional carbon source must be used to determine the NO3−–N content in the sewage. In the study of separated and combined partial denitrification and anammox coupling processes, a large number of literature reports have noted that the optimal range of C/N adjustment for the startup and operation of the reactor is 2.0 to 3.0.7,12,13,21,25
NO2−/NH4+ is a direct parameter to measure the optimal removal rate of TN. Studies have shown that the optimal stoichiometric ratio (1.32
:
1, often used as the nitrogen source addition ratio to start the coupling reactor) will reduce the TN removal rate.9,127 When NO2−/NH4+ is more than 1.3 during the nitrogen removal process, the NO2− in the reactor will gradually accumulate and affect the bacterial activity; when NO2−/NH4+ is less than 1.3, despite the high denitrification rate, the anammox process is inhibited and the TN removal rate is reduced by 20% to 60%.128 Wang et al.129 stated that when NO2−/NH4+ is between 0.1 and 0.5, anammox and denitrifying bacteria can coexist; however, the denitrification contribution rate is significantly higher than that of anammox. The calculation of the nitrogen path shows that during the coupling process,13 anammox is the main denitrification method (accounting for 78.9% of the total denitrification amount), which is the real reason for the stable operation of the coupling process. Maintaining a suitable NO2−/NH4+ value with influent water (stoichiometry ratio 1.32
:
1) is an important parameter for the rapid start-up and stable operation of the coupling reactor.
4.1.4 Other parameters. The light effect is the inhibition by light of the activity of photosensitive microorganisms.9 Because anammox bacteria are light-sensitive microorganisms, light energy can inhibit the activity of anammox bacteria, reducing the nitrogen removal rate by 30% to 50%. Therefore, protective measures should be taken in the laboratory environment to increase the nitrogen removal rate.26Hydraulic residence time (HRT) is the average residence time of sewage to be treated in a reactor. In the process of anaerobic ammonia oxidation and partial denitrification coupling, proper increase of HRT can improve the TN removal rate.13 When the nitrogen removal process (or bacteria) is inhibited, adjusting to the appropriate HRT can effectively restore nitrogen removal performance (or bacterial activity).12,130,131
4.2 Sludge
4.2.1 Sludge domestication. Anammox and partial denitrification coupling methods can be divided into split and integrated processes. In the split process, the sludge of absolute dominant bacteria (anammox and denitrification bacteria) is inoculated into two different reactors to remove nitrogen, which effectively avoids competition between the substrates and living space of microorganisms.132 The coupling process mainly starts by inoculating part of the denitrifying floc sludge and anammox granular sludge.22,132,133 It has been reported that denitrifying sludge is obtained by acclimation of nitrifying sludge.122 The advantage of this process is that the anammox activity and denitrification activity are positively related, and the synergistic metabolism can greatly improve the TN removal rate.134–136 Because the screening of the type of sludge is largely determined by the coupling process, the screening of functional anammox and denitrifying bacteria is often performed by adding screening factors (such as pH, T and salt) to the sewage during process operation and commissioning.31 Because of the large difference in demand for sludge-dominant bacteria for sewage treatment performance goals, sludge must be domesticated and cultivated.The most common sludge forms are biofilm, floc sludge (suspended state), and granular sludge. Raudkivi et al.137 stated that the tolerance performance of the three states during domestication is as follows: granular sludge > biofilm > flocculent sludge. Granular sludge and biofilm have high mass transfer resistance, which alleviates the inhibitory effects caused by transient impact and provides sufficient adaptation time for microorganisms. Therefore, in order to shorten the domestication time and achieve more bacteria load, it is important to choose appropriate sludge domestication.
4.2.2 Sludge retention. In order to maintain a stable anammox and partial denitrification coupling denitrification process, the sewage treatment system needs a sufficient sludge residence time (SRT). Jenni et al.138 pointed out that when a sufficient SRT is ensured, anammox and denitrifying bacteria can coexist even at high C/N. Anammox and denitrifying bacteria can only be guaranteed when the SRT is longer than the doubling time of sludge. In order to obtain better denitrification performance, the typical doubling time of anammox is 15 to 30 days.139 It is necessary to ensure an appropriate SRT in the reactor, which is vital to achieve a high nitrogen removal rate in the coupling process.13,139
4.3 Reactor quick start strategy
The optimized reactor operating parameters will shorten the reactor startup time and also increase the speed of the actual industrial application process of anammox and partial denitrification coupling. Research on anammox and partial denitrification coupling technology is listed in Table 8. Methods of quick start strategy parameter control include sludge selection, adjustment of initial conditions and reactor commissioning.
Table 8 Recent application research of anammox and partial denitrification coupling
Reactor |
Operating parameters |
Experimental results |
Conclusion and evaluation |
Time |
Reference |
UASB (2L) |
1. Influent NH4+–N and NO3−–N about 30 mg L−1 |
1. Effluent TN: 6.56 mg L−1, nitrogen removal rate 89.1%, NO3–N to NO2−–N conversion rate 90% |
1. Adjust liquid circulation and increase nitrogen removal performance |
225 d |
131 |
2. Carbon source: acetic acid, C/N: 2.0–3.4 |
|
2. The protein structure and composition of EPS play important roles in sludge stability |
3. Sludge source: DEAMOX-SBR reactor |
|
3. The mass transfer limitations affect anammox and PD activity |
4. Temperature: not controlled, seasonally changing |
|
|
UASB (8L) |
1. C/N: 0.2–0.6, NO2−/NH4+ ratio about 1.32 |
1. TN <5 mg N L−1 |
1. In the model establishment and experimental comparison, the uniform particle size model cannot reflect the real situation, and it is necessary to establish a model to accurately describe the granular anammox system |
200 d |
127 |
2. DO: 0.2–0.4 mg L−1, temperature: 16–30 °C |
2. TN removal rate 90% |
|
3. TN: 51.3 ± 8.4 mgN L−1, COD: 25.5 ± 6.9 mg COD·L−1 |
3. Nitrogen removal rate 2.28 kg N (m3 d)−1 |
|
SBR (5L), UASB (3.2L) |
1. NO3−: 1000 mgN L−1 : NH4+: 58.3 mg L−1 |
1. NO3–N removal rate 95.8% |
1. The strain produces a high concentration of SMP in the absence of substrate, inhibiting the growth of the strain |
215 d, 500 d |
13 |
2. Carbon source: acetic acid, COD: 82.5 mg L−1 |
2. NH4+–N removal rate 92.8% |
2. Anaerobic amoxidase activity is sensitive to high concentrations of organic matter |
3. Sludge source: PD reactor {NTR about 80%} |
3. High COD (76.5–98.6 mg L−1) anammox contributed 78.9% to TN removal |
|
4. Temperature: seasonal change (14.8–28.2 °C) |
|
|
SPNAD (10L) |
1. Influent NH4+–N, COD and TN: 1000 ± 250 mg L−1, 1100 ± 200 mg L−1 and 1300 ± 75 mg L−1 |
1. NRE: 98.7%, NRR: 0.23 kg (m3 d) |
1. Different kinetics have different effects on the anammox community |
200 d |
108 |
2. Temperature: 28 ± 3 °C, DO < 0.5 mg L−1, pH: 8 ± 0.2 |
2. Anammox contributes 69.3% to nitrogen removal, denitrification contribution rate 15.7% |
2. Microorganisms with different functionalities (richness): Nitrosomonas (3.0%), Cadillata (2.13%) and Spindle ella bacteria (25.3%) coexist |
3. Sludge source: pilot SBR and laboratory anammox |
|
|
UASB (1.96L) |
1. Sludge source: anammox sludge according to UASB reactor (120 d), conventional sludge from WWTPs |
1. TN removal rate 92.47% |
1. Anammox and PD gene expression differs with time |
180 d |
150 |
2. Temperature: 33 °C, influent pH: 7.5–8.0 |
2. The dominant species are Thauera 25.30% and Candidatus Brocadia 6.85% |
2. NarG can produce the nitrite required by anammox. NirS is a key gene for the reduction of nitrite to produce nitric oxide. Its activity plays an important role in denitrification and anammox reaction |
3. NO3−/NH4+: 1.6, C/N: 2.0, carbon source: acetate |
3. Anammox and PD gene richness |
|
SBR (10L), UASB (3.2L) |
1. Influent: NH4+: 57.8 mg N L−1, COD: 175.8 mg L−1 |
1. NO3–N removal rate 97.9% |
1. Candidatus Brocadia is a dominant species in the reactor sludge biome |
224 d |
7 |
2. Sludge source: NTR about 80% |
2. NH4+ and COD removal rate 95.2% and 81.6% |
2. The PD-Anammox process has the advantages of low N2O and low CO2 and is environment-friendly |
3. Influent: NO3− (20–40 mg N L−1). The three stages of C/N are 3.0, 2.8 and 2.6. Temperature: 18.7 °C∼27.8 °C |
3. Effluent TN, COD: 4.0 mg N L−1, 30.1 mg L−1 |
|
4.3.1 Sludge selection. According to Zhou et al.,140 adding quality sludge can shorten both the time of anammox and denitrification and the success time of the reactor. Moreover, when less active matrix anammox was added, the anammox and partial denitrification coupling reactor was successfully started and the start-up time was reduced by 5 days. Wang et al.141 inoculated anaerobic granular sludge (AGS) and activated sludge (AS) in a laboratory-scale reactor, and the reactor to which AS was added was successfully started; the reactor start-up time shortened from 21 days to 5 days (TN removal rate was 70%), while the reactor inoculated with AGS failed to start. Zhang et al.142 added 5 different sludge EPS in the interval test of anammox activity; they noted that denitrifying sludge EPS (DS-EPS) can increase the activity of anammox bacteria and shorten the startup time of the reactor.
4.3.2 Initial condition adjustment. When the reactor is started, the characteristics of the operating environment of the anammox and denitrifying bacteria (such as T, pH or NO2−/NH4+) are used as direct control variables for fast startup. Zhou et al.61 inoculated non-deoxygenated anammox and denitrifying sludge at room temperature from 24 °C to 30 °C and returned the washed-out sludge to the reactor to increase the residence time; this played an important role in the reactor startup and performance recovery. Yin et al.143 found that adding a small amount of reduced graphene oxide (RGO) to a started anammox reactor will increase the anammox bacteria activity, thereby shortening the reactor startup time and improving the nitrogen removal rate. Jara-Muñoz et al.144 also quickly started the coupling reactor under two conditions of hypoxia and higher temperatures (30 °C to 40 °C), with shortened the microbial doubling time. A number of studies have shown that during the coupling reactor start-up process, controlling the NO2−/NH4+ ratio at 1.32
:
1 is conducive to promoting the rapid growth of bacteria and shortening the reactor startup time.9,106,145
4.3.3 Reactor operation. During the operation of the reactor, it is necessary to regularly monitor the operating parameters (DO, NO2−, NO3−, etc.) to ensure better treatment performance (TN, COD removal rate, sludge concentration, etc.) and to debug the reactor operation parameters. These are conducive to the rapid startup and stable operation of the coupling reactor.
4.3.4 Improved reactor performance. Charles Glass et al.146 observed that denitrification was inhibited at around pH 6.5, and the NAR increased significantly when the pH was adjusted from 7.5 to 9.0. It is worth noting that in the long-term and short-term effects of pH on denitrification, a high pH value is beneficial to achieve partial denitrification of nitrite accumulation.147–149 The SPDAF (synergizes the partial denitrification, anammox and in situ fermentation) collaborative process established by Ji et al.30 has the disadvantages of slow degradation of organic matter and low utilization rate. The sludge in the fermentation tank was inoculated into the UASB reactor to promote denitrification. The bacteria consumed a large amount of organic matter to produce more nitrite and increased the rate of nitrogen removal by anammox. Zhang et al.19 added sponge bio-carriers to facilitate the enrichment of microorganisms and the formation of EPS. The richness of the biofilms increased by 6 times and the TN removal rate was maintained at 93%, which solved the problems of immature biofilms and insufficient anammox bacteria. Niu et al.27 investigated the stability of FA and FNA to the process and found that both FA and FNA affect the TN removal rate; therefore, the operating conditions were optimized to establish a clear parameter control range (pH 7.5 to 8.5, FA below 10 mg/100 mg NH4+–N and an FNA below 0.005 mg/100 mg NO2−–N), ensuring high nitrogen removal rate and effluent quality.
5 Conclusion
This paper discussed the coupling principles and inhibition mechanisms of anammox and summarized the coupling parameter control and optimization (or rapid) startup strategy. This paper focused on reviewing many practical issues that arise during the startup and nitrogen removal of anammox and some denitrification coupling reactors, summarizing the inhibition mechanisms and concentration control ranges of inhibition parameters (organics, salts, etc.), the influence and adjustment ranges of basic parameters (pH, T, DO, etc.), sludge differences (dominant bacteria), and reactor startup control strategies. Because anammox and partial denitrification coupling is affected by multiple parameters, it is utilized less in common sewage-treatment processes. In order to improve the denitrification performance and develop the coupling denitrification process, it is recommended that further studies on the coupling denitrification process focus on the following aspects:
(1) Research on the inhibition mechanisms. Studies on the inhibition performance and mechanisms of inhibitors, such as substrates, organic matter and salt.
(2) Optimization of operating parameters. Based on the anammox and partial denitrification coupling reactor, combined with the actual bio-dominant species (or characteristic strains), the optimal operating parameters should be adjusted to guide the rapid startup and stable nitrogen removal of the reactor.
(3) Research on the mass transfer mechanism. Based on the state of the sludge (particles, flocculent, etc.) and the distribution of strains in the sludge, studies can be conducted about the mass transfer mechanism under different conditions, the mass transfer relationship between the state of the sludge and the distribution of the species community.
(4) Recovery kinetics after inhibition. When the coupling is inhibited, some additives are often added to restore the activity of the partially coupling strains; therefore, the work process of the recovery process needs to be further explored.
(5) Mathematical model for the coupling process. A unified mathematical model should be established for the coupling process of anammox and denitrification, which is helpful for grasping the mechanism of sewage treatment, predicting the state of sewage treatment, and optimizing the parameter of sewage treatment.
Conflicts of interest
There are no conflicts of interest to declare.
Acknowledgements
The authors gratefully acknowledge the financial support of the National Key Research and Development Program of China (2016YFE0205600), Chongqing Basic Research And Frontier Exploration Project of China (cstc2018jcyjAX0638), Innovation Group of New Technologies for Industrial Pollution Control of Chongqing Education Commission (CXQT19023), Opening Foundation in the Key Research Platforms (KFJJ2018069), Scientific Research Team Project (ZDPTTD201917) and Scientific Research Foundation (1952005) of Chongqing Technology and Business University, and the Program of Chongqing Municipal Education Commission (CY190801).
References
- B. Kartal, J. G. Kuenen and V. M. C. M. Loosdrecht, Sewage treatment with anammox, Science, 2010, 328, 702 CrossRef CAS PubMed.
- M. M. Kuypers, H. K. Marchant and B. Kartal, The microbial nitrogen-cycling network, Nat. Rev. Microbiol., 2018, 16, 263 CrossRef CAS PubMed.
- R. Du, Y. Peng, S. Cao, B. Li, S. Wang and M. Niu, Mechanisms and microbial structure of partial denitrification with high nitrite accumulation, Appl Microbiol Biot, 2016, 100, 2011–2021 CrossRef CAS PubMed.
- D. Zhang, S. Xu, P. Antwi, L. Xiao, W. Luo, Z. Liu, J. Li, H. Su, C. Lai and F. Ayivi, Accelerated start-up, long-term performance and microbial community shifts within a novel upflow porous-plated anaerobic reactor treating nitrogen-rich wastewater via ANAMMOX process, RSC Adv., 2019, 9, 26263–26275 RSC.
- M. C. Van Teeseling, R. J. Mesman, E. Kuru, A. Espaillat, F. Cava, Y. V. Brun, M. S. Vannieuwenhze, B. Kartal and N. L. Van, Anammox Planctomycetes have a peptidoglycan cell wall, Nat. Commun., 2015, 6, 6878 CrossRef CAS PubMed.
- R.-C. Jin, G.-F. Yang, J.-J. Yu and P. Zheng, The inhibition of the Anammox process: A review, Chem. Eng. J., 2012, 197, 67–79 CrossRef CAS.
- S. Cao, R. Du, Y. Peng, B. Li and S. Wang, Novel two stage partial denitrification (PD)-Anammox process for tertiary nitrogen removal from low carbon/nitrogen (C/N) municipal sewage, Chem. Eng. J., 2019, 362, 107–115 CrossRef CAS.
- B. Ma, W. Qian, C. Yuan, Z. Yuan and Y. Peng, Achieving Mainstream Nitrogen Removal through Coupling Anammox with Denitratation, Environ. Sci. Technol., 2017, 51, 8405–8413 CrossRef CAS PubMed.
- M. Strous, J. Heijnen, J. G. Kuenen and M. Jetten, The sequencing batch reactor as a powerful tool for the study of slowly growing anaerobic ammonium-oxidizing microorganisms, Appl. Microbiol. Biotechnol., 1998, 50, 589–596 CrossRef CAS.
- T. Lotti, R. Kleerebezem, C. Lubello and M. C. van Loosdrecht, Physiological and kinetic characterization of a suspended cell anammox culture, Water Res., 2014, 60, 1–14 CrossRef CAS PubMed.
- S. Dasgupta, S. Wu and R. Goel, Coupling autotrophic denitrification with partial nitritation-anammox (PNA) for efficient total inorganic nitrogen removal, Bioresour. Technol., 2017, 243, 700–707 CrossRef CAS PubMed.
- R. Du, S. Cao, S. Wang, M. Niu and Y. Peng, Performance of partial denitrification (PD)-ANAMMOX process in simultaneously treating nitrate and low C/N domestic wastewater at low temperature, Bioresour. Technol., 2016, 219, 420–429 CrossRef CAS PubMed.
- R. Du, S. Cao, Y. Peng, H. Zhang and S. Wang, Combined partial denitrification (PD)-anammox: a method for high nitrate wastewater treatment, Environ. Int., 2019, 126, 707–716 CrossRef CAS PubMed.
- L. Wu, Z. Li, C. Zhao, D. Liang and Y. Peng, A novel partial-denitrification strategy for post-anammox to effectively remove nitrogen from landfill leachate, Sci. Total Environ., 2018, 633, 745–751 CrossRef CAS PubMed.
- S. Cao, R. Du, M. Niu, B. Li, N. Ren and Y. Peng, Integrated anaerobic ammonium oxidization with partial denitrification process for advanced nitrogen removal from high-strength wastewater, Bioresour. Technol., 2016, 221, 37–46 CrossRef CAS PubMed.
- X. Wang, J. Zhao, D. Yu, G. Chen, S. Du, J. Zhen and M. Yuan, Stable nitrite accumulation and phosphorous removal from nitrate and municipal wastewaters in a combined process of endogenous partial denitrification and denitrifying phosphorus removal (EPDPR), Chem. Eng. J., 2019, 355, 560–571 CrossRef CAS.
- J. Ji, Y. Peng, W. Mai, J. He, B. Wang, X. Li and Q. Zhang, Achieving advanced nitrogen removal from low C/N wastewater by combining endogenous partial denitrification with anammox in mainstream treatment, Bioresour. Technol., 2018, 270, 570–579 CrossRef CAS PubMed.
- R. Du, S. Cao, B. Li, M. Niu, S. Wang and Y. Peng, Performance and microbial community analysis of a novel DEAMOX based on partial-denitrification and anammox treating ammonia and nitrate wastewaters, Water Res., 2017, 108, 46–56 CrossRef CAS PubMed.
- H. Zhang, R. Du, S. Cao, S. Wang and Y. Peng, Mechanisms and characteristics of biofilm formation via novel DEAMOX system based on sequencing biofilm batch reactor, J. Biosci. Bioeng., 2019, 127, 206–212 CrossRef CAS PubMed.
- Z.-Z. Zhang, Y.-F. Cheng, B.-Q. Zhu, Y.-Y. Liu, Q. Zhang and R.-C. Jin, Achieving completely anaerobic ammonium removal over nitrite (CAARON) in one single UASB reactor: synchronous and asynchronous feeding regimes of organic carbon make a difference, Sci. Total Environ., 2019, 653, 342–350 CrossRef CAS PubMed.
- Z. Wang, L. Zhang, F. Zhang, H. Jiang, S. Ren, W. Wang and Y. Peng, Enhanced nitrogen removal from nitrate-rich mature leachate via partial denitrification (PD)-anammox under real-time control, Bioresour. Technol., 2019, 289, 121615 CrossRef PubMed.
- R. Du, S. Cao, B. Li, H. Zhang, X. Li, Q. Zhang and Y. Peng, Step-feeding organic carbon enhances high-strength nitrate and ammonia removal via DEAMOX process, Chem. Eng. J., 2019, 360, 501–510 CrossRef CAS.
- M. Zhang, S. Wang, B. Ji and Y. Liu, Towards mainstream deammonification of municipal wastewater: partial nitrification-anammox versus partial denitrification-anammox, Sci. Total Environ., 2019, 692, 393–401 CrossRef CAS PubMed.
- Y. Qin, Y. Cao, J. Ren, T. Wang and B. Han, Effect of glucose on nitrogen removal and microbial community in anammox-denitrification system, Bioresour. Technol., 2017, 244, 33–39 CrossRef CAS PubMed.
- R. Du, S. Cao, X. Li, J. Wang and Y. Peng, Efficient partial-denitrification/anammox (PD/A) process through gas-mixing strategy: System evaluation and microbial analysis, Bioresour. Technol., 2020, 300, 122675 CrossRef PubMed.
- J. Li, Y. Peng, L. Zhang, J. Liu, X. Wang, R. Gao, L. Pang and Y. Zhou, Quantify the contribution of anammox for enhanced nitrogen removal through metagenomic analysis and mass balance in an anoxic moving bed biofilm reactor, Water Res., 2019, 160, 178–187 CrossRef CAS PubMed.
- Q. Niu, S. He, Y. Zhang, H. Ma, Y. Liu and Y.-Y. Li, Process stability and the recovery control associated with inhibition factors in a UASB-anammox reactor with a long-term operation, Bioresour. Technol., 2016, 203, 132–141 CrossRef CAS PubMed.
- H. Wei, X. Wang, M. Hassan, H. Huang and B. Xie, Strategy of rapid start-up and the mechanism of de-nitrogen in landfill bioreactor, J. Environ. Manage., 2019, 240, 126–135 CrossRef CAS PubMed.
- C.-C. Wang, P.-H. Lee, M. Kumar, Y.-T. Huang, S. Sung and J.-G. Lin, Simultaneous partial nitrification, anaerobic ammonium oxidation and denitrification (SNAD) in a full-scale landfill-leachate treatment plant, J. Hazard. Mater., 2010, 175, 622–628 CrossRef CAS PubMed.
- J. Ji, Y. Peng, B. Wang, X. Li and Q. Zhang, A synergistic partial-denitrification, anammox and in situ fermentation (SPDAF) process for advanced nitrogen removal from domestic and nitrate-containing wastewater, Environ. Sci. Technol., 2020, 54, 3702–3713 CrossRef CAS PubMed.
- M. Strous, J. G. Kuenen and M. S. Jetten, Key physiology of anaerobic ammonium oxidation, Appl. Environ. Microbiol., 1999, 65, 3248–3250 CrossRef CAS PubMed.
- K. Isaka, Y. Kimura, M. Matsuura, T. Osaka and S. Tsuneda, First full-scale nitritation-anammox plant using gel entrapment technology for ammonia plant effluent, Biochem. Eng. J., 2017, 122, 115–122 CrossRef CAS.
- M. Hauck, F. A. Maalcke-Luesken, M. S. M. Jetten and M. A. J. Huijbregts, Removing nitrogen from wastewater with side stream anammox: What are the trade-offs between environmental impacts?, Resour., Conserv. Recycl., 2016, 107, 212–219 CrossRef.
- S. Ding, P. Bao, B. Wang, Q. Zhang and Y. Peng, Long-term stable simultaneous partial nitrification, anammox and denitrification (SNAD) process treating real domestic sewage using suspended activated sludge, Chem. Eng. J., 2018, 339, 180–188 CrossRef CAS.
- Y. Liu, H. H. Ngo, W. Guo, L. Peng, D. Wang and B. Ni, The roles of free ammonia (FA) in biological wastewater treatment processes: A review, Environ. Int., 2019, 123, 10–19 CrossRef CAS PubMed.
- I. Fernández, J. Dosta, C. Fajardo, J. L. Campos, A. Mosquera-Corral and R. Mendez, Short- and long-term effects of ammonium and nitrite on the Anammox process, J. Environ. Manage., 2012, 95, S170–S174 CrossRef PubMed.
- V. M. Vadivelu, J. Keller and Z. Yuan, Effect of free ammonia and free nitrous acid concentration on the anabolic and catabolic processes of an enriched Nitrosomonas culture, Biotechnol. Bioeng., 2006, 95, 830–839 CrossRef CAS PubMed.
- M. Waki, T. Yasuda, Y. Fukumoto, K. Kuroda and K. Suzuki, Effect of electron donors on anammox coupling with nitrate reduction for removing nitrogen from nitrate and ammonium, Bioresour. Technol., 2013, 130, 592–598 CrossRef CAS PubMed.
- Q. Wang, H. Duan, W. Wei, B. J. Ni, A. Laloo and Z. Yuan, Achieving Stable Mainstream Nitrogen Removal via the Nitrite Pathway by Sludge Treatment Using Free Ammonia, Environ. Sci. Technol., 2017, 51, 9800–9807 CrossRef CAS PubMed.
- S. Park, J. Chung, B. E. Rittmann and W. Bae, Nitrite accumulation from simultaneous free-ammonia and free-nitrous-acid inhibition and oxygen limitation in a continuous-flow biofilm reactor, Biotechnol. Bioeng., 2015, 112, 43–52 CrossRef CAS PubMed.
- S. He, Y. Zhang, Q. Niu, H. Ma and Y.-Y. Li, Operation stability and recovery performance in an Anammox EGSB reactor after pH shock, Ecol. Eng., 2016, 90, 50–56 CrossRef.
- W. Yang, S. He, M. Han, B. Wang, Q. Niu, Y. Xu, Y. Chen and H. Wang, Nitrogen removal performance and microbial community structure in the start-up and substrate inhibition stages of an anammox reactor, J. Biosci. Bioeng., 2018, 126, 88–95 CrossRef CAS PubMed.
- E. A. Giustinianovich, J. L. Campos, M. D. Roeckel, A. J. Estrada, A. Mosquera-Corral and A. Val Del Rio, Influence of biomass acclimation on the performance of a partial nitritation-anammox reactor treating industrial saline effluents, Chemosphere, 2018, 194, 131–138 CrossRef CAS PubMed.
- S. Sun, Y. Song, X. J. Yang, H. Hu, S. Wu, W.-k. Qi and Y.-Y. Li, Strategies for improving nitrogen removal under high sludge loading rate in an anammox membrane bioreactor operated at 25 °C, Chem. Eng. Sci., 2018, 183, 106–114 CrossRef CAS.
- M. S. Kowalski, T. Devlin, A. di Biase, S. Basu and J. A. Oleszkiewicz, Accelerated start-up of a partial nitritation-anammox moving bed biofilm reactor, Biochem. Eng. J., 2019, 145, 83–89 CrossRef CAS.
- A. Pichel, R. Moreno, M. Figueroa, J. L. Campos, R. Mendez, A. Mosquera-Corral and A. Val del Rio, How to cope with NOB activity and pig manure inhibition in a partial nitritation-anammox process?, Sep. Purif. Technol., 2019, 212, 396–404 CrossRef CAS.
- Y. Yang, L. Zhang, X. Han, S. Zhang, B. Li and Y. Peng, Determine the operational
boundary of a pilot-scale single-stage partial nitritation/anammox system with granular sludge, Water Sci. Technol., 2016, 73, 2085–2092 CrossRef CAS PubMed.
- X. Wang and D. Gao, In situ restoration of one-stage partial nitritation-anammox process deteriorated by nitrate build-up via elevated substrate levels, Sci. Rep., 2016, 6, 37–50 Search PubMed.
- D. Kang, Q. Lin, D. Xu, Q. Hu, Y. Li, A. Ding, M. Zhang and P. Zheng, Color characterization of anammox granular sludge: Chromogenic substance, microbial succession and state indication, Sci. Total Environ., 2018, 642, 1320–1327 CrossRef CAS PubMed.
- L. Miao, G. Yang, T. Tao and Y. Peng, Recent advances in nitrogen removal from landfill leachate using biological treatments - A review, J. Environ. Manage., 2019, 235, 178–185 CrossRef CAS PubMed.
- Y. Kimura, K. Isaka, F. Kazama and T. Sumino, Effects of nitrite inhibition on anaerobic ammonium oxidation, Appl. Microbiol. Biotechnol., 2010, 86, 359–365 CrossRef CAS PubMed.
- D. Puyol, J. M. Carvajal-Arroyo, R. Sierra-Alvarez and J. A. Field, Nitrite (not free nitrous acid) is the main inhibitor of the anammox process at common pH conditions, Biotechnol. Lett., 2014, 36, 547–551 CrossRef CAS PubMed.
- W. Wei, X. Zhou, D. Wang, J. Sun and Q. Wang, Free ammonia pre-treatment of secondary sludge significantly increases anaerobic methane production, Water Res., 2017, 118, 12–19 CrossRef CAS PubMed.
- Y. Zhang, S. He, Q. Niu, W. Qi and Y.-Y. Li, Characterization of three types of inhibition and their recovery processes in an anammox UASB reactor, Biochem. Eng. J., 2016, 109, 212–221 CrossRef CAS.
- S. He, Y. Chen, M. Qin, Z. Mao, L. Yuan, Q. Niu and X. Tan, Effects of temperature on anammox performance and community structure, Bioresour. Technol., 2018, 260, 186–195 CrossRef CAS PubMed.
- H. Duan, S. Gao, X. Li, N. H. Ab Hamid, G. Jiang, M. Zheng, X. Bai, P. L. Bond, X. Lu and M. M. Chislett, Improving wastewater management using free nitrous acid (FNA) – a review, Water Res., 2019, 11, 53–82 Search PubMed.
- Y. Liu, Q. Niu, S. Wang, J. Ji, Y. Zhang, M. Yang, T. Hojo and Y. Y. Li, Upgrading of the symbiosis of Nitrosomonas and anammox bacteria in a novel single-stage partial nitritation-anammox system: Nitrogen removal potential and Microbial characterization, Bioresour. Technol., 2017, 244, 463–472 CrossRef CAS PubMed.
- Z. Yin, C. E. D. d. Santos, J. G. Vilaplana, D. Sobotka, K. Czerwionka, M. H. R. Z. Damianovic, L. Xie, F. J. F. Morales and J. Makinia, Importance of the combined effects of dissolved oxygen and pH on optimization of nitrogen removal in anammox-enriched granular sludge, Process Biochem., 2016, 51, 1274–1282 CrossRef CAS.
- H. Bae, T. Paul, D. Kim and J.-Y. Jung, Specific ANAMMOX activity (SAA) in a sequencing batch reactor: optimization test with statistical comparison, Environ. Earth Sci., 2016, 75, 1452–1460 CrossRef.
- A. Alinsafi, N. Adouani, F. Béline, T. Lendormi, L. Limousy and O. Sire, Nitrite effect on nitrous oxide emission from denitrifying activated sludge, Process Biochem., 2008, 43, 683–689 CrossRef CAS.
- Y. Zhou, L. Ganda, M. Lim, Z. Yuan, S. Kjelleberg and W. J. Ng, Free nitrous acid (FNA) inhibition on denitrifying poly-phosphate accumulating organisms (DPAOs), Appl. Microbiol. Biotechnol., 2010, 88, 359–369 CrossRef CAS PubMed.
- Q. Niu, S. He, Y. Zhang, H. Ma, Y. Liu and Y. Y. Li, Process stability and the recovery control associated with inhibition factors in a UASB-anammox reactor with a long-term
operation, Bioresour. Technol., 2016, 203, 132–141 CrossRef CAS PubMed.
- H. Ma, Q. Niu, Y. Zhang, S. He and Y. Y. Li, Substrate inhibition and concentration control in an UASB-Anammox process, Bioresour. Technol., 2017, 238, 263–272 CrossRef CAS PubMed.
- Y. Zhang, Q. Niu, H. Ma, S. He, K. Kubota and Y. Y. Li, Long-term operation performance and variation of substrate tolerance ability in an anammox attached film expanded bed (AAFEB) reactor, Bioresour. Technol., 2016, 211, 31–40 CrossRef CAS PubMed.
- I. Çelen-Erdem and E. Sağır-Kurt, A pilot-scale study on the partial nitritation-anammox process for treatment of anaerobic sludge digester effluent, Desalin. Water Treat., 2019, 148, 95–101 CrossRef.
- Q. Guo, C.-C. Yang, J.-L. Xu, H.-Y. Hu, M. Huang, M.-L. Shi and R.-C. Jin, Individual and combined effects of substrate, heavy metal and hydraulic shocks on an anammox system, Sep. Purif. Technol., 2015, 154, 128–136 CrossRef CAS.
- D. Zhang, R. Vahala, Y. Wang and B. F. Smets, Microbes in biological processes for municipal landfill leachate treatment: Community, function and interaction, Int. Biodeterior. Biodegrad., 2016, 113, 88–96 CrossRef CAS.
- T. V. Huynh, P. D. Nguyen, T. N. Phan, D. H. Luong, T. T. V. Truong, K. A. Huynh and K. Furukawa, Application of CANON process for nitrogen removal from anaerobically pretreated husbandry wastewater, Int. Biodeterior. Biodegrad., 2019, 136, 15–23 CrossRef CAS.
- S. Lackner, A. Terada and B. F. Smets, Heterotrophic activity compromises autotrophic nitrogen removal in membrane-aerated biofilms: results of a modeling study, Water Res., 2008, 42, 1102–1112 CrossRef CAS PubMed.
- M. Oshiki, H. Satoh and S. Okabe, Ecology and physiology of anaerobic ammonium oxidizing bacteria, Environ. Microbiol., 2016, 18, 2784–2796 CrossRef CAS PubMed.
- D. Guven, A. Dapena, B. Kartal, M. C. Schmid, B. Maas, K. van de Pas-Schoonen, S. Sozen, R. Mendez, H. J. Op den Camp, M. S. Jetten, M. Strous and I. Schmidt, Propionate oxidation by and methanol inhibition of anaerobic ammonium-oxidizing bacteria, Appl. Environ. Microbiol., 2005, 71, 1066–1071 CrossRef PubMed.
- N. Chamchoi, S. Nitisoravut and J. E. Schmidt, Inactivation of ANAMMOX communities under concurrent operation of anaerobic ammonium oxidation (ANAMMOX) and denitrification, Bioresour. Technol., 2008, 99, 3331–3336 CrossRef CAS PubMed.
- M. Jia, C. M. Castro-Barros, M. K. H. Winkler and E. I. P. Volcke, Effect of organic matter on the performance and N2O emission of a granular sludge anammox reactor, Environ. Sci.: Water Res. Technol., 2018, 4, 1035–1046 RSC.
- Y. Feng, Y. Zhao, Y. Guo and S. Liu, Microbial transcript and metabolome analysis uncover discrepant metabolic pathways in autotrophic and mixotrophic anammox consortia, Water Res., 2018, 128, 402–411 CrossRef CAS PubMed.
- C. Cai, S. Hu, X. Chen, B. J. Ni, J. Pu and Z. Yuan, Effect of methane partial pressure on the performance of a membrane biofilm reactor coupling methane-dependent denitrification and anammox, Sci. Total Environ., 2018, 639, 278–285 CrossRef CAS PubMed.
- S. Q. Ni, J. Y. Ni, D. L. Hu and S. Sung, Effect of organic matter on the performance of granular anammox process, Bioresour. Technol., 2012, 110, 701–705 CrossRef CAS PubMed.
- S. Sheng, B. Liu, X. Hou, Z. Liang, X. Sun, L. Du and D. Wang, Effects of different carbon sources and C/N ratios on the simultaneous anammox and denitrification process, Int. Biodeterior. Biodegrad., 2018, 127, 26–34 CrossRef CAS.
- L. Fu, F. Zhang, Y. N. Bai, Y. Z. Lu, J. Ding, D. Zhou, Y. Liu and R. J. Zeng, Mass transfer affects reactor performance, microbial morphology, and community succession in the methane-dependent denitrification and anaerobic ammonium oxidation co-culture, Sci. Total Environ., 2019, 651, 291–297 CrossRef CAS PubMed.
- E. Torresi, M. Escola Casas, F. Polesel, B. G. Plosz, M. Christensson and K. Bester, Impact of external carbon dose on the removal of micropollutants using methanol and ethanol in post-denitrifying Moving Bed Biofilm Reactors, Water Res., 2017, 108, 95–105 CrossRef CAS PubMed.
- S. Peng, X. Han, F. Song, L. Zhang, C. Wei, P. Lu and D. Zhang, Inhibition of benzene, toluene, phenol and benzoate in single and combined on Anammox activity: implication to the denitrification-Anammox synergy, Biodegradation, 2018, 29, 567–577 CrossRef CAS PubMed.
- L. Lyu, K. Zhang, Z. Li, Y. Ma, T. Chai, Y. Pan, X. Wang, S. Li and T. Zhu, Inhibition of anammox activity by phenol: Suppression effect, community analysis and mechanism simulation, Int. Biodeterior. Biodegrad., 2018, 141, 30–38 CrossRef.
- S. Ding, J. Wu, M. Zhang, H. Lu, Q. Mahmood and P. Zheng, Acute toxicity assessment of ANAMMOX substrates and antibiotics by luminescent bacteria test, Chemosphere, 2015, 140, 174–183 CrossRef CAS PubMed.
- X. Zhang, Z. Chen, Y. Ma, T. Chen, J. Zhang, H. Zhang, S. Zheng and J. Jia, Impacts of erythromycin antibiotic on Anammox process: Performance and microbial community structure, Biochem. Eng. J., 2019, 143, 1–8 CrossRef CAS.
- X. Zhang, Z. Chen, Y. Ma, N. Zhang, Q. Pang, X. Xie, Y. Li and J. Jia, Response of Anammox biofilm to antibiotics in trace concentration: Microbial activity, diversity and antibiotic resistance genes, J. Hazard. Mater., 2019, 367, 182–187 CrossRef CAS PubMed.
- Z.-J. Shi, H.-Y. Hu, Y.-Y. Shen, J.-J. Xu, M.-L. Shi and R.-C. Jin, Long-term effects of oxytetracycline (OTC) on the granule-based anammox: Process performance and occurrence of antibiotic resistance genes, Biochem. Eng. J., 2017, 127, 110–118 CrossRef CAS.
- R.-C. Jin, P. Zheng, Q. Mahmood and B.-L. Hu, Osmotic stress on nitrification in an airlift bioreactor, J. Hazard. Mater., 2007, 146, 148–154 CrossRef CAS PubMed.
- Á. Val del Río, T. da Silva, T. H. Martins, E. Foresti, J. L. Campos, R. Méndez and A. Mosquera-Corral, Partial Nitritation-Anammox Granules: Short-Term Inhibitory Effects of Seven Metals on Anammox Activity, Water, Air, Soil Pollut., 2017, 228, 439–448 CrossRef.
- A. Dapena-Mora, I. Fernández, J. L. Campos, A. Mosquera-Corral, R. Méndez and M. S. M. Jetten, Evaluation of activity and inhibition effects on Anammox process by batch tests based on the nitrogen gas production, Enzyme Microb. Technol., 2007, 40, 859–865 CrossRef CAS.
- A. Val Del Rio, A. Pichel, N. Fernandez-Gonzalez, A. Pedrouso, A. Fra-Vazquez, N. Morales, R. Mendez, J. L. Campos and A. Mosquera-Corral, Performance and microbial features of the partial nitritation-anammox process treating fish canning wastewater with variable salt concentrations, J. Environ. Manage., 2018, 208, 112–121 CrossRef CAS PubMed.
- J. Li, P. Qi, R. Li, J. Wang and D. Wang, Carbon and nitrogen removal through “Candidatus Brocadia sinica”-dominated simultaneous anammox and denitrification (SAD) process treating saline wastewater, Biochem. Eng. J., 2018, 140, 72–76 CrossRef CAS.
- C. H. Ge, Y. Dong, H. Li, Q. Li, S. Q. Ni, B. Gao, S. Xu, Z. Qiao and S. Ding, Nitritation-anammox process - A realizable and satisfactory way to remove nitrogen from high saline wastewater, Bioresour. Technol., 2019, 275, 86–93 CrossRef CAS PubMed.
- X. Li, Y. Yuan, Y. Yuan, Z. Bi, X. Liu, Y. Huang, H. Liu, C. Chen and S. Xu, Effects of salinity on the denitrification efficiency and community structure of a combined partial nitritation- anaerobic ammonium oxidation process, Bioresour. Technol., 2018, 249, 550–556 CrossRef CAS PubMed.
- L. Quartaroli, L. C. Silva, C. M. Silva, H. S. Lima, S. O. de Paula, V. M. de Oliveira, S. d. S. M. de Cassia, M. C. Kasuya, M. P. de Sousa, A. P. Torres, R. S. Souza, J. P. Bassin and C. C. da Silva, Ammonium removal from high-salinity oilfield-produced water: assessing the microbial community dynamics at increasing salt concentrations, Appl. Microbiol. Biotechnol., 2017, 101, 859–870 CrossRef CAS PubMed.
- Z. Z. Zhang, Y. X. Ji, Y. F. Cheng, L. Z. Xu and R. C. Jin, Increased salinity improves the thermotolerance of mesophilic anammox consortia, Sci. Total Environ., 2018, 644, 710–716 CrossRef CAS PubMed.
- M. J. García-Ruiz, A. Castellano-Hinojosa, J. González-López and F. Osorio, Effects of salinity on the nitrogen removal efficiency and bacterial community structure in fixed-bed biofilm CANON bioreactors, Chem. Eng. J., 2018, 347, 156–164 CrossRef.
- C. Kalkan Aktan, A. E. Uzunhasanoglu and K. Yapsakli, Speciation of nickel and zinc, its short-term inhibitory effect on anammox, and the associated microbial community composition, Bioresour. Technol., 2018, 268, 558–567 CrossRef CAS PubMed.
- H. Chen, J.-J. Yu, X.-Y. Jia and R.-C. Jin, Enhancement of anammox performance by Cu(II), Ni(II) and Fe(III) supplementation, Chemosphere, 2014, 117, 610–616 CrossRef CAS PubMed.
- X. Cao, H. Cao, Y. Sheng, Y. Xie, K. Zhang, Y. Zhang and J. C. Crittenden, Mechanisms of Cu 2+ migration, recovery and detoxification in Cu 2+-,-containing wastewater treatment process with anaerobic granular sludge, Environ. Technol., 2014, 35, 1956–1961 CrossRef CAS PubMed.
- J. E. Aston, B. M. Peyton, B. D. Lee and W. A. Apel, Effects of ferrous sulfate, inoculum history, and anionic form on lead, zinc, and copper toxicity to Acidithiobacillus caldus strain BC13, Environ. Toxicol. Chem., 2010, 29, 2669–2675 CrossRef CAS PubMed.
- J. J. Xu, Z. Z. Zhang, Q. Q. Chen, Z. Q. Ji, Y. H. Zhu and R. C. Jin, The short- and long-term effects of Mn(2+) on biogranule-based anaerobic ammonium oxidation (anammox), Bioresour. Technol., 2017, 241, 750–759 CrossRef CAS PubMed.
- G. Li, D. Puyol, J. M. Carvajal-Arroyo, R. Sierra-Alvarez and J. Field, Inhibition of anaerobic ammonium oxidation by heavy metals, J. Chem. Technol. Biotechnol., 2015, 90, 830–837 CrossRef CAS.
- C. Yu, Y. X. Song, L. Y. Chai, C. S. Duan, C. J. Tang, M. Ali and C. Peng, Comparative evaluation of short-term stress of Cd(II), Hg(II), Pb(II), As(III) and Cr(VI) on anammox granules by batch test, J. Biosci. Bioeng., 2016, 122, 722–729 CrossRef CAS PubMed.
- D. Wu, Q. Zhang, W.-J. Xia, Z.-J. Shi, B.-C. Huang, N.-S. Fan and R.-C. Jin, Effect of divalent nickel on the anammox process in a UASB reactor, Chemosphere, 2019, 226, 934–944 CrossRef CAS PubMed.
- G.-F. Yang, W.-M. Ni, K. Wu, H. Wang, B.-E. Yang, X.-Y. Jia and R.-C. Jin, The effect of Cu(II) stress on the activity, performance and recovery on the Anaerobic Ammonium-Oxidizing (Anammox) process, Chem. Eng. J., 2013, 226, 39–45 CrossRef CAS.
- Z. Bi, S. Qiao, J. Zhou, X. Tang and Y. Cheng, Inhibition and recovery of Anammox biomass subjected to short-term exposure of Cd, Ag, Hg and Pb, Chem. Eng. J., 2014, 244, 89–96 CrossRef CAS.
- M. Kumar and J. G. Lin, Co-existence of anammox and denitrification for simultaneous nitrogen and carbon removal--Strategies and issues, J. Hazard. Mater., 2010, 178, 1–9 CrossRef CAS PubMed.
- S. Liu, F. Yang, Z. Gong, F. Meng, H. Chen, Y. Xue and K. Furukawa, Application of anaerobic ammonium-oxidizing consortium to achieve completely autotrophic ammonium and sulfate removal, Bioresour. Technol., 2008, 99, 6817–6825 CrossRef CAS PubMed.
- F. Zhang, Y. Peng, S. Wang, Z. Wang and H. Jiang, Efficient step-feed partial nitrification, simultaneous Anammox and denitrification (SPNAD) equipped with real-time control parameters treating raw mature landfill leachate, J. Hazard. Mater., 2019, 364, 163–172 CrossRef CAS PubMed.
- M. Kumar and J.-G. Lin, Co-existence of anammox and denitrification for simultaneous nitrogen and carbon removal—strategies and issues, J. Hazard. Mater., 2010, 178, 1–9 CrossRef CAS PubMed.
- J. Li, W. Zhu, H. Dong and D. Wang, Performance and kinetics of ANAMMOX granular sludge with pH shock in a sequencing batch reactor, Biodegradation, 2017, 28, 245–259 CrossRef CAS PubMed.
- M. Tomaszewski, G. Cema and A. Ziembinska-Buczynska, Influence of temperature and pH on the anammox process: A review and meta-analysis, Chemosphere, 2017, 182, 203–214 CrossRef CAS PubMed.
- Y. Sun, S. Zhou, H. Li and F. Qin, Start-up of ANAMMOX-denitrification reactor and effect of organic loading on its performance of synergistic interaction, J. Chem. Ind. Eng., 2009, 60, 2596–2602 CAS.
- M. Strous, E. Pelletier, S. Mangenot, T. Rattei, A. Lehner, M. W. Taylor, M. Horn, H. Daims, D. Bartol-Mavel and P. Wincker, Deciphering the evolution and metabolism of an anammox bacterium from a community genome, Nat. Commun., 2006, 440, 790 CrossRef PubMed.
- S. Lackner, E. M. Gilbert, S. E. Vlaeminck, A. Joss, H. Horn and M. C. van Loosdrecht, Full-scale partial nitritation/anammox experiences--an application survey, Water Res., 2014, 55, 292–303 CrossRef CAS PubMed.
- L. Cheng, X. Li, X. Lin, L. Hou, M. Liu, Y. Li, S. Liu and X. Hu, Dissimilatory nitrate reduction processes in sediments of urban river networks: Spatiotemporal variations and environmental implications, Biochim. Biophys. Acta, 2016, 219, 545–554 CAS.
- E. M. Gilbert, S. Agrawal, T. Schwartz, H. Horn and S. Lackner, Comparing different reactor configurations for Partial Nitritation/Anammox at low temperatures, Water Res., 2015, 81, 92–100 CrossRef CAS PubMed.
- T. Lotti, R. Kleerebezem and M. Van Loosdrecht, Effect of temperature change on anammox activity, Biotechnol. Bioeng., 2015, 112, 98–103 CrossRef CAS PubMed.
- N. Byrne, M. Strous, V. Crepeau, B. Kartal, J. L. Birrien, M. Schmid, F. Lesongeur, S. Schouten, A. Jaeschke, M. Jetten, D. Prieur and A. Godfroy, Presence and activity of anaerobic ammonium-oxidizing bacteria at deep-sea hydrothermal vents, ISME J., 2009, 3, 117–123 CrossRef CAS PubMed.
- D. W. Gao and Y. Tao, Versatility and application of anaerobic ammonium-oxidizing bacteria, Appl. Microbiol. Biotechnol., 2011, 91, 887–894 CrossRef CAS PubMed.
- Z. Zheng, J. Li, J. Ma, J. Du, W. Bian, Y. Li, Y. Zhang and B. Zhao, Nitrogen removal via simultaneous partial nitrification, anammox and denitrification (SNAD) process under high DO condition, Biodegradation, 2016, 27, 195–208 CrossRef CAS PubMed.
- S. Lackner, C. Lindenblatt and H. Horn, ‘Swinging ORP’as operation strategy for stable reject water treatment by nitritation–anammox in sequencing batch reactors, Chem. Eng. J., 2012, 180, 190–196 CrossRef CAS.
- Z. Wang, L. Zhang, F. Zhang, H. Jiang, S. Ren, W. Wang and Y. Peng, Enhanced nitrogen removal from nitrate-rich mature leachate via partial denitrification (PD)–anammox under real-time control, Bioresour. Technol., 2019, 121, 6–15 Search PubMed.
- H. Kim, A. Ogram and H.-S. Bae, Nitrification, Anammox and Denitrification along a Nutrient Gradient in the Florida Everglades, Wetlands, 2016, 37, 391–399 CrossRef.
- J. Yang, J. Trela, E. Plaza, O. Wahlberg and E. Levlin, Oxidation-reduction potential (ORP) as a control parameter in a single-stage partial nitritation/anammox process treating reject water, J. Chem. Technol. Biotechnol., 2016, 91, 2582–2589 CrossRef CAS.
- R. E. Murray, L. L. Parsons and M. S. Smith, Aerobic and anaerobic growth of rifampin-resistant denitrifying bacteria in soil, Appl. Microbiol. Biotechnol., 1990, 56, 323–328 CAS.
- Y. Miao, Y. Peng, L. Zhang, B. Li, X. Li, L. Wu and S. Wang, Partial nitrification-anammox (PNA) treating sewage with intermittent aeration mode: effect of influent C/N ratios, Chem. Eng. J., 2018, 334, 664–672 CrossRef CAS.
- T. Liu, B. Ma, X. Chen, B.-J. Ni, Y. Peng and J. Guo, Evaluation of mainstream nitrogen removal by simultaneous partial nitrification, anammox and denitrification (SNAD) process in a granule-based reactor, Chem. Eng. J., 2017, 327, 973–981 CrossRef CAS.
- J. R. Vázquez-Padín, M. Figueroa, I. Fernández, A. Mosquera-Corral, J. Campos and R. Mendez, Post-treatment of effluents from anaerobic digesters by the Anammox process, Water Sci. Technol., 2009, 60, 1135–1143 CrossRef PubMed.
- B. Wang, Y. Peng, Y. Guo, Y. Yuan, M. Zhao and S. Wang, Impact of partial nitritation degree and C/N ratio on simultaneous Sludge Fermentation, Denitrification and Anammox process, Bioresour. Technol., 2016, 219, 411–419 CrossRef CAS PubMed.
- M. S. Jetten, M. Strous, K. T. Van de Pas-Schoonen, J. Schalk, U. G. van Dongen, A. A. van de Graaf, S. Logemann, G. Muyzer, M. C. van Loosdrecht and J. G. Kuenen, The anaerobic oxidation of ammonium, FEMS Microbiol. Rev., 1998, 22, 421–437 CrossRef CAS PubMed.
- D. Choi, S. Cho and J. Jung, Key operating parameters affecting nitrogen removal rate in single-stage deammonification, Chemosphere, 2018, 207, 357–364 CrossRef CAS PubMed.
- R. Du, S. Cao, B. Li, H. Zhang, S. Wang and Y. Peng, Synergy of partial-denitrification and anammox in continuously fed upflow sludge blanket reactor for simultaneous nitrate and ammonia removal at room temperature, Bioresour. Technol., 2019, 274, 386–394 CrossRef CAS PubMed.
- R. Du, S. Cao, B. Li, S. Wang and Y. Peng, Simultaneous domestic wastewater and nitrate sewage treatment by DEnitrifying AMmonium OXidation (DEAMOX) in sequencing batch reactor, Chemosphere, 2017, 174, 399–407 CrossRef CAS PubMed.
- P. Jin, B. Li, D. Mu, X. Li and Y. Peng, High-efficient nitrogen removal from municipal wastewater via two-stage nitritation/anammox process: Long-term stability assessment and mechanism analysis, Bioresour. Technol., 2019, 271, 150–158 CrossRef CAS PubMed.
- Z. Zheng, S. Huang, W. Bian, D. Liang, X. Wang, K. Zhang, X. Ma and J. Li, Enhanced nitrogen removal of the simultaneous partial nitrification, anammox and denitrification (SNAD) biofilm reactor for treating mainstream wastewater under low dissolved oxygen (DO) concentration, Bioresour. Technol., 2019, 283, 213–220 CrossRef CAS PubMed.
- D. Wang, T. Li, K. Huang, X. He and X.-X. Zhang, Roles and correlations of functional bacteria and genes in the start-up of simultaneous anammox and denitrification system for enhanced nitrogen removal, Sci. Total Environ., 2019, 655, 1355–1363 CrossRef CAS PubMed.
- M. Raudkivi, I. Zekker, E. Rikmann, P. Vabamäe, K. Kroon and T. Tenno, Nitrite inhibition and limitation–the effect of nitrite spiking on anammox biofilm, suspended and granular biomass, Water Sci. Technol., 2016, 75, 313–321 CrossRef PubMed.
- S. Jenni, S. E. Vlaeminck, E. Morgenroth and K. M. Udert, Successful application of nitritation/anammox to wastewater with elevated organic carbon to ammonia ratios, Water Res., 2014, 49, 316–326 CrossRef CAS PubMed.
- T. Lotti, R. Kleerebezem, J. Abelleira-Pereira, B. Abbas and M. Van Loosdrecht, Faster through training: the anammox case, Water Res., 2015, 81, 261–268 CrossRef CAS PubMed.
- Z. Zhou, X. Lin, F. Wang, C. Gu, J. Shen, Y. Yuan and R. Jin, Removal of Nitrogen from Alcohol Wastewater by PN-ANAMMOX, Environ. Sci., 2017, 38, 3377–3384 Search PubMed.
- Q. Wang, Y. Wang, J. Lin, R. Tang, W. Wang, X. Zhan and Z.-H. Hu, Selection of seeding strategy for fast start-up of Anammox process with low concentration of Anammox sludge inoculum, Bioresour. Technol., 2018, 268, 638–647 CrossRef CAS PubMed.
- J. Zhang, Y. Miao, Q. Zhang, Y. Sun, L. Wu and Y. Peng, Mechanism of stable sewage nitrogen removal in a partial nitrification-anammox biofilm system at low temperatures: microbial community and EPS analysis, Bioresour. Technol., 2019, 12, 24–59 Search PubMed.
- X. Yin, S. Qiao, J. Zhou and X. Tang, Fast start-up of the anammox process with addition of reduced graphene oxides, Chem. Eng. J., 2016, 283, 160–166 CrossRef CAS.
- P. Jara-Muñoz, V. Guzmán-Fierro, C. Arriagada, V. Campos, J. L. Campos, J. J. Gallardo-Rodríguez, K. Fernández and M. Roeckel, Low oxygen start-up of partial nitrification-anammox process: mechanical or gas agitation?, J. Chem. Technol. Biotechnol., 2019, 94, 475–483 CrossRef.
- X. Huang, K. Urata, Q. Wei, Y. Yamashita, T. Hama and Y. Kawagoshi, Fast start-up of partial nitritation as pre-treatment for anammox in membrane bioreactor, Biochem. Eng. J., 2016, 105, 371–378 CrossRef CAS.
- C. Glass and J. Silverstein, Denitrification kinetics of high nitrate concentration water: pH effect on inhibition and nitrite accumulation, Water Res., 1998, 32, 831–839 CrossRef CAS.
- W. Qian, B. Ma, X. Li, Q. Zhang and Y. Peng, Long-term effect of pH on denitrification: High pH benefits achieving partial-denitrification, Bioresour. Technol., 2019, 278, 444–449 CrossRef CAS PubMed.
- B. Cui, X. Liu, Q. Yang, J. Li, X. Zhou and Y. Peng, Achieving partial denitrification through control of biofilm structure during biofilm growth in denitrifying biofilter, Bioresour. Technol., 2017, 238, 223–231 CrossRef CAS PubMed.
- J. Ji, Y. Peng, B. Wang and S. Wang, Achievement of high nitrite accumulation via endogenous partial denitrification (EPD), Bioresour. Technol., 2017, 224, 140–146 CrossRef CAS PubMed.
- D. Wang, Y. He and X. X. Zhang, A comprehensive insight into the functional bacteria and genes and their roles in simultaneous denitrification and anammox system at varying substrate loadings, Appl. Microbiol. Biotechnol., 2019, 103, 1523–1533 CrossRef CAS PubMed.
- R. R. Nair, P. B. Dhamole, S. S. Lele and S. F. D’Souza, Biological denitrification of high strength nitrate waste using preadapted denitrifying sludge, Chemosphere, 2007, 67, 1612–1617 CrossRef CAS PubMed.
|
This journal is © The Royal Society of Chemistry 2020 |
Click here to see how this site uses Cookies. View our privacy policy here.