DOI:
10.1039/C9RA10046F
(Paper)
RSC Adv., 2020,
10, 5905-5912
An experimental study on the removal of submicron fly ash and black carbon in a gravitational wet scrubber with electrostatic enhancement
Received
1st December 2019
, Accepted 13th January 2020
First published on 6th February 2020
Abstract
It is of great significance to adopt a cost-effective and highly efficient method to capture submicron particles produced by small-scale industrial boilers. In this study, a middle-scale wet electrostatic scrubbing (WES) setup was built to investigate its performance in the removal of both fly ash particles and black carbon, with special attention to the submicron size range. Major factors including the particle properties and charging conditions were expatiated in detail to popularize this method. The results showed that the efficiency increase in black carbon is significantly higher than that of fly ash particles at the charging condition. For the case of droplet charging, the highest efficiency increase in black carbon in the submicron size range is up to 60%, while that of fly ash is only 40% under same conditions. In comparison with particle charging, droplet charging plays a more significant role in removing both fly ash particles and black carbon, which is beneficial for reforming conventional wet scrubbers. Moreover, more small particles could adhere to the surface of large fly ash particles after scavenging while this phenomenon was not found for black carbon due to the characteristic of fractal agglomerates.
1. Introduction
It is predicted that coal will remain the second-largest primary energy source until 2040.1,2 Thus, reducing the particulate matter emitted by coal combustion remains an exacting task,3–6 particularly for a large number of small-scale industrial boilers. On account of inefficient combustion modes, these boilers can produce not only a substantial number of submicron fly ash but also black carbon,7 which might cause extremely detrimental effects on both human health and the atmospheric environment. On the one hand, submicron particles emitted from fossil fuels usually contain a great deal of toxic substances, such as heavy metals.8,9 These fine particles can penetrate far down into people's respiratory tracts, causing pulmonary injury and other diseases.10 On the other hand, atmospheric visibility can be heavily reduced by numerous submicron particles suspended in the atmosphere due to their long retention time.10 Even worse, black carbon particles also contribute to global warming because of their high absorbance of visible and IR light.10 Therefore, reducing the emission of submicron particles is of great importance to human sustainable development. In comparison with submicron fly ash particles, black carbon particles are usually more difficult to remove by wet scrubbers, which are used in a large proportion of small-scale boilers. Even though the total mass collection efficiency is already high,11 a large number of submicron particles, as the major contributor of atmospheric haze, can still penetrate through the wet tower.12–14 In order to overcome this issue in a cost-effective manner, a hybrid system combined with an electrostatic field, namely wet electrostatic scrubbing (WES), seems to be an ideal solution.15
WES is an upgrade to conventional wet scrubbing and has the advantages of simple configuration, low cost and a low pressure drop compared with other highly efficient dust-abatement technologies, such as bag filters.16 Experimental results acquired by Natale et al. showed that the water consumption of WES is one order of magnitude lower than that of inertial scrubbers.17 Pui et al. stated that WES is cheaper to operate than conventional wet scrubbers due to the lower water consumption and pressure drop.18 Jaworek et al. evaluated the electro-spraying devices and concluded that their energy consumption is negligible in contrast to the energy required for electrostatic precipitators.19 Based on the above-mentioned investigations, WES could be regarded as a cost-effective technique, which is suitable to reform wet scrubbers used in small-scale industrial boilers.
In addition to the economic feasibility, WES also has the technological rationality in that it significantly increases the collection efficiency of submicron particles16,20–22 because all charged droplets suspended in the tower are highly efficient moving collectors. Extensive studies have been reported to elaborate the principle and performance of this technique.17,19,23–25 Balachandran et al. investigated the removal of cigarette smoke, as the source of submicrometer dust, by charged droplets, indicating that the cleaning performance was four times better than that in uncharged cases.26 Jaworek et al. built a multi-nozzle electrospray system and found that the removal efficiency of smoke particles (<1 μm) is up to 80–90% with a very low water consumption.27 In addition, Jaworek et al. conducted a series of studies that included theories, simulations and reviews to expatiate the development of WES.15,19,28 Ha et al. employed emulsion oil as the particle source to evaluate the effectiveness of WES in the purification of marine exhaust gas. The results showed that the total PM collection efficiency was higher than 99%; however, the study did not give a special attention to submicron particles.29 D'Addio et al. designed a delicate setup to decouple the effects of droplet–particle interactions in the dynamic process of WES. Submicron particles used in the experiment were produced by burning an incense stick, and the corresponding results were proved to be in accordance with the atmospheric particle scavenging model.30,31 Natale et al. used the WES technique to purify both bacterial bioaerosol and combustion-source aerosol produced by a gasoline flame, validating the great potential of this technique.17,32 However, there are very few reports that extend this method to capture black carbon and fly ash particles produced in small-scale industrial boilers. Due to the differences in the particle properties, whether this method is effective or not is still ambiguous.
In this study, we built a medium-scale WES apparatus to investigate the performance of WES to remove both fly ash particles and black carbon. Special attention in this study is given to compare the removal characteristics of two types of submicron particles. It is expected that the WES technique could be applied in small-scale and coal-fired boilers in the future.
2. Experiment section
2.1 Setup and method
The layout of the experimental apparatus in this study is shown in Fig. 1. It mainly consists of particle generation, particle charging, droplet charging, a WES reactor and particle measurement. First of all, the airflow was filtered to generate a particle-free flow, and the standardized flow rate (180 m3 h−1) was monitored by a high-precision turbine flowmeter (LWQ-100, Fulang Instrument). On this basis, a micro-powder feeder (TWLX-15, Dongfang) was used to deliver the selected powder into the clean airflow to produce a particle-laden airflow. In order to avoid any effects from the moisture on the particle size distribution, the micro-powder feeder was continuously warmed to 55 °C by an electric tracing band. Then, the particle-laden gas stream went through a rotational motion in flow-equalizing equipment to evenly distribute the particles over the cross section of the gas channel. Subsequently, the stream entered into a wire-tube electrostatic precipitator (ESP, diameter: 100 mm), in which the residence time of the particle is approximately 0.1 s to reduce particle deposition on the anode surface. The ground electrode was composed of stainless steel, and the cathode was a nickel wire with a diameter of 0.5 mm. To further reduce the probability of particle loss in ESP, the maximum applied voltage on the cathode was limited to −16 kV, which is slightly higher than the corona inception voltage (−11.5 kV). At this voltage, not only could particles acquire charges, but also charged particles had a relatively low migration velocity to the ground electrode, so the charged particles could penetrate through the ESP successfully. Particles escaping from the ESP were introduced into a WES tower (diameter: 250 mm, height: 1.2 m) made by plexiglass. At the top cap of the tower, an electrically grounded twin-fluid nozzle (1/4JN-SS+SU12-SS, http://www.Shzoyo.com) was located in the center to produce a large number of droplets of tens to hundreds of micrometers. A coaxial copper ring of 93 mm in diameter was installed at the distance of 10 mm away from the bottom of the nozzle tip, so all the droplets could completely pass through the ring electrode. For the sake of charging droplets positively by the induction principle, a negative voltage up to −4000 V was applied on the ring electrode by a high-voltage power (DW-N303-1ACFD, Dongwen). The charged water spray and the particle-laden gas stream flowed co-currently downward along the tower.21 The temperature in this tower was monitored to ensure that the experiments were performed at a constant temperature. After scrubbing, a small percentage of the droplets could escape in the outflowing gas, which were removed at the exit of the tower before an induced-draft fan.21
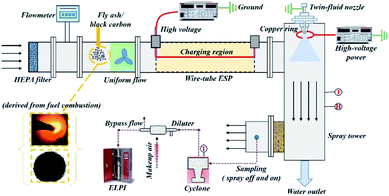 |
| Fig. 1 Wet electrostatic scrubbing setup. | |
The fractional particle concentrations before and after scrubbing were measured in real time via an Electrical Low Pressure Impactor (ELPI, Dekati). Before reaching the ELPI, large particles (>10 μm in aerodynamic diameter) in the sampling flow were removed by a cyclone separator to prevent the first-stage electrometer in the ELPI from overloading. Then, the dry particle-free makeup air was pumped into the sampling gas to dilute the particle concentration and reduce measurement interference caused by vapour condensation. In addition, it should be noted that the sampling position before scrubbing was also located at the outlet of the tower as long as both the high-voltage powers and nozzle were turned off. Fractional particle collection efficiency could be evaluated using the following equation:
|
η0(dp) = (n0(dp) − n1(dp))/n0(dp) × 100%,
| (1) |
where
n0(
dp) is the initial number concentration and
n1(
dp) is the number concentration after conventional wet scrubbing. For droplet charging or (and) particle charging, the efficiency increase could be calculated by
eqn (2):
|
η1(dp) = (n1(dp) − n2(dp))/n1(dp) × 100%,
| (2) |
where
n2(
dp) is the number concentration after wet electrostatic scrubbing.
2.2 Particle characterization
Two kinds of particles were adopted in the experiment, including fly ash produced in a coal-fired boiler and industrial black carbon. The morphologies of both the fly ash and black carbon were acquired by transmission electron microscope (TEM) tests, as shown in Fig. 2. Spherical particles account for a large proportion of the fly ash particles, while agglomerates account for the black carbon.
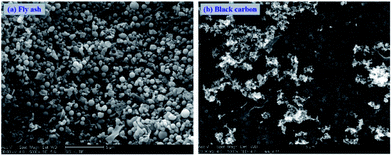 |
| Fig. 2 Particle morphology (a) fly ash, (b) black carbon. | |
Hydrophilic angles for two kinds of particles were measured using a hydrophilic angle analyzer (SZ-CAMB1, Germany), as shown in Fig. 3. First of all, the powder samples were pressed into disks. Then, distilled water droplets produced by a syringe fell on the disks. The shape of the water droplets on the disks was photographed by a micro-camera, and the corresponding hydrophilic angle was analyzed using the tangent method. Three measurements were made per sample in the hydrophilic angle analysis to prove the repeatability.
 |
| Fig. 3 Hydrophilic angle (a) fly ash, (b) black carbon. | |
The initial particle size distributions for both the fly ash and black carbon were measured by ELPI, as shown in Fig. 4. It could be clearly seen that the two kinds of particles are characterized by a similar size distribution, which minimizes the effect of particle concentration on the comparison of collection efficiency.
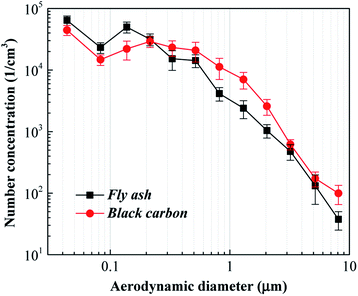 |
| Fig. 4 Particle size distributions of both fly ash and black carbon. | |
The measurements of particle charges acquired in a corona field were achieved using the multi-stage electrometers assembled in ELPI, but the charger in ELPI was turned off in this test. In order to reduce the loss of charged particles before ELPI, the maximum length of the sampling tube was restricted to 300 mm. Table 1 shows the mean charge of both the fly ash particles and black carbon.
Table 1 Mean charge of particles at 12 kV (E = 1.2 kV cm−1)a
Qf: Charges carried by fly ash; Qb: charges carried by black carbon. |
dp (μm) |
0.08 |
0.21 |
0.52 |
0.82 |
Qf (C) |
2.35 × 10−19 |
8.82 × 10−19 |
3.05 × 10−18 |
6.22 × 10−18 |
Qb (C) |
2.89 × 10−19 |
9.8 × 10−19 |
4.65 × 10−18 |
7.58 × 10−18 |
2.3 Droplet characterization
For droplet charging characterization, a Faraday cage was designed, as shown in Fig. 5. It was mainly made up of three-layer coaxial cylinders (diameter: 200 mm). The inner cylinder, composed of stainless steel, was connected to an electrometer to detect the induced current, while the outer metal layer was grounded to shield it from external electric noise. In the middle of the two metal layers, a cylinder made up of polytetrafluoroethylene (PTFE) acted as an insulator to isolate the electrified layer from the grounded layer. By means of the Faraday cage, the mean charge of the spray droplets could be evaluated using eqn (3):where I is the induced current measured using an electrometer, Qv is the spray volume flow rate, and Dav is the mean diameter of the spray droplets. For simplicity, in this study, the Sauter mean diameter (D32) used for a twin-fluid nozzle33 was adopted as the equivalent size to evaluate average droplet charge, as shown in eqn (4): |
D32 = 0.682dnRen−0.224Wen−0.456f−0.176,
| (4) |
where dn is the diameter of the nozzle, and f is the mass-flow rate ratio of the gas side to the liquid side. Ren and Wen are the Reynolds number and Weber number, respectively, with the characteristic dimension dn. Using the above equations, the mean charge of the droplets with the average diameter of 249.5 μm was calculated, as shown in Table 2. It should be noteworthy that the effects of induction charging on the droplet size were neglected in this study.
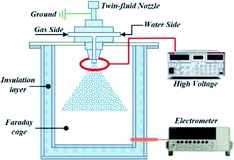 |
| Fig. 5 Experimental apparatus for droplet charging measurement. | |
Table 2 Mean charge of droplets at 8 L h−1 (0.042 L m−3)a
U, induction voltage. |
U (V) |
−1000 |
−2000 |
−3000 |
−4000 |
Qd (C) |
6.78 × 10−14 |
1.38 × 10−13 |
2.36 × 10−13 |
3.95 × 10−13 |
3. Results and discussion
3.1 Particle collection efficiency of the conventional wet scrubber at different liquid-to-gas ratios
The collection efficiencies of fly ash and black carbon using a conventional wet scrubber are shown in Fig. 6(a) and (b), respectively. Similar trends at different liquid-to-gas ratios could be clearly seen for two types of particles. In the range of 0.1 μm to 1 μm, the collection efficiency attains the minimum, which is due to the fact that either Brownian diffusion or impaction is not a dominant mechanism. For larger particles (1 μm to 5 μm), there is the maximum collection efficiency on account of inertial impaction and directional interception. When the particle diameter is larger than 5 μm, the collection efficiency steeply decreases at low liquid-to-gas ratios but increases again at high liquid-to-gas ratios. Given that the largest particle size corresponds to the lowest particle concentration in Fig. 4, only a high droplet concentration in tower can capture particles available in this size range.
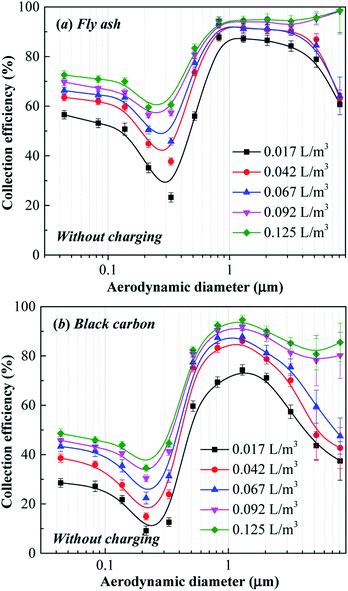 |
| Fig. 6 Collection efficiency of a wet scrubber for (a) fly ash, (b) black carbon. | |
As a contrast, the collection efficiency of black carbon is significantly lower than that of fly ash, particularly for particles smaller than 1 μm in aerodynamic diameter. On the one hand, according to the TEM results in Fig. 2, the fly ash particles are nearly spherical in shape while the black carbon particles are characterized by fluffy agglomerates. At the same size, agglomerates should be removed with higher collection efficiency than nearly spherical particles due to the stronger interception mechanism, particularly in the case of low particle concentration.34 Kim et al. stated that the larger interception length of agglomerates is responsible for the smaller penetration rate than spherical particles in the absence of an electric field.35 Even though the fibrous filter used in Kim's experiment is different from the wet scrubber in this study, the particle collection mechanisms are extremely similar. On this basis, Su et al. further confirmed that this effect is more significant for low particle concentrations.34 On the other hand, the hydrophilic angle of fly ash is close to 0°, while that of black carbon is approximately 43° from Fig. 3, which means fly ash has more hydrophilicity than black carbon. In general, the stronger the particle hydrophilicity is, the higher the collection efficiency of the conventional wet scrubber is. In this study, particle hydrophilicity is the dominant factor affecting the collection efficiency compared with particle morphology, so the collection efficiency of fly ash is higher as a whole.
3.2 Effect of droplet charging on efficiency increase
Ordinarily, the charge-to-mass ratio is used as the parameter to evaluate the performance of droplet charging, but it is difficult to acquire on large-scale industrial units due to the complexity of testing facilities. In order to estimate the effect of induction-charging on particle removal in the field studies, a simple self-defined equation is shown below: |
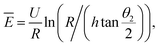 | (5) |
where Ē is the equivalent electric field strength, U is the high voltage applied on the ring electrode, R is the radius of the copper ring electrode, h is the vertical distance from the nozzle tip to the ring electrode and θ2 is the spray angle of the nozzle.
Fig. 7 shows a geometric model of a charging water spray. The charging performance mainly depends on the applied voltage and the distance from the nozzle tip to the ring electrode, if the nozzle type and ring material are determined.
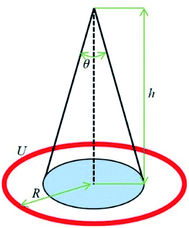 |
| Fig. 7 Geometric model of charging water spray. | |
According to Fig. 6, conventional wet scrubbing is not equipped with the ability to collect submicron particles with high efficiency, in contrast to micron particles. It is for this reason that only particles smaller than 1 μm were selected in this and follow-up sections to challenge the electrostatic scrubbing technique. In order to further underline the effect of electrostatic scrubbing, eqn (2) was adopted, and the corresponding results for both fly ash and black carbon are shown in Fig. 8(a) and (b), respectively. It could be clearly seen that droplet charging causes a more significant improvement to the collection efficiency of black carbon relative to that of fly ash particles. The average efficiency increase at 61.1 kV m−1 for submicron fly ash particles is approximately 27%, while it is close to 41% for black carbon. This phenomenon indicates that droplet charging plays a better role in capturing particles that cannot be removed efficiently by conventional wet scrubbers. Namely, the addition of electrostatic field to conventional wet scrubbers could weaken the adverse effects of particle hydrophobicity on the collection efficiency. In addition, in Fig. 8(b), the increasing rate of net collection efficiency with an equivalent electric field strength is slightly larger than that in Fig. 8(a), which is possibly caused by the greater number of image charges carried by conductive black carbon.
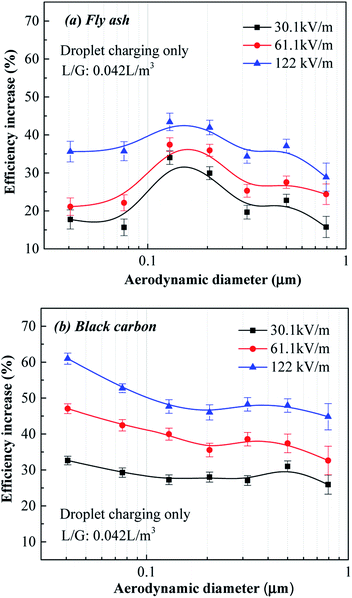 |
| Fig. 8 Efficiency increase under droplet charging only (a) fly ash, (b) black carbon. | |
3.3 Effect of particle charging on efficiency increase
Fig. 9(a) and (b) show the efficiency increase for fly ash and black carbon in the case of particle charging, respectively. In contrast to larger particles (0.1 μm to 1 μm), nanoparticles (<0.1 μm) have the lower efficiency increase for two types of particles, which is related to the number of negative ions attaching to the particles. In this study, it is in the classical corona field that particles acquire charges, so particle diameter has the crucial effects on the magnitude of charges acquired by particles. In general, the charges of a large particle are higher than those of a nanoparticle by several to dozens fold, resulting in a significantly stronger electrostatic force with droplets.
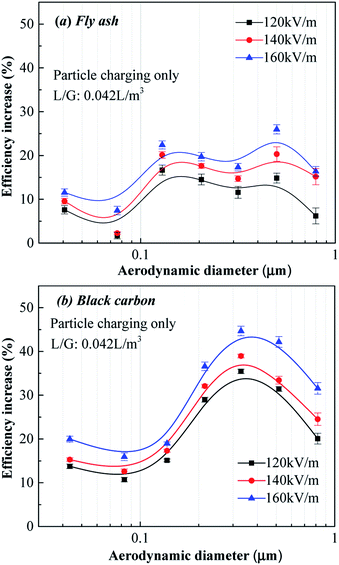 |
| Fig. 9 Efficiency increase under particle charging only (a) fly ash, (b) black carbon. | |
In contrast, corona charging provides more significant improvement to the collection efficiency of black carbon, particularly for larger particles (0.1 μm to 1 μm). This phenomenon could be explained reasonably by the greater electrostatic force between the charged black carbon and electrically neutral droplets. It is for this reason that a larger number of black carbon particles migrate to the droplet surface and subsequently settle with the droplets in the wet scrubber. It is noteworthy to mention that there is no external electric field in the wet tower, so the stronger electrostatic field is totally produced by charged black carbon, which acquires more charges in the corona charger upstream. According to the classical theory of the corona field, the particle charging mechanisms include diffusion charging and field charging.15 For diffusion charging, the particle morphology is an important factor that affects the number of gas ions carried by particles at the outlet of the corona charger.36 In this study, black carbon particles are characterized by loose agglomerates, while fly ash particles are mainly compact spheres. Extensive research has suggested that an agglomerate can acquire more gas ions than a spherical particle at the same mobility diameter due to the larger surface area and capacitance.37,38 With regard to the field charging mechanism, particle conductivity is another crucial factor to acquire gas ions in a corona charger except for particle morphology.39 Under the same conditions, black carbon with strong conductivity could obtain more gas ions than fly ash, which belongs to a kind of dielectric material. Based on the above two reasons, the mean charge of black carbon particles is higher than that of fly ash particles with the same diameter, which was also validated in Table 1.
3.4 Effect of opposite charging on efficiency increase
Fig. 10(a) and (b) show the efficiency increase of fly ash and black carbon in the cases of both particle and droplet charging, respectively. As a contrast, the efficiency increases for both fly ash and black are slightly higher than that in Fig. 8 and significantly greater than that in Fig. 9. This phenomenon indicates that droplet charging plays a more important role in removing PM1 (particulate matter smaller than 1 μm) than particle charging, which is due to the stronger electric field produced by a charged droplet than a charged particle. In comparison with the particle charges presented in Table 1, the droplet charges in Table 2 are higher by a few orders of magnitude. Therefore, it is pretty appropriate to apply the technique of droplet charging in conventional wet scrubbers. In addition, it is suitable to combine droplet charging with conventional dry ESPs by reforming the last stage, involving the multiple electric fields. In general, charged particles account for a large proportion of the total particles escaping from ESPs, which is a potential advantage for combining with charged droplets. In this manner, not only is the particle collection efficiency further improved but also the issue of dust reentrainment in ESPs can be eliminated. Above all, wet electrostatic scrubbing is proven to be an efficient method to increase the collection efficiency of black carbon, which has a great potential for application in industrial boilers.
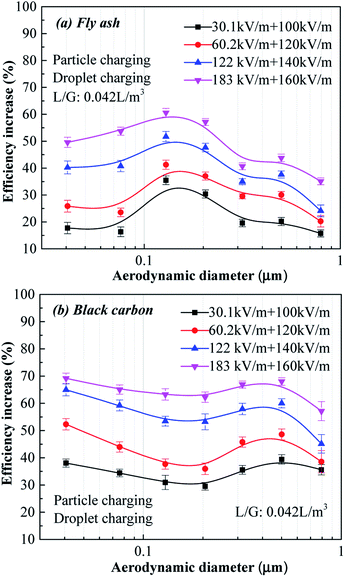 |
| Fig. 10 Efficiency increase under both particle and droplet charging (a) fly ash, (b) black carbon. | |
Fig. 11(a) and (b) show the morphologies of fly ash before and after scavenging, respectively. In the comparison, a portion of small-sized particles were deposited on the surfaces of the large particles after wet scavenging. A plausible reason for this phenomenon is that the airflow in the wet tower is heavy with moisture after scavenging, close to or even exceeding the saturation concentration of water vapour. Under this condition, the particles could absorb moisture from the wet airflow or the water vapour could condense onto the surface of ash particles and form a condensation nucleus, which is somewhat beneficial for particle agglomeration. In addition, the water-molecule concentration is probably not uniform over the whole space of the wet scrubber, which might enhance the migration capability of the small-sized particles and corresponding collision probability with large particles due to the concentration gradient effect. A more detailed theoretical model is required in the future to better expatiate this experimental evidence. For black carbon, the above-mentioned phenomenon was not found due to the characteristic of fractal agglomerates.
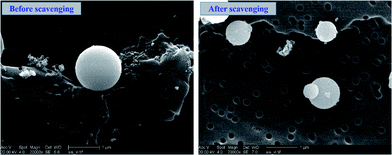 |
| Fig. 11 Morphologies of fly ash particles before and after scavenging. | |
4. Conclusions
In this study, a middle-scale setup was built to study the performance of the WES technique for removing both fly ash particles and black carbon, with special attention given to submicron particles. Major factors including particle properties and charging conditions were expatiated in detail, and the following key conclusions could be derived:
(1) For a conventional wet scrubber, the collection efficiency of black carbon is lower than that of fly ash, particularly for particles smaller than 1 μm in aerodynamic diameter. However, the efficiency increase in black carbon is significantly higher than that of fly ash due to the addition of the electrostatic field to the particles or/and droplets.
(2) Droplet charging plays a more important role in removing PM1 than particle charging due to the stronger electrostatic field produced by charged droplets, which indicates that water spray charging is appropriate for application with conventional wet scrubbers or in the last stage of conventional ESPs.
(3) After scavenging, more small particles could adhere to the surfaces of large fly ash particles, while this phenomenon was not found for black carbon due to the characteristic of fractal agglomerates. Further studies should focus on establishing a more accurate model to explain the scavenging process of particles with different properties.
Conflicts of interest
There are no conflicts to declare.
Acknowledgements
This work was financially supported by the General Programs of the National Natural Science Foundation of China (Grant No. 51676058).
References
- A. Jaworek, A. T. Sobczyk, A. Krupa, A. Marchewicz, T. Czech and L. Śliwiński, Hybrid electrostatic filtration systems for fly ash particles emission control, Sep. Purif. Technol., 2018, 213, 283–302 CrossRef.
- H. Sun, D. Feng, Y. Zhao, D. Guo, Y. Ma, H. Tan and S. Sun, Characteristics of Gas–Liquid–Solid Products in Corn Straw Gasification: Effect of the Char–Tar–H2O Interaction, Energy Fuels, 2019, 33, 9974–9984 CrossRef CAS.
- Z. Yang, C. Zheng, X. Zhang, Q. Chang, W. Weng, Y. Wang and X. Gao, Highly efficient removal of sulfuric acid aerosol by a combined wet electrostatic precipitator, RSC Adv., 2018, 8, 59–66 RSC.
- A. Jaworek, A. Marchewicz, A. T. Sobczyk, A. Krupa and T. Czech, Two-stage electrostatic precipitators for the reduction of PM2.5 particle emission, Prog. Energy Combust. Sci., 2018, 67, 206–233 CrossRef.
- Q. Du, L. Su, H. Dong, J. Gao, Z. Zhao, D. Lv and S. Wu, The experimental study of a water-saving wet electrostatic precipitator for removing fine particles, J. Electrost., 2016, 81, 42–47 CrossRef.
- L. Su, Q. Du, Y. Wang, H. Dong, J. Gao, M. Wang and P. Dong, Purification characteristics of fine particulate matter treated by a self-flushing wet electrostatic precipitator equipped with a flexible electrode, J. Air Waste Manage. Assoc., 2018, 68, 725–736 CrossRef CAS PubMed.
- M. E. Andersen, N. Modak, C. K. Winterrowd, C. W. Lee, W. L. Roberts, J. O. Wendt and W. P. Linak, Soot, organics, and ultrafine ash from air-and oxy-fired coal combustion, Proc. Combust. Inst., 2017, 36, 4029–4037 CrossRef CAS.
- O. Sippula, J. Hokkinen, H. Puustinen, P. Yli-Pirilä and J. Jokiniemi, Comparison of particle emissions from small heavy fuel oil and wood-fired boilers, Atmos. Environ., 2009, 43, 4855–4864 CrossRef CAS.
- G. Zhao, Z. Zhao, X. Guo, Q. Du, J. Gao, H. Dong, Y. Cao, Q. Han and L. Su, Emission and morphological characteristics and elemental compositions of fine particulate matter from an industrial pulverized coal boiler equipped with a fabric filter in China, Energy Fuels, 2016, 30, 4307–4317 CrossRef CAS.
- A. Jaworek, A. Marchewicz, A. T. Sobczyk, A. Krupa and T. Czech, Two-stage electrostatic precipitators for the reduction of PM2.5 particle emission, Prog. Energy Combust. Sci., 2018, 67, 206–233 CrossRef.
- B. A. Danzomo, M. E. Salami, S. Jibrin, M. R. Khan and I. M. Nor, Performance evaluation of wet scrubber system for industrial air pollution control, ARPN J. Eng. Appl. Sci., 2012, 7, 1669–1677 Search PubMed.
- Q. Zhang, Z. Wan, B. Hemmings and F. Abbasov, Reducing black carbon emissions from Arctic shipping: Solutions and policy implications, J. Cleaner Prod., 2019, 241, 118261 CrossRef CAS.
- H. T. Kim, C. H. Jung, S. N. Oh and K. W. Lee, Particle removal efficiency of gravitational wet scrubber considering diffusion, interception, and impaction, Environ. Eng. Sci., 2001, 18, 125–136 CrossRef CAS.
- B. K. Lee, B. R. Mohan, S. H. Byeon, K. S. Lim and E. P. Hong, Evaluating the performance of a turbulent wet scrubber for scrubbing particulate matter, J. Air Waste Manage. Assoc., 2013, 63, 499–506 CrossRef CAS PubMed.
- A. Jaworek, A. Krupa, A. T. Sobczyk, A. Marchewicz, M. Szudyga, T. Antes, W. Balachandran, F. D. Natale and C. Carotenuto, Submicron particles removal by charged
sprays. Fundamentals, J. Electrost., 2013, 71, 345–350 CrossRef CAS.
- C. Carotenuto, F. D. Natale and A. Lancia, Wet electrostatic scrubbers for the abatement of submicronic particulate, Chem. Eng. J., 2010, 165, 35–45 CrossRef CAS.
- F. D. Natale, C. Carotenuto, L. D'Addio, A. Jaworek, A. Krupa, M. Szudyga and A. Lancia, Capture of fine and ultrafine particles in a wet electrostatic scrubber, J. Environ. Chem. Eng., 2015, 3, 349–356 CrossRef.
- D. Y. H. Pui, S. C. Chen and Z. Zuo, PM2.5 in China: Measurements, sources, visibility and health effects, and mitigation, Particuology, 2014, 13, 1–26 CrossRef CAS.
- A. Jaworek, W. Balachandran, A. Krupa, J. Kulon and M. Lackowski, Wet electroscrubbers for state of the art gas cleaning, Environ. Sci. Technol., 2006, 40, 6197–6207 CrossRef PubMed.
- F. D. Natale, C. Carotenuto, L. D'Addio and A. Lancia, Effect of gas temperature on the capture of charged particles by oppositely charged water droplets, Aerosol Sci. Technol., 2016, 50, 110–117 CrossRef.
- H. Zhao and C. G. Zheng, Modeling of gravitational wet scrubbers with electrostatic enhancement, Chem. Eng. Technol., 2008, 31, 1824–1837 CrossRef CAS.
- E. A. Almuhanna, R. G. Maghirang, J. P. Murphy and L. E. Erickson, Laboratory scale electrostatically assisted wet scrubber for controlling dust in livestock buildings, Appl. Eng. Agric., 2009, 25, 745–750 Search PubMed.
- Z. Zuo, J. Wang, Y. Huo and R. Xu, Characteristics of particles deposited on a single free-fall charged droplet, Aerosol Sci. Technol., 2017, 51, 258–268 CrossRef CAS.
- Z. Zuo, J. Wang, Y. Huo and R. Xu, Numerical study of particle motion near a charged collector, Particuology, 2017, 32, 103–111 CrossRef CAS.
- Y. Ru, L. Zhao, L. J. S. Hadlocon, H. Zhu and S. K. Ramdon, Laboratory evaluation of electrostatic spray wet scrubber to control particulate matter emissions from poultry facilities, Environ. Technol., 2017, 38, 23–33 CrossRef CAS PubMed.
- W. Balachandran, A. Jaworek, A. Krupa, J. Kulon and M. Lackowski, Efficiency of smoke removal by charged water droplets, J. Electrost., 2003, 58, 209–220 CrossRef CAS.
- A. Jaworek, W. Balachandran, M. Lackowski, J. Kulon and A. Krupa, Multi-nozzle electrospray system for gas cleaning processes, J. Electrost., 2006, 4, 194–202 CrossRef.
- A. Jaworek, K. Adamiak, W. Balachandran, A. Krupa, P. Castle and W. Machowski, Numerical simulation of scavenging of small particles by charged droplets, Aerosol Sci. Technol., 2002, 36, 913–924 CrossRef CAS.
- T. H. Ha, O. Nishida, H. Fujita and H. Wataru, Simultaneous removal of NOx and fine diesel particulate matter (DPM) by electrostatic water spraying scrubber, J. Mar. Eng. Technol., 2009, 8, 45–53 Search PubMed.
- L. D'Addio, F. D. Natale, C. Carotenuto, W. Balachandran and A. Lancia, A lab-scale system to study submicron particles removal in wet electrostatic scrubbers, Chem. Eng. Sci., 2013, 97, 176–185 CrossRef.
- L. D'Addio, C. Carotenuto, W. Balachandran, A. Lancia and F. D. Natale, Experimental analysis on the capture of submicron particles (PM0.5) by wet electrostatic scrubbing, Chem. Eng. Sci., 2014, 106, 222–230 CrossRef.
- F. D. Natale, L. Manna, F. La Motta, R. Colicchio, E. Scaglione, C. Pagliuca and P. Salvatore, Capture of bacterial bioaerosol with a wet electrostatic scrubber, J. Electrost., 2018, 93, 58–68 CrossRef.
- Y. Wang, X. Li, M. Zhang, D. Xu, L. Wang, D. Lu, T. Wang and B. Li, Distribution characteristics of particle size of tobacco casing atomized by two-fluid nozzle, Tob. Sci. Technol., 2018, 51, 78–86 CrossRef.
- L. Su, Q. Du, M. Tang, Q. Ou and D. Y. H. Pui, Effects of Particle Hydrophilicity and Morphology on Aerosol Scavenging in a Wet Electrostatic Scrubber, Aerosol Air Qual. Res., 2019, 19, 2331–2340 CrossRef.
- S. C. Kim, J. Wang, M. S. Emery, W. G. Shin, G. W. Mulholland and D. Y. H. Pui, Structural property effect of nanoparticle agglomerates on particle penetration through fibrous filter, Aerosol Sci. Technol., 2009, 43, 344–355 CrossRef CAS.
- W. G. Shin, J. Wang, M. Mertler, B. Sachweh, H. Fissan and D. Y. H. Pui, The effect of particle morphology on unipolar diffusion charging of nanoparticle agglomerates in the transition regime, J. Aerosol Sci., 2010, 41, 975–986 CrossRef CAS.
- L. N. Cao, S. C. Chen, H. Fissan, C. Asbach and D. Y. H. Pui, Development of a geometric surface area monitor (GSAM) for aerosol nanoparticles, J. Aerosol Sci., 2017, 114, 118–129 CrossRef CAS.
- L. Su, Q. Ou, L. N. Cao, Q. Du and D. Y. H. Pui, Real-time measurement of nano-agglomerate and aggregate mass and surface area concentrations with a prototype instrument, Aerosol Sci. Technol., 2019, 53, 1453–1467 CrossRef CAS.
- Z. Long and Q. Yao, Evaluation of various particle charging models for simulating particle dynamics in electrostatic precipitators, J. Aerosol Sci., 2010, 41, 702–718 CrossRef CAS.
|
This journal is © The Royal Society of Chemistry 2020 |
Click here to see how this site uses Cookies. View our privacy policy here.