DOI:
10.1039/C9RA09790B
(Paper)
RSC Adv., 2020,
10, 2846-2855
In vivo toxicity evaluation of two polyoxotungstates with potential antidiabetic activity using Wistar rats as a model system
Received
22nd November 2019
, Accepted 2nd January 2020
First published on 15th January 2020
Abstract
In this study, the in vivo hypoglycemic effect of a donut-shaped polyanion salt (NH4)14[Na@P5W30O110]·31H2O {NaP5W30} and its Ag-containing derivative K14[Ag@P5W30O110]·22H2O·6KCl {AgP5W30}, as well as their hepatotoxicity and nephrotoxicity, was evaluated. In the screening hypoglycemic study, Wistar albino rats with streptozotocin induced diabetes were treated intraperitoneally with three single doses (5, 10, and 20 mg per kg per b.w.) of both investigated polyoxotungstates. The blood glucose levels, measured before and after 2, 4 and 6 h polyoxotungstate application, showed that both studied compounds induced the most pronounced and time dependent glucose lowering effects at the doses of 20 mg kg−1. Thus, daily doses of 20 mg kg−1 were administered to Wistar albino rats orally for 14 days in further toxicity examinations. The serum glucose concentration and biochemical parameters of kidney and liver function, as well as a histopathological analysis of kidney and liver tissues were evaluated 14 days after the polyoxotungstate administration. Both investigated compounds did not induce statistically significant alterations of the serum glucose and uric acid concentrations, as well as some of the liver function markers (serum alanine and aspartate aminotransferases, and alkaline phosphatase activities). However, the significant decrease in serum total protein and albumin concentrations and the increase in biochemical parameters of renal function – serum urea (up to 63.1%) and creatinine concentrations (up to 23.3%) were observed for both polyoxotungstates. In addition, the detected biochemical changes were in accordance with kidney and liver histhopathological analysis. Accordingly, the hepatotoxic and nephrotoxic effects of these potential antidiabetic polyoxotungstates could be considered as mild.
Introduction
Diabetes mellitus (DM) is the most common chronic metabolic disease in humans. The hallmark of metabolic abnormality associated with DM is the hyperglycemia either due to the defective secretion of insulin or the insulin insensitivity or both.1 DM is classified into two main types: type 1 or immune-mediated diabetes (previously referred to as insulin-dependent or juvenile-onset diabetes) and type 2 (previously called insulin-independent or adult-onset diabetes). Immune-mediated diabetes represents approximately 5–10% of all cases of diabetes, and is characterized by the autoimmune destruction of the β cells in the endocrine pancreas, that usually results in the absolute insulin deficiency. On the other hand, type 2 accounts for about 90–95% of all diabetes cases, in which there is a progressive defect in insulin secretion on the background of insulin resistance.2 Both forms of DM may lead to macrovascular (myocardial infarction, stroke and limb amputation) and microvascular (retinopathy, nephropathy, and neuropathy) complications, and early mortality.3 Lifestyle-directed interventions, insulin, sulfonylureas, metformin, dipeptidyl peptidase-4 (DPP-4) inhibitors, sodium glucose transporter 2 inhibitors and glucagon-like peptide-1 (GLP-1) receptor agonist are used in the treatment of DM by decreasing the level of glucose in the blood.4,5 The actual therapies are effective, but often restricted because of their ways of application, side effects, loss of the efficacy after long-term use, cost and unavailability in rural areas where they are mostly needed.6 Thus, the development of new potent and comfortable classes of blood glucose-lowering medications with minimal side effects to supplement actual therapeutics is urgently needed. Accordingly, in vivo studies7–9 revealed that some polyoxometalates (POMs) induced normoglycemic effects in diabetic animal models. Therefore the investigation of POM normoglycemic properties might be a promising research direction resulting in the development of comfortable and low-cost POM-based antidiabetic drugs.
POMs are discrete, negatively-charged metal-oxo clusters comprising early d-block metal ions in high oxidation states (e.g. W6+, V5+). Earlier studies showed that these inorganic drug candidates possess potential anti-viral,10–12 -bacterial,13,14 -protozoal,15 -tumor,16,17 -Alzheimer18 and -diabetic activities.8,9 However, their molecular modes of action are poorly understood. The approved selective and potent inhibition of enzymes located on the cell surface, such as cholinesterases,19 phosphatases,15,20 ecto-nucleotidases21,22 and kinases,23 is supposed to be a putative mechanism of the POM biological activities.
The aim of this study was to evaluate in vivo hypoglycemic effects of the donut-shaped polyanion salt (NH4)14[Na@P5W30O110]·31H2O {NaP5W30} and its Ag-containing derivative K14[Ag@P5W30O110]·22H2O·6KCl {AgP5W30} using Wistar albino rats with streptozotocin (STZ)-induced diabetes as an experimental model. Furthermore, as these POMs demonstrated promising hypoglycemic action, their hepatotoxicity and nephrotoxicity were evaluated by monitoring some biochemical and histopathological parameters before further studies regarding mechanism of the antidiabetic action.
Material and methods
Chemicals
All reagents and chemicals employed for the POM synthesis were of high-purity grade and used as purchased without further purification. STZ was purchased from Sigma-Aldrich (Munich, Germany).
Synthesis of polyoxotungstates
{NaP5W30} and {AgP5W30} were synthesized according to the previously published procedures.24
Experimental animals
Male Wistar albino rats 6 weeks old and weighing 250–350 g bred in the animal house of Military Medical Academy Research Facility (Belgrade, Serbia) were used for this experiment. This study was carried out in strict accordance with the Animal Welfare Act of the Republic of Serbia (Official Gazette of the Republic of Serbia no. 41/09), Directive 2010/63 EU on the Protection of Animals Used for Scientific Purposes, and the Guide for the Care and Use of Laboratory Animals (National Research Council, 8th ed. Washington, USA). The methodology used in our investigation was approved by the Ethical Commission for the Welfare Protection of Experimental Animals (Faculty of Medicine, University of Belgrade, Serbia). The animals were kept in the clean Plexiglas cages (48 × 37.5 × 21 cm), and were maintained at the local animal house conditions of temperature 23 ± 2 °C, humidity 65 ± 5%, and 12 h light/dark cycle with free access to food and water. After randomization into various groups, the rats were acclimatized to the laboratory conditions of temperature and photoperiod for a period of 1–2 weeks before initiation of the experiments. The weight of the rats was measured before the start of the experiment, and was followed up each day through the course of the experiment.
In vivo screening of hypoglycemic effect induced by polyoxotungstates
Induction of diabetes. DM was induced by a single intraperitoneal injection of freshly prepared STZ, single dose of 50 mg per kg per body weight (b.w.).25 Diabetes in STZ-rats was confirmed by measuring the fasting blood glucose level, 96 h after the STZ injection. Rats with blood glucose levels >12 mmol L−1 were considered as diabetic, and separated for the study. The glucose levels from the tail-vein blood samples were followed by GlucoSure Autocode Blood Glucose Monitoring System (Houston, Texas, USA).
Experimental design. One week after the diabetes induction, when hyperglycemia was stabilized, the rats were divided into different groups (n = 5 per group). Three different single doses (5, 10, and 20 mg per kg per b.w.) of the both investigated polyoxotungstates were administered intraperitoneally (ip). Blood glucose levels were determined before and after 2, 4 and 6 h of polyoxotungstate application. The dose of 20 mg per kg per b.w. showed maximum hypoglycemic effect, and thus was selected for further toxicity examinations.
Toxicity evaluation of {NaP5W30} and {AgP5W30}
Experimental design. The rats were distributed into three groups (n = 7 per group):• Control, 0.9% NaCl
• {NaP5W30}, 20 mg kg−1
• {AgP5W30}, 20 mg kg−1
The animals in the Control group received saline (0.9% NaCl) per os for 14 days. The animals in the groups {NaP5W30} and {AgP5W30} received the investigated POMs per os, in the daily doses of 20 mg per kg per b.w. for 14 days. All experimental animals were followed for 14 days when the rats were sacrificed, and the blood samples were collected via cardiac puncture. The kidney and liver samples of each animal were taken post mortem, for a histological evaluation. Since the chosen polyoxotungstates had never been in vivo tested before, the selection of exposure periods for monitoring of parameters was made according to recommended protocol (OECD Guidelines for the Testing of Chemicals, Acute Oral Toxicity – Fixed Dose Procedure).
Determination of biochemical parameters. The samples of collected blood were centrifuged, and biochemical markers of kidney (serum concentrations of urea – SUr, creatinine – SCr and uric acid – SUA) and liver function (serum activities of aspartate aminotransferase – AST, alanine aminotransferase – ALT, and alkaline phosphatase – ALP, as well as serum total protein – TP, and albumin – Alb concentrations), and glucose concentrations – SGl were determined in the serum samples spectrophotometrically. The results were expressed as median (range). One Way ANOVA followed by Tukey's Multiple Range Test (GraphPad Prism 5, La Jolla California, USA) was used to determine the statistical significance of difference among the groups.
Histological evaluation
Tissues preparation. All tissue samples were fixed in 4% buffered formaldehyde for light microscopy, and in 3% glutaraldehyde for electron microscopy.
Light microscopy. The tissue samples fixed in formaldehyde were dehydrated and embedded in paraffin. 5 μm thick paraffin sections were stained with Hematoxylin and Eosin (HE). Micrographs were taken under the light microscope (Leica DMLS, Hicksville, New York, USA) by a digital camera (Leica DFC295, Hicksville, New York, USA).
Transmission electron microscopy. Tissue samples fixed with 3% glutaraldehyde in cacodylate buffer were postfixed in 1% OsO4. After dehydration in graded alcohols, the cells were embedded in Epoxy medium (Sigma-Aldrich, 45345). Thin sections were mounted on copper grids (Sigma-Aldrich, G4901), and stained with uranyl acetate and lead citrate for the examination on an electron microscope (Morgagni 268D, FEI, Hillsboro, OR).
Results
Influence of {NaP5W30} on the blood glucose levels in STZ-induced diabetic rats
The blood glucose level measured in STZ-induced diabetic rats before and after 2, 4 and 6 h {NaP5W30} ip application in three different single doses (5, 10, and 20 mg per kg per b.w.) are expressed as mean ± S.E.M (Fig. 1A). The repeated measures ANOVA test (GraphPad Prism 5, La Jolla California, USA) was used for the determination of the statistical significance. A time- (Fig. 1A) and dose- (Fig. 1B) dependent hypoglycemic effect was observed. The investigated polyoxotungstate (5 mg per kg per b.w) significantly reduced (P < 0.05) the blood glucose level (in mmol L−1) after 2 h, 4 h, and 6 h administration (22.94 ± 1.28, 21.51 ± 1.31, and 20.30 ± 0.86, respectively) compared to the pretreatment diabetic control value (28.06 ± 1.20). Similarly, 10 mg per kg per b.w. of {NaP5W30} significantly reduced (P < 0.05) the blood glucose level 2 h after the administration, and the hypoglycemic effect was sustained over a 2 to 6 hour period. 20 mg kg−1 of {NaP5W30} showed the maximum hypoglycemic effect. A significant decrease (P < 0.05) in the blood glucose level after 2, 4 and 6 h application of the investigated compounds (24.22 ± 1.16 mmol L−1, 21.36 ± 1.30 mmol L−1, and 18.40 ± 1.02 mmol L−1, respectively) in respect to the pretreatment diabetic control value (29.62 ± 1.24 mmol L−1) was obtained (Fig. 1A).
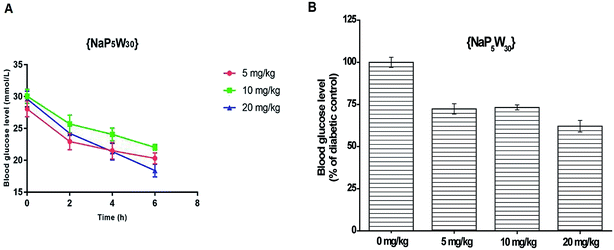 |
| Fig. 1 The effect of {NaP5W30} on the blood glucose level in STZ-diabetic rats. The investigated compound was administered intraperitoneally (t = 0 h) in three different single doses (5, 10, and 20 mg per kg per b.w.). (A) The blood glucose level was determined 2, 4, and 6 hours after the POM application. The values are expressed as mean ± S.E.M. The repeated measures ANOVA test was used to determine the statistical significance. The blood glucose levels after 2 h, 4 h, and 6 h of {NaP5W30} administration were significantly reduced (P < 0.05) in respect to the corresponding pretreatment diabetic control values for all administrated doses (5, 10, and 20 mg kg−1). (B) The blood glucose level (expressed as % of diabetic control) at three {NaP5W30} doses 6 hours after the treatment. | |
Influence of {AgP5W30} on the blood glucose levels in STZ-induced diabetic rats
The effect of {AgP5W30} on the blood glucose level in STZ-induced diabetic rats after 2, 4 and 6 h ip administration in three different single doses (5, 10, and 20 mg per kg per b.w.) was also examined. The results (expressed as mean ± S.E.M) showed that 5 mg per kg per b.w. and 10 mg per kg per b.w. of {AgP5W30} did not have a significant effect (P > 0.05) on the blood glucose level in STZ-induced diabetic rats (Fig. 2). On the other hand, 20 mg per kg per b.w of the investigated polyoxotungstate significantly reduced (P < 0.05) the blood glucose level (in mmol L−1) from 24.08 ± 4.41 to 21.54 ± 4.36, 17.06 ± 3.74, and 14.60 ± 3.54, 2 h, 4 h and 6 h, respectively, after the administration (Fig. 2). The repeated measures ANOVA test (GraphPad Prism 5, La Jolla California, USA) determined the statistical significance of difference between the groups.
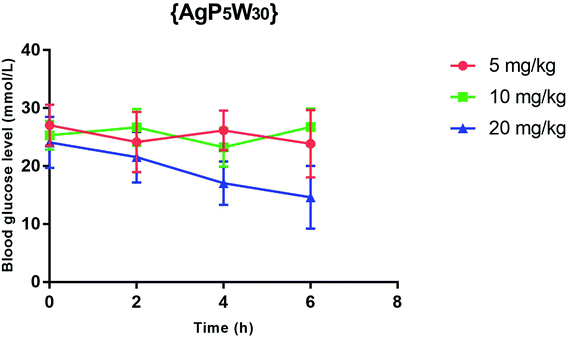 |
| Fig. 2 The effect of {AgP5W30} on the blood glucose level in STZ-diabetic rats. The investigated polyoxotungstate was administered intraperitoneally (t = 0 h) in three different single doses (5, 10, and 20 mg per kg per b.w.). The blood glucose level determined 2, 4, and 6 hours after the POM application. The values are expressed as mean ± S.E.M. The repeated measures ANOVA test was used to determine the statistical significance of difference among groups. | |
Toxicity evaluation of {NaP5W30} and {AgP5W30}
General habit of animals. The rats were observed twice daily (morning and afternoon). Sleeping and feeding monitoring, as well as the inspection of skin and visible mucosa was followed through the whole experiment period according to ref. 26. During the two-week follow-up, the rats did not show any significant differences in behaviour regarding sleeping and feeding. Oral administration of POMs for two weeks did not exert any effect on growth rate compared with untreated animals. All changes in body weight were in line with the timeframe of monitoring. Additionally, any changes in appearances of the skin and mucosa of the animals were not observed in the follow-up period.
Biochemical parameters. Fig. 3 shows the effect of {NaP5W30} and {AgP5W30} on SGl in normal rats. The changes in the serum glucose concentration were within the physiological limits, and no a statistical difference (P > 0.05) was observed between the untreated and treated animals.
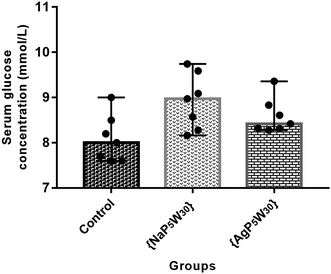 |
| Fig. 3 The effects of {NaP5W30} (20 mg per kg per day per os) and {AgP5W30} (20 mg per kg per day per os) on serum glucose concentrations. In Control group, the rats received saline (0.9% NaCl) per os for 14 days. Blood samples were taken after 14 days POM (or saline) application. The results are presented as median (range) values. One Way ANOWA followed by Tukey's Multiple Range Test was used to determine the statistical significance of difference among groups. | |
To ascertain whether POMs induced renal damage, SUr, SCr and SUA levels were measured in the rat serums. The effects of both investigated compounds on these parameters are shown in Fig. 4. The results demonstrated that {NaP5W30} and {AgP5W30} induced statistically significant increase of SUr level (in mmol L−1) [7.65 (7.13–8.87)] and [6.71 (6.30–7.07)] vs. [4.69 (4.05–5.76); P < 0.001 and P < 0.05, respectively] (Fig. 4A), and SCr level (in mmol L−1) [40.7 (39.5–43.8)] and [39.5 (38.9–40.1)] vs. [33.00 (31.7–33.7); P < 0.001 and P < 0.001, respectively] (Fig. 4B) compared with the Control group. On the contrary, SUA level was within the physiological limits and no statistical difference (P > 0.05) was observed between the untreated and treated animals (Fig. 4C).
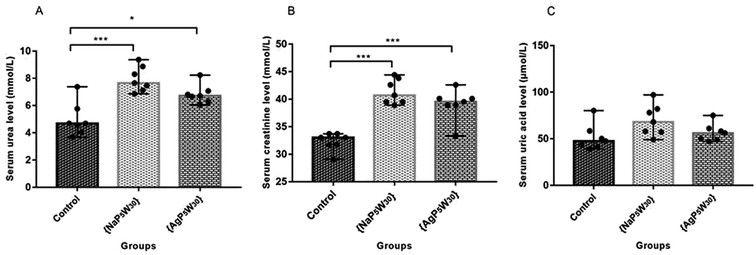 |
| Fig. 4 The effects of {NaP5W30} (20 mg per kg per day per os) and {AgP5W30} (20 mg per kg per day per os) on renal function parameters (A, B and C: serum urea, creatinine and uric acid concentration, respectively). In Control group, the rats received saline (0.9% NaCl) per os for 14 days. Blood samples were taken after 14 days POM (or saline) application. The results are presented as median (range) values. One Way ANOVA followed by Tukey's Multiple Range Test was used to determine the statistical significance of difference among groups.*P < 0.05; ***P < 0.001. | |
The hepatotoxic effects of the studied POMs were evaluated through the liver enzyme activity (AST, ALT, and ALP), as well as TP and Alb concentration in the rat serums. Both investigated compounds did not induce a statistically significant difference (P > 0.05) in AST, ALT, and ALP activities between the untreated and treated rats (Fig. 5A–C). However, both {NaP5W30} and {AgP5W30} induced statistically significant decrease in TP concentration (in g L−1) [59.60 (57.30–61.40)] and [58.20 (57.20–59.60)] vs. [68.90 (64.50–69.90); P < 0.001 and P < 0.001, respectively], and Alb concentration (in g L−1): [28.90 (28.30–30.50)] and [28.90 (28.40–30.30)] vs. [35.10 (34.00–35.90); P < 0.001 and P < 0.001, respectively], compared with the Control group (Fig. 5D and E).
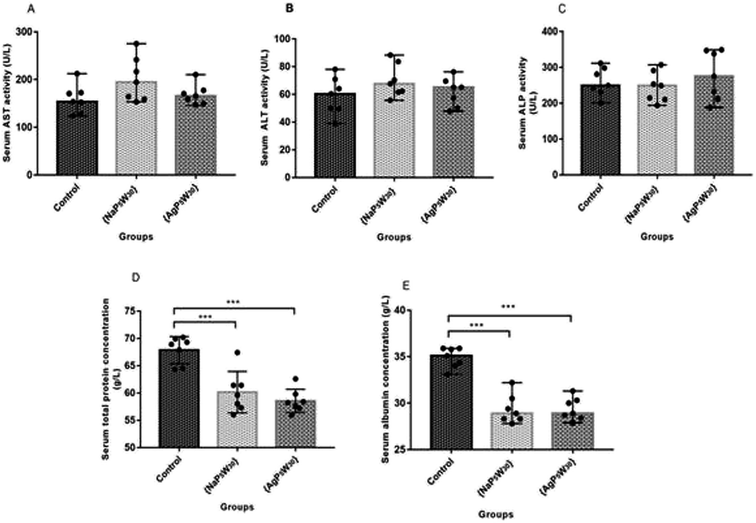 |
| Fig. 5 The effects of {NaP5W30} (20 mg per kg per day per os) and {AgP5W30} (20 mg per kg per day per os) on liver function parameters (A, B, C, D and E: AST, ALT and ALP activities, and total protein and albumin concentrations in serum, respectively). In Control group, the rats received saline (0.9% NaCl) per os for 14 days. Blood samples were taken after 14 days POM (or saline) application. The results are presented as median (range) values. One Way ANOVA followed by Tukey's Multiple Range Test was used to determine the statistical significance of difference among groups. *P < 0.05; ***P < 0.001. | |
Histopathological evaluation of POM-induced renal toxicity
Light microscopy. The photomicrographs of the HE-stained kidney tissues excised from the treated and untreated rats are shown in Fig. 6. The sections obtained from the animals orally treated with both {NaP5W30} and {AgP5W30} (20 mg per kg per day, 14 days) were similar to the normal rats; showing the kidney section with normal histology of glomeruli, tubules, interstitium and blood vessels.
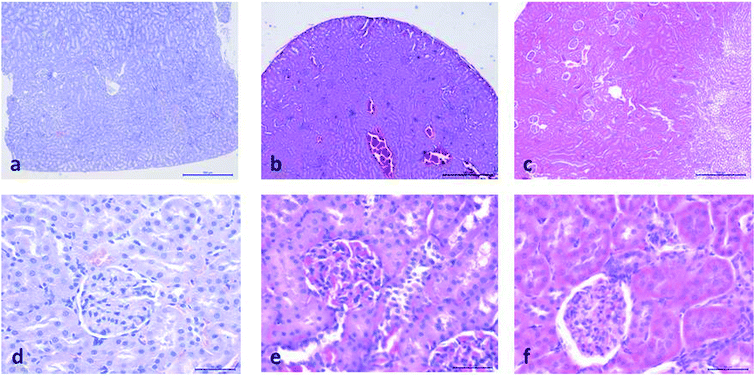 |
| Fig. 6 Photomicrographs of HE-stained rat kidney sections. Tissues were stained with haematoxylin and eosin, and image was captured under light microscope with ×5 (a–c) and ×40 (d–f) magnifications; (a and d) = tissues from control rats treated with saline; (b and e) = tissues from rats treated with 20 mg per kg per day {NaP5W30}; (c and f) are tissues from 20 mg per kg per day {AgP5W30}-treated rats. No difference between the kidney tissues from the treated and control rats. | |
Transmission electron microscopy (TEM). The tubules in the kidney samples obtained from the animals orally treated with both {NaP5W30} and {AgP5W30} (20 mg per kg per day, 14 days) altered the mitochondria that appeared dark and oedematous (Fig. 7b and c), while the glomeruli were not altered.
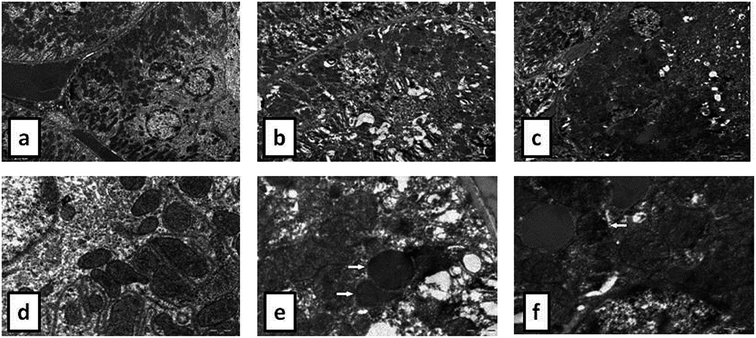 |
| Fig. 7 TEM micrographs presenting the ultrastructure of tubules in examined kidneys in untreated rat (a and d), in rat treated with 20 mg per kg per day {NaP5W30} (b and e) and in rat treated with 20 mg per kg per day {AgP5W30} (c and f). Low power magnification (×2800) of tubules: unaltered tubules with prominent basal labyrinth in control rat (a), tubules with disorganized basal labyrinth and condensation of mitochondrial matrix in {NaP5W30} (b) and {AgP5W30} (c) treated rats. High power magnification (×14 000) of: the cytoplasm of epithelial cell in tubules with unaltered mitochondria in kidney of control rat (d), cytoplasm of tubular epithelial cell in kidney tubules with electron dense mitochondria (arrow) in {NaP5W30} treated animal (e), and cytoplasm of epithelial cells of kidney tubules with distorted mitochondria (arrow) in {AgP5W30} treated animal (f). | |
Histopathological evaluation of POM-induced liver toxicity
Light microscopy. The discrete fields of focal necrosis between the lobules were observed in liver tissue samples obtained from the animals orally treated with {NaP5W30} (20 mg per kg per day, 14 days) (Fig. 8b and c). Additionally, liver section from the {AgP5W30}-treated rats also showed focal necrosis in periportal regions (Fig. 8e and f).
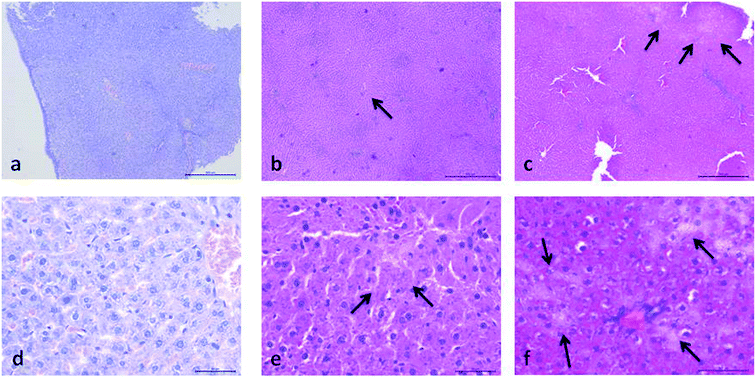 |
| Fig. 8 Photomicrographs of HE-stained rat liver sections. Tissues were stained with haematoxylin and eosin, and image was captured under light microscope with ×5 (a–c) and ×40 (d–f) magnifications; (a and d) = tissues from control rats treated with saline; (b and e) = tissues from rats treated with 20 mg per kg per day {NaP5W30} showing: discrete fields of focal nescrosis between lobules (arrows); (c and f) are tissues from 20 mg per kg per day {AgP5W30}-treated rats showing: focal necrosis in periportal regions (arrows). | |
Transmission electron microscopy. The hepatocytes in the liver samples obtained from the animals orally treated with {NaP5W30} (20 mg per kg per day, 14 days) exhibited the perilobular discrete fields of the focal necrosis accompanied with the extravasated erythrocytes (Fig. 9b). The liver samples of the animals treated with {AgP5W30} had more extensive fields of the focal necrosis (Fig. 9c).
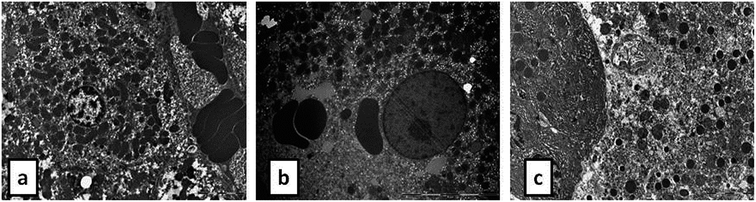 |
| Fig. 9 TEM micrographs presenting the ultrastructure of liver (×3500) in untreated rat (a), in rat treated with 20 mg per kg per day {NaP5W30} (b), and in rat treated with 20 mg per kg per day {AgP5W30} (c). Ultrastructural features of unaltered hepatocytes in control rat (a). Liver tissue with field of necrosis accompanied with extravasated erythrocytes in rat treated with {NaP5W30} (b). Small fields of coagulation necrosis in {AgP5W30} treated rat (c). | |
Discussion
In this study hypoglycemic potential of a donut-shaped polyoxotungstate {NaP5W30} and its Ag+-containing derivative {AgP5W30} was verified for the first time using Wistar albino rats with STZ-induced diabetes as a model system. Both studied compounds induced the most pronounced and time dependent glucose lowering effects at doses of 20 mg kg−1 resulting in the similar decrease of the glucose level (approximately 40% compared to the untreated rats, 6 h after the application). The obtained hypoglycemic effect of the investigated polyoxotungstates is in agreement with previously published in vivo studies that reported effective hypoglycemic actions of various types of polyoxotungstates on different diabetic animal models.7–9 According to literature, a few putative mechanisms of POM induced normoglycemic action were indicated: α-glucosidase activity inhibition that consequently results in the reduced absorption of intestinal carbohydrates,8 insulin mimetic effects including glucose uptake stimulation and lipolysis inhibition,27 prevention of STZ-induced apoptosis of pancreatic β-cells, and stimulation of insulin synthesis.7 Moreover, some polyoxotungstates were found to prevent the development of diabetic life-threatening microvascular and macrovascular complications by significant limitation of aldose reductase activities and accumulation of advanced glycation end products.8
The preference in the investigation of agents as potential drugs for the treatment of different disorders is the assessment of their safety. Although the evidence about the biological activity of some POMs was reported for the first time almost fifty years ago, there is a relative lack of relevant data related to toxic properties of POMs. Therefore, the present study was designed to examine the in vivo nephrotoxic and hepatotoxic effects of two structurally similar polyoxotungstates with above described hypoglycemic activity.
The results of the acute 14 day oral toxicity study of the investigated polyoxotungstates showed no adverse effects on the animal behaviour regarding sleeping and feeding. Statistically significant differences in food and water intake and body mass between the treated and control rats were not observed. Since changes in body mass is a sensitive marker of the general health of the animals,28 it could be concluded that both {NaP5W30} and {AgP5W30} in the oral dose of 20 mg per kg per day did not induce severe toxic effects in the rats during the two-week follow-up period of the experiment (Table 1). These findings are in agreement with previously published similar effects obtained for the animals (rats and mice) treated with various other polyoxotungstates.7,9,19
Table 1 The effect of the investigated POMs on the body weight
Time point (days) |
Body weight in grams (mean ± S.E.M) |
Control (n = 7) |
{NaP5W30} (n = 7) |
{AgP5W30} (n = 7) |
0 |
258.00 ± 16.85 |
254.50 ± 16.47 |
255.40 ± 5.10 |
2 |
268.10 ± 16.81 |
270.60 ± 17.80 |
256.40 ± 6.02 |
4 |
281.20 ± 16.92 |
282.10 ± 18.22 |
259.89 ± 6.58 |
6 |
280.60 ± 14.64 |
281.00 ± 16.25 |
254.40 ± 6.82 |
8 |
272.60 ± 12.97 |
286.00 ± 14.37 |
257.20 ± 6.83 |
10 |
290.60 ± 17.51 |
290.60 ± 17.51 |
290.61 ± 17.51 |
12 |
293.20 ± 16.77 |
300.40 ± 15.66 |
297.90 ± 6.65 |
14 |
290.10 ± 13.31 |
301.77 ± 16.11 |
296.10 ± 9.93 |
In addition, both studied polyoxotungstates (20 mg per kg per day, 14 days) did not affect the serum glucose concentration in the healthy rats (Fig. 3). Accordingly, our findings and the results of ref. 9 and 8 suggested that polyoxotungstates administration within the dose tested in these studies may not lead to hypoglycemia, which has been recognized as a side effect of some currently available antidiabetic agents.29
During the development of a new drug, it is necessary to examine the nephrotoxic properties of potential pharmaceutical agents, because the kidney constitutes the major excretory organ for most substances. Thus, the combination of functional and histological parameters was evaluated for the purpose of detecting nephrotoxic properties of the studied POMs. The results of renal functional parameters (Fig. 4) showed that the administration of both investigated POMs (20 mg per kg per day, 14 days) induced statistically significant increase of SUr and SCr levels. On the other hand, SUA level was within the physiological limits and no statistical difference was observed between the untreated and treated animals. These nephrotoxic effects were confirmed by TEM morphological studies of excised kidneys that also showed mild renal damage (Fig. 7), although light microscopy did not indicate differences between samples taken from the POM treated and control rats (Fig. 6). The morphological studies by TEM (Fig. 7) showed dark and oedematous mitochondria in tubules of kidney samples obtained from animals treated with both investigated compounds. These nephrotoxic findings for the polyoxotungstates could be explained by the reported highest accumulation of the anti-viral polyoxotungstate in the kidneys after the oral application,30 but mechanisms of POM-induced renal toxicity are still unknown. In addition, the morphological changes of mitochondria indicated that the one of putative nephrotoxic mechanisms of POMs is oxidative stress increase in the kidney.
The biochemical and histopathological analysis were performed to evaluate the possible alterations in liver function and morphology induced by {NaP5W30} and {AgP5W30}. The results of liver function indicators demonstrated that the administration of the investigated clusters did not induce statistically significant changes in serum AST, ALT, and ALP activities (Fig. 5A–C). On the contrary, a statistically significant decrease in serum concentration of TP and Alb was detected for both polyoxotungstates (Fig. 5D and E). The aminotransferase activities in serum are elevated with acute hepatic cell injury, while elevated serum ALP activities are associated with cholestasis.31 Besides, TP and Alb concentrations are monitored in basic liver function tests as the indicators of hepatic synthetic function. Accordingly, the obtained results of hepatotoxicity evaluation indicated that both investigated compounds (20 mg per kg per day, 14 days) induced neither massive necrosis of hepatocytes nor cholestasis; however, synthetic dysfunction of liver was detected after both POMs administration. Moreover, the results of the biochemical analysis were confirmed by histological examination of excised liver from the POM-treated and the control rats. The results of histological analysis (Fig. 8 and 9) revealed discrete fields of focal necrosis between lobules accompanied with extravasated erythrocytes in {NaP5W30}-treated rats, while {AgP5W30} induced more extensive fields of focal necrosis in periportal regions. According to these results, both investigated POMs with potential antidiabetic properties only affected synthetic function of liver without massive and severe damage in the applied dose.
Conclusions
It could be concluded that both investigated polyoxotungstates demonstrated similar hypoglycemic effects in rats with STZ-induced diabetes. Additionally, hepatotoxic and nephrotoxic effects induced by 2 week POM treatments could be considered as mild. The obtained results imply it is noteworthy to carry out further studies, involving a mode of action as well as detailed toxicological examinations, on these POM-based potential antidiabetic agents. Moreover, this study presents a good platform for design of novel low-cost inorganic antidiabetics with more advanced characteristics.
Author contributions
M. D., M. Č., J. T., and D. K. designed and coordinated the experiments, and wrote the paper. A. M. and U. K. synthesized POMs, and wrote the paper. M. Ć., T. K. S., and M. M. carried out histopathological experiments. M. S. M., S. I., and B. R. carried out biochemical experiments. All authors contributed to the interpretation of the results.
Conflicts of interest
The authors declare that there are no conflicts of interest.
Acknowledgements
This work was supported by the bilateral project Serbia–Germany (no. 451-03-01038/2015-09/16, DAAD-PPP), and the Ministry of Education, Science and Technological Development of the Republic of Serbia (projects no. 172023, 175036, and S35). U. K. thanks the German Research Council (DFG) (KO-2288/20-1 and KO-2288/16-1) and Jacobs University for support. We also gratefully acknowledge the CMST COST Action CM1203 (PoCheMoN).
References
- Z. Punthakee, R. Goldenberg, P. Katz and D. C. C. P. G. E. Committee, Definition, classification and diagnosis of diabetes, prediabetes and metabolic syndrome, Can. J. Diabetes, 2018, 42, S10–S15 CrossRef PubMed.
- A. D. Association, Diagnosis and classification of diabetes mellitus, Diabetes Care, 2014, 37, S81–S90 CrossRef PubMed.
- M. C. Thomas, M. E. Cooper and P. Zimmet, Changing epidemiology of type 2 diabetes mellitus and associated chronic kidney disease, Nat. Rev. Nephrol., 2016, 12, 73–81 CrossRef CAS PubMed.
- M. J. Davies, D. A. D'Alessio, J. Fradkin, W. N. Kernan, C. Mathieu, G. Mingrone, P. Rossing, A. Tsapas, D. J. Wexler and J. B. Buse, Management of hyperglycaemia in type 2 diabetes, 2018. A consensus report by the American Diabetes Association (ADA) and the European Association for the Study of Diabetes (EASD), Diabetologia, 2018, 61, 2461–2498 CrossRef PubMed.
- C. V. Rizos, A. Kei and M. S. Elisaf, The current role of thiazolidinediones in diabetes management, Arch. Toxicol., 2016, 90, 1861–1881 CrossRef CAS PubMed.
- V. Volarevic, M. Misirkic, L. Vucicevic, V. Paunovic, B. Simovic Markovic, M. Stojanovic, M. Milovanovic, V. Jakovljevic, D. Micic, N. Arsenijevic, V. Trajkovic and M. L. Lukic, Metformin aggravates immune-mediated liver injury in mice, Arch. Toxicol., 2015, 89, 437–450 CrossRef CAS PubMed.
- Ş. Bâlici, M. Wankeu-Nya, D. Rusu, G. Z. Nicula, M. Rusu, A. Florea and H. Matei, Ultrastructural analysis of in vivo hypoglycemiant effect of two polyoxometalates in rats with streptozotocin-induced diabetes, Microsc. Microanal., 2015, 21, 1236–1248 CrossRef PubMed.
- Z. Ilyas, H. S. Shah, R. Al-Oweini, U. Kortz and J. Iqbal, Antidiabetic potential of polyoxotungstates: in vitro and in vivo studies, Metallomics, 2014, 6, 1521–1526 RSC.
- K. Nomiya, H. Torii, T. Hasegawa, Y. Nemoto, K. Nomura, K. Hashino, M. Uchida, Y. Kato, K. Shimizu and M. Oda, Insulin mimetic effect of a tungstate cluster. Effect of oral administration of homo-polyoxotungstates and vanadium-substituted polyoxotungstates on blood glucose level of STZ mice, J. Inorg. Biochem., 2001, 86, 657–667 CrossRef CAS PubMed.
- A. Flütsch, T. Schroeder, M. G. Grütter and G. R. Patzke, HIV-1 protease inhibition potential of functionalized polyoxometalates, Bioorg. Med. Chem. Lett., 2011, 21, 1162–1166 CrossRef PubMed.
- Y. Qi, Y. Xiang, J. Wang, Y. Qi, J. Li, J. Niu and J. Zhong, Inhibition of hepatitis C virus infection by polyoxometalates, Antiviral Res., 2013, 100, 392–398 CrossRef CAS PubMed.
- X. Sun, Y. Wu, W. Gao, K. Enjyoji, E. Csizmadia, C. E. Müller, T. Murakami and S. C. Robson, CD39/ENTPD1 expression by CD4+ Foxp3+ regulatory T cells promotes hepatic metastatic tumor growth in mice, Gastroenterology, 2010, 139, 1030–1040 CrossRef CAS PubMed.
- N. I. Gumerova, E. Al-Sayed, L. Krivosudsky, H. Cipcic-Paljetak, D. Verbanac and A. Rompel, Antibacterial Activity of Polyoxometalates Against Moraxella catarrhalis, Front. Chem., 2018, 6, 336 CrossRef PubMed.
- M. Inoue, T. Suzuki, Y. Fujita, M. Oda, N. Matsumoto, J. Iijima and T. Yamase, Synergistic effect of polyoxometalates in combination with oxacillin against methicillin-resistant and vancomycin-resistant Staphylococcus aureus: a high initial inoculum of 1 × 108 cfu/ml for in vivo test, Biomed. Pharmacother., 2006, 60, 220–226 CrossRef CAS PubMed.
- T. L. Turner, V. H. Nguyen, C. C. McLauchlan, Z. Dymon, B. M. Dorsey, J. D. Hooker and M. A. Jones, Inhibitory effects of decavanadate on several enzymes and Leishmania tarentolae in vitro, J. Inorg. Biochem., 2012, 108, 96–104 CrossRef CAS PubMed.
- H. Cao, C. Li, W. Qi, X. Meng, R. Tian, Y. Qi, W. Yang and J. Li, Synthesis, cytotoxicity and antitumour mechanism investigations of polyoxometalate doped silica nanospheres on breast cancer MCF-7 cells, PLoS One, 2017, 12, e0181018 CrossRef PubMed.
- X. Wang, J. Liu, J. Li, Y. Yang, J. Liu, B. Li and M. T. Pope, Synthesis and antitumor activity of cyclopentadienyltitanium substituted polyoxotungstate [CoW11O39(CpTi)]7- (Cp=η5-C5H5), J. Inorg. Biochem., 2003, 94, 279–284 CrossRef CAS PubMed.
- J. Iqbal, M. Barsukova-Stuckart, M. Ibrahim, S. U. Ali, A. A. Khan and U. Kortz, Polyoxometalates as potent inhibitors for acetyl and butyrylcholinesterases and as potential drugs for the treatment of Alzheimer's disease, Med. Chem. Res., 2013, 22, 1224–1228 CrossRef CAS.
- M. B. Colovic, B. Medic, M. Cetkovic, T. Kravic Stevovic, M. Stojanovic, W. W. Ayass, A. S. Mougharbel, M. Radenkovic, M. Prostran, U. Kortz and D. Z. Krstic, Toxicity evaluation of two polyoxotungstates with anti-acetylcholinesterase activity, Toxicol. Appl. Pharmacol., 2017, 333, 68–75 CrossRef CAS PubMed.
- R. Raza, A. Matin, S. Sarwar, M. Barsukova-Stuckart, M. Ibrahim, U. Kortz and J. Iqbal, Polyoxometalates as potent and selective inhibitors of alkaline phosphatases with profound anticancer and amoebicidal activities, Dalton Trans., 2012, 41, 14329–14336 RSC.
- S. Y. Lee, A. Fiene, W. Li, T. Hanck, K. A. Brylev, V. E. Fedorov, J. Lecka, A. Haider, H. J. Pietzsch, H. Zimmermann, J. Sevigny, U. Kortz, H. Stephan and C. E. Muller, Polyoxometalates--potent and selective ecto-nucleotidase inhibitors, Biochem. Pharmacol., 2015, 93, 171–181 CrossRef CAS PubMed.
- C. E. Muller, J. Iqbal, Y. Baqi, H. Zimmermann, A. Rollich and H. Stephan, Polyoxometalates-a new class of potent ecto-nucleoside triphosphate diphosphohydrolase (NTPDase) inhibitors, Bioorg. Med. Chem. Lett., 2006, 16, 5943–5947 CrossRef PubMed.
- R. Prudent, V. Moucadel, B. Laudet, C. Barette, L. Lafanechere, B. Hasenknopf, J. Li, S. Bareyt, E. Lacote, S. Thorimbert, M. Malacria, P. Gouzerh and C. Cochet, Identification of polyoxometalates as nanomolar noncompetitive inhibitors of protein kinase CK2, Chem. Biol., 2008, 15, 683–692 CrossRef CAS PubMed.
- A. Haider, K. Zarschler, S. A. Joshi, R. M. Smith, Z. Lin, A. S. Mougharbel, U. Herzog, C. E. Müller, H. Stephan and U. Kortz, Preyssler-Pope-Jeannin Polyanions [NaP5W30O110]14− and [AgP5W30O110]14−: Microwave-Assisted Synthesis, Structure, and Biological Activity, Z. Anorg. Allg. Chem., 2018, 644, 752–758 CrossRef CAS.
- S. Y. Saad and T. A. Najjar, Effects of STZ-induced diabetes and its treatment with vanadyl sulphate on cyclosporine A-induced nephrotoxicity in rats, Arch. Toxicol., 2005, 79, 493–499 CrossRef CAS PubMed.
- G. J. Krinke, The Laboratory Rat (Handbook of Experimental Animals), Elsevier, 2000 Search PubMed.
- C. Carpene, S. Garcia-Vicente, M. Serrano, L. Marti, C. Belles, M. Royo, J. Galitzky, A. Zorzano and X. Testar, Insulin-mimetic compound hexaquis (benzylammonium) decavanadate is antilipolytic in human fat cells, World J. Diabetes, 2017, 8, 143–153 CrossRef PubMed.
- J. El Hilaly, Z. H. Israili and B. Lyoussi, Acute and chronic toxicological studies of Ajuga iva in experimental animals, J. Ethnopharmacol., 2004, 91, 43–50 CrossRef PubMed.
- R. W. Snyder and J. S. Berns, Reviews: use of insulin and oral hypoglycemic medications in patients with diabetes mellitus and advanced kidney disease, Semin. Dial., 2004, 17, 365–370 CrossRef PubMed.
- J. Wang, X. Qu, Y. Qi, J. Li, X. Song, L. Li, D. Yin, K. Xu and J. Li, Pharmacokinetics of anti-HBV polyoxometalate in rats, PLoS One, 2014, 9, e98292 CrossRef PubMed.
- P. L. Wolf, Biochemical diagnosis of liver disease, Indian J. Clin. Biochem., 1999, 14, 59–90 CrossRef CAS PubMed.
|
This journal is © The Royal Society of Chemistry 2020 |