DOI:
10.1039/C9RA08651J
(Paper)
RSC Adv., 2020,
10, 1042-1049
A fluorescence immunoassay based on CdTe
:
Zn/ZnS quantum dots for the rapid detection of bacteria, taking Delftia tsuruhatensis CM’13 as an example†
Received
22nd October 2019
, Accepted 9th November 2019
First published on 11th December 2019
Introduction
Quantum dots (QDs) are nanocrystals made of semiconductor materials often composed of atoms from group II–VI or III–V elements in the periodic table and are defined as particles with physical dimensions smaller than the exciton Bohr radius (they have sizes in the range of 1–10 nm). Based on the quantum confinement effect, the electrons and holes in the interior of QDs are locked in a tiny three-dimensional space, so that the continuous energy band structure is converted into a discrete structure having molecular activity to emit fluorescence. QDs possess many unique and distinctive characteristics. For example, the emission spectrum can be adjusted by changing the size of QDs;1 the stability of fluorescence is good and the fluorescence intensity is 20 times that of rhodamine.2 Moreover, QDs exhibit wide excitation spectra and narrow emission spectra, due to which they can be used to achieve simultaneous detection; QDs also have good biocompatibility. They can be combined with some biomolecules to act as markers to detect living organisms.3–5 At present, QDs have received extensive attention in the biological, chemical, and optoelectronic fields.6–8
Cd-based QDs have become a hot research topic due to their higher fluorescence quantum yields.9,10 However, due to the disadvantages of core QDs (CdTe), such as aggregation, poor fluorescence stability, short shelf life, and easily lost fluorescence,1 they can be used on newly prepared materials briefly. A single-core QD nanomaterial is coated with other compounds to form core–shell QD. The presence of the shell reduces the surface defects and protects the core from decomposition under photoexcitation, thereby increasing the fluorescence yield, fluorescence intensity, and stability of mononuclear quantum dots.11 With the development of nanomaterials by researchers, a new type of doped QDs could be synthesized.12 Doped QDs refer to nanocrystals formed by adding a small amount of transition metal ions or rare earth ions during the synthesis of QDs. The excitation wavelength of the synthesized doped QDs depends mainly on the band gap of the host material and the wavelength of the luminescence is related to the doped atoms selected. The advantage of doping QDs is that they have a large Stokes displacement, which helps prevent the phenomenon of self-priming. Therefore, CdTe
:
Zn/ZnS-doped QDs synthesized in this study have a longer fluorescence lifetime, stronger photostability, and higher fluorescence efficiency compared with traditional CdTe/ZnS QDs, which indicates that doped QDs are attractive fluorescent probes for use in the analytical detection field.
Fluorescent immunoassay (FLISA) is an efficient high-throughput detection method. The principles of its operation and detection are similar to ELISA. The difference between ELISA and FLISA is that ELISA uses enzyme-labeled secondary antibodies to determine the absorbance of the substrate, while FLISA employs a fluorescent substance as the label to measure the fluorescence intensity. The QDs-based FLISA is being widely studied nowadays. Zhang et al. used functionalized Cd-doped ZnO QDs to covalently couple with bisphenol A (BPA) antibody to detect BPA by competitive FLISA. The effective linear working range was 20.8–330.3 ng mL−1 and the LOD was 13.1 ng mL−1.13 Xiong et al. reported a new direct competition dcFLISA for the detection of ochratoxin A (OTA) by introducing large size polymer bead-loaded quantum dots (QBs). At optimal parameters, the linear range of dcFLISA for OTA detection was 0.05 pg mL−1–1.56 pg mL−1 with a maximum inhibitory concentration of 0.14 ± 0.04 pg mL−1 (n = 5).14 Yang et al. introduced FLISA for specific quantitative detection of bovine alpha-lactalbumin (α-La) in dairy products. It was found that there was a good linear relationship between the inhibition efficiency and the logarithm of α-La's concentrations after optimization. The α-La's concentrations ranged from 0.1–1000 ng mL−1, the half maximal inhibitory concentration (IC50) was 0.03 μg mL−1, and LOD was 0.1 ng mL−1, showing that the established FLISA had sensitivity and was successfully applied to the detection of α-La in commercial dairy products.15 There are also other methods using QDs such as impedance,16 ELISA,17 test strips,18,19 and PCR for detecting bacteria.20,21 Above all, FLISA has been widely used for detection in many fields, while the application of FLISA in microbiological detection is correspondingly rare.
In this study, we successfully established FLISA to detect D. tsuruhatensis CM13 with the help of the high fluorescence, good stability, and good biocompatibility of QDs. Compared with the traditional ELISA (Fig. 1), our new FLISA provided rapid and accurate detection method of food spoilage bacteria, ensuring the quality and safety of food during storage and transportation.
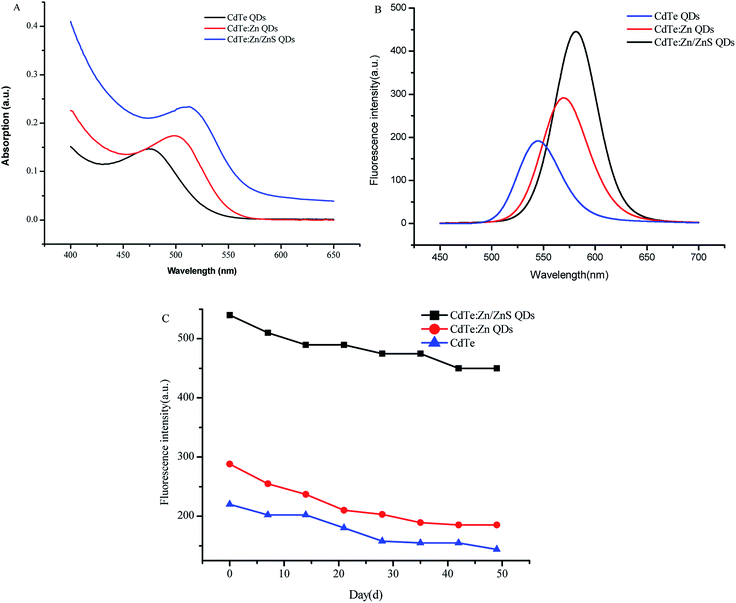 |
| Fig. 1 The absorption spectrum (A) of CdTe, CdTe : Zn, and CdTe : Zn/ZnS QDs was obtained from 400 nm to 650 nm and the fluorescence emission spectrum (B) was tested in the range of 450 nm to 700 nm. (C) Comparisons of stability between CdTe : Zn/ZnS QDs, CdTe : Zn QDs, and CdTe QDs. | |
Experiment
Materials and reagents
The D. tsuruhatensis CM13 used in the experiments were isolated and purified from eggs purchased from the Jiufengshan breeding site. Other strains were provided by Center for Disease Control and Prevention of HuBei, WuHan. Sodium borohydride (NaHB4) and cadmium chloride (CdCl2) were purchased from Sinopharm Chemical Reagent Co., Ltd; 1-(3-dimethylaminopropyl)-3-ethylcarbodiimide hydrochloride (EDC·HCl) and N-hydroxysuccinimide (NHS) were obtained from Shanghai Crystal Pure Reagent Co., Ltd. Goat anti-rabbit IgG-HRP and Goat anti-rabbit IgG were provided by Hubei Jing Mao Biotech Co., Ltd and Boster Biological Technology Co., Ltd, respectively.
Preparation of water-soluble CdTe
:
Zn/ZnS QDs
One hundred mg NaHB4 was quickly added into the reaction flask wrapped with aluminum membrane with 3 mL of ultrapure water and 63.8 mg Te powder with the needle inserted on the lid to discharge hydrogen, then put in a water bath at 40 °C for 1 h until the solution was clear purple with white precipitate to obtain sodium hydrogen telluride precursor.
After a series of optimization (ESI 1†), we took 91.34 mg of CdCl2 and 43.9 mg of zinc acetate, and dissolved them in 100.0 mL ultrapure water. Then, 78 μL of MPA was added subsequently and the pH value was adjusted to 7.5 by 0.1 mol L−1 sodium hydroxide. After aerating with nitrogen under magnetic stirring for 30 min, sodium hydrogen telluride precursor was added and heated at 100 °C for 2.5 h to prepare CdTe
:
Zn QDs.
Fifty mL CdTe
:
Zn QDs solution in a four-neck flask was filled with nitrogen. After a series of optimization (ESI 2†), we decided to magnetically stir for 30 min and heat at 85 °C, then quickly added 0.5 mL zinc acetate solution (0.1 mol L−1) and 0.5 mL of sulfur the acetamide solution (0.1 mol L−1), finally refluxing for 3.5 h to obtain CdTe
:
Zn/ZnS QDs.
Preparation of D. tsuruhatensis CM13 polyclonal antibody
D. tsuruhatensis CM13 polyclonal antibody was prepared by immunizing New Zealand white rabbits by multiple-site subcutaneous injection, using heat-inactivated D. tsuruhatensis CM13 with initial immune dose of 107 CFU mL−1 (1 mL), immunizing the rabbits with a dose of 108 CFU mL−1 (1 mL) every 2 weeks for three months, and collecting the blood from the ear vein on the seventh day after each immunization to measure titers by indirect ELISA. The titer of D. tsuruhatensis CM13 polyclonal antibody was 1
:
12
800 after purification and the molecular weight was 148 kD by sodium dodecyl sulfate polyacrylamide gel electrophoresis. Our animal experiment was approved by Huazhong Agricultural University and carried out in strict compliance with the guidelines of China Experimental Animal Welfare Ethics Committee.
Conjugation of CdTe
:
Zn/ZnS QDs and antibody
Purification of CdTe
:
Zn/ZnS QDs. With magnetic stirring, the ethanol solution was slowly added to the prepared CdTe
:
Zn/ZnS QDs solution until the turbidity appeared, continuing to stir for 5 min, and centrifuged at 5000 rpm for 5 min. The supernatant was removed and the procedure was repeated three times. Then, the precipitate was re-dissolved with ultrapure water and stored under dark.We employed amino acid-thiol covalent method to conjugate CdTe
:
Zn/ZnS QDs with antibody after optimizing (ESI 3†). 120 μL of CdTe
:
Zn/ZnS QDs solution was mixed with EDC·HCl (80 μL, 0.05 mol L−1) and NHs (60 μL, 0.05 mol L−1), and then, 720 μL of MES buffer (pH 5.5) was added. After standing for 30 min avoiding light at room temperature and centrifuging the mixed solution at 12
000 rpm for 2 min, the precipitate was re-dissolved in borate buffer (pH 9.0). Then, 120 μL of the antibody (1 mg mL−1) was added and reacted at 37 °C in a shaker for 2 h under dark. The mixed solution was transferred to 4 °C overnight. In the following day, centrifugation of the solution at 12
000 rpm for 30 min was done and the precipitates were stored in PBS buffer (0.01 mol L−1, pH 7.4) at 4 °C in the dark.
Established of FLISA for the detection of D. tsuruhatensis CM13
The operation of FLISA was similar to ELISA. Firstly, a certain concentration of antigen (108 CFU mL−1) was centrifuged and washed three times. Secondly, the blocking solution (10% BSA, 200 μL) was added at 37 °C for 1.5 h, centrifuged, and washed 3 times. Then, rabbit serum was added and incubated at 37 °C for 1 h, centrifuged, and washed 3 times. All of the above conditions have been properly optimized (ESI 4†). After that, CdTe
:
Zn/ZnS QDs-labeled goat anti-rabbit IgG was added and incubated at 37 °C for 1 h, centrifuged, and washed 5 times. Finally, the precipitates were dissolved in PBS and transferred to a black clear bottom 96-well plate measured by a multi-functional microplate reader. The excitation wavelength was 528 nm. The measurement method was bottom reading.
Results and discussion
Characterization of the QDs
The fluorescence emission spectra and UV absorption spectra of the QDs are shown in Fig. 1. From the emission spectra of QDs, the fluorescence emission peaks were about 545, 565, and 578 nm, respectively. The fluorescence intensity of CdTe
:
Zn/ZnS QDs is 140% higher than that of CdTe QDs. The full wavelength at half maximum (FWHM) of the emission spectrum was about 45 nm, which indicated that the prepared CdTe
:
Zn/ZnS QDs solution had better chromatic dispersion. In addition, the UV absorption spectra showed that the maximal absorption of CdTe was approximately 475 nm. The CdTe
:
Zn and CdTe
:
Zn/ZnS QDs had shifted their peaks at 500 nm and 525 nm, respectively. The stability of the QDs is shown in Fig. 1C, which have a strong fluorescence signal. Additionally, Fig. 2 shows the transmission electron microscopic images of the QDs. It could be seen that CdTe and CdTe
:
Zn QDs were aggregated easily. However, CdTe
:
Zn/ZnS QDs were composed of linear crystals with less agglomeration, uniform distribution, and a particle size of approximately 3 nm.
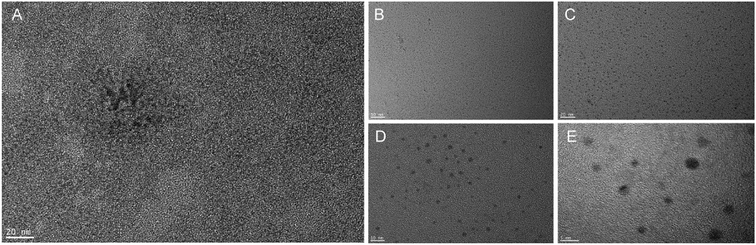 |
| Fig. 2 TEMs of CdTe : Zn QDs (A) and CdTe : Zn/ZnS QDs (B–E). Note: (B–E) were CdTe : Zn/ZnS QDs under 50 nm, 20 nm, 10 nm, 5 nm field of vision, respectively. | |
The characterization results demonstrated that the prepared core–shell QDs doped Zn has good spectral properties and aqueous dispersion that can be utilized as fluorescent probes in bioanalysis.
Conjugation of CdTe
:
Zn/ZnS QDs and antibody
The conjugation of the antibody and CdTe
:
Zn/ZnS QDs was observed by agarose gel electrophoresis. The results are shown in Fig. 3. Lanes 1–4 were added corresponding to CdTe
:
Zn/ZnS QDs, antibody, CdTe
:
Zn/ZnS QDs-antibody, and PBS, respectively. From Fig. 3, we could find that the CdTe
:
Zn/ZnS QDs were conjugated with the antibody because of slower migration with increased molecular weight. The emission spectrum was also detected. Compared to QDs, the antibody-conjugated product shows 27.65% lower fluorescence intensity in Fig. 4.
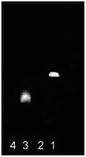 |
| Fig. 3 Agarose gel fluorescent spectra of CdTe : Zn/ZnS QDs before and after conjugating with antibody. Note: lane 1-CdTe : Zn/ZnS QDs; lane 2-antibody; lane 3-CdTe : Zn/ZnS QDs-antibody, lane 4-PBS. | |
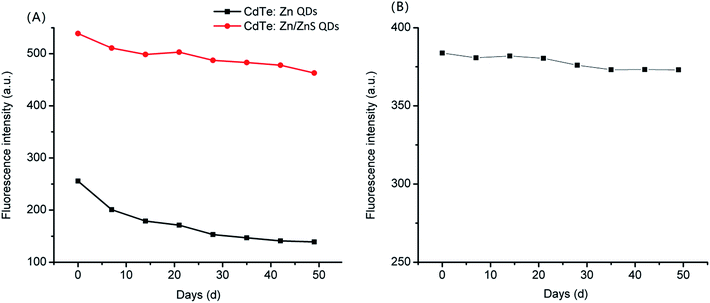 |
| Fig. 4 Stability of CdTe : Zn/ZnS QDs and CdTe : Zn QDs (A); stability of CdTe : Zn/ZnS QDs-antibody (B). | |
Activity of antibody after coupling with CdTe
:
Zn/ZnS QDs
The activity of the antibody coupled to CdTe
:
Zn/ZnS QDs was determined by dot blotting. 1 μL, 5 μL, 10 μL, and 15 μL of antigen solution (107 CFU mL−1) were added to the nitrocellulose membrane (b–e) and the PBS buffer was the blank control (a). The results are shown in Fig. 5. It could be seen that more the amount of antigen added, the higher the brightness of the spot under UV imaging. On the other hand, the weaker light in the blank area was caused by non-specific adsorption. It could be inferred that the antibody was successfully coupled to CdTe
:
Zn/ZnS QDs and the antibody still retained its biological activity after being conjugated with CdTe
:
Zn/ZnS QDs (Fig. 6).
 |
| Fig. 5 The ultraviolet imagery of spots mark. Note: (a) was PBS; (b)–(e) were expressing the amount of antigen were 1 μL, 5 μL, 10 μL, and 15 μL, respectively. | |
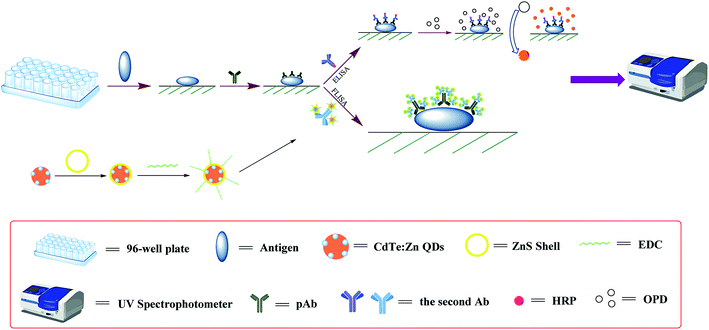 |
| Fig. 6 A schematic diagram of detection principles of ELISA and FLISA, respectively. | |
Detection of D. tsuruhatensis CM13 by ELISA and FLISA
The FLISA method is a kind of detection method similar to the ELISA method; they have similar detection principles and operation procedures. The difference between the FLISA method and the ELISA method is that the FLISA method eliminates the step of coloration of the coated antigen and the substrate, which greatly saves time. In addition, the ELISA method is based on the OPD color reaction, the OD value at 492 nm to reflect the amount of the antigen, FLISA is used to measure the antigen by measuring the fluorescence intensity of the CdTe
:
Zn/ZnS QDs-labeled goat anti-rabbit IgG-polyclonal antibody-antigen.
In this study, D. tsuruhatensis CM13 was detected by ELISA and FLISA in Fig. 7, respectively. The LOD by ELISA and FLISA were 5
log CFU mL−1 (with the linear correlation of 105 CFU mL−1–108 CFU mL−1) and 3.097
log CFU mL−1 (with the linear correlation of 103 CFU mL−1–108 CFU mL−1), respectively. The FLISA method was 80 times more sensitive than the ELISA.
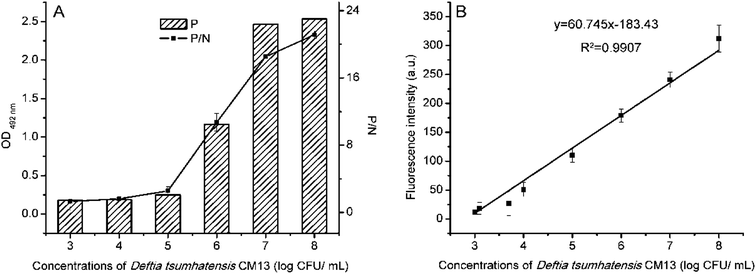 |
| Fig. 7 Detection limits of ELISA (A) and FLISA (B) for detecting D. tsuruhatensis CM13, respectively. | |
Artificial simulation experiments
In artificial simulation experiments, low-fat milk was diluted 10-fold. With a series of concentrations of D. tsuruhatensis CM13 mixed, it was detected by ELISA and FLISA in Fig. 8, respectively. In this experiment, LOD of D. tsuruhatensis of FLISA (4.398
log CFU mL−1) was about 40 times more sensitive than ELISA (6
log CFU mL−1).
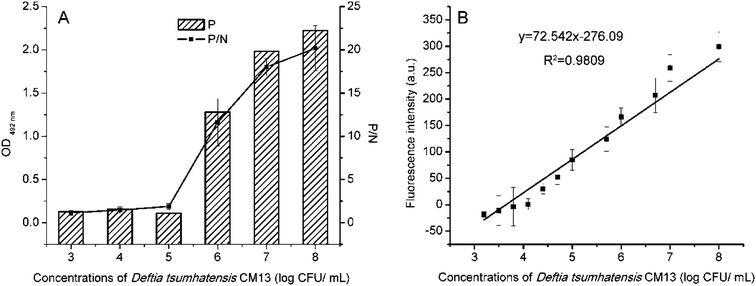 |
| Fig. 8 Detection limits of ELISA (A) and FLISA (B) for detecting D. tsuruhatensis CM13 in artificial contamination test. | |
Stability of the FLISA method
In the FLISA method, the stability of FLISA was decided by CdTe
:
Zn/ZnS QDs-labeled goat anti-rabbit IgG. When storing for 74 d, the conjugates showed more severe sedimentation, which may be due to the agglomeration of CdTe
:
Zn/ZnS QDs-antibody conjugates after long-term standing. When storing for 102 d, the CdTe
:
Zn/ZnS QDs CdTe
:
Zn/ZnS QDs-labeled goat anti-rabbit IgG became dark and the fluorescence disappeared. This may be due to the mix of oxygen at the same time that the CdTe
:
Zn/ZnS QDs-antibody conjugates with the agglomerate, which oxidized the CdTe
:
Zn/ZnS QDs and became dark; thus, the fluorescence disappeared.
D. tsuruhatensis CM13 was detected by FLISA every week. As shown in Table 1, when the detection reagents of FLISA method were stored for 35 d, the LOD was changed to 3.699
log CFU mL−1. When storing for 81 d, the LOD was changed to 4
log CFU mL−1. If stored for 88 d, the LOD was 5.989
log CFU mL−1. It could be found that with the extension of storage time, the LOD of D. tsuruhatensis CM13 was reduced.
Table 1 Stability of FLISA for the detection of D. tsumhatensis CM13a
Fluorescence intensity (a.u.) |
Day |
0 |
7 |
14 |
21 |
28 |
35 |
42 |
49 |
59 |
66 |
74 |
81 |
88 |
95 |
“—” expressed that the fluorescence intensity was lower than 0. |
Concentrations (log CFU mL−1) |
5 |
87 |
87 |
77 |
69 |
53 |
62 |
68 |
69 |
56 |
46 |
28 |
29 |
12 |
4 |
4 |
53 |
51 |
44 |
34 |
27 |
28 |
34 |
35 |
30 |
35 |
12 |
22 |
16 |
— |
3.699 |
21 |
29 |
29 |
14 |
14 |
11 |
8 |
13 |
10 |
7 |
4 |
4 |
— |
— |
3.098 |
12 |
11 |
5 |
3 |
3 |
7 |
— |
— |
— |
— |
— |
— |
— |
— |
Comparison of the FLISA method with other detection methods
At present, metabolic methods, molecular biology methods, and immunological methods are popular for detecting microorganisms. From Table 2, the LOD of ELISA, one of the typical immunological method, is about 103–106 CFU mL−1. In addition, our study used also ELISA to detect D. tsuruhatensis CM13 and the LOD was 105 CFU mL−1. In real detection, the microorganisms just contaminate most food at low levels, while the lower LOD of ELISA cannot detect microorganisms directly without enrichment. It may result in negative results or excessive detection time and limit wide application in food companies and actual testing. The immunochromatographic test strip is one of the immunoassay methods. The most widely used is colloidal gold immunochromatographic test strips. The LOD is generally 105–106 CFU mL−1. However, with the development of immunochromatographic test strips, various fluorescent substances (such as quantum dots) have also appeared as markers to detect microorganisms, so that the detection limit is 10–100 times higher than that of colloidal gold (up to 104 CFU mL−1).5 On the other hand, the fluorescence of QDs will reduce or disappear due to oxidation during storage, leading to the poor stability of the test strips (effect 30 days only). PCR and metabolic methods have both lower LOD, especially PCR, where the LOD of the microorganisms can be up to 102 CFU mL−1 but the process of PCR to detect microorganisms, such as increasing bacteria, extracting DNA, amplification, and electrophoresis, are necessary for low bacterial concentration. Also, the operation is complicated. Metabolic methods are usually used to determine the total number of microorganisms. ATP method, which is one of the metabolic methods, is relatively mature and widely used. It also has good sensitivity in detecting the total number of microorganisms, while the ATP from different types of microorganisms is same, so the result is sometimes not very accurate. An impedimetric biosensing technique, which is an electrochemical method, offers several advantages such as good sensitivity, miniaturization potential, and mass production.23,24 However, this technology is costly and technically strong, and its application is difficult to popularize. In this study, we successfully established a FLISA method to detect D. tsuruhatensis CM13; the LOD was about 1.25 × 103 CFU mL−1, which was about 80 times more sensitive than the ELISA method. In addition, the FLISA method was simple, had a short detection time (about 4 h), low cost, high sensitivity, and could be used to directly detect food. But some samples with 15% salt (NaCl), 10% sugar (glucose), 8% amino acid (L-Met), or 6% protein (whey protein), the detection must be performed after suitable dilution (ESI 6†). It indicates that the FLISA method has a wide range of applications. Finally, immunofluorescence assay kit method based on doped QDs has a better stability than fluorescent immunochromatographic test strips. When the reagents of FLISA were stored for about 3 months, the LOD of D. tsuruhatensis CM13 could still be up to 105 CFU mL−1.
Table 2 Detection limits/detection ranges of detection methods for microorganisms
Detection methods |
Objects |
Detection limit/detection range |
Total time |
Ref. |
FLISA |
S. typhimurium |
3 × 103 CFU mL−1 |
4 h |
Study support (ESI 5) |
ELISA |
S. typhimurium |
103–104 CFU mL−1 |
>1 d |
Wilanee et al. 201117 |
Immunochromatographic test strip |
S. choleraesuis |
5 × 105 CFU mL−1 |
20 min |
Xia et al. 201618 |
PCR |
S. typhimurium |
102 CFU mL−1 |
12–18 h |
Wang et al. 201820 |
ATP bioluminescence |
Salmonella |
103 CFU mL−1 |
20 min |
Lee et al. 201722 |
Impedance method |
S. typhimurium |
7.6 × 101 CFU mL−1 |
1 h |
Settu et al. 201516 |
Conclusion
In this study, stable and high PLQY CdTe
:
Zn/ZnS novel doped QDs were prepared by water synthesis method. D. tsuruhatensis CM13 extracted from foods were used as the research objects to establish a fluorescence immunoassay (FLISA), thus providing a new detection method for the rapid detection of microorganisms. The whole detection time was less than that of ELISA and this method would be a new protocol for the detection of microorganisms.
Conflicts of interest
There are no conflicts to declare.
Acknowledgements
This research is supported by the Fundamental Research Funds for the Central Universities (Program No. 2662019PY067).
References
- J. H. Bang and P. V. Kamat, ACS Nano, 2009, 3, 1467–1476 CrossRef CAS PubMed.
- C. Shi, X. Y. Huang, C. Q. Dong, H. J. Chen and J. C. Ren, Chin. Chem. Lett., 2009, 20, 1119–1122 CrossRef CAS.
- Y. Li, R. Afrasiabi, F. Fathi, N. Wang, C. Xiang, R. Love, Z. She and H.-B. Kraatz, Biosens. Bioelectron., 2014, 58, 193–199 CrossRef CAS PubMed.
- O. Minikh, M. Tolba, L. Brovko and M. Griffiths, J. Microbiol. Methods, 2010, 82, 177–183 CrossRef CAS PubMed.
- Z. Yu, H. Lv and D. Tang, Mater. Lett., 2018, 210, 207–210 CrossRef CAS.
- J. Yu, J. Su, J. Zhang, X. Wei and A. Guo, RSC Adv., 2017, 7, 17819–17823 RSC.
- A. Yang, Y. Zheng, C. Long, H. Chen, B. Liu, X. Li, J. Yuan and F. Cheng, Food Chem., 2014, 150, 73–79 CrossRef CAS PubMed.
- J. Zhang, S.-Q. Zhao, K. Zhang and J.-Q. Zhou, Chemosphere, 2014, 95, 105–110 CrossRef CAS PubMed.
- N. Gaponik, in Cadmium telluride quantum dots: advances and applications, CRC Press, Boca Raton, Florida, 2013, pp. 61–86 Search PubMed.
- J. Donegan and Y. Rakovich, Cadmium telluride quantum dots: advances and applications, Pan Stanford, 2016 Search PubMed.
- A. Samanta, Z. Deng and Y. Liu, Langmuir, 2012, 28, 8205–8215 CrossRef CAS PubMed.
- F. Di Nardo, L. Anfossi, C. Giovannoli, C. Passini, V. V. Goftman, I. Y. Goryacheva and C. Baggiani, Talanta, 2016, 150, 463–468 CrossRef CAS PubMed.
- Z. Zhang, X. Xia, X. Xiang, F. Huang and L. Han, Sens. Actuators, B, 2018, 257, 1–8 CrossRef CAS.
- S. Xiong, Y. Zhou, X. Huang, R. Yu, W. Lai and Y. Xiong, Anal. Chim. Acta, 2017, 972, 94–101 CrossRef CAS PubMed.
- C. Yang, G. Feng, S. Wang, J. Dai, Y. Zhang and S. Zhou, Chem. Phys. Lett., 2017, 684, 409–413 CrossRef CAS.
- K. Settu, C.-J. Chen, J.-T. Liu, C.-L. Chen and J.-Z. Tsai, Biosens. Bioelectron., 2015, 66, 244–250 CrossRef CAS PubMed.
- W. Chunglok, D. K. Wuragil, S. Oaew, M. Somasundrum and W. Surareungchai, Biosens. Bioelectron., 2011, 26, 3584–3589 CrossRef CAS PubMed.
- S. Xia, Z. Yu, D. Liu, C. Xu and W. Lai, Food Control, 2016, 59, 507–512 CrossRef CAS.
- Z. Baoqing, L. Taobo, Z. Zhongxu, L. Rui, L. Fan and X. Hengyi, J. Dairy Sci., 2017, 100, 8804–8813 CrossRef PubMed.
- M. Wang, J. Yang, Z. Gai, S. Huo, J. Zhu, J. Li, R. Wang, S. Xing, G. Shi and F. Shi, Int. J. Food Microbiol., 2018, 266, 251–256 CrossRef CAS PubMed.
- D.-B. Wang, B. Tian, Z.-P. Zhang, J.-Y. Deng, Z.-Q. Cui, R.-F. Yang, X.-Y. Wang, H.-P. Wei and X.-E. Zhang, Biosens. Bioelectron., 2013, 42, 661–667 CrossRef CAS PubMed.
- J. Lee, C. Park, Y. Kim and S. Park, BioChip J., 2017, 11, 287–293 CrossRef CAS.
- T. Wen, R. Wang, A. Sotero and Y. Li, Sensors, 2017, 17, 1973 CrossRef PubMed.
- I. Palchetti and M. Mascini, Anal. Bioanal. Chem., 2008, 391, 455–471 CrossRef CAS PubMed.
Footnotes |
† Electronic supplementary information (ESI) available. See DOI: 10.1039/c9ra08651j |
‡ Yao Ruan and Huanhuan Xu equally contributed to this study. |
|
This journal is © The Royal Society of Chemistry 2020 |
Click here to see how this site uses Cookies. View our privacy policy here.