DOI:
10.1039/C9RA09013D
(Paper)
RSC Adv., 2020,
10, 818-827
Sulphated alumina tungstic acid (SATA): a highly efficient and novel heterogeneous mesostructured catalyst for the synthesis of pyrazole carbonitrile derivatives and evaluation of green metrics†
Received
1st November 2019
, Accepted 18th December 2019
First published on 3rd January 2020
Abstract
A novel mesostructured catalyst sulphated alumina tungstic acid (SATA) has been prepared by an easy route. Various techniques such as IR, XRD, SEM, EDX, TEM, TGA and BET were used to characterize the synthesized catalyst. The catalytic activity of the meso material has been explored by synthesizing a series of new pyrazole carbonitrile derivatives from aromatic aldehydes, ethylcyanoacetate, phenylhydrazine/hydrazine hydrate in ethanol under reflux conditions. Furthermore, the “greenness” of this protocol when estimated by green metrics, displayed satisfactory results. The protocol is free from column chromatography, and toxic solvents and is more efficient as compared to reported ones.
Introduction
Various eco-friendly sulfonic acid-based materials have been successfully used as catalysts in organic synthesis1 due to their higher acidic strength, increased thermal stability, reduced toxicity, etc.2 Various sulfonic acids have been heterogenized on solid supports to meet sustainability requirements such as recyclability, increased efficiency in terms of higher surface area/large pore space e.g., sulfonated silica materials,3 sulfonated carbon materials,4 sulphated zirconia,5 sulphated hybrid materials,6 sulfonated magnetic materials,7 etc.8 Solid acids are one of the most important classes of catalyst used in the chemical industry.2,3,9 Compared to liquid acids, solid acids cause less corrosion of the facility,10–12 can be easily separated from the reaction medium for recycling,13–15 and their properties may be tunable for specific feedstock and products.16–18 Among the solid acids, a great deal of attention has been paid to tungstic acid, as it had shown considerable superiorities, especially in the heterogeneous form in terms of acidity, thermal stability, and hydrophobicity, in comparison with the other materials,19,20 and thus hold a promising candidate for application in various organic reactions. Basic reactions such as hydrodesulfurization, hydrodenitrogenation, and hydrodearomatisation are the most important reactions catalyzed by tungstic acid in the chemical industry.21,22 Since then, tungstic acid has received continuous attention because of its increasing advantages in catalysis. Other organic reactions catalysed by tungstic acid include oxidation,23 hydroxylation,24 epoxidation25 and many other transformations.26–28 In spite of these advantageous properties and applications, tungstic acid suffer from severe drawbacks such as their deactivation during reaction due to coke formation on the surface and low surface area.29 This limits its applications in many reactions.30 These drawbacks, however, can be removed by dispersing them on solid support materials with a high surface area. In this regard, mesoporous materials are good candidates as they possess unique structural features such as huge BET, large surface area, tunable pore diameter, flexibility to accommodate several functional groups and metals on to the surface. The introduction of mesoporous materials has brought tremendous economic and environmental revolution31 and plays a key role in catalysis32 at the industrial scale. Among mesoporous materials, mesoporous alumina is a good choice as it is cheap and easily available and also possesses beneficial properties such as high thermal stability, high purity, favourable bulk density, etc.33
In the present work, we have developed an easy route for the synthesis of mesoporous sulphated alumina tungstic acid and explored its catalytic activity by synthesizing a series of new pyrazole carbonitrile derivatives via multicomponent reaction. It is worthy to mention that pyrazole carbonitriles are interesting scaffolds due to their potential biological activities including anti-HIV34 antibacterial and antifungal35 anticancer36 anti-depressant activities37 etc.
Results and discussion
The synthesis of catalyst sulphated alumina tungstic acid (SATA) is shown in Scheme 1. H+ ion concentration of the catalyst was obtained as 0.44 meq. g−1 using back titration method.
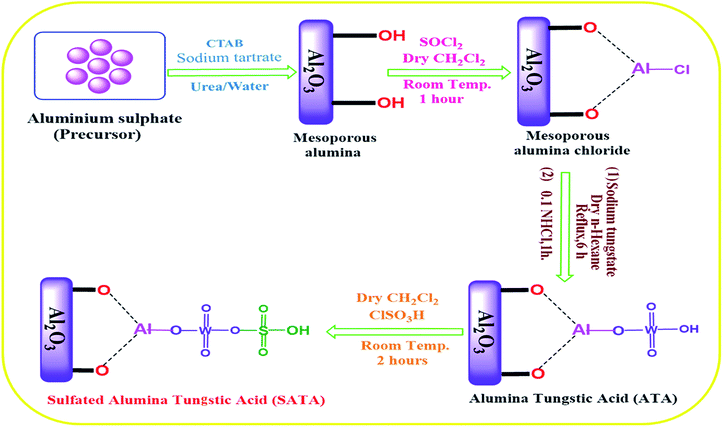 |
| Scheme 1 Flow diagram depicting preparation of catalyst. | |
The IR spectrum (Fig. 1a), exhibited absorption peak at 3420 cm−1 due to surface OH groups. The absorption peaks between 510–1000 cm−1 were due to bending and stretching vibrations of Al–O bond.38 Sulphated alumina tungstic acid (SATA) (Fig. 1b) showed bands at 1647 cm−1 due to WO42− group, 1130 cm−1 due to SO22− group and 600–700 cm−1 due to S–O bond.
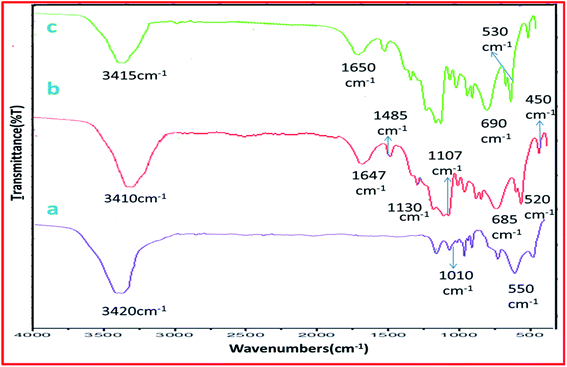 |
| Fig. 1 FT-IR spectra of (a) mesoporous alumina (b) fresh SATA and (c) recycled SATA. | |
In the XRD spectrum of catalyst sulphated alumina tungstic acid (SATA) (Fig. 2a) peaks at 29.89°, 44.96°, 59.95°, 64.99°, 69.92° corresponded to γ-Al2O3 and peaks at 24.98°, 34.95°, 39.98°, 50.45°, 54.89° were due to different phases of α-Al2O3.39 The XRD spectrum of SATA exhibited an additional peak at 22° (Fig. 2b) due to WO4 group.40 Some insignificant changes in the nature of peaks were observed because of bonded sulfonic acid groups on the alumina surface.
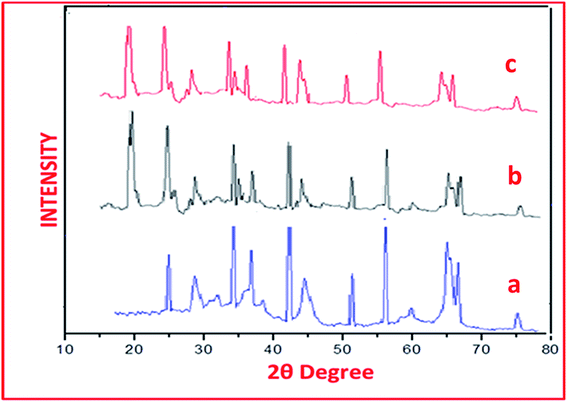 |
| Fig. 2 XRD spectra of (a) mesoporous alumina, (b) fresh SATA and (c) recycled SATA. | |
SEM images of synthesized mesoporous alumina (Fig. 3a) showed the spherical shape of the synthesized mesoporous Al2O3 particles. After functionalization, there were insignificant morphological changes in the synthesized material sulphated alumina tungstic acid (Fig. 3b). The EDX analysis (Fig. 3c) of the catalyst showed the presence of constituent elements W, Al, O, and S. TEM image (Fig. 4A) of the catalyst (SATA) exhibited a wormhole-like porous structure. Furthermore the TEM image of the recycled catalyst (Fig. 4B) showed same morphology indicating that the structure of the synthesized catalyst was not disturbed.
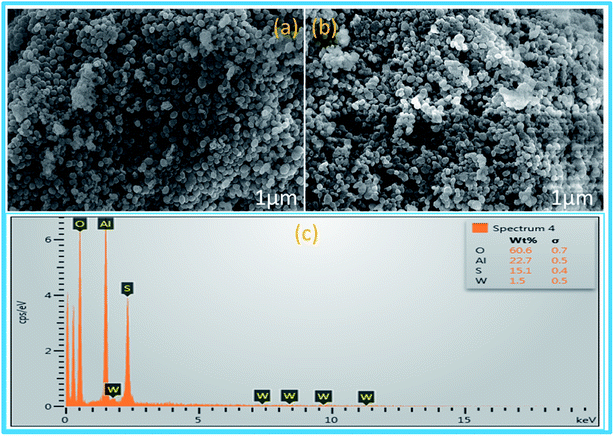 |
| Fig. 3 SEM images of (a) freshly synthesized mesoporous Al2O3, (b) SATA and (c) EDX analysis of SATA. | |
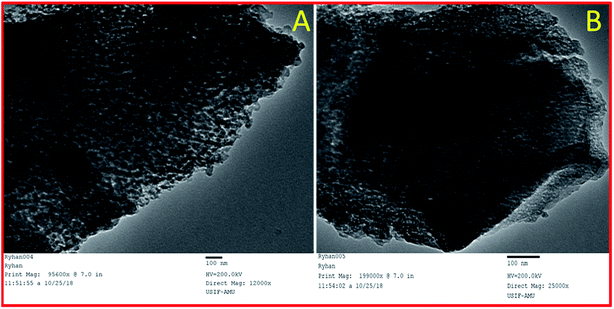 |
| Fig. 4 TEM images of (A) fresh catalyst SATA and (B) recycled catalyst SATA. | |
The surface area of catalyst (SATA) was found to be 298.54 m2 g−1 (Fig. 5) which was slightly lower than the mesoporous alumina (308 m2 g−1).41 The decrease in the surface area could be due to functionalization of mesoporous alumina.
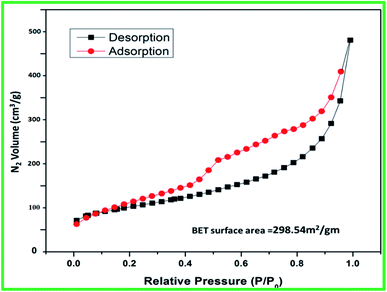 |
| Fig. 5 BET analysis of synthesized catalyst SATA. | |
The TGA curve (Fig. 6) showed a weight loss of 18.8% up to 114 °C due to removal of water molecules trapped in alumina framework. Another weight loss of 6.58% at 303 °C can be attributed to the decomposition of bonded sulfonic acid groups from the surface of mesoporous alumina.
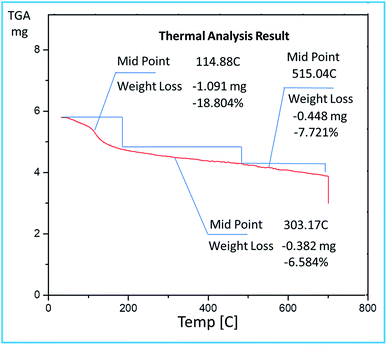 |
| Fig. 6 TGA curve of synthesized catalyst SATA. | |
Optimization of reaction conditions
To find out suitable reaction conditions using SATA for the synthesis of pyrazole carbonitriles, various parameters such as different catalysts/solvents, catalyst loading, temperature etc. were tried to get maximum yield of product (4a). To find suitable catalyst for our reaction we first tried the reaction in the absence of any catalyst. The reaction failed to produce any yield indicating the need of a catalyst (Table 1, entry 1). Then effect of the different sulphuric acid based catalysts under reflux condition was examined. Among them, CH3COOH and H2SO4 catalyzed reactions did not give satisfactory result (Table 1, entries 2 and 3). Among silica, alumina and zirconia-based sulphuric acids, H2SO4–alumina afforded slightly better product yield (Table 1, entry 6). Similarly, PEG and xanthan like supported sulphuric acid organic polymers also gave unsatisfactory yields (Table 1, entries 8 and 9). In the presence of sodium tungstate, the product yield was slightly increased but took a longer time (Table 1, entry 10). The use of alumina tungstic acid (ATA) showed better results (Table 1, entry 11). However, the best results were obtained with sulphated ATA (SATA) affording 94% product yield in 15 min (Table 1, entry 12). Then our study focused on the development of the optimal reaction conditions for this transformation, which included solvent screening and influence of the catalyst amount. Several organic solvents like isopropanol, acetonitrile, methanol, hexane, toluene, and ethane diol when tested, (Table 1, entries 19–24), ethanol was found as a preferred solvent for maximum conversion of substrate into products (94%) in the minimum time period (15 min) (Table 1, entry 12). To find the optimized amount of the catalyst, the reaction was carried out by varying the amount of the catalyst on the model reaction (Table 2). It was found that the conversion of the pyrazole carbonitrile derivative increased linearly with increase in the amount of catalyst from 50–100 mg (Table 2, entry 1–3). With further increase in the amount of catalyst, the product yield was not increased, so 100 mg of the catalyst was found to be the optimum amount of catalyst for the desired reaction. The temperature effect played a vital role in the synthesis of pyrazole carbonitriles. The reaction was unsuccessful at room temperature while increasing it upto 80 °C product formation also increased linearly and completed at 80 °C (Table 3). As indicated in Table 3, the effect of increasing temperature (from 25 to 80 °C) is directly related to the improved yield of the product. Upon increasing the temperature further, the reaction afforded lesser yield of product. Thus, 80 °C was found to be the optimum temperature for the reaction.
Table 1 Effect of various reaction media for the model reactiona
Entry |
Catalyst |
Condition |
Timeb |
Yieldc |
Referencesd |
Reaction conditions: 4-chlorobenzaldehyde 1a (1 mmol), ethyl cyanoacetate 2 (1 mmol), phenylhydrazine 3a (1 mmol), in presence of different reaction media. Reaction progress monitored by TLC. Isolated yield. References of the catalysts used before for the similar kind of reactions under different reaction conditions. |
1 |
— |
Ethanol/reflux |
18 h |
— |
|
2 |
AcOH (10 mol%) |
Ethanol/reflux |
9 h |
56 |
42 |
3 |
H2SO4 (10 mol%) |
Ethanol/reflux |
8 h |
54 |
43 |
4 |
H2SO4–SiO2 (200 mg) |
Ethanol/reflux |
5 h |
62 |
44 |
5 |
H2SO4–ZrO2 (200 mg) |
Ethanol/reflux |
6 h |
58 |
45 |
6 |
H2SO4–Al2O3 (200 mg) |
Ethanol/reflux |
3.5 h |
70 |
46 |
7 |
H2SO4–cellulose (200 mg) |
Ethanol/reflux |
6.5 h |
60 |
47 |
8 |
H2SO4–zanthan (200 mg) |
Ethanol/reflux |
6 h |
56 |
48 |
9 |
H2SO4–PEG (200 mg) |
Ethanol/reflux |
5.2 h |
64 |
49 |
10 |
Na2WO3 (10 mol%) |
Ethanol/reflux |
4 h |
68 |
50 |
11 |
ATA (200 mg) |
Ethanol/reflux |
92 min |
76 |
Present work |
12 |
ATA–OSO3H(SATA) (100 mg) |
Ethanol/reflux |
15 min |
94 |
Present work |
13 |
FeCl3 (10 mol%) |
Ethanol/reflux |
6.2 h |
42 |
51 |
14 |
ZnCl2 (10 mol%) |
Ethanol/reflux |
6 h |
40 |
52 |
15 |
ZnO (10 mol%) |
Ethanol/reflux |
7 h |
24 |
53 |
16 |
MgO (10 mol%) |
Ethanol/reflux |
7 h |
26 |
54 |
17 |
SATA (100 mg) |
Solvent free |
10 h |
Traces |
|
18 |
SATA (100 mg) |
Water |
7 h |
52 |
|
19 |
SATA (100 mg) |
Methanol |
7 h |
78 |
|
20 |
SATA (100 mg) |
Isopropanol |
7.2 h |
72 |
|
21 |
SATA (100 mg) |
Acetonitrile |
7 h |
56 |
|
22 |
SATA (100 mg) |
Hexane |
8 h |
52 |
|
23 |
SATA (100 mg) |
Toluene |
7.2 h |
56 |
|
24 |
SATA (100 mg) |
Ethylene glycol |
7 h |
48 |
|
Table 2 Effect of catalyst loading on the model reactiona
Entrya |
Catalyst loading (mg) |
Timeb (min) |
Yieldc (%) |
Reaction conditions: 4-chlorobenzaldehyde 1a (1 mmol), ethyl cyanoacetate 2 (1 mmol), phenylhydrazine 3a (1 mmol), in presence of different amounts of SATA in ethanol under reflux condition. Reaction progress monitored by TLC. Isolated yield. |
1 |
30 |
52 |
70 |
2 |
50 |
45 |
78 |
3 |
100 |
15 |
94 |
4 |
150 |
15 |
88 |
5 |
200 |
15 |
88 |
Table 3 Effect of temperature on model reactiona
Entry |
Temperature (°C) |
Timeb |
Yieldc (%) |
Reaction conditions: 4-chlorobenzaldehyde 1a (1 mmol), ethyl cyanoacetate 2 (1 mmol), phenylhydrazine 3a (1 mmol), in presence of SATA (100 mg) in 5 mL ethanol/reflux. Reaction progress monitored by TLC. Isolated yield. |
1 |
25 |
24 h |
No reaction |
2 |
45 |
1.5 h |
40 |
3 |
65 |
60 min |
75 |
4 |
75 |
45 min |
82 |
5 |
80 |
15 min |
94 |
6 |
100 |
24 min |
90 |
In the light of green chemistry, it was vital to certify our procedure as eco-friendly, which was accomplished in terms of atom economy (AE), reaction mass efficiency (RME), overall efficiency (OE), carbon efficiency (CE), process mass intensity (PMI), E-factor and solvent intensity (SI).55–58 Details of reactants and products used for metric calculations are given in ESI.† The calculated values for AE (76–83%), OE (91–94%), CE (83–89%), E-factor (19–12), SI (19–12) and PMI (20–13) are summarized in (Table 4) which endorse this presented method as green and sustainable.
Table 4 Summary of green metrics calculations of the synthesized compounds (4a–l)
Entry |
% yield |
% AEa |
% CEb |
% RMEc |
% OEd |
PMIe |
SIf |
E-factorg |
% AE: percentage atom economy. % CE: percentage carbon efficiency. % RME: percentage reaction mass efficiency. % OE: percentage overall efficiency. PMI: process mass intensity. SI: solvent intensity. E-factor. Catalyst amount was not used in the calculations because of its reusability.47 Calculations up to the crude product in all cases. |
4a |
94 |
81.73 |
88.88 |
76.84 |
94.01 |
15.47 |
14.17 |
14.47 |
4b |
94 |
82.25 |
88.88 |
77.41 |
94.11 |
14.97 |
13.68 |
13.97 |
4c |
94 |
82.25 |
88.88 |
77.41 |
94.11 |
14.97 |
13.68 |
13.97 |
4d |
92 |
81.73 |
88.88 |
75.17 |
91.97 |
15.81 |
14.48 |
14.81 |
4e |
94 |
83.73 |
88.88 |
78.76 |
94.06 |
13.58 |
12.31 |
12.58 |
4f |
92 |
81.50 |
89.47 |
74.90 |
91.90 |
16.03 |
14.70 |
15.03 |
4g |
94 |
78.86 |
83.33 |
72.25 |
94.00 |
20.46 |
19.08 |
19.46 |
4h |
92 |
77.69 |
83.33 |
71.47 |
91.99 |
19.98 |
18.58 |
18.98 |
4i |
94 |
77.69 |
83.33 |
73.02 |
93.98 |
19.57 |
18.21 |
18.57 |
4j |
92 |
76.86 |
83.75 |
70.71 |
91.99 |
20.91 |
19.50 |
19.91 |
4k |
92 |
89.13 |
83.33 |
82.01 |
92.01 |
17.43 |
16.21 |
16.43 |
4l |
94 |
76.50 |
84.61 |
71.91 |
94.00 |
20.86 |
19.47 |
19.86 |
Catalytic reaction
After optimizing the reaction conditions, a variety of pyrazole carbonitriles were synthesized (Table 5) (Scheme 2). The results indicated that reaction proceeded smoothly in the presence of a catalyst (SATA) giving an excellent yield of products successfully within a shorter period of time.
Table 5 Synthesis of pyrazole carbonitrile derivatives in presence of SATA under reflux conditionsa
Entry |
1a–f |
Products |
Timeb (min) |
Yieldc (%) |
Reaction of aldehydes (1a–f) (1 mmol), ethylcyanoacetate (2) (1 mmol) and hydrazines (3a/3b) in presence of SATA (100 mg) in 5 mL ethanol/reflux. Reaction progress was monitored by TLC. Isolated yield. |
1 |
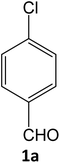 |
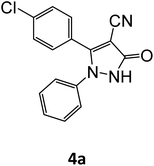 |
15 |
94 |
2 |
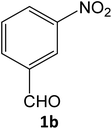 |
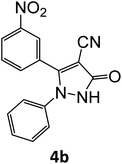 |
16 |
94 |
3 |
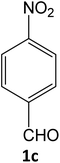 |
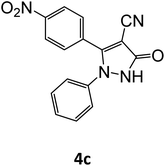 |
15 |
94 |
4 |
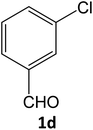 |
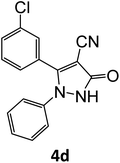 |
16 |
92 |
5 |
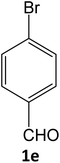 |
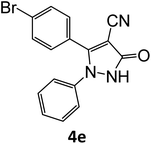 |
15 |
94 |
6 |
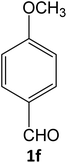 |
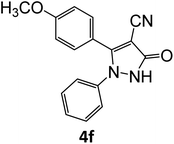 |
15 |
92 |
7 |
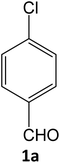 |
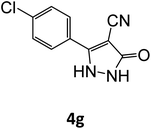 |
15 |
94 |
8 |
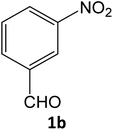 |
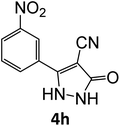 |
17 |
92 |
9 |
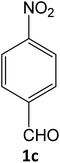 |
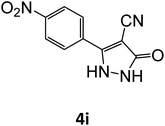 |
15 |
94 |
10 |
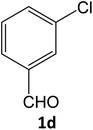 |
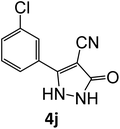 |
16 |
92 |
11 |
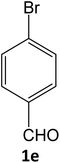 |
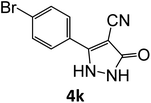 |
15 |
92 |
12 |
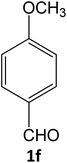 |
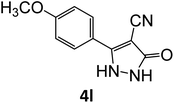 |
15 |
94 |
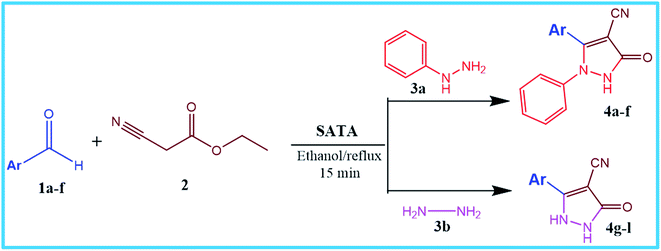 |
| Scheme 2 General scheme for the formation of pyrazole carbonitrile derivatives. | |
In order to demonstrate the advantage of our protocol, a comparison with reported available protocols in the literature was done (Table 6). In comparison to reported procedures, the present protocol proved competent, environmentally benign and practically more sound (Table 6). Moreover, catalyst can be easily separated at the end of reaction and has shown good reusability.
Table 6 Comparison of efficiency of sulphated alumina tungstic acid (SATA) with reported methods
Entry |
Catalyst/material |
Reaction condition |
Reaction medium |
Yield (%) |
Time |
Reference |
1 |
SATA |
Reflux |
Ethanol |
94 |
15 min |
Present work |
2 |
CuO/ZrO2 |
Stirring, 40 °C |
Water |
88 |
1.5 h |
59 |
3 |
Fe3O4@Si@MoO2 |
RT |
Solvent free |
88 |
30 min |
60 |
4 |
Ti(NMe2)2(pypyr)2 |
Reflux, 100 °C |
Toluene |
71 |
36 h |
61 |
5 |
(Bim)OH |
Grinding |
Water |
75 |
30 min |
62 |
6 |
Yb(PFO)3 |
120 °C |
Solvent free |
76 |
1.5 h |
63 |
Reusability and heterogenity of the catalyst
The recycling experiments were performed to explore the recyclability level of our catalytic scheme. The recyclability level was studied for the heterogeneous nature of the catalyst (Fig. 7). The recovered catalyst catalysed the formation of 4a successfully for five consecutive cycles with good activity. The morphology of the recovered catalyst was almost unchanged as shown by XRD and TEM analysis (Fig. 2c and 4b) respectively. Furthermore, to figure out the heterogeneity of the catalyst sulphated alumina tungstic acid (SATA), hot filtration test was accomplished. The model reaction containing 4-chlorobenzaldehyde (1a), ethyl cyanoacetate (2), phenylhydrazine (3a) and (SATA) (100 mg) in ethanol was refluxed for 13 min and filtered off while hot. Taking this filtrate as reaction mixture, the reaction was further continued under similar reaction conditions for 30 min and it was found that the reaction could not proceed further. Therefore, it was concluded that the catalyst was heterogeneous in nature.
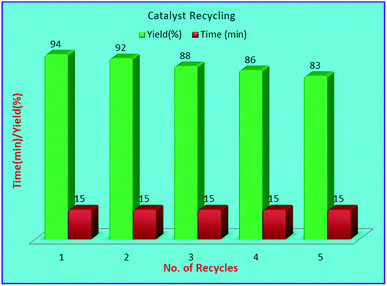 |
| Fig. 7 Recycling data of the catalyst. | |
Conclusion
Herein, a good catalytic system based on mesoporous alumina was synthesized. The heterogeneous catalyst displayed excellent activity for the synthesis of pyrazole carbonitriles. All the substrates could be easily converted to products with high yield. The distinguishing features of this method included easy separation of the catalyst, mild reaction conditions, enhanced reaction rates, cleaner reaction profiles, and easy product separation procedures, which make this method attractive. Furthermore, the method described produced negligible waste, with good to excellent values of green chemistry metrics (AE, CE, RME, OE, PMI, SI, and E-factor), and showed standard choice in this framework.
Experimental aspect
Preparation of mesoporous alumina (Al2O3) and alumina tungstic acid (ATA)
Mesoporous alumina was synthesized according to reported procedure64 with slight modifications. Cetrimonium bromide (CTAB) (0.2 mmol), aluminium sulphate (Al2(SO4)3·18H2O) (1 mmol), urea (CO(NH2)2) (4 mmol), and sodium tartrate (C4H4O6Na2) (0.7 mmol) were suspended in demineralized water for about 0.5 h until complete dissolution. The solution was then kept in an autoclave with Teflon lining with maintained temperature at 165 °C for 8 h. The white precipitate as formed, after allowing autoclave to cool was collected, washed carefully with distilled water, dried at 80 °C for 12 h and finally calcined at 550 °C for 3 h to get rid of the impurities.
Preparation of alumina tungstic acid (ATA)
Dropwise addition of thionyl chloride (SOCl2 20 g) to synthesized mesoporous alumina (20 g) in dry CH2Cl2 (50 mL) at ambient temperature with constant stirring was performed. HCl and SO2 gases were evolved and reaction was allowed to proceed for another 1 h. After that CH2Cl2 (solvent) was removed from the reaction mixture under suction to obtain alumina chloride. In next step mesoporous AlCl3 (6.00 g) as formed and Na2WO4 (7.03 g) were taken in solvent n-hexane (10 mL) and refluxed with stirring for 6 h. The solid as formed was filtered out from reaction mixture, washed with water, dried and stirred for another 1 h with 0.1 N HCl (40 mL) (Scheme 1). Finally, the solid was removed from the reaction mixture by filtration, washed several times with distilled water, and dried to obtain alumina tungstic acid (ATA).
Preparation of sulphated alumina tungstic acid (SATA)
Alumina tungstic acid (ATA) (2.5 g) was taken in a flat bottomed suction flask equipped with a dropping funnel, added CH2Cl2 (dry, 0.075 L) and stirred for 10 min. To the above reaction mixture, added dropwise chloro-sulfonic acid (1.75 mL) during 30 min. Upon complete addition, stirring of the reaction mixture was continued upto 90 min. HCl was removed under reduced pressure. Solid was filtered and thoroughly washed using CH2Cl2 as solvent and dried at 120 °C for 3 h to afford sulphated alumina tungstic acid (SATA).
General procedure for synthesis of pyrazole carbonitrile derivatives
Equimolar quantity of aldehyde (1a–e) ethyl cyanoacetate (2), hydrazine (3a–b) and catalyst (SATA) (100 mg) in 5 mL ethanol was refluxed for an appropriate time (Table 2). After completion of the reaction (monitored by TLC) the reaction mixture was filtered off to remove the solid catalyst, which was washed with ethanol (3 × 15 mL) and ethyl acetate (2 × 10 mL) for further use. The filtrate was evaporated under reduced pressure to afford product (4). The crude product (4) was purified by recrystallization using ethanol/DMSO as solvent.
Conflicts of interest
The authors declare no conflict of interest.
Acknowledgements
Authors provide special thanks to CST, UP, Lucknow, bearing ref. no. CST/SERPD/D-283 (dated, 14 May 2015) for financial support. We acknowledge DRS II (UGC, New Delhi), USIF, AMU, Aligarh, for SEM-EDX, TEM facilities, IIT Guwahati for XRD, BET analyses and SAIF Punjab for providing NMR and Mass spectra.
References
- W. F. Hölderich, J. Röseler, G. Heitmann and A. T. Liebens, Catal. Today, 1997, 37, 353–366 CrossRef.
- A. Corma, Chem. Rev., 1995, 95, 559–614 CrossRef CAS.
- A. Corma, Chem. Rev., 1997, 97, 2373–2420 CrossRef CAS PubMed.
- S. Namba, N. Hosonuma and T. Yashima, J. Catal., 1981, 72, 16–20 CrossRef CAS.
- I. Nowak and M. Ziolek, Chem. Rev., 1999, 99, 3603–3624 CrossRef CAS PubMed.
- M. A. Harmer, W. E. Farneth and Q. Sun, J. Am. Chem. Soc., 1996, 118, 7708–7715 CrossRef CAS.
- J. A. Bootsma and B. H. Shanks, Appl. Catal., A, 2007, 327, 44–51 CrossRef CAS.
-
(a) I. V Kozhevnikov, Chem. Rev., 1998, 98, 171–198 CrossRef PubMed;
(b) R. A. Rather and Z. N. Siddiqui, RSC Adv., 2019, 9, 15749–15762 RSC.
- M. E. Davis, Nature, 2002, 417, 813–821 CrossRef CAS PubMed.
- A. Corma and H. García, Chem. Rev., 2003, 103, 4307–4366 CrossRef CAS PubMed.
- A. Zheng, S. Liu and F. Deng, Chem. Rev., 2007, 117, 12475–12531 CrossRef PubMed.
- M. Choi, K. Na, J. Kim, Y. Sakamoto, O. Terasaki and R. Ryoo, Nature, 2009, 461, 246–249 CrossRef CAS PubMed.
- X. Zhang, Y. Zhao and Q. H. Yang, J. Catal., 2014, 320, 180–188 CrossRef CAS.
- J. C. Jiang, F. Gándara, Y. B. Zhang, K. Na, O. M. Yaghi and W. G. Klemperer, J. Am. Chem. Soc., 2014, 136, 12844–12847 CrossRef CAS PubMed.
- M. Hara, T. Yoshida, A. Takagaki, A. Takata, J. N. Kondo, S. Hayashi and K. Domen, Angew. Chem., Int. Ed., 2004, 43, 2955–2958 CrossRef CAS PubMed.
- E. Nikolla, Y. Román-Leshkov, M. Moliner and M. E. Davis, ACS Catal., 2011, 1, 408–410 CrossRef CAS.
- K. Nakajima, Y. Baba, R. Noma, M. Kitano, J. N. Kondo, S. Hayashi and M. Hara, J. Am. Chem. Soc., 2011, 133, 4224–4227 CrossRef CAS PubMed.
- M. Dusselier, P. V. Wouwe, A. Dewaele, P. A. Jacobs and B. F. Sels, Science, 2015, 349, 78–80 CrossRef CAS PubMed.
- T. Okuhara, Chem. Rev., 2002, 102, 3641–3666 CrossRef CAS PubMed.
- N. Mizuno, K. Yamaguchi and K. Kamata, Catal. Surv. Asia, 2011, 15, 68–79 CrossRef CAS.
- J. Song, Z.-F. Huang, L. Pan, J.-J. Zou, X. Zhang and L. Wang, ACS Catal., 2015, 5, 6594–6599 CrossRef CAS.
- B. A. Wasmi, A. A. Al-Amiery, A. A. H. Kadhum and A. B. Mohamad, J. Nanomater., 2014, 7, 2 Search PubMed.
- G. B. Payne and C. W. Smith, J. Org. Chem., 1957, 22, 1682–1685 CrossRef CAS.
- F. B. Cao, H. Jiang and G. Hong, Chin. J. Org. Chem., 2005, 25, 96–100 CAS.
- J. Ichihara, Tetrahedron Lett., 2001, 42, 695–697 CrossRef CAS.
- V. K. Singh and P. T. Deota, Synth. Commun., 1988, 18, 617–624 CrossRef CAS.
- P. T. Deota, R. Desai and V. B. Valodkar, J. Chem. Res., 1998, 9, 562–563 RSC.
- C. Hui, D. Wei-Lin, G. Ruihua, C. Yong, L. Hexing and F. Kangnian, Appl. Catal., A, 2007, 328, 226–236 CrossRef.
- A. F. Lee, J. A. Bennett, J. C. Manayil and K. Wilson, Chem. Soc. Rev., 2014, 43, 7887–7916 RSC.
- S. M. Vahdat, S. Khaksar, M. Akbari and S. Baghery, Arabian J. Chem., 2019, 12, 1515–1521 CrossRef CAS.
- S. K. Kundu and A. Bhaumik, ACS Sustainable Chem. Eng., 2015, 3, 1715–1723 CrossRef CAS.
-
(a) G. M. Ziarani, A. Badiei, N. Lashgari, T. Pourjafar and Z. Farahani, Bulg. Chem. Commun., 2014, 46, 719–723 Search PubMed;
(b) R. A. Rather and Z. N. Siddiqui, J. Organomet. Chem., 2018, 868, 164–174 CrossRef CAS.
- G. D. Yadav and J. J. Nair, Microporous Mesoporous Mater., 1999, 33, 1–48 CrossRef CAS.
- Y. Wang, D. Wang, M. Tan, B. Jiang, J. Zheng, N. Tsubaki and M. Wu, ACS Appl. Mater. Interfaces, 2015, 7, 26767–26775 CrossRef CAS PubMed.
- M. A. Zolfigol, M. Navazeni, M. Yarie and R. Ayazi-Nasrabadi, Can. J. Chem., 2017, 95, 1248–1252 CrossRef CAS.
- H. Sharghi and O. Asemani, Synth. Commun., 2009, 39, 860–867 CrossRef CAS.
- J. Kim, D. Lee, C. Park, W. So, M. Jo, T. Ok, J. Kwon, S. Kong, S. Jo and Y. Kim, ACS Med. Chem. Lett., 2012, 3, 678–682 CrossRef CAS PubMed.
- A. D. Cross, Introduction to Practical Infrared Spectroscopy, Butterworth, Washington D.C., 2nd edn, 1964 Search PubMed.
- X. Su, S. Chen and Z. Zhou, Appl. Surf. Sci., 2012, 258, 5712–5715 CrossRef CAS.
- N. Ahmed and Z. N. Siddiqui, J. Mol. Catal. A: Chem., 2014, 387, 45–56 CrossRef CAS.
- P. Kim, Y. Kim, C. Kim, H. Kim, Y. Park, J. H. Lee, I. K. Song and J. Yi, Catal. Lett., 2003, 89, 185–192 CrossRef CAS.
- H. Hügel, Molecules, 2009, 14, 4936–4972 CrossRef PubMed.
- S. Fustero, A. Simón-Fuentes and J. F. Sanz-Cervera, Org. Prep. Proced. Int., 2009, 41, 253–290 CrossRef CAS.
- A. R. Kiasat, F. Kazemi and K. Nourbakhsh, Phosphorus, Sulfur, Silicon Relat. Elem., 2004, 179, 569–573 CrossRef CAS.
- E. Kolvari, N. Koukabi, M. M. Hosseini, M. Vahidian and E. Ghobadi, RSC Adv., 2016, 6, 7419–7425 RSC.
- N. Ahmed and Z. N. Siddiqui, J. Mol. Catal. A: Chem., 2014, 394, 232–243 CrossRef CAS.
- J. Safari, S. H. Banitaba and S. D. Khalili, J. Mol. Catal. A: Chem., 2011, 335, 46–50 CrossRef CAS.
- B. S. Kuarm, J. V. Madhav, S. V. Laxmi and B. Rajitha, Synth. Commun., 2012, 42, 1211–1217 CrossRef CAS.
- R. H. Vekariya and H. D. Patel, RSC Adv., 2015, 5, 49006–49030 RSC.
- J. H. Clark, Acc. Chem. Res., 2002, 35, 791–797 CrossRef CAS PubMed.
- V. R. Mudumala, R. R. Chinthaparthi and T. J. Yeon, Tetrahedron, 2014, 70, 3762–3769 CrossRef.
- R. Konakanchi, S. Kankala and L. R. Kotha, Synth. Commun., 2018, 48, 1777–1785 CrossRef CAS.
- H. Sachdeva and R. Saro, Sci. World J., 2013, 680671 Search PubMed.
- M. Abaszadeh, M. Seifi and A. Asadipour, Synth. React. Inorg., Met.-Org., Nano-Met. Chem., 2016, 46, 512–517 CrossRef CAS.
- Green metrics reviews, see:
(a) F. Roschangar, R. A. Sheldon and C. H. Senanayake, Green Chem., 2015, 17, 752–768 RSC;
(b) J. Augé, Green Chem., 2008, 10, 225–231 RSC;
(c) C. Jimenez-Gonzalez, C. S. Ponder, Q. B. Broxterman and J. B. Manley, Org. Process Res. Dev., 2011, 15, 912–917 CrossRef CAS.
-
(a) X. Zhang, G. Dhawan, A. Muthengi, S. Liu, W. Wang, M. Legris and W. Zhang, Green Chem., 2017, 19, 3851–3855 RSC;
(b) P. Wadhwa, T. Kaur and A. Sharma, RSC Adv., 2015, 5, 44353–44360 RSC.
- C. Jiménez-González, D. J. Constable and C. S. Ponder, Chem. Soc. Rev., 2012, 41, 1485–1498 RSC.
- M. M. Khan, S. S. Khan, S. Shareef and S. C. Sahoo, RSC Adv., 2018, 8, 41892–41903 RSC.
- S. Maddila, S. Rana, R. Pagadala, S. Kankala, S. Maddila and S. B. Jonnalagadda, Catal. Commun., 2015, 61, 26–30 CrossRef CAS.
- J. Rakhtshah, S. Salehzadeh, E. Gowdini, F. Maleki, S. Baghery and M. A. Zolfigol, RSC Adv., 2016, 6, 104875–104885 RSC.
- A. A. Dissanayake and A. L. Odom, Chem. Commun., 2012, 48, 440–442 RSC.
- M. Srivastava, P. Rai and J. Singh, New J. Chem., 2014, 38, 302–307 RSC.
- L. Shen, S. Cao, N. Liu, J. Wu, L. Zhu and X. Qian, Synlett, 2008, 1341–1344 CAS.
- M. B. Yue, T. Xue, W. Q. Jiao, Y. M. Wang and M.-Y. He, Solid State Sci., 2011, 13, 409 CrossRef CAS.
Footnote |
† Electronic supplementary information (ESI) available. See DOI: 10.1039/c9ra09013d |
|
This journal is © The Royal Society of Chemistry 2020 |
Click here to see how this site uses Cookies. View our privacy policy here.