DOI:
10.1039/C9RA10171C
(Paper)
RSC Adv., 2020,
10, 3407-3415
A comparative study between Cu(INA)2-MOF and [Cu(INA)2(H2O)4] complex for a click reaction and the Biginelli reaction under solvent-free conditions†
Received
4th December 2019
, Accepted 9th January 2020
First published on 21st January 2020
Abstract
The catalytic activity of metal–organic framework Cu(INA)2 (INA = isonicotinate ion) and the complex [Cu(INA)2(H2O)4] were studied in the Copper-catalyzed Azide–Alkyne Cycloaddition (CuAAC) and Biginelli reaction under solvent-free reaction conditions. The robust, efficient and eco-friendly new method allowed the preparation of a variety of 1,2,3-triazole compounds in good to excellent yields and high selectivity for the 1,4-disubstituted triazole. Moreover, for the Biginelli reaction between aldehydes, ethyl acetoacetate and urea, the corresponding dihydropyrimidinones (DHPMs) were also obtained in satisfactory yields under mild reaction conditions for both catalysts. The comparative study between Cu(INA)2-MOF and [Cu(INA)2(H2O)4] complex demonstrated better results for the Cu-MOF, for both the yields and the regioselectivity of the products. Furthermore, no change in the heterogeneous catalyst structure was observed after the reaction, allowing them to be recovered and reused without any loss of activity.
Introduction
Coordination polymers of metal–organic frameworks (MOFs) have been gaining considerable attention in recent years in the field of catalysis. They have specific properties, such as porosity, high surface area, high thermal stability and high crystallinity, which make them potential catalysts in several organic reactions.1 These properties are possible due to the auto-organisation system of the MOFs, generated by the careful combination of a metallic centre and organic ligands, allowing the formation of a porous coordination polymer structure that may be either 2-dimensional or 3-dimensional.2 Moreover, these specific properties combined with the low solubility of MOFs permit their wide application as heterogeneous catalysts.3 This also facilitates the recovery and reuse of MOFs, which can be characterised as green and recyclable catalysts.4 In addition, other important applications of MOFs are gas storage, separation, and drug delivery, and they exhibit optical and magnetic properties.5
Among the MOFs used in heterogeneous catalysis, the copper metal–organic framework (Cu-MOF) has emerged as a potent and useful catalyst in several transformations as a result of the abundance, low toxicity and low cost of copper and because of the presence of a coordinatively unsaturated metal centre in its structure.6 For instance, Phan, Troung and co-workers have used Cu(INA)2-MOF (INA = isonicotinate ion) as a heterogeneous catalyst for the N-arylation of several heterocycles in excellent yields.7 More recently, the same research group reported the nucleophilic trifluoromethylation of aromatic boronic acids by using the same efficient Cu(INA)2-MOF catalyst.8
Depending on reaction conditions, the combination of Cu(II) and isonicotinate anion can produce two structures: the Cu(INA)2-MOF9,10 or the complex [Cu(INA)2(H2O)4].11,12 The difference between the copper catalytic centres of these structures is shown in Fig. 1. Despite the fact that these compounds are prepared by distinct methods, the hydrolysis of Cu(INA)2-MOF affords [Cu(INA)2(H2O)4], which is reverted to the MOF by thermal treatment.13
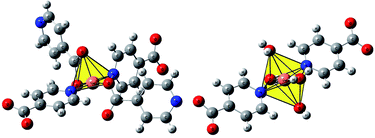 |
| Fig. 1 The copper catalytic centre of the Cu(INA)2-MOF (left, adapted from ref. 10b) and [Cu(INA)2(H2O)4] complex (right, adapted from ref. 11). Cu; O; N; C. | |
Aware of the effectiveness of Cu(INA)2-MOF in the organic reactions cited above, we decided to extend the use of this catalyst to the Copper-Catalysed Azide–Alkyne Cycloaddition (CuAAC) and Biginelli reactions, performing a comparative study of its catalytic activity with the [Cu(INA)2(H2O)4] complex. It is noteworthy that some MOFs have recently been used as catalysts for both of these kinds of reactions, affording the synthesis of corresponding 1,2,3-triazoles and dihydropyrimidinones (DHPMs), respectively.14,15 Both of these heterocyclic compounds have been widely studied as building blocks for the synthesis of biologically active compounds with important pharmacological properties.16,17 For example, triazoles and DHPMs containing an organoselenium unit (synthesised by molecular hybridisation strategy) have attracted considerable attention as potential multi-targeted anticancer or anti-Alzheimer's actives.18
Thus, in connection with our interests in the application of MOFs in catalysis19 and in the development of sustainable chemical protocols,20 herein we describe the straightforward methods for the click (CuAAC) and Biginelli multicomponent reactions, employing Cu(INA)2-MOF and the [Cu(INA)2(H2O)4] complex as catalysts, under solvent-free conditions. We found that the click reaction was fast and selective for the synthesis of 1,4-disubstituted-1,2,3-triazoles, including the synthesis of triazoles containing a selenium atom. Besides this, the reuse of the catalyst was shown to be possible.
Experimental
Materials and methods
All reagents and solvents were commercially available and used without any further purification. Thin-layer chromatography (TLC) was performed using Merck Silica Gel GF254, 0.25 mm thickness. Flash column chromatography was performed using Merck Silica Gel 60 (230–400 mesh). Melting points were measured on a Fisatom 430 D. All compounds were characterised by 1H NMR and 13C NMR; the spectra can be found in the ESI.† The spectra were obtained using a Bruker DPX 300 (1H at 300 MHz and 13C at 75 MHz), using CDCl3 and DMSO-d6 as solvents. All 1H NMR shifts are reported in δ units, parts per million (ppm), and were measured relative to the signal for TMS (0.00 ppm). Data are reported as follows: chemical shift (δ), multiplicity, coupling constant (J) in hertz and integrated intensity. Abbreviations to denote the multiplicity of a particular signal are as follows: s (singlet), d (doublet), t (triplet), q (quartet) and m (multiplet). All 13C NMR shifts are reported in ppm relative to deuterated-chloroform (77.23 ppm), unless otherwise stated, and all spectra were obtained with 1H decoupling. High-resolution mass spectra (HRMS) were recorded on a Shimadzu LCMS-IT-TOF ESI-TOF mass spectrometer. High-resolution exact mass measurements were performed using atmospheric-pressure chemical ionisation (APCI), positive mode, on a Bruker Daltonics micrOTOF-Q II mass spectrometer and electrospray ionization 10 (ESI), positive mode, on a quadrupole time-of-flight (TOF) Agilent QTOF-MS spectrometer. The thermogravimetric analyses (TGA) were performed on a PerkinElmer STA 6000 in a nitrogen atmosphere flowing at 10 mL min−1, with a heating rate of 50 °C min−1 from 25 °C to 900 °C. The X-ray diffraction (XRD) analyses were performed on a Bruker D2 Phaser Diffractometer equipped with CuKα radiation (λ = 1.5418 Å). The diffraction patterns were obtained at angles between 10° and 80° (θ–2θ).
Cu(INA)2-MOF synthesis
This MOF was synthesised using a slightly altered synthesis route based on a method reported previously.10 A mortar and pestle were used to grind a mixture of Cu(OAc)2·H2O (0.5 g, 2.5 mmol) and isonicotinic acid (0.5 g, 4 mmol) without solvent. The formation of the reaction product was indicated by the characteristic odour of acetic acid, released as a by-product. After this, the mixture was transferred to a beaker and was allowed to react for 24 h. The desired product still contained some water and acetic acid, so it was heated to 200 °C for 4 h to remove the by-products, to give the crystalline and porous framework in quantitative yield. The XRD analysis used to confirm the identity of Cu-MOF is found in Fig. 3. For IR and TGA analysis, see ESI.†
[Cu(INA)2(H2O)4]-complex synthesis
The complex of Cu(INA)2 was obtained according to literature procedure.12 For the XRD, IR and TGA analyses used to confirm the identity of the compound, see ESI.†
General procedure for the click reaction
In a 10 mL tube, benzyl azide or 4-azidoanisole (0.5 mmol), one of the acetylenes (0.5 mmol) and Cu(INA)2-MOF or [Cu(INA)2(H2O)4] complex (1 mg) were added and stirred at 80 °C for the time indicated in Table 2. The reaction was monitored by thin layer chromatography (TLC) to determine reaction time. The organic compounds were solubilised in dichloromethane, separated by centrifugation and concentrated under vacuum. For compounds 3f–k (Table 2) catalysed by Cu-complex, the crude product was purified by flash column chromatography through silica gel, using an appropriate mixture of hexane and ethyl acetate as the eluent. The identity and purity of the products were confirmed by 1H NMR, 13C NMR and melting points, and all spectral data were in perfect agreement with those reported in the literature (see ESI†).
General procedure for the Biginelli reaction
An aldehyde (0.5 mmol), urea (0.6 mmol), ethyl acetoacetate (1 mmol) and catalyst (10 mg) were placed in a 10 mL tube and stirred at 80 °C for the time indicated in Table 4. The organic compounds were then extracted with ethyl acetate (3 × 10 mL), filtered and concentrated under vacuum. The crude product was purified by flash column chromatography through silica gel, using an appropriate mixture of hexane and ethyl acetate as the eluent. The identity and purity of the products were confirmed by 1H NMR and 13C NMR, and all spectral data were in perfect agreement with those reported in the literature (see ESI†).
Recycling of the catalysts and leaching test
The recyclability of the catalysts was investigated. After complete separation of the organic phase of the reaction by centrifugation, the catalysts were washed with dichloromethane and then dried in an oven at 100 °C for 1 h. The dried solid catalyst was reused in subsequent reactions under solvent-free conditions, at 80 °C.
For metal leaching test the Inductively Coupled Plasma Optical Emission Spectrometer (ICP-OES) measurements were performed on a PerkinElmer Optima 7000 DV ICP-OES. The calibration curve was performed from copper concentrations of 1, 5, 10, 20 and 30 ppm, which obtained an r2 of 0.9999169. The sample was prepared by digestion of the organic matter with hot sulphuric acid and some drops of nitric acid.
Results and discussion
Catalytic studies of Cu(INA)2-MOF and [Cu(INA)2(H2O)4] complex in the synthesis of a 1,2,3-triazole
Initially, we focused our attention on the optimisation of the reaction conditions using benzyl azide (1a) and phenylacetylene (2a) as model substrates catalysed by Cu(INA)2-MOF or by [Cu(INA)2(H2O)4] complex (Table 1). The reaction time was determined by consumption of the starting materials (TLC analysis) or by the product precipitation. At first, the catalyst loading in the reaction system was evaluated. Initially, the reaction was performed with 10 mol% of [Cu(INA)2(H2O)4] complex, and the desired product 3a was obtained in 75% yield (Table 1, entry 1).
Table 1 Optimisation of click reactiona

|
Entry |
Catalyst (mol%) |
Time |
T (°C) |
Yieldb (%) |
Reaction conditions: benzyl azide (0.5 mmol), phenylacetylene (0.5 mmol). Isolated yield. Mixture of regioisomers. |
1 |
[Cu(INA)2(H2O)4]complex (10.0) |
10 min |
80 |
75 |
2 |
[Cu(INA)2(H2O)4] complex (5.0) |
8 min |
80 |
92 |
3 |
[Cu(INA)2(H2O)4] complex (1.0) |
12 min |
80 |
93 |
4 |
[Cu(INA)2(H2O)4] complex (0.5) |
20 min |
80 |
80 |
5 |
[Cu(INA)2(H2O)4] complex (1.0) |
4 h |
50 |
35 |
6 |
[Cu(INA)2(H2O)4] complex (1.0) |
24 h |
r.t. |
30 |
7 |
Cu(INA)2-MOF (5.0) |
2 min |
80 |
98 |
8 |
Cu(INA)2-MOF (1.0) |
4 min |
80 |
95 |
9 |
Cu(INA)2-MOF (1.0) |
15 min |
50 |
50 |
10 |
Cu(INA)2-MOF (0.5) |
10 min |
80 |
70 |
11 |
Cu(INA)2-MOF (1.0) |
18 h |
r.t. |
65 |
12 |
— |
6 h |
80 |
20c |
When the amount of the catalysts was decreased to 5 mol%, the yield increased significantly to 92 and 98% (Table 1, entries 2 and 7, respectively). Notably when 1 mol% of [Cu(INA)2(H2O)4] complex or Cu(INA)2-MOF was used, no significant change in the yield was observed, once the reaction is performed in solvent free conditions, a greater amount of solid impairs solubilisation under continuous stirring, thus even with a lower catalyst load the yield remains, furnishing the desired triazole 3a in 93% and 95% yield, respectively (Table 1, entries 3 and 8). However, further decreasing the amount of catalyst provided the product in lower yield in both cases (Table 1, entries 4 and 10).
Focusing on the influence of the temperature on the reaction, we discovered it had a great influence on the yield values. Thus, when the reaction was carried out at 50 °C, using [Cu(INA)2(H2O)4] complex for 4 h and Cu(INA)2-MOF for 15 min, the yield decreased to 35 and 50%, respectively (Table 1, entries 5 and 9). Moreover, a significant decrease in the yield and longer reaction time was observed when the reaction was carried out at room temperature (Table 1, entries 6 and 11).
It is important to note that, in the absence of catalyst, the reaction was not effective, and a mixture of 1,4- and 1,5-regioisomers of 1,2,3-triazoles was observed (Table 1, entry 12). This emphasises the activity and regioselectivity of Cu-MOF and Cu-complex in this kind of transformation.
Having established the best reaction conditions, we evaluated the scope of the protocol for both catalysts by using a variety of substrates identified in Table 2.
Table 2 Synthesis of Cu-MOF-catalysed 1,4-disubstituted-1,2,3-triazole derivativesa

|
Entry |
R1 |
R2 |
Product |
[Cu(INA)2(H2O)4]-complex |
Cu(INA)2-MOF |
Time |
Yieldb (%) |
Time (h) |
Yieldb (%) |
Reaction conditions: azide (0.5 mmol), acetylene (0.5 mmol), catalyst (1 mol%). Isolated yield. A mixture of 1 : 1.4 ratio of the 1,4- and 1,5-regioisomers determined by 1H NMR spectroscopy. |
1 |
PhCH2, 1a |
Ph, 2a |
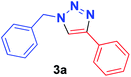 |
12 min |
93 |
4 min |
95 |
2 |
1a |
4-OMeC6H4, 2b |
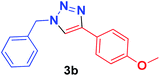 |
5 min |
85 |
4 min |
90 |
3 |
1a |
4-MeC6H4, 2c |
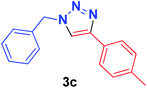 |
6 min |
81 |
5 min |
90 |
4 |
1a |
1-Naphthyl, 2d |
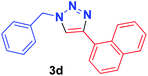 |
1 h |
97 |
1 h |
95 |
5 |
1a |
4-NH2C6H4, 2e |
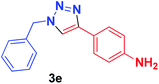 |
10 min |
70 |
10 min |
96 |
6 |
4-OMeC6H4, 1b |
2a |
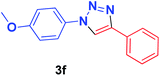 |
10 min |
86 |
10 min |
92 |
7 |
1b |
2e |
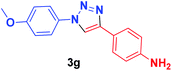 |
40 min |
70 |
40 min |
90 |
8 |
1b |
2c |
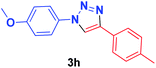 |
— |
— |
1 h |
95 |
9 |
1a |
PhCH2Se, 2f |
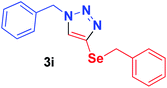 |
2 h |
80 |
2 h |
86 |
10 |
1a |
PhSe, 2g |
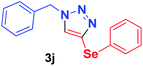 |
1.5 h |
96c |
1.5 h |
98 |
11 |
1a |
PhSeCH2, 2h |
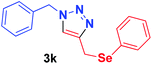 |
12 h |
50 |
12 h |
70 |
We explored the reaction of different aromatic acetylenes 2a–e with benzyl azide (1a), and no significant difference in reactivity was observed whether electron-withdrawing or electron-donating groups were attached to the aromatic ring, affording the desired 1,2,3-triazoles 3a–e in excellent yields and short reaction times (Table 2, entries 1–5). It is worth noting that the Cu(INA)2-MOF provided better results than the complex.
In addition, when the 4-azidoanisole (1b) was used as substrate in the presence of phenylacetylene (2a) and 4-ethynylaniline (2d), the corresponding products 3f and 3g were obtained in 86 and 70% yield, respectively, for [Cu(INA)2(H2O)4] complex. On the other hand, when Cu(INA)2-MOF was used as catalyst, the same products 3f and 3g were obtained in 92 and 90% yields, respectively (Table 2, entries 6 and 7). Furthermore, the product 3h was only synthesised in the presence of Cu-MOF (Table 2, entry 8).
Because of the important biological activity of triazoles containing an organoselenium unit,14 we decided to extend this reaction system to acetylenes containing the selenium atom, 2f–h. Thus, it was possible to synthesise the compounds 3i–k in satisfactory yields (50–98%) with both catalysts (Table 2, entries 9–11). However, the product 3j was obtained in an approximately 1
:
1.4 ratio of the 1,4- and 1,5-regioisomers under Cu-complex catalysis, whereas the reaction was regioselective (1,4-substituted triazole only) when catalysed by the Cu-MOF (Table 2, entry 10). It is worth mentioning that, the product 3i appears to have been synthesised herein for the first time in the literature.
It is remarkable that all products of the reactions with Cu(INA)2-MOF could be simply extracted with dichloromethane and separated by centrifugation without the necessity of purification by chromatographic column. However, when the [Cu(INA)2(H2O)4] complex was used, the products 3f–k required a simple purification by column.
Moreover, in order to investigate the synthetic utility of our methodology, we evaluated whether the reaction could be performed at the gram scale under the optimised conditions (Scheme 1). Fortunately, the corresponding product 3a was obtained in 95% yield using Cu(INA)2-MOF as catalyst and 90% using [Cu(INA)2(H2O)4] complex as catalyst, which is very important in organic synthesis, indicating that this methodology can be used to prepare 1,4-disubstituted-1,2,3-triazoles on a larger scale.
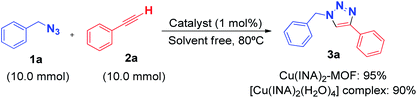 |
| Scheme 1 Scale-up of the reaction. | |
Catalytic studies of Cu(INA)2-MOF and [Cu(INA)2(H2O)4] complex in the Biginelli reaction
For the purpose of optimising our protocol, we used benzaldehyde (4a), urea (5) and ethyl acetoacetate (6) as common substrates. Evaluated were some parameters such as catalyst loading, temperature, reaction time and solvent (Table 3). The one-pot procedure was initially studied by using 10 mol% of [Cu(INA)2(H2O)4] complex in solvent-free conditions at 100 °C, affording the desired dihydropyrimidinone (DHPM) 7a in 90% yield (Table 3, entry 1). However, on decreasing the temperature to 80 °C, no significant difference in the yield was observed (Table 3, entry 2). On the order hand, when the reaction was performed at 50 °C, the product 7a was obtained in only 25% yield (Table 3, entry 3). Moreover, at room temperature the product was not obtained, and all starting material was recovered; this shows that temperature influences the reaction (Table 3, entry 4). In addition, when the reaction time was reduced from 2.5 to 2 h at 80 °C, no significant change in the yield was observed (Table 3, entry 5).
Table 3 Optimisation of Biginelli reactiona

|
# |
Cu(INA)2 (mol%) |
Time (h) |
T (°C) |
Yieldb (%) |
Reaction conditions: urea (0.6 mmol), benzaldehyde (0.5 mmol), ethyl acetoacetate (1.0 mmol) and Cu(INA)2 (10 mol%). Isolated yield. |
1 |
[Cu(INA)2(H2O)4] complex (10) |
2.5 |
100 |
90 |
2 |
[Cu(INA)2(H2O)4] complex (10) |
2.5 |
80 |
88 |
3 |
[Cu(INA)2(H2O)4] complex (10) |
2.5 |
50 |
25 |
4 |
[Cu(INA)2(H2O)4] complex (10) |
2.5 |
r.t. |
— |
5 |
[Cu(INA)2(H2O)4] complex (10) |
2.0 |
80 |
90 |
6 |
[Cu(INA)2(H2O)4] complex (10) |
1.5 |
80 |
80 |
7 |
[Cu(INA)2(H2O)4] complex (5) |
2.0 |
80 |
60 |
8 |
— |
2.0 |
80 |
35 |
9 |
Cu(INA)2-MOF (10) |
2.0 |
80 |
99 |
To evaluate the catalyst loading, we carried out the reaction with 5 mol% of [Cu(INA)2(H2O)4] complex, and the yield of the desired product was reduced to 60% (Table 3, entry 7). It is notable that, in the absence of the catalyst, the DHPM 7a was obtained in only 35% yield (Table 3, entry 8).
Aiming to determine the effect of the MOF in the Biginelli reaction, we extended our Cu-complex protocol in Table 3, entry 5, by substituting Cu(INA)2-MOF as catalyst. As a result, the product 7a was obtained in almost quantitative yield (Table 3, entry 9). Next, we explored the generality of this protocol for both catalysts, performing a comparative study between catalytic activity of the [Cu(INA)2(H2O)4] complex and of the Cu(INA)2-MOF in the Biginelli reaction (Table 4).
Table 4 Synthesis of DHPM derivatives under Cu(INA)2 catalysisa

|
Entry |
R |
Product |
[Cu(INA)2(H2O)4] complex |
Cu(INA)2-MOF |
Time (h) |
Yieldb (%) |
Time (h) |
Yieldb (%) |
Reaction conditions: aldehyde (0.5 mmol), urea (0.6 mmol), ethyl acetoacetate (1.0 mmol) and catalyst (10 mol%). Isolated yield. |
1 |
C6H5, 4a |
7a |
2.0 |
90 |
2.0 |
99 |
2 |
4-OMeC6H4, 4b |
7b |
2.5 |
71 |
2.5 |
80 |
3 |
2-OMeC6H4, 4c |
7c |
2.5 |
80 |
2.5 |
82 |
4 |
3,4,5-OMeC6H2, 4d |
7d |
2.5 |
44 |
2.5 |
55 |
5 |
4-MeC6H4, 4e |
7e |
2.0 |
50 |
2.0 |
55 |
6 |
3-OH-C6H4, 4f |
7f |
2.0 |
50 |
2.0 |
55 |
7 |
2-NO2C6H4, 4g |
7g |
1.0 |
82 |
1.0 |
85 |
8 |
2-Pyridine, 4h |
7h |
2.5 |
68 |
2.5 |
72 |
9 |
2-Naphthyl, 4i |
7i |
24.0 |
25 |
24.0 |
33 |
Firstly, the reaction was conducted with several aldehydes, using the optimal reaction conditions catalysed by [Cu(INA)2(H2O)4] complex. For example, when the reaction was performed using different electron-donating groups attached to the aromatic ring, such as 4-methoxy, 2-methoxy, 3,4,5-trimethoxy, 4-methyl and 3-hydroxy substituents, which decrease the aldehyde reactivity, the corresponding DHPMs 7b–f were obtained in 44–80% yields (Table 4, entries 2–6).
On the other hand, when we used aldehydes containing electron-withdrawing groups, such as ortho-nitrobenzaldehyde (4g), the desired product 7g was obtained in 82% yield (Table 4, entry 7). Moreover, it was possible to synthesise a DHPM compound 7h containing a heterocyclic unit from 2-pyridinecarboxaldehyde (4h) in 68% yield (Table 4, entry 8). Subsequently, the use of naphthaldehyde (4i) afforded the corresponding product 7i in 25% yield; this low yield could be associated with hindrance due to the bulky and rigid naphthyl group (Table 4, entry 9).
The same aldehydes 4a–i were used for the Biginelli reaction catalysed by Cu(INA)2-MOF, and in all cases the DHPMs 7a–i were obtained in better yields compared to Cu-complex (Table 4, entries 1–9).
Thus, according to the reactivity of Cu-(INA)2-MOF compared with the Cu-complex, some points can be noted regarding the structures of these compounds. The copper catalytic centre is less sterically hindered in the MOF structure because the geometry is distorted square pyramidal, while in the complex it is octahedral (Fig. 1). Moreover, for the cycloaddition reaction, a catalyst–substrate bond is necessary, and for this to occur, at least one of the water molecules coordinated to the metal must be removed in the case of the complex.
Recyclability
In order to evaluate the recyclability of the catalysts, we recovered the [Cu(INA)2(H2O)4]-complex and the Cu(INA)2-MOF and reused them in subsequent reactions between benzyl azide (1a) and phenylacetylene (2a) (Fig. 2). So, after the reaction was finished, the product 3a was solubilised with dichloromethane and separated by centrifugation. After isolation of the liquid organic phase and evaporation of the solvent, the catalysts were reused directly, without any further treatment. The recovered catalysts were then used for five more reaction cycles each, without a significant decrease in the yield of product 3a.
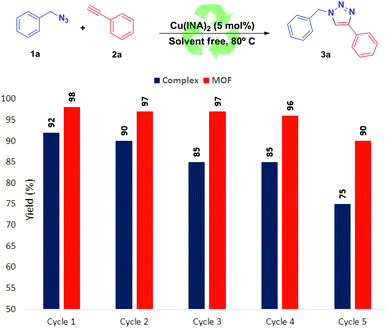 |
| Fig. 2 Catalyst reuse. | |
However, the Cu(INA)2-MOF produced a better result in catalyst reuse, as shown in Fig. 2.
The crystal structure of Cu(INA)2-MOF remained unchanged after five catalytic cycles, as shown in the X-ray diffraction patterns in Fig. 3.
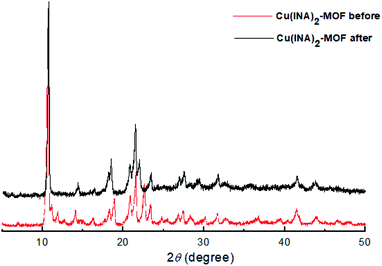 |
| Fig. 3 XRD analysis of Cu(INA)2-MOF before and after five catalytic cycles. | |
Leaching test
The heterogeneity of CuAAC reaction was investigated by the leaching test, where the amount of copper was determined by ICP-OES analysis of the solid obtained after the reaction. Thus, the catalyst was filtered with dichloromethane (using a polypropylene syringe filter) and the solvent was evaporated under reduced pressure. After this, digestion of the organic sample was performed and analysed by ICP-OES. The analysis indicates that there are no traces of leached copper into the sample.
A plausible mechanism for click reaction
On the basis of the work of Luz et al.,21 which did not found evidence of Cu(I) species generated during Cu-MOF catalysed 1,3-dipolar cycloaddition reaction, it was proposed that the active species for the click reaction with Cu-MOF consist of Cu(II) active sites. Thus, in accordance with previous reports,21,22 a plausible reaction pathway for the synthesis of 1,4-disubstituted-1,2,3-triazoles is illustrated in Scheme 2.
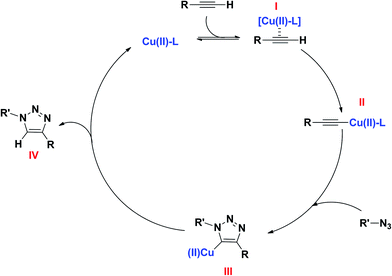 |
| Scheme 2 A plausible reaction pathway for the synthesis of 1,4-disubstituted-1,2,3-triazoles. | |
In this pathway the catalytic cycle begins with the copper coordination to the alkyne (I), which increases the acidity of terminal alkyne, affording the formation of copper acetylide (II). Subsequently, this species reacts with organic azide, via 1,3-dipolar cycloaddition, to afford the triazolyl-copper species (III). Lastly, the desired triazole (IV) is obtained through protonation of intermediate III, followed by catalyst regeneration.
Conclusions
We report herein the catalytic activity of Cu(INA)2-MOF in the Copper-Catalysed Azide–Alkyne Cycloaddition (CuAAC) and Biginelli reactions and the comparative study with its analogous complex [Cu(INA)2(H2O)4]. The straightforward methodologies allowed the preparation of 1,2,3-triazoles and dihydropyrimidinones (DHPMs) under solvent-free conditions. Remarkably, the Cu-MOF was found to be a more highly efficient catalyst than the Cu-complex for these reactions, affording the desired products in very high yields and producing selectively the 1,4-disubstituted-1,2,3-triazoles. These MOF-catalysed reactions showed advantages that include the use of a recyclable catalyst, ease of scale-up to gram scale, atom-economy, short reaction time, absence of base and reducing agents and a solvent-free approach. Indeed, all these features make this a green alternative for easy access to 1,4-disubstituted-1,2,3-triazoles and DHPMs.
Conflicts of interest
The authors declare no conflict of interest.
Acknowledgements
The authors gratefully thank Araucaria Foundation for a fellowship to FGM (FAAP-Unioeste 003/2017) and The National Council Scientific and Technological Development for financial support (CNPQ-429831/2018-8).
Notes and references
-
(a) Y.-Z. Chen, R. Zhang, L. Jiao and H.-L. Jiang, Coord. Chem. Rev., 2018, 362, 1–23 CrossRef CAS;
(b) Y.-B. Huang, J. Liang, X.-S. Wang and R. Cao, Chem. Soc. Rev., 2017, 46, 126–157 RSC;
(c) C. J. Doonan and C. J. Sumby, CrystEngComm, 2017, 19, 4045–4049 RSC.
-
(a) H. Furukawa, K. E. Cordova, M. O'Keeffe and A. M. Yaghi, Science, 2013, 341, 1230444 CrossRef PubMed;
(b) R. J. Kuppler, D. J. Timmons, Q.-R. Fang, J.-R. Li, T. A. Makal, M. D. Young, D. Yuan, D. Zhao, W. Zhuang and H.-C. Zhou, Coord. Chem. Rev., 2009, 253, 3042–3066 CrossRef CAS;
(c) A. Y. Robin and K. M. Fromm, Coord. Chem. Rev., 2006, 250, 2127–2157 CrossRef CAS.
-
(a) L. Zhu, X.-Q. Liu, H.-L. Jiang and L.-B. Sun, Chem. Rev., 2017, 117, 8129–8176 CrossRef CAS PubMed;
(b) A. H. Chughtai, N. Ahmad, H. A. Younus, A. Laypkov and F. Verpoort, Chem. Soc. Rev., 2015, 44, 6804–6849 RSC;
(c) J. Gascon, A. Corma, F. Kapteijn and F. X. Llabrés i Xamena, ACS Catal., 2014, 4, 361–378 CrossRef CAS;
(d) D. Deng, H. Guo, B. Ji, W. Wang, L. Ma and F. Luo, New J. Chem., 2017, 41, 12611–12616 RSC.
-
(a) X. Li, F. Chen and G.-P. Lu, Tetrahedron Lett., 2018, 59, 4226–4230 CrossRef CAS;
(b) R. Oozeerally, D. V. Burnett, T. W. Chamberlain, R. I. Walton and V. Degirmenci, ChemCatChem, 2018, 10, 706–709 CrossRef CAS PubMed;
(c) C.-S. Cao, Y. Shi, H. Xu and B. Zhao, Dalton Trans., 2018, 47, 4545–4553 RSC;
(d) A. Chatterjee, X. Hu and F. L.-Y. Lam, Catal. Today, 2018, 314, 129–136 CrossRef CAS;
(e) J. C. R. Freitas, C. K. Oliveira, E. C. Cunha, I. Malvestiti, S. Alves Jr, R. L. Longo and P. H. Menezes, Tetrahedron Lett., 2013, 54, 1558–1561 CrossRef CAS.
-
(a) H. Li, K. Wang, Y. Sun, C. T. Lollar, J. Li and H.-C. Zhou, Mater. Today, 2018, 21, 108–121 CrossRef CAS;
(b) B. Li, H.-M. Wen, W. Zhou and B. Chen, J. Phys. Chem. Lett., 2014, 5, 3468–3479 CrossRef CAS PubMed;
(c) M.-X. Wu and Y.-W. Yang, Adv. Mater., 2017, 29, 1606134 CrossRef PubMed;
(d) L. Wang, M. Zheng and Z. Xie, J. Mater. Chem. B, 2018, 6, 707–717 RSC;
(e) G. M. Espallargas and E. Coronado, Chem. Soc. Rev., 2018, 47, 533–557 RSC.
-
(a) Z. Ahmadzadeh, J. Mokhtari and M. Rouhani, RSC Adv., 2018, 8, 24203–24208 RSC;
(b) A. Taher, D. W. Kim and I.-M. Lee, RSC Adv., 2017, 7, 17806–17812 RSC;
(c) Y. Jiang, J. Huang, M. Hunger, M. Maciejewskia and A. Baikera, Catal. Sci. Technol., 2015, 5, 897–902 RSC;
(d) P. Rani and R. Srivastava, Tetrahedron Lett., 2014, 55, 5256–5260 CrossRef CAS;
(e) N. T. S. Phan, T. T. Nguyen and P. H. L. Vu, ChemCatChem, 2013, 5, 3068–3077 CrossRef CAS;
(f) I. Luz, F. X. Llabrés i Xamena and A. Corma, J. Catal., 2012, 285, 285–291 CrossRef CAS;
(g) D. Deng, H. Guo, G. Kang, L. Ma, X. He and B. Ji, CrystEngComm, 2015, 17, 1871–1880 RSC.
- T. Truong, C. V. Nguyen, N. T. Truong and N. T. S. Phan, RSC Adv., 2015, 5, 107547–107556 RSC.
- T. V. Nguyen, T. D. Ong, A. H. M. Lam, V. T. Pham, N. T. S. Phan and T. Truong, Mol. Catal., 2017, 436, 60–66 CrossRef CAS.
- Y. Liu, Y. Lu, H. Tsai, J. Wang and K. Lu, J. Solid State Chem., 2001, 158, 315–319 CrossRef CAS.
-
(a) A. Pichon, A. Lazuen-Garay and S. L. James, CrystEngComm, 2006, 8, 211–214 RSC;
(b) P. Wang, G. Li, Y. Chen, S. Chen, S. L. James and W. Yuan, CrystEngComm, 2012, 14, 1994–1997 RSC.
- M. Almášia, Z. Vargováa, R. Gyepesb, R. Vargac and V. Zeleňák, Inorg. Chem. Commun., 2014, 46, 118–121 CrossRef.
- K. Waizump, M. Takuno, N. Fukushima and H. Masuda, J. Coord. Chem., 1998, 44, 269–279 CrossRef.
- C. Lin, S. Chui, S. Lo, F. Shek, M. Wu, K. Suwinska, J. Lipkowski and I. Williams, Chem. Commun., 2002, 15, 1642–1643 RSC.
- For MOFs in CuAAC reaction:
(a) X. Jia, G. Xu, Z. Du and Y. Fu, Polyhedron, 2018, 151, 515–519 CrossRef CAS;
(b) Y.-H. Hu, J.-C. Wang, S. Yang, Y.-A. Li and Y.-B. Dong, Inorg. Chem., 2017, 56, 8341–8347 CrossRef CAS PubMed;
(c) P. Li, S. Regati, H. Huang, H. D. Arman, J. C.-G. Zhao and B. Chen, Inorg. Chem. Front., 2015, 2, 42–46 RSC;
(d) I. Luz, F. X. Llabrés i Xamena and A. Corma, J. Catal., 2010, 276, 134–140 CrossRef CAS.
- For MOFs in Biginelli reaction:
(a) A. K. Gupta, D. De, K. Tomar and P. K. Bharadwaj, Dalton Trans., 2018, 47, 1624–1634 RSC;
(b) A. Verma, D. De, K. Tomar and P. K. Bharadwaj, Inorg. Chem., 2017, 56, 9765–9771 CrossRef CAS PubMed;
(c) T. K. Pal, D. De, S. Senthilkumar, S. Neogi and P. K. Bharadwaj, Inorg. Chem., 2016, 55, 7835–7842 CrossRef CAS PubMed;
(d) A. Dakshinamoorthy and H. Garcia, Chem. Soc. Rev., 2014, 43, 5750–5765 RSC.
- For pharmacological properties of the triazole derivatives:
(a) Y. Tian, Z. Liu, J. Liu, B. Huang, D. Kang, H. Zhang, E. De Clercq, D. Daelemans, C. Pannecouque, K.-H. Lee, C.-H. Chen, P. Zhan and X. Liu, Eur. J. Med. Chem., 2014, 151, 339–350 CrossRef PubMed;
(b) N. M. Meghani, H. H. Amin and B.-J. Lee, Drug Discovery Today, 2017, 22, 1604–1619 CrossRef CAS PubMed;
(c) D. Dheer, V. Singh and R. Shankar, Bioorg. Chem., 2017, 71, 30–54 CrossRef CAS PubMed;
(d) F. Pagliai, T. Pirali, E. Del Grosso, R. Di Brisco, G. C. Tron, G. Sorba and A. A. Genazzani, J. Med. Chem., 2006, 49, 467–470 CrossRef CAS PubMed;
(e) H. C. Kolb and K. B. Sharpless, Drug Discovery Today, 2003, 8, 1128–1137 CrossRef CAS PubMed.
- For recent pharmacological properties of the DHPM derivatives:
(a) V. Vendrusculo, V. P. Souza, L. A. M. Fontoura, M. G. M. D'Oca, T. P. Banzato, P. A. Monteiro, R. A. Pilli, J. E. Carvalho and D. Russowsky, Med. Chem. Commun., 2018, 9, 1553–1564 RSC;
(b) F. S. De Oliveira, P. M. De Oliveira, L. M. Farias, R. C. Brinkerhoff, R. C. M. A. Sobrinho, T. M. Treptow, C. R. M. D'Oca, M. A. G. Marinho, M. A. Hort, A. P. Horn, D. Russowsky and M. G. M. D'Oca, Med. Chem. Commun., 2018, 9, 1282–1288 RSC;
(c) H. Kaoukabi, Y. Kabri, C. Curti, M. Taourirte, J. C. Rodriguez-Ubis, R. Snoeck, G. Andrei, P. Vanelle and H. B. Lazrek, Eur. J. Med. Chem., 2018, 155, 772–781 CrossRef CAS PubMed;
(d) M. Damgaard, A. Al-Khawaja, M. Nittegaard-Nielsen, R. F. Petersen, P. Wellendorph and B. Frølund, Eur. J. Med. Chem., 2017, 138, 300–312 CrossRef CAS PubMed;
(e) R. Kaur, S. Chaudhary, K. Kumar, M. K. Gupta and R. K. Rawal, Eur. J. Med. Chem., 2017, 132, 108–134 CrossRef CAS.
-
(a) F.-H. Cui, J. Chen, Z.-Y. Mo, S.-X. Su, Y.-Y. Chen, X.-l. Ma and H.-T. Tang, Org. Lett., 2018, 20, 925–929 CrossRef CAS PubMed;
(b) E. H. G. da Cruz, M. A. Silvers, G. A. M. Jardim, J. M. Resende, B. C. Cavalcanti, I. S. Bomfim, C. Pessoa, C. A. de Simone, G. V. Botteselle, A. L. Braga, D. K. Nair, I. N. N. Namboothiri, D. A. Boothman and E. N. S. Júnior, Eur. J. Med. Chem., 2016, 122, 1–16 CrossRef CAS PubMed;
(c) F. A. R. Barbosa, R. F. S. Canto, S. Saba, J. Rafique and A. L. Braga, Bioorg. Med. Chem., 2016, 24, 5762–5770 CrossRef CAS PubMed;
(d) R. F. S. Canto, F. A. R. Barbosa, V. Nascimento, A. S. de Oliveira, I. M. C. Brighente and A. L. Braga, Org. Biomol. Chem., 2014, 12, 3470–3477 RSC.
- T. A. Grigolo, S. D. Campos, F. Manarin, G. V. Botteselle, P. Brandão, A. A. Amaral and E. A. Campos, Dalton Trans., 2017, 46, 15698–15703 RSC.
-
(a) Y. H. Matzkeit, B. L. Tornquist, F. Manarin, G. V. Botteselle, J. Rafique, S. Saba, A. L. Braga, J. F. Felix and R. Schneider, J. Non-Cryst. Solids, 2018, 498, 153–159 CrossRef CAS;
(b) S. Saba, G. V. Botteselle, M. Godoi, T. E. A. Frizon, F. Z. Galetto, J. Rafique and A. L. Braga, Molecules, 2017, 22, 1367 CrossRef PubMed;
(c) M. Godoi, G. V. Botteselle, J. Rafique, M. S. T. Rocha, J. M. Pena and A. L. Braga, Eur. J. Org. Chem., 2013, 5188–5194 Search PubMed.
- I. Luz, F. X. Llabrés i Xamena and A. Corma, J. Catal., 2010, 276, 134–140 CrossRef CAS.
- F. Himo, T. Lovell, R. Hilgraf, V. V. Rostovtsev, L. Noodleman, K. B. Sharpless and V. V. Fokin, J. Am. Chem. Soc., 2005, 127, 210–216 CrossRef CAS PubMed.
Footnote |
† Electronic supplementary information (ESI) available. See DOI: 10.1039/c9ra10171c |
|
This journal is © The Royal Society of Chemistry 2020 |