DOI:
10.1039/C9RA10191H
(Paper)
RSC Adv., 2020,
10, 6953-6959
Synthesis, characterization, and properties of a novel aromatic ester-based polybenzoxazine
Received
5th December 2019
, Accepted 16th January 2020
First published on 20th February 2020
Abstract
Polybenzoxazines with molecular design flexibility have excellent properties by using suitable raw materials. A new benzoxazine monomer terephthalic acid bis-[2-(6-methyl-4H-benzo[e][1,3]oxazin-3-yl)]ethyl ester (TMBE) with bis-ester groups has been synthesized from the simple esterification reaction of terephthaloyl chloride and 2-(6-methyl-4H-benzo[e][1,3]oxazin-3-yl)-ethanol (MB-OH). The chemical structure of TMBE was characterized by Fourier transform infrared spectroscopy (FT-IR) and nuclear magnetic resonance spectroscopy (1H-NMR, 13C-NMR). Polymerization behavior of TMBE was studied by differential scanning calorimetry (DSC) and FT-IR after each cure stage. The cross-linked polybenzoxazine (PTMBE) gave a transparent film through the thermal casting method. The dynamic mechanical analysis of PTMBE showed that the Tg was 110 °C. Thermogravimetric analysis reveals better thermal stability as evidenced by the 5% and 10% weight-loss temperatures (Td5 and Td10) of PTMBE, which were 263 and 289 °C, respectively, with a char yield of 27% at 800 °C. The tensile test of the film revealed that the elongation at break was up to 14.2%.
1. Introduction
As a new phenolic-type resin, polybenzoxazines possess attractive properties, such as high glass transition temperature,1,2 flame retardancy,3 low water absorption,4 and low cost.5 They are applied to the field of halogen-free flame retardant laminates, vacuum pump rotors, printed circuit boards, friction materials, composites, and other fields.6,7 Nevertheless, inherent brittleness of polybenzoxazines, especially in aromatic polybenzoxazines, limits their further development in the chemical industry.
There are generally three reported approaches to improve the toughness of polybenzoxazines. The first approach is introducing rubber,8,9 the flexible heat-resistant linear polymer10–14 into resins as the toughening agent to improve toughness. The second approach is designing inherently tough benzoxazine monomers containing soft segments to afford tough polybenzoxazines.15,16 The third approach is the toughening of polybenzoxazines by inorganic nanoparticles.17–19 Among them, the designing of inherently tough benzoxazine monomers and polybenzoxazines prepolymers was a significant approach to improve the flexibility of polybenzoxazines from the molecular level, and to increase the degree of chemical crosslinking between the two phases.
The tough benzoxazine monomers were synthesized, including monofunctional and difunctional benzoxazines from available phenols containing flexible groups, primary amines and formaldehyde.4,20–23 These monomers improve the flexibility of polybenzoxazines with dramatically scarifying their heat resistance. Therefore, some main-chain type polybenzoxazines24,25 were synthesized by Mannich reaction with polyether diamines of different molecular weights or PDMS with different molecular weights for the diamine terminal, bisphenol A, and formaldehyde. Such high-molecular-weight prepolymers exhibited significantly improved toughness due to the soft segments and generated a high cross-linking degree due to the polybenzoxazine component.
The other approach to improve toughness is indirectly preparing of polybenzoxazine prepolymers using polyesterification,14 coupling reactions, alternating copolymerization of donor–acceptor monomers,26 Diels–Alder reaction,27 polyetherification,28 hydrosilylation29 These synthetic approaches also necessarily yield high-performance polybenzoxazines with improved toughness. The novel polyesters were synthesized from the polycondensation reaction of bisbenzoxazine-diol, pyromellitic dianhydride, and 4,4′-(hexafluoroisopropylidene)diphthalic anhydride.14 The molecular weights of polyesters were in the range of 5800–7000 Da with benzoxazine moieties in the main chain exhibited high flexibility induced by the soft pentyl and esters groups and comparable thermal stability concerning low molar mass analogous. Aydogan29 prepared polysiloxanes containing benzoxazine units in the main chain by hydrosilylation of 1,1,3,3-tetramethyldisiloxane and diallyl functional benzoxazines. To further improve its flexibility, polysiloxanes chain was extended by the reaction of poly (bisbenzoxazinedimethylsiloxane)s and octamethyl-cyclotetrasiloxane in the presence of tetrabutylammonium hydroxide as a catalyst.
Based on this idea, our work presents a facile route for synthesizing high-purity aromatic benzoxazine containing bis-ester groups. First, 2-(6-methyl-4H-benzo[e][1,3]oxazin-3-yl)-ethanol (MB-OH) was synthesized from formaldehyde solution (37%), ethanolamine, and p-cresol. Then, terephthalic acid bis-[2-(6-methyl-4H-benzo[e][1,3]oxazin-3-yl)]ethyl ester (TMBE) with bis-ester groups has been synthesized from the simple esterification reaction of MB-OH and terephthaloyl chloride. The crosslinked polybenzoxazine (PTMBE) gave transparent flexible film through the thermal casting method. The thermal and mechanical properties of aromatic ester-based polybenzoxazine were studied and compared with respect to polybenzoxazines analogous.
2. Material and methods
2.1. Materials
Potassium hydroxide, p-cresol (98%) were purchased from Sinopharm Chemical Reagent Co., Ltd. 2-Aminoethanol, dioxane, triethylamine (Et3N), methylene chloride was obtained from Tianjin Fu Jin Fine Chemical Co., Ltd. Formaldehyde (37 wt% in water), terephthaloyl chloride, n-hexane was used as received. Methylene chloride used after distillation.
2.2. Synthesis of MB-OH
MB-OH was synthesized according to the literature.12 A suspension of paraformaldehyde (15.3 mL, 210 mmol), 30 mL 1,4-dioxane were added to a 250 mL three-neck flask in an ice bath. Then 2-aminoethanol (6.0 mL, 100 mmol) was added drop-wise to the system. The mixture stirred at room temperature for about 30 min. After adding p-cresol (10.6 mL, 100 mmol), the temperature was gradually increased to 70 °C, and stirring was continued for another 3.5 h. A yellowish viscous fluid was obtained after removing the solvent through a rotary evaporator. Then the viscous liquid was dissolved in dichloromethane, washed with 1 L of 0.1 N aqueous KOH and finally three times with 1 L of distilled water. The methylene chloride solution was dried with MgSO4, filtered, and concentrated under vacuum to afford crude product. The crude product was recrystallized with n-hexane to give pure white solid of MB-OH (9.53 g, 51%).
1H NMR (CDCl3, 300 MHz, δ): 3.98 ppm (s, Ar–*CH2–N), 4.85 ppm (s, O–*CH2–N), 2.95 ppm (t, N–*CH2–C), 3.69 ppm (t, C–*CH2–O), 2.24 ppm (s, Ph–*CH3).
FTIR (KBr) ν: 1034 cm−1 (C–O–C symmetric stretching), 1232 cm−1 (C–O–C asymmetric stretching), 1502 cm−1 (trisubstituted benzene ring), 940 cm−1 (oxazine ring).
2.3. Synthesis of TMBE
Into a 100 mL dry round-bottom flask equipped with a calcium chloride drying tube, 20 mL dry methylene chloride, MB-OH (1.9 g, 10 mmol), triethylamine (1.7 mL, 1.4 mmol) were added and mixed at room temperature for about 30 min. Then terephthaloyl chloride (1.0 mL, 5.0 mmol) was added at 5 °C. The mixtures were stirred at room temperature for 2 d and kept refluxing for 4 h. A yellow transparent solution was obtained after cooling to room temperature. The solution was washed with 1 L of 0.5 N aqueous NaOH and finally three times with 1 L of distilled water. The methylene chloride solution was dried with MgSO4, filtered, and concentrated under vacuum to afford crude product (1.6 g, yield: 61%). The product was purified by chromatography (vmethylene chloride
:
vethyl acetate = 2
:
1) to afford white power solid.
1H NMR (CDCl3, 300 MHz, δ): 4.05 ppm (s, Ar–*CH2–N), 4.88 ppm (s, O–*CH2–N), 3.18 ppm (t, N–*CH2–C), 4.52 ppm (t, C–*CH2–O), 2.27 ppm (s, Ph–*CH3).
13C NMR (CDCl3, 300 MHz, δ): 50.70 ppm (Ar–*CH2–N), 82.85 ppm (O–*CH2–N), 50.38 ppm (N–*CH2–C), 63.65 ppm (C–*CH2–O), 18.30 ppm (Ph–*CH3), 165.71 ppm (O–C*
O).
FTIR (KBr) ν: 1019 cm−1 (C–O–C symmetric stretching), 1277 cm−1 (C–O–C asymmetric stretching), 1506 cm−1 (trisubstituted benzene ring), 932 cm−1 (oxazine ring), 1720 cm−1 (C
O stretching).
2.4. Polymerization of TMBE
The amount of TMBE was melted, stirred and transferred to a rectangular aluminum foil mold and then cured at 120 °C/2 h, 140 °C/2 h, 160 °C/2 h, 180 °C/2.5 h in an air-circulating oven. After that, samples were allowed to cool slowly to room temperature to be tested.
2.5. Characterizations
FTIR spectra were obtained with Bruker Tensor 27 FTIR spectrometer (Bruker, GM) in which samples were preparing as KBr pellets. 1H-NMR and 13C-NMR spectra were recorded on a Bruker Avance 300 instrument (Bruker) using CDCl3 as the solvent and TMS as the internal standard. Differential scanning calorimetry (DSC) was conducted using a DSC SP instrument (Rheometric Scientific) at a heating rate of 10 °C min−1 under nitrogen. Thermogravimetric analysis (TGA) was performed on a TGA/DSC STARe System instrument (Mettler-Toledo) at a heating rate of 10 °C min−1 under N2 atmosphere. The gas flow rate was 100 mL min−1. The dynamic mechanical analysis (DMA) of samples was carried out using a Mettler-Toledo DMA/SDTA861e instrument (Mettler-Toledo). The specimen with dimensions of approximately 3.00 × 2.20 × 0.8 mm3 was tested by a shear mode at 1 Hz in the temperature range of 30–200 °C at a heating rate of 3 °C min−1. Tensile measurement were also recorded with Material Testing Machine Model UTM5105 at a crosshead speed of 1 mm min−1. Each sample with a dimension of approximately 20.0 × 5.0 × 1.0 mm3 was tested from an average of at least 5 tests.
3. Results and discussion
3.1. Preparation of TMBE and its polymer
Scheme 1 illustrates our strategy for the preparation of TMBE and PTMBE. First, according to reference, we synthesized containing hydroxyl benzoxazine (MB-OH). Second, using triethylamine as deacid reagent, dry dichloromethane as solvent, TMBE was obtained via the esterification reaction of MB-OH and terephthaloyl chloride at a molar ratio of 2
:
1. TMBE was easily soluble in common low-boiling organic reagents, such as dichloromethane, chloroform, and tetrahydrofuran. Finally, PTMBE was formed by the cross-linking of TMBE under thermal curing reaction with polymerization temperature of 180 °C.
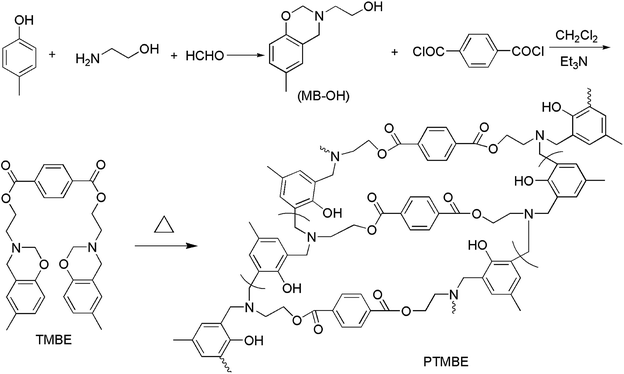 |
| Scheme 1 Synthetic route to TMBE and PTMBE. | |
3.2. Characterization of TMBE
The chemical structure of TMBE was confirmed by NMR and FT-IR spectral analysis. As can be seen from Fig. 1 where the 1H NMR spectrum of TMBE is presented, the appearance of the protons resonating at 4.05 ppm (–O–*CH2–N) and 4.88 ppm (Ar–*CH2–N) was clear evidence for the benzoxazine ring formation on TMBE. Moreover, the peaks at 3.18 ppm and 4.52 ppm assigned to protons of –N–*CH2–C– and C–*CH2–O– were observed, respectively. The integral ration of these peaks was close to the theoretical ratio of 2
:
2
:
2
:
2. The signal peak appeared at 2.27 ppm corresponding to the Ph–*CH3. The peak appeared at 8.10 ppm corresponding to the protons of the benzene ring linked to the ester group. The peaks appeared at 6.68 ppm, 6.75 ppm, and 6.95 ppm assigned to protons of benzene ring linked to the oxazine ring.
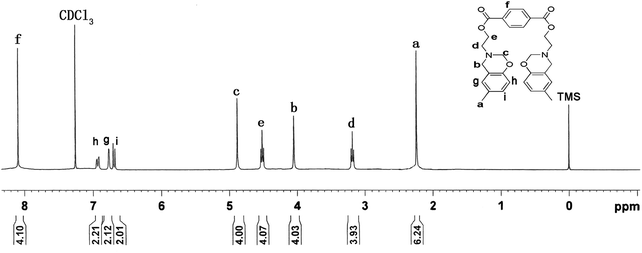 |
| Fig. 1 1H-NMR spectrum of TMBE. | |
The 13C NMR spectrum presented from Fig. 2 further proved the structure of TMBE. The carbon resonances of the oxazine ring at 50.7 ppm for Ar–*CH2–N and at 82.9 ppm for O–*CH2–N were clearly observed, respectively. The carbon resonances at 50.38 and 63.65 ppm of N–*CH2–C and C–*CH2–O were clearly appeared, respectively. The peak at 165.71 and 20.30 ppm were characteristic absorptions of O–C*
O and Ph–*CH3, respectively. The resonance at 128.46 and 133.96 ppm corresponded to carbon atoms in the aromatic ring of terephthalate. Others were characteristic absorptions attributed to the aromatic ring of benzoxazine.
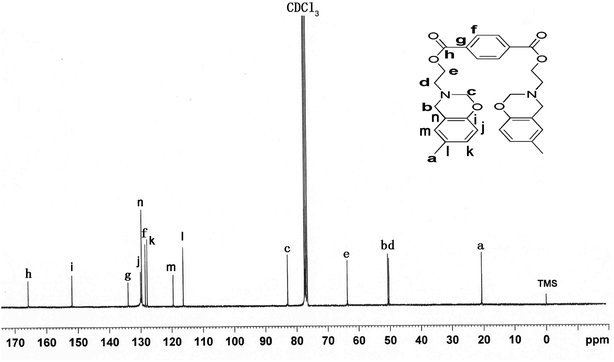 |
| Fig. 2 13C-NMR spectrum of TMBE. | |
FT-IR spectra also give evidence for the formation of benzoxazine containing bis-ester groups (Fig. 3). The characteristic peak at 932 cm−1 due to the C–H out-of-plane vibration in the benzene ring where an oxazine ring attached was clearly observed. Additionally, the strong peak at 1720 cm−1 was characteristic absorption of the carbonyl in terephthalate.
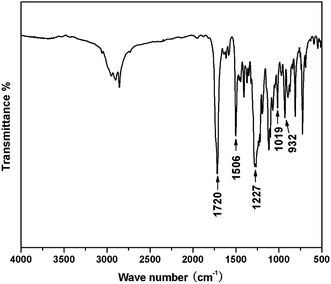 |
| Fig. 3 FTIR spectrum of the TMBE. | |
3.3. Polymerization of TMBE
The DSC heating-scan thermogram of TMBE was depicted in Fig. 4. The sharp endothermic peak appearing at 139 °C corresponds to the melting temperature (Tm) of TMBE. TMBE showed unimodal curing behaviour as observed from the single exothermic peak, which is from the ring-opening polymerization of the benzoxazine ring. The onset and peak top temperatures of the peak are 176 and 231 °C, respectively. The polymerization temperature of TMBE is similar to the values reported to other traditional benzoxazine compounds.30 DSC result of curing TMBE show the presence of aliphatic chains (C4) in the benzoxazine structure has little effect on the polymerization behavior of TMBE and only a lower temperature is required for polymerization.
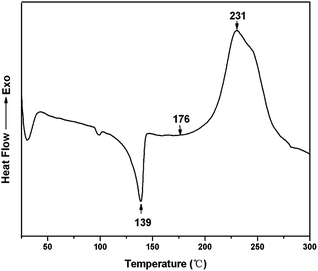 |
| Fig. 4 DSC thermogram of TMBE. | |
The polymerization behavior of TMBE was also monitored by FTIR after curing at each temperature (Fig. 5). With the increase of temperature, the intensity of the characteristic absorption peak at 932 cm−1 due to benzoxazine structure gradually decreased due to the opening of the oxazine ring and disappeared after the 180 °C for 2.5 h. The intensity of absorption bands at 1502 cm−1 due to the trisubstituted benzene ring (stretching) also decreased. Meanwhile, some new absorption bands appeared at 3442 cm−1 due to the phenolic hydroxy and at 1481 cm−1 due to the tetrasubstituted benzene ring, suggesting that the ring-opening polymerization of TMBE occurred. Both DSC and IR spectroscopy indicates the formation of polybenzoxazine by polymerization at 180 °C.
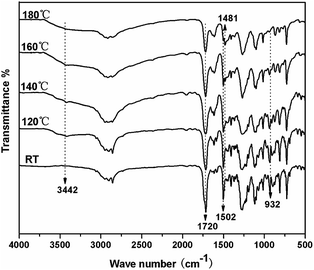 |
| Fig. 5 FTIR spectra of TMBE after curing at each temperature. | |
3.4. Thermal property of PTMBE
Dynamic mechanical analysis (DMA) was performed to study the viscoelastic properties of PTMBE (Fig. 6). At 30 and 80 °C, the storage modulus (G′) values are 168 and 100 MPa, respectively, whereas the loss modulus (G′′) values are 11 and 27 MPa, respectively. For poly(BA-a) (bisphenol-A and aniline-based polybenzoxazine), the storage modulus G′ of 1.9 × 103 MPa is higher than that of PTMBE due to their high aromatic content. It has been clearly demonstrated from DMA that the flexibility of PTMBE is better than poly(BA-a). The Tg was observed at 110 and 89 °C from the maximum loss factor and maximum loss modulus, respectively. The decrease of Tg can be attributed to the flexible alkyl chain in PTMBE because of a lower energy barrier for motion.
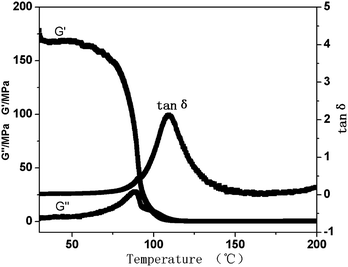 |
| Fig. 6 DMA curves of PTMBE. | |
TGA was performed to determine the thermal stability of PTMBE. Fig. 7 shows the TGA thermograms of PTMBE. In nitrogen, 5 and 10% weight loss temperatures (Td5 and Td10) of PTMBE were 263 and 289 °C, respectively. The char yield of PTMBE was up to 27.0% at 800 °C. The Td5 and Td10 of PTMBE were decreased by about 50 °C compared with poly(BA-a). The char yield of PTMBE at 800 °C has a slight drop compared with poly(BA-a). PTMBE shows the lower thermal stability than poly(BA-a) attributed as mentioned previously to the flexible aliphatic chain.
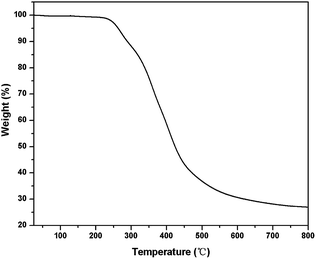 |
| Fig. 7 TGA curve of PTMBE. | |
3.5. Mechanical properties of PTMBE
The stress–strain curve was showed to study the mechanical property for the PTMBE film (Fig. 8). The PTMBE film showed elongation at a break of 14.2% and the tensile strength of 11.3 MPa. It is reported that the PB-a film is very brittle with elongation at break of 1.3%.30 The elongation at break of PTMBE was larger than that of PB-a. Namely, PTMBE had remarkably improved toughness, and the film is easy to bend. Interestingly, in spite of the introduction of the aromatic ester group, PTMBE still showed the high elongation at break comparing with polybenzoxazines20 only containing linear long aliphatic chains (C12). The toughness enhancement of PTMBE is attributed to the long flexible alkyl chains, which enhances the mobility of segments under load and thereby increases the ultimate elongation. The tensile modulus of PTMBE is 248.0 MPa, lower than that of PBA-a (4.3 GPa) with rigid backbone.30 Therefore, the incorporation of the long aliphatic chain and aromatic ester group into PTMBE network structure gave flexible polymer film without excessively scarifying its heat resistance.
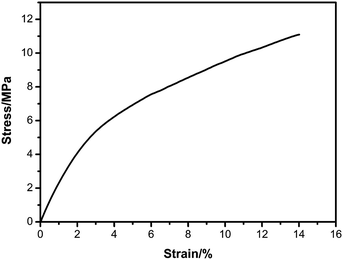 |
| Fig. 8 Stress–strain curve of PTMBE. | |
A yellowish PTMBE film (7 × 6 cm2) with 150–200 μm in thickness was obtained through the thermal casting method in Fig. 9(a). A larger-sized film (20 × 20 cm2) was also prepared in our lab according to the same procedure. Besides, the PTMBE film had remarkably improved toughness, and is easy to bend in Fig. 9(b).
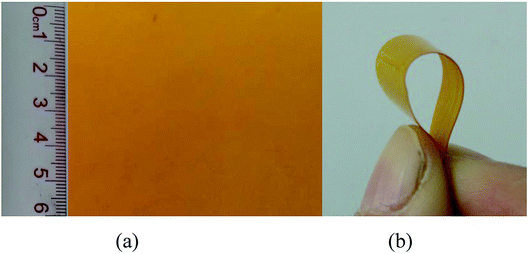 |
| Fig. 9 Appearance of PTMBE film (a) and (b). | |
4. Conclusions
By utilizing a clean and facile route, we have prepared a high-purity aromatic ester-based benzoxazine monomer and its polymer. Thermally activated polymerization of benzoxazine monomer provided flexible, uniform polymer film. The novel polybenzoxazine film exhibited significantly improved toughness due to the long aliphatic chain. The storage modulus and glass transition temperature of PTMBE were 168 MPa and 110 °C, respectively. Regarding thermal stability, PTMBE showed lower thermal stability than poly(BA-a) due to the aliphatic hydrocarbon chain. It is anticipated to find applications of PTMBE as a self-healing material.
Conflicts of interest
There are no conflicts to declare.
Acknowledgements
The authors are thankful for the financial supports from Shandong Provincial Natural Science Foundation, China (ZR2018MB022).
References
- X. Ning and H. Ishida, Phenolic materials via ring-opening polymerization of benzoxazines: effect of molecular structure on mechanical and dynamic mechanical properties, J. Polym. Sci., Part B: Polym. Phys., 1994, 32, 921–927 CrossRef CAS.
- M. Goto, Y. Miyagi, M. Minami and F. Sanda, Synthesis and crosslinking reaction of polyacetylenes substituted with benzoxazine rings: thermally highly stable benzoxazine resins, J. Polym. Sci., Part A: Polym. Chem., 2018, 56, 1884–1893 CrossRef CAS.
- S. Gao, Y. Liu, S. Y. Feng and Z. J. Lu, Synthesis of borosiloxane/polybenzoxazine hybrids as highly efficient and environmentally friendly flame retardant materials, J. Polym. Sci., Part A: Polym. Chem., 2017, 55, 2390–2396 CrossRef CAS.
- H. Xu, W. Zhang, Z. Lu and G. Zhang, Synthesis and properties of thermosetting resin based on urushiol, RSC Adv., 2013, 3, 3677–3682 RSC.
- N. N. Ghosh, B. Kiskan and Y. Yagci, Polybenzoxazines-new high performance thermosetting resins: synthesis and properties, Prog. Polym. Sci., 2007, 32, 1344–1391 CrossRef CAS.
- Y. Gu and Q. C. Ran, in Handbook of Benzoxazine Resins, ed. H. Ishida and T. Agag, Elsevier, Amsterdam, ch. 28, 2011, pp. 481–494 Search PubMed.
- M. Xu, M. Liu, S. Dong and X. Liu, Design of low temperature self-cured phthalonitrile-based polymers for advanced glass fiber composite laminates, J. Mater. Sci., 2013, 48, 8108–8116 CrossRef CAS.
- T. Takeichi, Y. Guo and T. Agag, Synthesis and characterization of poly(urethane-benzoxazine) films as novel type of polyurethane/phenolic resin composites, J. Polym. Sci., Part A: Polym. Chem., 2000, 38, 4165–4176 CrossRef CAS.
- T. Takeichi, T. Kano and T. Agag, Synthesis and thermal cure of high molecular weight polybenzoxazine precursors and the properties of the thermosets, Polymer, 2005, 46, 12172–12180 CrossRef CAS.
- A. Chernykh, T. Agag and H. Ishida, Synthesis of linear polymers containing benzoxazine moieties in the main chain with high molecular design versatility via click reaction, Polymer, 2009, 50, 382–390 CrossRef CAS.
- B. Kiskan, Y. Yagci and H. Ishida, Synthesis, characterization, and properties of new thermally curable polyetheresters containing benzoxazine moieties in the main chain, J. Polym. Sci., Part A: Polym. Chem., 2008, 46, 414–420 CrossRef CAS.
- M. Baqar, T. Agag, H. Ishida and S. Qutubuddin, Poly(benzoxazine-co-urethane)s: a new concept for phenolic/urethane copolymers via one-pot method, Polymer, 2011, 52, 307–317 CrossRef CAS.
- A. D. Baranek, L. L. Kendrick, J. Narayanan, G. E. Tyson, S. Wand and D. L. Patton, Flexible aliphatic-bridged bisphenol-based polybenzoxazines, Polym. Chem., 2012, 3, 2892–2900 RSC.
- A. Tuzun, B. Kiskan, N. Alemdar, A. T. Erciyes and Y. Yagci, Benzoxazine containing polyester thermosets with improved adhesion and flexibility, J. Polym. Sci., Part A: Polym. Chem., 2010, 48, 4279–4284 CrossRef CAS.
- B. S. Rao and A. Palanisamy, A new thermoset system based on cardanol benzoxazine and hydroxy benzoxazoline with lower cure temperature, Prog. Inorg. Chem., 2012, 74, 427–434 CAS.
- T. Agag, S. Y. An and H. Ishida, 1,3-bis(benzoxazine) from cashew nut shell oil and diaminodiphenyl methane and its composites with wood flour, J. Appl. Polym. Sci., 2013, 127, 2710–2714 CrossRef CAS.
- Y. P. Chen, A. Q. Dayo and W. B. Liu, Synthesis of cardanol-based phthalonitrile monomer and its copolymerization with phenol-aniline-based benzoxazine, J. Appl. Polym. Sci., 2019, 136, 47505–47513 CrossRef.
- A. B. Rajput, S. J. Rahaman, G. Sarkhel, M. K. Patra, S. R. Vadera, P. M. Singru, Y. Yagci and N. N. Ghosh, Synthesis, characterization, and properties of flexible magnetic nanocomposites of cobalt ferrite-polybenzoxazine-linear low-density polyethylene, J. Appl. Polym. Sci., 2012, 128, 3726–3733 CrossRef.
- S. Li, T. Zou, L. Feng, X. Liu and M. Tao, Preparation and properties of cardanol-based polybenzoxazine/SiO2 hybrids by sol-gel technique, J. Appl. Polym. Sci., 2012, 128, 4164–4171 CrossRef.
- D. J. Allen and H. Ishida, Physical and mechanical properties of flexible polybenzoxazine resins: effect of aliphatic diamine chain length, J. Appl. Polym. Sci., 2006, 101, 2798–2809 CrossRef CAS.
- T. Agag, L. Jin and H. Ishida, A new synthetic approach for difficult benzoxazines: preparation and polymerization of 4, 4’-diaminodiphenyl sulfone-based benzoxazine monomer, Polymer, 2009, 50, 613–626 CrossRef.
- T. Agag, A. Akelah, A. Rehab and S. Mostafa, Flexible polybenzoxazine thermosets containing pendent aliphatic chains, Polym. Int., 2012, 61, 124–128 CrossRef CAS.
- D. B. Austin, L. K. Laken, N. Jananee, E. T. Ginger, W. Steven and L. P. Derek, Flexible aliphatic-bridged bisphenol-based polybenzoxazine, Polym. Chem., 2012, 3, 2892–2898 RSC.
- T. Agag, S. Geiger, S. M. Alhassan, S. Qutubuddin and H. Ishida, Low-Viscosity Polyether-Based Main-Chain Benzoxazine Polymers: Precursors for Flexible Thermosetting Polymers, Macromolecules, 2010, 43, 7122–7127 CrossRef CAS.
- T. Takeichi, T. Kano, T. Agag, T. Kawauchi and N. Furukawa, Preparation of high molecular weight polybenzoxazine prepolymers containing siloxane unites and properties of their thermosets, J. Polym. Sci., Part A: Polym. Chem., 2010, 48, 5945–5952 CrossRef CAS.
- B. Gacal, L. Cianga, T. Agag, T. Takeichi and Y. Yagci, Synthesis and characterization of maleimide (Co)polymers with pendant benzoxazine groups by photoinduced radical polymerization and their thermal curing, J. Polym. Sci., Part A: Polym. Chem., 2007, 45, 2774–2786 CrossRef CAS.
- C. I. Chou and Y. L. Liu, High performance thermosets from a curable Diels-Alder polymer possessing benzoxazine groups in the main chain, J. Polym. Sci., Part A: Polym. Chem., 2008, 46, 6509–6517 CrossRef CAS.
- K. D. Demir, B. Kiskan, S. S. Latthe, A. L. Demirel and Y. Yagci, Thermally curable fluorinated main chain benzoxazine polyethers via Ullmann coupling, Polym. Chem., 2013, 4, 2106–2114 RSC.
- B. Aydogan, D. Sureka, B. Kiskan and Y. Yagci, Polysiloxane-containing benzoxazine moieties in the main chain, J. Polym. Sci., Part A: Polym. Chem., 2010, 48, 5156–5162 CrossRef CAS.
- T. Agag and T. Takeichi, Synthesis and characterization of novel benzoxazine monomers containing allyl groups and their high performance thermosets, Macromolecules, 2003, 36, 6010–6017 CrossRef CAS.
|
This journal is © The Royal Society of Chemistry 2020 |
Click here to see how this site uses Cookies. View our privacy policy here.