DOI:
10.1039/C9RA09524A
(Paper)
RSC Adv., 2020,
10, 8002-8007
The inhibition of H1N1 influenza induced apoptosis by sodium selenite through ROS-mediated signaling pathways
Received
15th November 2019
, Accepted 15th February 2020
First published on 24th February 2020
Abstract
The high variability of influenza viruses has made it more difficult for people to cope with influenza. When antigen transformation occurs, even new influenza without preventive vaccines may be produced, which poses a great threat to human health. Selenium is an essential trace element in humans and mammals, and has many biological activities. It has attracted people's research interest in recent years. In this study, MDCK cells were used as a model to observe the effect of sodium selenite on H1N1 influenza virus. Our research showed that sodium selenite (Na2SeO3) has an anti-influenza H1N1 virus effect, and the anti-viral effect of sodium selenite was further demonstrated by caspase-3, AKT, MAPK and p53 signaling pathways. The investigations of the mechanism revealed that the sodium selenite could block H1N1 influenza from infecting MDCK cells through inhibiting the production of ROS. The results demonstrate that selenium supplementation may provide a feasible approach to inhibit the infection of H1N1 influenza virus.
1 Introduction
Influenza viruses cause seasonal epidemics of human diseases as well as large-scale morbidity and high mortality.1–3 The classification of influenza A virus is based on different combinations of hemagglutinin (HA) and neuraminidase (NA) which were the protein on the surface of the virus.4 In the past century, there were three influenza A viruses: H1N1, H2N2 and H3N2 leading to human pandemics.5 Nowadays, antiviral treatment relies mainly on drugs that target the virus.6 Neuraminidase inhibitors inhibit the release of budding virus particles by host cells, primarily by binding to the active site of neuraminidase. The M2 inhibitor achieves an antiviral effect by blocking the ion channel activity of the influenza A virus M2 protein during the viral phase.7 Continuous antigenic drift and antigenic translation of viral surface glycoproteins make it a major factor in the unpredictable effects of influenza vaccines.8–10 Vaccine protection is not effective enough to provide reliable protection for humans.11–13 At present, due to the rising resistance of influenza virus to existing anti-influenza drugs, we urgently need to find new antiviral drugs.14–16
As an essential trace element, selenium participates in many biological activities of the human body, including the intensification, differentiation and proliferation of immune cells.17–19 Inorganic selenium has four forms of existence in nature: selenate, selenite, elemental Se and selenide.20–22 These forms are converted by the biological system into selenoamino acids selenocysteine and selenomethionine, a more bioavailable organic form.23 The prognosis of some infectious diseases has been confirmed to be related to the body's selenium content.24 In some studies, selenium has been shown to have antiviral effects, but the exact mechanism is not clear.25–28 It is an important component of glutathione peroxidase (GSH-Px) and active center, acting as a free radical scavenger to protect cells from oxidative stress.29–31
Free radicals in the human body can damage cells, tissues and organs, affect health, and shorten life expectancy.32–35 Antioxidants are “free agent scavengers” and have a catalytic effect on the treatment and prevention of many diseases.36,37 Studies have shown that more ROS are detected in host cells after viral infection, which is related to the degree of viral infection.38–40 Therefore, the ROS level can indirectly reflect the level of virus replication and speculate that virus replication and intracellular redox system linked.41–43 Several apoptosis related protein, antioxidant proteins and pxidative damage inhibitors have been reported by Leung et al.44–46 Flavokawain A, a novel Chaconne from Kava extract induces apoptosis in bladder cancer cells by Zi et al.47 The apoptotic effect of nanosilver is mediated by a ROS and JNK dependent mechanism involving the mitochondrial pathway in NIH3T3 cells by Hsin et al.48 Similarly, the goal of preventing the replication of influenza A virus can be achieved by inhibiting activity and inhibiting the production of oxygen.49 The oxidative stress pathway is very important for targeted treatment.50 Therefore, this study ascertains how sodium selenite will antagonize H1N1 influenza virus induced MDCK cells apoptosis through ROS-mediated signaling pathways.
2 Experimental
2.1 Materials
MDCK cells were purchased from American Type Culture Collection (ATCC® CCL-34™). H1N1 influenza virus was provided by Virus laboratory, Guangzhou institute of pediatrics, Guangzhou Women and Children's Medical Center, Guangzhou Medical University. Dulbecco's modified Eagle's medium (DMEM), trypsin–EDTA (0.25%) (phenol red) and fetal bovine serum (FBS) were purchased from Gibco. TPCK Trypsin was purchased from Thermo Scientific. Caspase-3, PARP, p53 and AKT antibody were obtained form Cell Signaling Technology. 2′,7′-Dichlorofluorescein diacetate (DCFH-DA) were obtained from Sigma. Cell Counting Kit was obtained from Beyotime Biotechnology. Milli-Q water was purification from Millipore in all experiments.
2.2 Preparation of Na2SeO3 stock solution
The preparation of sodium selenite solution was as follow: 0.017 g of sodium selenite solid powder was put into 100 mL of distilled water. The concentration of stock solution was 1 mM, the stock solution was put in 4 °C refrigerator for use.
2.3 Determination of the TCID50 of H1N1
Cells was cultured in 96-well plate at a density of 8 × 104 cells per well and incubated for 24 hours. Then removed the original medium, washed the plates twice with PBS, the virus stock was placed in an EP tube and diluted with a medium containing 2 μg mL−1 TPCK trypsin-free serum at a ratio of 10 times, 10−1, 10−2, 10−3, 10−4, 10−5, 10−6, 10−7, 10−8, 10−9 respectively, and each dilution was inoculated with a tandem A total of 8 wells, each inoculated with 100 μL. The control group was also set up in a column and cultured in 36.5 °C and 5% CO2 incubator. After 2 hours of adsorption, removed the medium, washed the plates twice with PBS, then 200 μL of serum-free medium containing 2 μg mL−1 TPCK trypsin was added and placed in incubator. 50% cell culture infectious dose (TCID50) was then calculated according to the Reed–muench calculation method.
2.4 Cell culture and cell viability by cell counting kit-8 assay B (CCK-8)
MDCK cells were seeded in 96-well culture plates at a density of 4 × 104 cells per well for 24 h, there were four groups as follow: normal cell control group, single drug control group, virus control group and virus plus drug treatment group. After the virus was incubated for 2 hours, all the groups were changed medium and washed with PBS twice. Serum-free medium 200 μL was added to the normal cell control group and the virus control group, and the serum-free medium containing 1 μM of sodium selenite 200 μL was added to the drug-only control group and the virus plus drug-treated group. Then these four groups were placed in the incubator. Observed cytopathic conditions for 72 h, added 10 μL of CCK-8 solution to each well, and the mixture was incubated for 2 hours. Cell viability was measured at 450 nm using a microplate spectrophotometer.
2.5 Activation of caspase-3
Caspase-3 activity was measured according to the methods described as previously described.51 Cell lysates, detection buffer and specific caspase substrates Ac-DEVD-pNA (2 mM) were added into a 96-well plate for 2 h at 37 °C. The activation of caspase-3 was measured through the conversion of pNA, which was measured by 405 nm absorbance using a microplate reader.
2.6 Measurement of reactive oxygen species (ROS) level
The level of intracellular ROS induced by Na2SeO3 was detected as describe previously.52 In brief, after treatment with Na2SeO3 in different time, the MDCK cells were stained with DCFH-DA (10 μM) for 20 min. The level of ROS was detected by a fluorescence microscope. The fluorescence intensity was determined by a fluorescence plate reader with an excitation wavelength of 488 nm and emission wavelength of 525 nm respectively.
2.7 Western blotting analysis
The expression of proteins associated with different signaling pathways after treatment with Na2SeO3 in MDCK cells were analyzed by western blot as previously reported.53 In brief, the four groups MDCK cells were lysed with RIPA solution after treatment with treatment for 48 h, followed by concentration detection of the proteins with a BCA kit. After that, equivalent amount of proteins were loaded in SDS-PAGE, then transferred to polyvinylidene difluoride membranes, and finally visualized by X-ray film.
2.8 Statistical analysis
All results were all obtained from three independent tests and presented as mean ± S.D. The differences among multiple groups were compared using one-way analysis of variance (ANOVA). The probability of P value of <0.05 (*) and P value of <0.01 (**) were deemed to be statistically significant in all experiments.
3 Results and discussion
3.1 TCID50 of H1N1
As shown in Table 1, the Reed–Muench assay was used to test the titer of H1N1 (TCID50 = 107.7). H1N1 virus was used for in vitro study at the titer of 100 TCID50.
Table 1 The Reed–Muench assay was used to test the titer of H1N1 (TCID50 = 107.7)
Dilution of H1N1 |
Number of cells inoculated |
CPE |
No CPE |
Accumulation |
Ratio of CPE |
CPE |
No CPE |
10−1 |
8 |
8 |
0 |
54 |
0 |
0 |
10−2 |
8 |
8 |
0 |
46 |
0 |
0 |
10−3 |
8 |
8 |
0 |
38 |
0 |
0 |
10−4 |
8 |
8 |
0 |
30 |
0 |
0 |
10−5 |
8 |
8 |
0 |
25 |
0 |
0 |
10−6 |
8 |
7 |
1 |
17 |
1 |
94.4% |
10−7 |
8 |
4 |
4 |
10 |
5 |
66.7% |
10−8 |
8 |
5 |
3 |
6 |
8 |
42.9% |
10−9 |
8 |
1 |
7 |
1 |
15 |
6.25% |
10−10 |
8 |
0 |
8 |
0 |
23 |
0 |
Blank control |
8 |
0 |
8 |
|
|
|
3.2 In vitro antiviral activity by Na2SeO3
The CCK-8 assay was performed to measure the anti-proliferation activity of H1N1 infected MDCK cells after treatment with Na2SeO3. As shown in Fig. 1A, the cell survival rate of cells which were treated with Na2SeO3 after infected by H1N1 (58.8%) was higher than cells without any treatment (26.4%), the resulted showed remarkably suppressed the viability of H1N1 and less cytotoxicity to normal cells (84.1%). As shown in Fig. 1B, after treatment with H1N1, the cell number decrease obviously, while cell survival rate in infected cells treated with Na2SeO3 was increased. These results indicated Na2SeO3 suppressed the infection of H1N1 effectively.
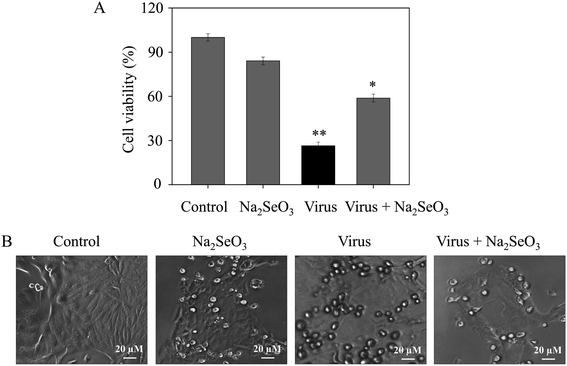 |
| Fig. 1 Effects of Na2SeO3 on the growth of H1N1 infection of MDCK cells by CCK-8 assay. (A) Antiviral activity of Na2SeO3. Concentration of Na2SeO3 was 1 μM. (B) Morphological changes in H1N1-infected MDCK cells observed by phase-contrast microscopy. Bars with different characters are statistically different at *p < 0.05 or **p < 0.01 level. | |
3.3 Inhibition of caspase-3 activation by Na2SeO3
Caspase-3 was confirmed as an important mediator of apoptosis in mammalian cells. As shown in Fig. 2, the treatment of MDCK cells with H1N1 influenza virus significantly increased the activity of caspase-3 (215%), but Na2SeO3 decreased the activity of caspase-3 (137%). These results show that Na2SeO3 inhibits H1N1 influenza virus activity through caspase-3 mediated apoptosis pathway.
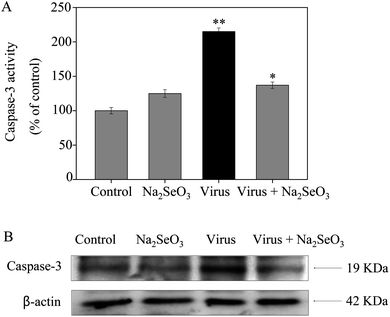 |
| Fig. 2 Inhibition of caspase-3 activity by Na2SeO3. Cells were treated with Na2SeO3 and caspase-3 activity was detected by synthetic fluorogenic substrate. Concentration of Na2SeO3 was 1 μM. Bars with different characters are statistically different at *p < 0.05 or **p < 0.01 level. | |
3.4 Inhibition of ROS generation by Na2SeO3
The ROS generation was monitored by DCF fluorescence assay to indicate its role in the action mechanisms of Na2SeO3. As shown in Fig. 3A, the H1N1 influenza virus increased the intracellular ROS generation in MDCK cells (190%). Na2SeO3 significantly decreased the intracellular ROS generation (135%). Stronger fluorescent intensity of DCF was found in MDCK cells by H1N1 influenza virus than Na2SeO3 as shown in Fig. 4B. These results indicated the involvement of ROS in the antiviral action of Na2SeO3.
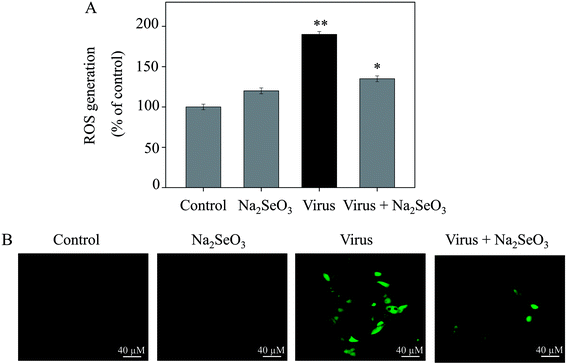 |
| Fig. 3 ROS overproduction was inhibited by Na2SeO3 in H1N1 infection of MDCK cells (A) ROS levels were detected by DCF fluorescence intensity. (B) H1N1 infection of MDCK cells were preincubated with 10 μM DCF for 30 min and then treated with Na2SeO3. Concentration of Na2SeO3 was 1 μM. Bars with different characters are statistically different at *p < 0.05 or **p < 0.01 level. | |
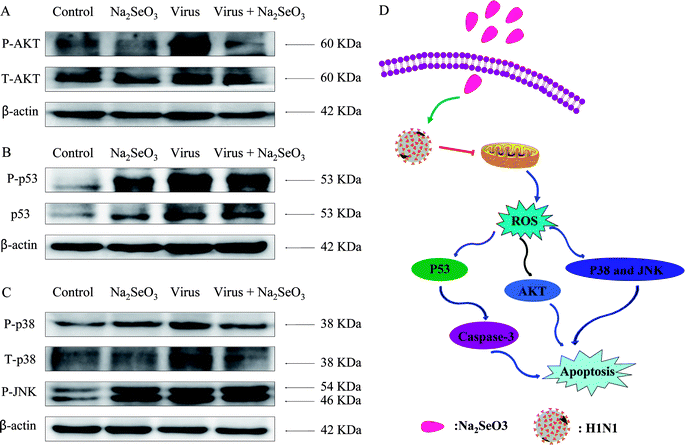 |
| Fig. 4 Intracellular apoptotic signaling pathways by Na2SeO3 in H1N1 infection of MDCK cells. (A) Activation of AKT signaling pathway. (B) Phosphorylation status expression levels of p53 pathways. (C) Phosphorylation status expression levels of p38 and JNK pathways. (D) The main signaling pathway of ROS-mediated signaling pathways. | |
3.5 ROS-mediated signaling pathways
The overexpression of ROS could lead to DNA damage and result in cell apoptosis through regulation of AKT and p53 signaling pathways. Due to the detection of ROS overproduction in cells exposed to H1N1 influenza virus, western blotting was used to examine the effects on the ROS-mediated downstream pathways. As shown in Fig. 4A–C, treatments of the cells with Na2SeO3 obviously inhibited the expression levels of AKT, p53, p38 and JNK in MDCK cells. As showed in Fig. 4D, the results revealed that Na2SeO3 inhibited H1N1 influenza virus-induced MDCK cells apoptosis by ROS-mediated AKT and p53 and MAPK signaling pathways.
4 Conclusions
In conclusion, this study demonstrates that Na2SeO3 has the ability to inhibit the infection of H1N1 influenza virus in vitro. Na2SeO3 significantly reduced the population of apoptotic cells infected by H1N1 influenza virus. The potential molecular mechanisms have shown that the main mode by Na2SeO3 reduced cell death is apoptosis. The mechanism suggests that Na2SeO3 inhibits caspase-3 mediated apoptosis via ROS production. More importantly, studies of apoptotic signaling pathways triggered by Na2SeO3 in MDCK cells were the AKT, p53 and MAPK pathways. Therefore, our results indicate that Na2SeO3 has the ability to inhibit influenza virus in a safe dose range, and it can provide a selenium species for the development of antiviral drugs for influenza.
Author contributions
Yinghua Li and Guifang Gong designed the study, analyzed the experimental data and drafted the manuscript. Kunyan He and Qiumei Yang carried out the experiments. Min Guo, Tiantian Xu, Changbing Wang, Mingqi Zhao, Yi Chen, Miaomiao Du and Bingyuan Li participated in its design. Yanqing Huang and Bing Zhu refined the manuscript and coordination. All authors read and approved the final manuscript.
Conflicts of interest
The authors declare no competing financial interest.
Acknowledgements
This work was supported by the Technology Planning Project of Guangzhou (201804010183), the Medical Scientific Research Foundation of Guangdong Province (A2018306), the Pediatrics Institute Foundation of Guangzhou Women and Children’s Medical Centre (YIP-2018-036 and YIP-2019-025), the Pediatrics Institute Foundation of Guangzhou Women and Children's Medical Centre (Pre-NSFC-2019-017 and IP-2018-004), the Guangdong Natural Science Foundation (2016A030313495). Science and Technology Plan Project of Guangdong Province (2014A020212602). Project of Traditional Chinese Medicine Bureau of Guangdong Province (20192075). The Guangzhou Medical University student’s science and technology innovation project (2019AEK02).
Notes and references
- A. Stevaert and L. Naesens, Med. Res. Rev., 2016, 36, 1127–1173 CrossRef PubMed
. - S. J. Nabel GJ, Nature, 2019, 565, 29–31 CrossRef PubMed
. - A. D. Iuliano, K. M. Roguski, H. H. Chang, D. J. Muscatello, R. Palekar, S. Tempia, C. Cohen, J. M. Gran, D. Schanzer, B. J. Cowling, P. Wu, J. Kyncl, L. W. Ang, M. Park, M. Redlberger-Fritz, H. Yu, L. Espenhain, A. Krishnan, G. Emukule, L. van Asten, S. Pereira da Silva, S. Aungkulanon, U. Buchholz, M. A. Widdowson and J. S. Bresee, Lancet, 2018, 391, 1285–1300 CrossRef
. - C. Paules and K. Subbarao, Lancet, 2017, 390, 697–708 CrossRef
. - E. C. Hutchinson, Trends Microbiol., 2018, 26, 809–810 CrossRef CAS PubMed
. - Y. H. Li, Z. F. Lin, G. F. Gong, M. Guo, T. T. Xu, C. B. Wang, M. Q. Zhao, Y. Xia, Y. Tang, J. Y. Zhong, Y. Chen, L. Hua, Y. Q. Huang, F. L. Zeng and B. Zhu, J. Mater. Chem. B, 2019, 7, 4252–4262 RSC
. - R. G. Webster and E. A. Govorkova, Ann. N. Y. Acad. Sci., 2014, 1323, 115–139 CrossRef CAS PubMed
. - O. Suptawiwat, A. Kongchanagul, C. Boonarkart and P. Auewarakul, Virus Res., 2018, 250, 43–50 CrossRef CAS PubMed
. - K. S. Xue, L. H. Moncla, T. Bedford and J. D. Bloom, Trends Microbiol., 2019, 26, 781–793 CrossRef PubMed
. - L. H. Moncla, N. W. Florek and T. C. Friedrich, Trends Microbiol., 2017, 25, 432–434 CrossRef CAS PubMed
. - V. N. Petrova and C. A. Russell, Nat. Rev. Microbiol., 2018, 16, 60 CrossRef CAS PubMed
. - F. Krammer, Biotechnol. J., 2015, 10, 690–U670 CrossRef CAS PubMed
. - K. Hoschler and M. C. Zambon, Lancet, 2017, 390, 627–628 CrossRef
. - D. M. Lyons and A. S. Lauring, Viruses, 2018, 10, 407 CrossRef PubMed
. - R. Nachbagauer and F. Krammer, Clin. Microbiol. Infect., 2017, 23, 222–228 CrossRef CAS PubMed
. - G. Dos Santos, E. Neumeier and R. Bekkat-Berkani, Hum. Vaccines Immunother., 2016, 12, 699–708 CrossRef PubMed
. - K. S. Prabhu and X. G. Lei, Adv. Nutr., 2016, 7, 415–417 CrossRef CAS PubMed
. - M. Roman, P. Jitaru and C. Barbante, Metallomics, 2014, 6, 25–54 RSC
. - Q. Li, G. Y. Chen, W. Wang, W. J. Zhang, Y. Y. Ding, T. Zhao, F. Li, G. H. Mao, W. W. Feng, Q. Wang, L. Q. Yang and X. Y. Wu, Int. J. Biol. Macromol., 2018, 117, 878–889 CrossRef CAS PubMed
. - T. Zhang, G. Zhao, X. Y. Zhu, K. F. Jiang, H. C. Wu, G. Z. Deng and C. W. Qiu, J. Cell. Physiol., 2019, 234, 2511–2522 CrossRef CAS PubMed
. - G. F. Gong, B. L. Fu, C. X. Ying, Z. Q. Zhu, X. Q. He, Y. Y. Li, Z. X. Shen, Q. S. Xuan, Y. Q. Huang, Y. Lin and Y. H. Li, RSC Adv., 2018, 8, 39957–39966 RSC
. - M. Guo, Y. H. Li, Z. F. Lin, M. Q. Zhao, M. S. Xiao, C. B. Wang, T. T. Xu, Y. Xia and B. Zhu, RSC Adv., 2017, 7, 52456–52464 RSC
. - E. Mangiapane, A. Pessione and E. Pessione, Curr. Protein Pept. Sci., 2014, 15, 598–607 CrossRef CAS PubMed
. - M. Kieliszek, Molecules, 2019, 24, 1298 CrossRef CAS PubMed
. - J. C. Avery and P. R. Hoffmann, Nutrients, 2018, 10, 1203 CrossRef PubMed
. - L. M. Watanabe, F. Barbosa, A. A. Jordao and A. M. Navarro, Nutrition, 2016, 32, 1238–1242 CrossRef CAS PubMed
. - H. C. Anyabolu, E. A. Adejuyigbe and O. O. Adeodu, AIDS Res. Treat., 2014, 2014, 351043 CAS
. - B. Shojadoost, R. R. Kulkarni, A. Yitbarek, A. Laursen, K. Taha-Abdelaziz, T. N. Alkie, N. Barjesteh, W. M. Quinteiro, T. K. Smith and S. Sharif, Vet. Immunol. Immunopathol., 2019, 207, 62–68 CrossRef CAS PubMed
. - K. Ivory, E. Prieto, C. Spinks, C. N. Armah, A. J. Goldson, J. R. Dainty and C. Nicoletti, Clin. Nutr., 2017, 36, 407–415 CrossRef CAS PubMed
. - D. L. Hatfield, P. A. Tsuji, B. A. Carlson and V. N. Gladyshev, Trends Biochem. Sci., 2014, 39, 112–120 CrossRef CAS PubMed
. - X. Y. Han, X. Y. Song, F. B. Yu and L. X. Chen, Chem. Sci., 2017, 8, 6991–7002 RSC
. - M. Gao, R. Wang, F. B. Yu and L. X. Chen, Biomaterials, 2018, 160, 1–14 CrossRef CAS PubMed
. - X. Zhang, Y. Huang, X. Y. Han, Y. Wang, L. W. Zhang and L. X. Chen, Anal. Chem., 2019, 91, 14728–14736 CrossRef CAS PubMed
. - Y. Huang, F. B. Yu, J. C. Wang and L. X. Chen, Anal. Chem., 2016, 88, 4122–4129 CrossRef CAS PubMed
. - X. Y. Song, X. Y. Han, F. B. Yu, X. Y. Zhang, L. X. Chen and C. J. Lv, Theranostics, 2018, 8, 2217–2228 CrossRef CAS PubMed
. - K. Neha, M. R. Haider, A. Pathak and M. S. Yar, Eur. J. Med. Chem., 2019, 178, 687–704 CrossRef CAS PubMed
. - M. L. D. Leal, F. L. Pivot, G. C. Fausto, A. R. Aires, T. H. Grando, D. H. Roos, J. H. Sudati, C. Wagner, M. M. Costa, M. B. Molento and J. B. T. da Rocha, Exp. Parasitol., 2014, 144, 39–43 CrossRef CAS PubMed
. - C. W. Pyo, N. Shin, K. I. Jung, J. H. Choi and S. Y. Choi, Biochem. Biophys. Res. Commun., 2014, 450, 711–716 CrossRef CAS PubMed
. - K. M. Gowdy, Q. T. Krantz, C. King, E. Boykin, I. Jaspers, W. P. Linak and M. I. Gilmour, Part. Fibre Toxicol., 2010, 7, 34 CrossRef CAS PubMed
. - F. C. Camini, C. C. D. Caetano, L. T. Almeida and C. L. D. Magalhaes, Arch. Virol., 2017, 162, 907–917 CrossRef CAS PubMed
. - L. Liu, X. Tu, Y. F. Shen, W. C. Chen, B. Zhu and G. X. Wang, Fish Shellfish Immunol., 2017, 67, 211–217 CrossRef CAS PubMed
. - M. M. Liu, F. Z. Chen, T. Liu, F. M. Chen, S. W. Liu and J. Yang, Microbes Infect., 2017, 19, 580–586 CrossRef CAS PubMed
. - X. G. Xu, Y. Xu, Q. Zhang, F. Yang, Z. Yin, L. X. Wang and Q. F. Li, Vet. Microbiol., 2019, 232, 1–12 CrossRef CAS PubMed
. - T. S. Kang, W. Wang, H. J. Zhong, Z. Z. Dong, Q. Huang, S. W. Mok, C. H. Leung, V. K. Wong and D. L. Ma, Cancer Lett., 2017, 396, 76–84 CrossRef CAS PubMed
. - K. J. Wu, J. M. Huang, H. J. Zhong, Z. Z. Dong, K. Vellaisamy, J. J. Lu, X. P. Chen, P. Chiu, D. W. J. Kwong, Q. B. Han, D. L. Ma and C. H. Leung, PLoS One, 2017, 12, 1–12 Search PubMed
. - K. J. Wu, H. J. Zhong, G. Yang, C. Wu, J. M. Huang, G. Li, D. L. Ma and C. H. Leung, Chemistry, 2018, 13, 275–279 CAS
. - X. Zi and A. R. Simoneau, Cancer Res., 2005, 65, 3479–3486 CrossRef CAS PubMed
. - Y. H. Hsin, C. F. Chen, S. Huang, T. S. Shih, P. S. Lai and P. J. Chueh, Toxicol. Lett., 2008, 179, 130–139 CrossRef CAS PubMed
. - R. Vlahos, J. Stambas and S. Selemidis, Trends Pharmacol. Sci., 2012, 33, 3–8 CrossRef CAS PubMed
. - Z. U. Rehman, C. C. Meng, Y. J. Sun, A. Safdar, R. H. Pasha, M. Munir and C. Ding, Oxid. Med. Cell. Longevity, 2018, 5123147 Search PubMed
. - Y. Li, Z. Lin, M. Zhao, T. Xu, C. Wang, L. Hua, H. Wang, H. Xia and B. Zhu, ACS Appl. Mater. Interfaces, 2016, 8, 24385–24393 CrossRef CAS PubMed
. - Y. Li, Z. Lin, M. Guo, M. Zhao, Y. Xia, C. Wang, T. Xu and B. Zhu, Int. J. Nanomed., 2018, 13, 2005–2016 CrossRef CAS PubMed
. - Y. H. Li, Z. F. Lin, M. Q. Zhao, M. Guo, T. T. Xu, C. B. Wang, H. M. Xia and B. Zhu, RSC Adv., 2016, 6, 89679–89686 RSC
.
Footnote |
† Guifang Gong and Yinghua Li contributed equally to the work. |
|
This journal is © The Royal Society of Chemistry 2020 |