Dimerization of conserved ascaroside building blocks generates species-specific male attractants in Caenorhabditis nematodes†
Received
17th April 2020
, Accepted 23rd June 2020
First published on 24th June 2020
Abstract
Comparative ascaroside profiling of Caenorhabditis nematodes using HPLC-ESI-(−)-MS/MS precursor ion scanning revealed a class of highly species-specific ascaroside dimers. Their 2- and 4-isomeric, homo- and heterodimeric structures were identified using a combination of HPLC-ESI-(+)-HR-MS/MS spectrometry and high-resolution dqf-COSY NMR spectroscopy. Structure assignments were confirmed by total synthesis of representative examples. Functional characterization using holding assays indicated that males of Caenorhabditis remanei and Caenorhabditis nigoni are exclusively retained by their conspecific ascaroside dimers, demonstrating that dimerization of conserved monomeric building blocks represents a yet undescribed mechanism that generates species-specific signaling molecules in the Caenorhabditis genus.
Introduction
Chemical communication in nematodes is mediated by ascarosides, glycolipids of the 3,6-dideoxy-arabino-aldohexose L-ascarylose linked to a wide range of homologous aglycones derived from the peroxisomal β-oxidation cycle (Fig. 1).1–8
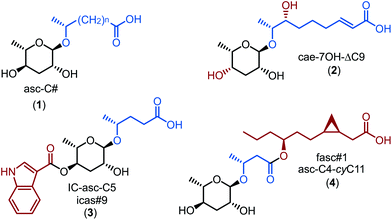 |
| Fig. 1 General structure of common ascarosides (1) along with species-specific derivatives from C. nigoni (2 and 3) and C. remanei (4); L-ascarylose unit in black, aglycone moiety in blue, and species-specific modifications in red. | |
Ascaroside signalling is widely conserved in nematodes9 and modulates a large diversity of biological functions.7,8 Over the past decade the development of sensitive ascaroside-selective screens based on characteristic fragment ions observed during electrospray ionization-tandem mass spectrometry (ESI-MS/MS)10,11 or electron ionization-mass spectrometry (EI-MS)12 along with comparative analysis of wild-type and peroxisomal β-oxidation mutant metabolomes13–15 has facilitated the identification of several hundreds of ascaroside structures, most of which have not yet been characterized with respect to their potential biological functions. Systematic bioassays with ascarosides, while limited in number and scope, established that even minor changes in molecular structure dramatically impact their biological activities,16 which demonstrates that ascaroside signalling represents a complex “chemical language”. Comparative analysis of several closely related Caenorhabditis species revealed that most simple ascarosides are highly conserved. Short chain ascarosides carrying (ω–1)-linked odd numbered C5, C7, C9, and C11 acyl chains as aglycones (1) are particularly abundant and widely distributed.17 These common ascarosides serve as scaffolds for species-specific modifications including the hydroxylation of the aglycone17,18 and epimerization of the L-ascarylose unit (e.g. 2),19 as well as the attachment of additional building blocks from diverse primary metabolic pathways to generate complex modular libraries (e.g.3).13,15,20–22 Here we show that homo- and heterodimerization of conserved ascaroside building blocks represents another mechanism that creates species-specific signalling molecules in the Caenorhabditis.
Results and discussion
Comparative ascaroside profiling reveals species-specific dimers
Comparative ascaroside profiling of the nematode culture supernatants (the exometabolomes) of Caenorhabditis nigoni and Caenorhabditis remanei (Fig. 2) using HPLC-ESI-(−)-MS/MS precursor ion scanning for the highly characteristic fragment ion at m/z 73.0 [C3H5O2]10 revealed a diversity of common simple ascarosides with (ω–1)-linked side chains ranging from C4 to C11 (1, n = 1–8). In addition, some species-specific derivatives could be identified, including hydroxyacyl ascarosides17 and the corresponding 4-epimeric caenorhabdoside cae-7OH-ΔC9 (2),19 as well as indole ascarosides dominated by IC-asc-C5 (3)23 from C. nigoni or the fatty acid ascarosides dominated by asc-C4-cyC11 (4) from C. remanei.24
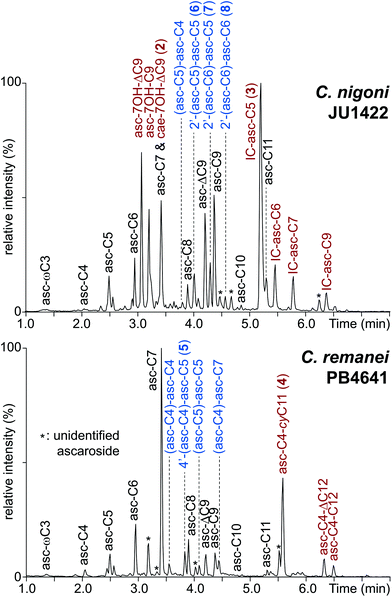 |
| Fig. 2 Ascaroside profiling in C. nigoni and C. remanei by MS/MS precursor ion scanning for m/z 73.0 [C3H5O2] reveals common ascarosides (black) along with species-specific components (red) including homo- and heterodimers (blue). | |
A yet uncharacterized class of putative ascarosides was observed in small quantities in both C. nigoni and C. remanei (Fig. 2) as well as other Caenorhabditis species. Their universal molecular formula C(20+n)H(34+2n)O11 (n = 0–10) was determined by high resolution mass spectrometry (HR-MS), which suggested a homologous series of ascaroside dimers (Table S1†). Systematic analysis of the ESI-(–)-HR-MS/MS spectra (Fig. 3) revealed neutral loss of an ascarylose unit [M − C6H13O4] to form ion I along with a monomeric ascaroside building block II from cleavage of the ester linkage, thus, facilitating the differentiation of isomeric homo and hetero-dimers. ESI-(+)-HR-MS/MS results in an oxonium ion III corresponding to the esterified ascaroside building block, as well as the loss of the terminal aglycone to yield fragment ion IV composed of both ascarylose units connected by their aglycone linker, which ultimately facilitates the assignment of both monomeric building blocks along with their order of attachment.
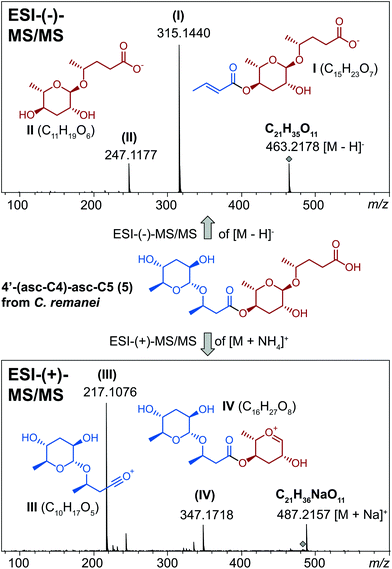 |
| Fig. 3 ESI-MS/MS analysis facilitates structure assignment of dimeric ascarosides as shown for 4′-(asc-C4)-asc-C5 (5) from C. remanei (monomeric units in red and blue). | |
Comparative profiling of fourteen Caenorhabditis species demonstrated a high degree of species-specificity. Eight out of fourteen species were found to be devoid of dimeric ascarosides, which are abundant in members of the Elegans group but could not be detected in the model organism C. elegans (Fig. S1†). Monomeric building blocks and their order of assembly were identified by HPLC-ESI-(+)-MS/MS (Fig. S2†), which resulted in the characterization of 32 dimeric ascarosides (Table S1†). The dominating ascaroside dimers were identified as 4′-(asc-C4)-asc-C5 (5) in C. remanei, 2′-(asc-C6)-asc-C5 (7) in C. nigoni, (asc-C4)-asc-C4 in C. brenneri, (asc-C5)-asc-C5 in C. tropicalis, (asc-C6)-asc-C6 in C. sinica, and (asc-C7)-asc-C9 in C. briggsae, (Fig. S3†). Considering that the monomeric building blocks with sidechains ranging from C4 to C11 are conserved in Caenorhabditis spp.17 this specific-specificity demonstrates that the biogenesis of ascaroside dimers is tightly controlled. Dimeric ascarosides carrying unsaturated side chains (such as (asc-ΔC9)-asc-C7) were also detected (Table S1†). Furthermore, traces of three ascaroside trimers in C. nigoni were identified as ((asc-C6)-asc-C6)-asc-Cx (x = 4, 5, and 6) (Fig. S4, Table S4†).
Structure assignments were confirmed by isolation of representative components (Fig. 4) from crude Caenorhabditis exometabolome extracts by solid phase extraction (SPE) and semi-preparative HPLC on RP-C18. Fractionation of 1.5 L of the C. remanei liquid culture supernatant afforded approximately 130 μg of the 4-linked 4′-(asc-C4)-asc-C5 (5) (Fig. S5†). Inspection of high resolution dqf-COSY spectra indicated that the characteristic 4-linkage of 5 (that could not be derived from the MS/MS data) could already be deduced in the partially purified metabolome mixture obtained after the 1st SPE fractionation step (Fig. S6†), which was utilized for the structure assignment of additional ascaroside dimers. Combined SPE and HPLC fractionation of 1.6 L of the C. nigoni liquid culture supernatant gave mixtures enriched in 2-linked 2′-(asc-C5)-asc-C5 (6), 2′-(asc-C6)-asc-C5 (7), and 2′-(asc-C6)-asc-C6 (8) (Fig. S7†) that were identified using a combination of ESI-(+)-HR-MS/MS (Fig. S8†) and dqf-COSY techniques (Fig. S9, Table S2†), demonstrating that their species-specific biosynthesis in the Caenorhabditis spp. includes the formation of homo- and heterodimeric 2- and 4-isomers (Fig. 4).
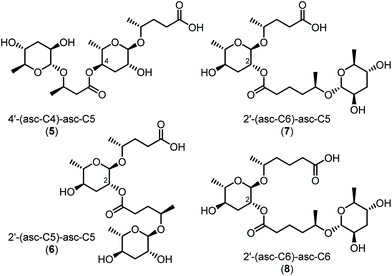 |
| Fig. 4 Ascaroside dimers isolated from Caenorhabditis remanei PB4641 (5) and Caenorhabditis nigoni JU1422 (6–8). | |
Total synthesis of ascaroside dimers
In order to unambiguously establish their structure assignments and to obtain pure materials for the characterization of biological activities, 2- and 4-isomeric pairs of homodimeric 2′/4′-(asc-C6)-asc-C6 and heterodimeric 2′/4′-(asc-C6)-asc-C5 were synthesized as shown in Fig. 5. Glycosylation of homologous (R)-2-hydroxyalkenes with 2,4-di-O-benzoyl-ascarylose (9, prepared in 8 steps from commercially available L-(+)-rhamnose)25,26 was accomplished using the trichloroacetimidate route27 to afford the (ω–1)-linked terminal alkenyl ascarosides (10a/b). After alkaline hydrolysis, cross metathesis with benzyl acrylate catalysed by Grubbs 2nd generation catalyst28 furnished the α,β-unsaturated benzyl esters 11a/b that served as monomeric building blocks for the synthesis of ascaroside dimers. Furthermore, one part of the hexenoate homolog 11b was derivatized with tert-butyldimethylsilyl chloride and O-debenzylated by palladium catalysed hydrogenation to yield the second monomeric building block 12. Coupling of the homologous ascaroside benzyl esters (11a or 11b) with the 2,4-di-O-TBS protected ascaroside (12) by Steglich esterification29 afforded mixtures of the trimeric 2,4-diester and both dimeric monoesters. Using an optimized 2
:
1 ratio of the monomeric building blocks 11 and 12 afforded predominantly the 2- and 4-isomeric dimers that were separated by column chromatography on silica gel. Next, palladium catalysed hydrogenation furnished the free acids that were finally deprotected using hydrogen fluoride in pyridine to give the 2-isomeric (7 and 8) and 4-isomeric (13a/b) ascaroside dimers that were isolated by RP-C18-SPE. Comparative NMR and HPLC-MS analysis confirmed that synthetic 2′-(asc-C6)-asc-C5 (7) and 2′-(asc-C6)-asc-C6 (8) are identical to the natural products isolated from C. nigoni (Fig. S10 and S11†).
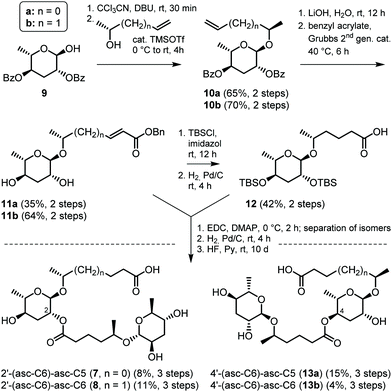 |
| Fig. 5 Total synthesis of homo- and hetero-dimeric ascarosides from C. nigoni. | |
Functional characterization of ascaroside dimers
Potential behavioural activities were characterized using a holding assay that quantifies nematode retention in ascaroside conditioned scoring regions in comparison to solvent control (Fig. 6). Females of C. remanei and C. nigoni did not respond to any of the dimeric ascarosides tested. C. remanei males were retained by 100 fmol of the conspecific 4′-(asc-C4)-asc-C5 (5), but not by the hetero-specific 2′-(asc-C6)-asc-C5 (7) and 2′-(asc-C6)-asc-C6 (8). In contrast C. nigoni males were retained by 100 fmol of the conspecific 2′-(asc-C6)-asc-C5 (7) and 2′-(asc-C6)-asc-C6 (8) but not by any of the hetero-specific 4-linked components such as 4′-(asc-C4)-asc-C5 (5), 4′-(asc-C6)-asc-C5 (13a), and 4′-(asc-C6)-asc-C6 (13b), demonstrating that males of both species are exclusively retained by their own conspecific ascaroside dimers.
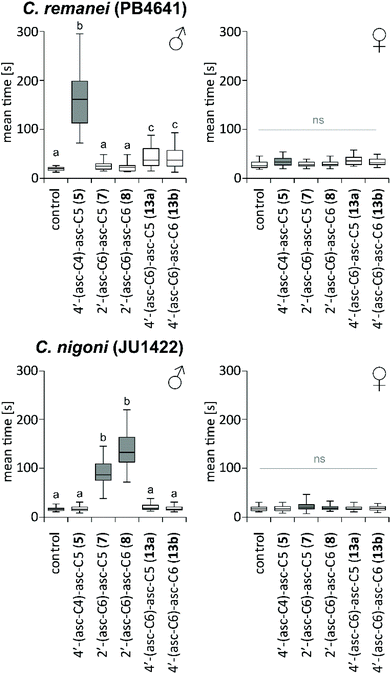 |
| Fig. 6 Species-specific retention of C. remanei and C. nigoni in response to 100 fmol of the ascaroside dimers shows male-specific retention to conspecific components (different letters indicate statistically significant differences between groups, P < 0.01, ANOVA with Dunett's post hoc test, n = 20, values represent means ± 1 SD). | |
Conclusions
In conclusion, combination of HR-MS/MS and NMR techniques along with total synthesis facilitated the identification of 2- and 4-isomeric homologous series of ascaroside dimers in Caenorhabditis spp. The homodimeric 4′-(asc-C7)-asc-C7 (SMID: dasc#1)30 has previously been shown to act as a modulator of mouth form dimorphism in the androdioecious nematode Pristionchus pacificus, diplogastridae, which also produces a variety of 2-linked ascaroside dimers that carry an additional 4-linked ureido-isobutyrate moiety.31,32 Among the gonochoristic C. remanei and C. nigoni the males are exclusively retained by ecologically significant amounts of their conspecific ascaroside dimers, demonstrating that dimerization represents an effective mechanism to generate species-specific signalling molecules. Considering just the most prevalent monomeric ascaroside building blocks with (ω–1)-linked acyl aglycones ranging from 4 to 11 carbons, their dimerization via the 2- or 4-position results in 128 theoretically possible structures of which 27 were now characterized in Caenorhabditis species using HR-MS/MS techniques. Incorporation of unsaturated aglycones as well as the assembly of ascaroside trimers further expands the structural diversity of these components. Remarkably, dimers carrying even numbered aglycones were particularly prevalent, although the corresponding monomeric building blocks such as asc-C4 (1, n = 1) and asc-C6 (1, n = 3) are rare and cannot be produced by canonical β-oxidation of the predominating homologous series of odd numbered ascarosides. Along with the hydroxylation of the ascaroside aglycones, epimerization of the ascarylose unit, and attachment of additional building blocks from primary metabolic pathways, the dimerization of monomeric units represents a highly effective mechanism to generate species-specific ascarosides, which form a complex chemical language in nematode communication.
Experimental
Organisms
Wild-type Caenorhabditis isolates of C. afra JU1286 (Ghana), C. brenneri PB2801 (Costa Rica), C. briggsae AF16 (India), C. doughertyi JU1771 (India), C. elegans N2 (UK), C. japonica DF5081 (Japan), C. monodelphis JU1667 (Germany), C. nigoni JU1422 (India), C. portoensis EG4788 (Portugal), C. remanei PB4641 (United States), C. sinica JU727 (China), C. tropicalis JU1373 (La Réunion), C. virilis JU1968 (France), and C. wallacei JU1904 (Indonesia), as well as Escherichia coli OP50 (uracil auxotroph) were obtained from the Caenorhabditis elegans Genetics Center (CGC).
Preparation of exometabolome extracts
Fourteen wild-type Caenorhabditis species were cultivated at 23 °C on Nematode Growth Medium (NGM) agar33 seeded with E. coli OP50. Mixed stage nematodes from five 10 cm plates were collected in M9 phosphate buffer33 to serve as inoculums for liquid cultures grown in 100 ml S-medium33 at 23 °C and 150 rpm. Concentrated E. coli OP50 bacteria pellet (from an overnight culture in lysogeny broth (LB) medium at 37 °C and 170 rpm and collected by centrifugation at 5000g for 10 min) was provided as food from day 1 to day 7, after which the cultures were starved for 7 days. After 14 days, nematodes were separated by centrifugation (5 min at 5000g). The filtered supernatant representing the exometabolome was frozen at −80 °C, lyophilized, and extracted with 3 × 100 ml methanol for 12 h each. The combined extract was filtered, concentrated to dryness at 40 °C under reduced pressure, reconstituted in 1 ml methanol, and aliquots were analysed by HPLC-HR-MS/MS in positive and negative mode, as well as HPLC-ESI-(−)-MS/MS precursor ion scanning for m/z 73.0 [C3H5O2].
Liquid chromatography-electrospray ionization-high resolution-tandem mass spectrometry (HPLC-ESI-HR-MS/MS)
HPLC-ESI-HRMS analysis of crude nematode exometabolome extracts and exometabolome fractions was performed using a Dionex UltiMate 3000 HPLC instrument coupled to a Bruker Maxis ultrahigh resolution (UHR) qTOF mass spectrometer equipped with an electrospray ionization (ESI) unit operated in positive or negative mode. Chromatographic separations were achieved using an Agilent ZORBAX Eclipse XDB-C18 column (250 × 3 mm, 5 μm particle diameter) with a flow rate of 400 μl min−1 and gradient elution starting at 3% acetonitrile in 0.5% aqueous acetic acid (v/v) for 5 minutes followed by a linear increase to 100% acetonitrile with 0.5% acetic acid (v/v) within 35 minutes. Data were analysed with the Compass DataAnalysis 4.3 software (Bruker).
Liquid chromatography electrospray ionization precursor ion scanning (HPLC-ESI-MS/MS)
MS/MS precursor ion scanning for m/z 73.0 [C3H5O2] was performed using an Agilent 1260 HPLC instrument (Agilent Technologies) coupled to an API5000 Triple Quadrupole mass spectrometer (AB Sciex, Darmstadt) equipped with an electrospray ionization (ESI) unit operated in negative mode. A collision energy of −34 was applied. Chromatographic separations were achieved using an Agilent ZORBAX Eclipse XDB-C18 column (50 × 4.6 mm, 1.8 μm particle diameter) (Agilent Technologies) with a flow rate of 1.1 ml min−1 and gradient elution starting at 5% acetonitrile in 0.05% aqueous formic acid (v/v) followed by a linear in-crease to 95% acetonitrile with 0.05% formic acid (v/v) within 10 minutes. Data were analysed with the Analyst 1.6 software (AB Sciex).
NMR spectroscopy
NMR spectra were recorded in CD3OD at 400 MHz for 1H and 100 MHz for 13C using a Bruker Avance III HD 400 instrument equipped with a 5 mm BBFO probe (MPICE), Bruker Avance II 400 instrument equipped with a 5 mm BBFO probe (NPAC, UniNE), or at 700 MHz for 1H and 175 MHz for 13C using a Bruker Avance III HD 700 instrument equipped with a 1.7 mm TCI microcryoprobe (MPICE). Residual solvent signals with 1H at 3.31 ppm and 13C at 49.05 ppm were used as internal standard. Two-dimensional homonuclear double quantum filtered (dqf)-COSY spectra were recorded using phase cycling for coherence selection. For the isolated compounds a total of 32 scans were acquired using a time domain of 8k in F2 (acquisition time of 1.2 s) and 512 increments in F1. Spectra were zero-filled prior to Fourier transformation, phased, and baseline corrected using the Topspin 3.2 (Bruker) and MNova 9.0 (Mestrelab Research) software.
Enrichment of dimeric ascarosides from Caenorhabditis exometabolome extracts
Dimeric ascarosides were enriched from exometabolome extracts obtained from 1.6 L of a Caenorhabditis nigoni JU1422 liquid culture supernatant17 and 1.5 L of a Caenorhabditis remanei PB4641 liquid culture supernatant24 obtained as previously described. The filtered supernatant was frozen at −80 °C, lyophilized, and the residue extracted with 3 × 100 ml methanol for 12 h each. The filtered extracts were concentrated to dryness under reduced pressure and the resulting exometabolome extract were adsorbed onto 2 g of Celite and fractionated by solid phase extraction (SPE) on 5 g reverse phase C18 cartridges (Chromabond, Macherey-Nagel) using a stepwise gradient of methanol in water as eluent (0 to 100% in 10% steps, v/v) to afford 10 fractions (20 ml each). Fractions were concentrated to dryness under reduced pressure and analyzed by HPLC-ESI-HR-MS/MS. Fraction eluting with 40–70% methanol that were found to be rich in dimeric ascarosides were either fractionated again by solid phase extraction (SPE) on 1 g reverse phase C18-endcapped cartridges (Chromabond, Macherey-Nagel) using a stepwise gradient of methanol in water as eluent (0 to 100% in 10% steps, v/v) to afford 10 fractions (5 ml each) and/or fractions containing the target components according to HPLC-ESI-(−)-HR-MS were subsequently submitted to semi-preparative HPLC using an Agilent HP-1100 HPLC instrument equipped with a Grom-Sil 120 ODS-4 HE column (250 × 8 mm, 5 μm) coupled to a Gilson 206 Abimed fraction collector. A flow rate of 2 ml min−1 with gradient elution was used starting at 3% acetonitrile in 0.5% aqueous acetic acid (v/v) for 3 minutes, followed by a linear increase to 100% acetonitrile with 0.5% acetic acid (v/v) within 30 minutes. Aliquots of 10 μl were analysed by HPLC-ESI-(−)-HR-MS as described before. Fractions containing the target compounds were concentrated to dryness under reduced pressure, dried in high vacuum, and the residues were dissolved in 550 μl CD3OD and analysed by one- and two-dimensional NMR spectroscopy.
(4R)-4-[[3,6-Dideoxy-4-O-[(3R)-3-[(3,6-dideoxy-α-L-arabino-hexopyranosyl)oxy]-1-oxo-butyl]-α-L-arabino-hexopyranosyl]oxy]-pentanoic acid (4′-(asc-C4)-asc-C5, 5) isolated from the C. remanei PB4641 exometabolome (approximately 130 μg); 1H NMR (700 MHz, CD3OD) δ 1.15 (3H, d, J = 6.1 Hz), 1.16 (3H, d, J = 6.1 Hz), 1.21 (3H, d, J = 6.2 Hz), 1.22 (3H, d, J = 6.2 Hz), 1.72 (ddd, J = 13.0 Hz, J = 11.7 Hz, J = 3.7 Hz), 1.83 (2H, m), 1.92 (1H, dt, J = 13.3 Hz, J = 3.9 Hz), 1.92 (1H, ddd, J = 13.2 Hz, J = 11.4 Hz, J = 3.8 Hz), 2.07 (1H, dt, J = 12.6 Hz, J = 3.8 Hz), 2.22 (1H, ddd, J = 14.9 Hz, J = 9.8 Hz, J = 6.1 Hz), 2.35 (1H, ddd, J = 15.1 Hz, J = 10.0 Hz, J = 6.4 Hz), 2.50 (1H, dd, J = 15.1 Hz, J = 5.4 Hz), 2.56 (1H, dd, J = 15.0 Hz, J = 7.5 Hz), 3.51 (1H, ddd, J = 11.6 Hz, J = 9.5 Hz, J = 4.8 Hz), 3.62 (1H, dq, J = 9.6 Hz, J = 6.2 Hz), 3.70 (1H, s.br), 3.72 (1H, s.br), 3.82 (1H, m), 3.90 (1H, dq, J = 9.6 Hz, J = 6.1 Hz), 4.23 (1H, m), 4.67 (1H, s), 4.69 (1H, s), 4.87 (1H, ddd, J = 11.3 Hz, J = 9.6 Hz, J = 6.1 Hz); 13C NMR (175 MHz, CD3OD) δ 18.0, 18.0, 18.7, 18.7, 32.7, 35.0, 35.2, 35.6, 43.1, 67.8, 67.9, 69.0, 69.2, 69.3, 71.0, 71.4, 72.2, 96.8, 97.1, 171.8, 182.1 (Table S2†); ESI-(−)-HR-MS: obs. m/z 463.2176 [M − H]−, calc. m/z 463.2185 for C21H35O11 (Table S1†).
(4R)-4-[[3,6-Dideoxy-2-O-[(4R)-4-[(3,6-dideoxy-α-L-arabino-hexopyranosyl)oxy]-1-oxo-pentyl]-α-L-arabino-hexopyranosyl]oxy]-pentanoic acid (2′-(asc-C5)-asc-C5, 6) enriched from the C. nigoni JU1422 exometabolome (approximately 50 μg) along with dominating asc-C8; 1H NMR (400 MHz, CD3OD) δ 1.14 (3H, d, J = 6.1 Hz), 1.14 (3H, d, 6.3 Hz), 1.22 (3H, d, J = 6.2 Hz), 1.23 (3H, d, J = 6.2 Hz), 1.77 (1H, m), 1.80 (4H, m), 1.87 (1H, ddd, J = 13.2 Hz, J = 11.4 Hz, J = 3.2 Hz), 1.96 (1H, m), 2.01 (1H, dt, J = 13.1 Hz, J = 4.1 Hz), 2.32 (2H, dt, J = 5.0 Hz, J = 7.2 Hz), 2.39 (2H, m), 3.41 (ddd, J = 11.4 Hz, J = 9.6 Hz, J = 4.6 Hz), 3.52 (1H, m), 3.62 (1H, m), 3.68 (1H, dq, J = 9.7 Hz, J = 6.3 Hz), 3.72 (1H, s.br), 3.80 (1H, m), 3.83 (1H, m), 4.65 (1H, s), 4.71 (1H, s), 4.79 (1H, s.br) (Table S2†); ESI-(−)-HR-MS: obs. m/z 477.2348 [M − H]−, calc. m/z 477.2341 for C22H37O11 (Table S1†).
(4R)-4-[[3,6-Dideoxy-2-O-[(5R)-5-[(3,6-dideoxy-α-L-arabino-hexopyranosyl)oxy]-1-oxo-hexyl]-α-L-arabino-hexopyranosyl]oxy]-pentanoic acid (2′-(asc-C6)-asc-C5, 7) enriched from the C. nigoni JU1422 exometabolome (approximately 100 μg) along with dominating asc-ΔC9; 1H NMR (400 MHz, CD3OD) δ 1.14 (3H, d, J = 6.2 Hz), 1.14 (3H, d, J = 6.3 Hz), 1.22 (3H, d, J = 6.2 Hz), 1.22 (3H, d, J = 6.2 Hz), 1.56 (2H, m), 1.70 (1H, m), 1.77 (1H, ddd, J = 13.3 Hz, J = 11.4 Hz, J = 3.0 Hz), 1.80 (3H, m), 1.90 (1H, ddd, J = 13.3 Hz, J = 11.2 Hz, J = 3.4 Hz), 1.96 (1H, dt, J = 13.1 Hz, J = 4.1 Hz), 2.01 (1H, dt, J = 13.2 Hz, J = 3.9 Hz), 2.32 (2H, m), 2.39 (2H, dt, J = 3.1 Hz, J = 7.4 Hz), 3.40 (ddd, J = 11.5 Hz, J = 9.7 Hz, J = 4.9 Hz), 3.52 (1H, ddd, J = 11.3 Hz, J = 9.6 Hz, J = 4.7 Hz), 3.62 (1H, dq, J = 9.5 Hz, J = 6.2 Hz), 3.71 (1H, dq, J = 9.7 Hz, J = 6.3 Hz), 3.73 (1H, s.br), 3.81 (1H, m), 3.83 (1H, m), 4.65 (1H, s), 4.71 (1H, s), 4.79 (1H, s.br) (Table S2†); ESI-(−)-HR-MS: obs. m/z 491.2495 [M − H]−, calc. m/z 491.2498 for C23H39O11 (Table S1†).
(5R)-5-[[3,6-Dideoxy-2-O-[(5R)-5-[(3,6-dideoxy-α-L-arabino-hexopyranosyl)oxy]-1-oxo-hexyl]-α-L-arabino-hexopyranosyl]oxy]-hexanoic acid (2′-(asc-C6)-asc-C6, 8) isolated from the C. nigoni JU1422 exometabolome (approximately 100 μg) along with minor amounts of asc-C9; 1H NMR (400 MHz, CD3OD) δ 1.14 (3H, d, J = 6.3 Hz), 1.14 (3H, d, J = 6.3 Hz), 1.22 (3H, d, J = 6.2 Hz), 1.22 (3H, d, J = 6.2 Hz), 1.57 (4H, m), 1.65 (1H, m), 1.69 (1H, m), 1.76 (1H, m), 1.77 (1H, ddd, J = 13.0 Hz, J = 11.5 Hz, J = 3.1 Hz), 1.79 (1H, m), 1.89 (1H, ddd, J = 13.4 Hz, J = 11.5 Hz, J = 3.3 Hz), 1.95 (1H, dt, J = 13.2 Hz, J = 3.9 Hz), 2.00 (1H, dt, J = 13.3 Hz, J = 3.9 Hz), 2.32 (2H, t, J = 7.2 Hz), 2.39 (2H, dt, J = 3.0 Hz, J = 7.5 Hz), 3.40 (ddd, J = 11.5 Hz, J = 9.7 Hz, J = 4.9 Hz), 3.51 (1H, ddd, J = 11.5 Hz, J = 9.7 Hz, J = 4.9 Hz), 3.62 (1H, dq, J = 9.7 Hz, J = 6.3 Hz), 3.70 (1H, dq, J = 9.7 Hz, J = 6.3 Hz), 3.72 (1H, s.br), 3.79 (1H, m), 3.80 (1H, m), 4.65 (1H, s), 4.71 (1H, s), 4.78 (1H, s.br) (Table S2†); ESI-(−)-HR-MS: obs. m/z 505.2664 [M − H]−, calc. m/z 505.2654 for C24H41O11 (Table S1†).
Holding assay to evaluate nematode behavioural response
Nematode preference for environments conditioned with 100 fmol of ascaroside dimers was measured using a modified holding assay. On a 6 cm Petri dish filled with 6 ml peptone-free nematode growth medium (NGM) agar, circular scoring regions of 9 mm diameter were marked. Next, 1 μl of 10% aqueous methanol (v/v, as solvent control) or 100 nM solutions of ascaroside dimers such as natural 4′-(asc-C4)-asc-C5 (5) isolated from C. remanei PB4641, synthetic 2′-(asc-C6)-asc-C5 (7) and 2′-(asc-C6)-asc-C6 (8) identical to the natural products isolated from C. nigoni JU1422, or their synthetic 4-isomers 4′-(asc-C6)-asc-C5 (13a) and 4′-(asc-C6)-asc-C6 (13b) in 10% aqueous methanol (v/v) were placed in the centre of the scoring areas onto the agar and left to dry for 5 minutes. Young adult nematodes from non-starved and non-crowded 6 cm NGM agar plates seeded with E. coli OP50 were sorted by sex and transferred to peptone-free unseeded NGM agar plates for approximately 30 min before being used for the assay to minimize the amount of concomitant bacteria. Individual worms (up to 3) were placed into the centre of the conditioned scoring region and the time required for the nematodes to leave the scoring region was recorded. Nematodes were defined to have left the scoring area when no part of the nematode was still within the circular boundary. A total number of 20 worms per condition were analysed and experiments were repeated on two separate days with comparable results. A one-way ANOVA with Dunett's post-test was performed using the SPSS statistics software version 26 (IBM) to evaluate the effect of ascaroside dimers versus solvent control on mean times nematode spent in scoring regions.
(3R)-3-[(2,4-Di-O-benzoyL-3,6-dideoxy-α-L-arabino-hexopyranosyl)oxy]-1-butene (10a).
A solution of 2,4-di-O-benzoyL-3,6-dideoxy-α-L-arabino-hexopyranose (9, 395 mg, 1.11 mmol) (synthesized in 6 steps from L-rhamnose25,26) in dry dichloromethane (15 ml) was treated with trichloroacetonitrile (222 μL, 2.22 mmol) and 1,8-diazabicyclo[5.4.0]undec-7-ene (DBU, 16 μL 0.11 mmol). After stirring for 30 min, the solution was concentrated under reduced pressure and the residue was quickly chromatographed over a short column of silica gel using 10% (v/v) ethyl acetate in hexane as eluent to afford 2,4-di-O-benzoyl-3,6-dideoxy-α-L-arabino-hexopyranosyl-1-(2,2,2-trichloroethanimidate) (462 mg, 0.92 mmol, 83% yield) as a colourless oil that was immediately used for the next step.
A solution of 2,4-di-O-benzoyl-3,6-dideoxy-α-L-arabino-hexopyranosyl-1-(2,2,2-trichloroethanimidate) (462 mg, 0.92 mmol) and (R)-(−)-3-butene-2-ol (104 mg, 1.44 mmol) in dry dichloromethane (15 mL) at 0 °C was treated with catalytic amounts of trimethylsilyl triflate (5 μL). After stirring at 0 °C for 1 h and another 3 h at room temperature the mixture was concentrated under reduced pressure and fractionated by column chromatography on silica gel using 2.5% (v/v) ethyl acetate in toluene as eluent to afford (3R)-3-[(2,4-di-O-benzoyl-3,6-dideoxy-α-L-arabino-hexopyranosyl)oxy]-1-butene (10a, 297 mg, 0.72 mmol, 78% yield) as a colourless oil. 1H NMR (400 MHz, CD3OD) δ 1.26 (3H, d, J = 6.2 Hz), 1.31 (3H, d, J = 6.4 Hz), 2.23 (1H, m), 2.42 (1H, td, J = 13.5 Hz, J = 3.8 Hz), 4.13 (1H, dq, J = 9.7 Hz, J = 6.1 Hz), 4.31 (1H, m), 4.98 (1H, s), 5.14 (1H, d, J = 10.3 Hz), 5.19 (2H, m), 5.28 (1H, d, J = 17.2 Hz), 5.96 (1H, ddd, J = 6.3 Hz, J = 10.6 Hz, J = 17.1 Hz), 7.47 (4H, m), 7.59 (2H, m), 8.04 (2H, d, J = 7.2 Hz), 8.11 (2H, d, J = 7.1 Hz); 13C {1H} NMR (100 MHz, CD3OD) δ 17.7, 19.9, 29.7, 66.9, 70.6, 70.9, 74.3, 94.6, 114.8, 128.4 (4C), 129.6 (2C), 129.9 (2C), 130.0, 133.15, 133.24, 140.1, 165.7, 165.8; ESI-(+)-HR-MS: obs. m/z 433.1631 [M + Na]+, calc. m/z 433.1622 for C24H26NaO6.
(4R)-4-[(2,4-Di-O-benzoyl-3,6-dideoxy-α-L-arabino-hexopyranosyl)oxy]-1-pentene (10b).
A solution of 2,4-di-O-benzoyl-3,6-dideoxy-α-L-arabino-hexopyranosyl-1-(2,2,2-trichloroethanimidate) (462 mg, 0.92 mmol; see 10a for its preparation) and (R)-(−)-4-pentene-2-ol (126 mg, 1.46 mmol) in dry dichloromethane (15 mL) at 0 °C was treated with catalytic amounts of trimethylsilyl triflate (5 μL). After stirring at 0 °C for 1 h and another 3 h at room temperature the mixture was concentrated under reduced pressure. The residue was fractionated by column chromatography on silica gel using 2.5% (v/v) ethyl acetate in toluene as eluent to afford (4R)-4-[(2,4-di-O-benzoyl-3,6-dideoxy-α-L-arabino-hexopyranosyl)oxy]-1-pentene (10b, 332 mg, 0.78 mmol, 85% yield) as a colourless oil. 1H NMR (400 MHz, CD3OD) δ 1.20 (3H, d, J = 6.1 Hz), 1.24 (3H, d, J = 6.2 Hz), 2.19 (1H, ddd, J = 13.5 Hz, J = 11.3 Hz, J = 2.8 Hz), 2.31 (1H, m), 2.40 (2H, m), 3.92 (1H, tq, J = 9.2 Hz, J = 6.1 Hz), 4.20 (1H, dq, J = 9.7 Hz, J = 6.2 Hz), 4.96 (1H, s), 5.12 (4H, m), 5.92 (1H, ddt, J = 17.2 Hz, J = 9.9 Hz, J = 7.2 Hz), 7.50 (4H, m), 7.62 (2H, m), 8.01 (2H, d, J = 7.2 Hz), 8.09 (2H, d, J = 7.2 Hz); 13C {1H} NMR (100 MHz, CD3OD) δ 18.1, 19.3, 30.6, 42.7, 68.2, 72.0, 72.7, 73.8, 95.2, 117.6, 129.7 (4C), 130.5 (2C), 130.7 (2C), 131.2, 134.48, 134.54, 136.3, 167.0, 167.1; ESI-(+)-HR-MS: obs. m/z 447.1782 [M + Na]+, calc. m/z 447.1778 for C25H28NaO6.
Benzyl (2E,4R)-4-[(3,6-dideoxy-α-L-arabino-hexopyranosyl)oxy]-2-pentenoate (11a).
A solution of (3R)-3-[(2,4-di-O-benzoyl-3,6-dideoxy-α-L-arabino-hexopyranosyl)oxy]-1-butene (10a, 180 mg, 0.439 mmol) in methanol (1.8 ml) was treated with 820 μL of saturated aqueous lithium hydroxide (105 mg, 4.39 mmol) solution. The mixture was stirred for 12 h at room temperature, the pH neutralized with acetic acid, and the solution concentrated under reduced pressure. The product was purified by column chromatography on silica gel using 10% methanol (v/v) in dichloromethane as eluent to afford (3R)-3-[(3,6-dideoxy-α-L-arabino-hexopyranosyl)oxy]-1-butene (64.7 mg, 0.320 mmol, 73% yield) as a colourless oil. 1H NMR (400 MHz, CD3OD) δ 1.19 (3H, d, J = 6.2 Hz), 1.22 (3H, d, J = 6.4 Hz), 1.79 (1H, ddd, J = 13.2 Hz, J = 11.2 Hz, J = 3.1 Hz), 1.95 (1H, ddd, J = 13.1 Hz, J = 3.9 Hz, J = 3.9 Hz), 3.51 (1H, ddd, J = 11.1 Hz, J = 9.5 Hz, J = 4.6 Hz), 3.61 (1H, qd, J = 9.3 Hz, J = 6.0 Hz), 3.75 (1H, br s), 4.23 (1H, qd, J = 6.3 Hz, J = 6.4 Hz), 4.66 (1H, s), 5.06 (1H, ddd, J = 10.5 Hz, J = 1.6 Hz, J = 1.6 Hz), 5.22 (1H, ddd, J = 17.3 Hz, J = J = 1.5 Hz), 5.90 (1H, ddd, J = 17.2 Hz, J = 10.5 Hz, J = 6.1 Hz); ESI-(+)-HR-MS: obs. m/z 225.1103 [M + Na]+, calc. m/z 225.1097 for C10H18NaO4.
A solution of (3R)-3-[(3,6-dideoxy-α-L-arabino-hexopyranosyl)oxy]-1-butene (64.7 mg 0.320 mmol) in dry dichloromethane (6.5 ml) was treated with benzyl acrylate (240 μL, 259.6 mg, 1.6 mmol) and Grubbs 2nd generation catalyst (27.2 mg, 32.0 μmol, 10 mol%). After stirring at reflux for 6 h the solution was concentrated under reduced pressure and the residue fractionated on silica gel using 5% (v/v) methanol in dichloromethane as eluent to afford benzyl (2E,4R)-4-[(3,6-dideoxy-α-L-arabino-hexopyranosyl)oxy]-2-pentenoate (11a, 51.2 mg, 0.152 mmol, 48% yield) as a colourless oil. 1H NMR (400 MHz, CD3OD) δ 1.15 (3H, d, J = 5.80 Hz), 1.28 (3H, d, J = 6.6 Hz), 1.79 (1H, ddd, J = 13.3 Hz, J = 10.9 Hz, J = 3.1 Hz), 1.96 (1H, m), 3.52 (2H, m), 3.78 (1H, br.s), 4.44 (1H, m), 4.68 (1H, s), 5.18 (2H, s), 6.07 (1H, dd, J = 15.8 Hz, J = 1.7 Hz), 6.99 (1H, dd, J = 15.7 Hz, J = 5.4 Hz), 7.34 (5H, m); 13C {1H} NMR (100 MHz, CD3OD) δ 17.9, 19.6, 36.0, 67.3, 68.2, 69.6, 71.4, 72.4, 98.6, 120.6, 129.26, 129.31 (2C), 129.6 (2C), 151.6, 167.8; ESI-(+)-HR-MS: obs. m/z 359.1474 [M + Na]+, calc. m/z 359.1465 for C18H24NaO6.
Benzyl (2E,5R)-5-[(3,6-dideoxy-α-L-arabino-hexopyranosyl)oxy]-2-hexenoate (11b).
A solution of (4R)-4-[(2,4-di-O-benzoyl-3,6-dideoxy-α-L-arabino-hexopyranosyl)oxy]-1-pentene (10b, 200 mg, 0.471 mmol) in methanol (2 mL) was treated with 881 μL of saturated aqueous lithium hydroxide (112 mg, 4.71 mmol) solution. The mixture was stirred for 12 h at room temperature, the pH neutralized with acetic acid, and the solution concentrated under reduced pressure. The product was purified by column chromatography on silica gel using 10% (v/v) methanol in dichloromethane as eluent to afford (4R)-4-[(3,6-dideoxy-α-L-arabino-hexopyranosyl)oxy]-1-pentene (88.8 mg, 0.411 mmol, 87% yield) as a colourless oil. 1H NMR (400 MHz, CD3OD) δ 1.13 (3H, d, J = 6.1 Hz), 1.20 (3H, d, J = 6.2 Hz), 1.76 (1H, ddd, J = 13.1 Hz, J = 11.3 Hz, J = 3.1 Hz), 1.95 (1H, ddd, J = 12.8 Hz, J = 3.3 Hz, J = 0.9 Hz), 2.28 (2H, m), 3.51 (1H, ddd, J = 11.3 Hz, J = 9.5 Hz, J = 4.6 Hz), 3.65 (1H, dq, J = 9.5 Hz, J = 6.4 Hz), 3.72 (1H, br s), 3.82 (1H, m), 4.64 (1H, s), 5.06 (2H, m), 5.88 (1H, ddt, J = 17.2 Hz, J = 10.3 Hz, J = 7.2 Hz); ESI-(+)-HR-MS: obs. m/z 239.1266 [M + Na]+, calc. m/z 239.1254 for C11H20NaO4.
A solution of (4R)-4-[(3,6-dideoxy-α-L-arabino-hexopyranosyl)oxy]-1-pentene (98.8 mg 0.457 mmol) in dry dichloromethane (10 mL) was treated with benzyl acrylate (343 μL, 370 mg, 2.28 mmol) and Grubbs 2nd generation catalyst (38.8 mg, 45.7 μmol, 10 mol%). After stirring at reflux for 6 h the solution was concentrated under reduced pressure and the residue fractionated on silica gel using 5% methanol in dichloromethane (v/v) as eluent to afford benzyl (2E,5R)-5-[(3,6-dideoxy-α-L-arabino-hexopyranosyl)oxy]-2-hexenoate (11b, 118.9 mg 0.339 mmol, 74% yield) as a colourless oil. 1H NMR (400 MHz, CD3OD) δ 1.16 (3H, d, J = 5.9 Hz), 1.17 (3H, d, J = 6.1 Hz), 1.74 (1H, ddd, J = 13.1 Hz, J = 11.0 Hz, J = 2.9 Hz), 1.94 (1H, dt, J = 12.9 Hz, J = 3.9 Hz), 2.45 (2H, m), 3.50 (1H, ddd, J = 11.1 Hz, J = 9.4 Hz, J = 4.5 Hz), 3.59 (1H, dq, J = 9.6 Hz, J = 6.0 Hz), 3.72 (1H, br.s), 3.94 (1H, m), 4.63 (1H, s), 5.17 (2H, s), 5.97 (1H, d, J = 15.7 Hz), 7.05 (1H, dt, J = 15.6 Hz, J = 7.3 Hz), 7.35 (5H, m); 13C {1H} NMR (100 MHz, CD3OD) δ 18.1, 19.5, 35.9, 40.8, 67.2, 68.3, 69.9, 71.4, 72.4, 98.2, 124.0, 129.18, 129.21 (2C), 129.5 (2C), 147.9, 167.7; ESI-(+)-HR-MS: obs. m/z 373.1629 [M + Na]+, calc. m/z 373.1622 for C19H26NaO6.
(5R)-5-[(2,4-Di-O-tert-butyldimethylsilyl-3,6-dideoxy-α-L-arabino-hexopyranosyl)oxy]-hexanoic acid (12).
A solution of benzyl (2E,5R)-5-[(3,6-dideoxy-α-L-arabino-hexopyranosyl)oxy]-2-hexenoate (11b, 55 mg, 157 μmol) in dry dichloromethane (5 mL) was treated with imidazole (53 mg, 785 μmol) and tert-butyldimethylsilyl chloride (118 mg, 785 μmol). After stirring overnight, the reaction was quenched with water (1 mL) and the organic phase dried over sodium sulphate and concentrated under reduced pressure. The residue was fractionated by column chromatography on silica gel using 15% (v/v) ethyl acetate in hexane as eluent to afford benzyl (2E,5R)-5-[(2,4-di-O-tert-butyldimethylsilyl-3,6-dideoxy-α-L-arabino-hexopyranosyl)oxy]-2-hexenoate (40 mg, 69.1 μmol, 44% yield) as a colourless oil. 1H NMR (400 MHz, CD3OD) δ 0.07 (12H, m), 0.89 (9H, s), 0.92 (9H, s), 1.12 (3H, d, J = 6.0 Hz), 1.14 (3H, d, J = 6.2 Hz), 1.74 (1H, ddd, J = 12.9 Hz, J = 10.2 Hz, J = 2.7 Hz), 1.82 (1H, ddd, J = 12.9 Hz, J = J = 3.7 Hz), 2.43 (2H, ddd, J = 7.3 H z, J = 6.2 Hz, J = 1.2 Hz), 3.59 (1H, dq, J = 8.9 Hz, J = 5.9 Hz), 3.65 (1H, ddd, J = 9.9 Hz, J = 9.2 Hz, J = 4.3 Hz), 3.80 (1H, br.s), 3.92 (1H, tq, J = 6.2 Hz, J = 6.1 Hz), 4.53 (1H, s), 5.13 (1H, d, J = 12.5 Hz), 5.17 (1H, d, J = 12.5 Hz), 5.96 (1H, dt, J = 15.7 Hz, J = 1.7 Hz), 7.03 (1H, dt, J = 15.5 Hz, J = 7.4 Hz), 7.32 (5H, m); 13C {1H} NMR (100 MHz, CD3OD) δ −4.71 (2C), −4.4, −3.8, 18.6, 18.8, 18.9, 19.6, 26.3, 26.4 (3C), 37.9, 40.8, 67.1, 70.1, 71.2, 71.6, 72.7, 98.3, 124.1, 129.14 (2C), 129.15, 129.5 (2C), 137.6, 147.9, 167.5; ESI-(+)-HR-MS: obs. m/z 601.3334 [M + Na]+, calc. m/z 601.3351 for C31H54NaO6Si2.
A solution of benzyl (2E,5R)-5-[(2,4-di-O-tert-butyldimethylsilyl-3,6-dideoxy-α-L-arabino-hexopyranosyl)oxy]-2-hexenoate (40 mg, 69.1 μmol) in MeOH (0.5 mL) was treated with 10 mol% palladium on carbon (20 mg) and hydrogenated under H2 atmosphere (1 atm). After stirring for 4 h the catalyst was removed by filtration over Celite and the product was obtained after concentration under reduced pressure to afford (5R)-5-[(2,4-di-O-tert-butyldimethylsilyl-3,6-dideoxy-α-L-arabino-hexopyranosyl)oxy]-hexanoic acid (12, 32.7 mg, 66.7 μmol, 96% yield) as a colourless oil. 1H NMR (400 MHz, CD3OD) δ 0.09 (12H, m), 0.91 (9H, s), 0.92 (9H, s), 1.13 (3H, d, J = 6.1 Hz), 1.17 (3H, d, J = 6.0 Hz), 1.45–1.78 (3H, m), 1.85 (1H, ddd, J = 12.7 Hz, J = J = 3.8 Hz), 2.31 (2H, td, J = 7.7 Hz, J = 2.4 Hz), 3.63 (1H, m), 3.67 (1H, m), 3.79 (1H, m), 3.80 (1H, s.br), 4.54 (1H, s); 13C {1H} NMR (100 MHz, CD3OD) δ −4.80, −4.78, −4.4, −3.9, 18.5, 18.8, 18.9, 19.2, 26.25 (3C), 26.30 (3C), 31.3, 33.4, 38.0, 70.2, 71.3, 71.6, 71.7, 97.8, 177.4; ESI-(+)-HR-MS: obs. m/z 513.3047 [M + Na]+, calc. m/z 513.3038 for C24H50NaO6Si2.
Benzyl (2E,4R)-4-[[3,6-dideoxy-2-O-[(5R)-5-[(2,4-di-O-tert-butyldimethylsilyl-3,6-dideoxy-α-L-arabino-hexopyranosyl)oxy]-1-oxohexyl]-α-L-arabino-hexopyranosyl]oxy]-2-pentenoate & benzyl (2E,4R)-4-[[3,6-dideoxy-4-O-[(5R)-5-[(2,4-di-O-tert-butyldi-methylsilyl-3,6-dideoxy-α-L-arabino-hexopyranosyl)oxy]-1-oxohexyl]-α-L-arabino-hexopyranosyl]oxy]-2-pentenoate.
A solution of (5R)-5-[(2,4-di-O-tert-butyldimethylsilyl-3,6-dideoxy-α-L-arabino-hexopyranosyl)oxy]-hexanoic acid (12, 5.0 mg, 10.2 μmol) in dry dichloromethane (0.5 mL) at 0 °C was treated with 4-dimethylaminopyridine (DMAP, 2.5 mg, 20.4 μmol) and 1-ethyl-3-(3-dimethylaminopropyl) carbodiimide hydrochloride (EDC HCl, 3.9 mg, 20.4 μmol). After stirring for 5 minutes a solution of benzyl (2E,4R)-4-[(3,6-dideoxy-α-L-arabino-hexopyranosyl)oxy]-2-pentenoate (11a, 6.9 mg, 20.4 μmol) in dry dichloromethane (0.5 mL) was added. After 2 h at 0 °C, the solution was concentrated under reduced pressure and the residue was fractionated by column chromatography on silica gel using a stepwise gradient from 10% to 30% (v/v) ethyl acetate in hexane as eluent to afford the 2-linked benzyl (2E,4R)-4-[[3,6-dideoxy-2-O-[(5R)-5-[(2,4-di-O-tert-butyldimethylsilyl-3,6-dideoxy-α-L-arabino-hexopyranosyl)oxy]-1-oxohexyl]-α-L-arabino-hexopyranosyl]oxy]-2-pentenoate (2.1 mg, 2.57 μmol, 25% yield) and the 4-linked benzyl (2E,4R)-4-[[3,6-dideoxy-4-O-[(5R)-5-[(2,4-di-O-tert-butyldimethylsilyl-3,6-dideoxy-α-L-arabino-hexopyranosyl)oxy]-1-oxohexyl]-α-L-arabino-hexopyranosyl]oxy]-2-pentenoate (2.6 mg, 3.18 μmol, 31% yield) as colourless oils.
Benzyl (2E,4R)-4-[[3,6-dideoxy-2-O-[(5R)-5-[(2,4-di-O-tert-butyldimethylsilyl-3,6-dideoxy-α-L-arabino-hexopyranosyl)oxy]-1-oxohexyl]-α-L-arabino-hexopyranosyl]oxy]-2-pentenoate.
1H NMR (400 MHz, CD3OD) δ 0.08 (12H, s), 0.90 (9H, s), 0.92 (9H, s), 1.12 (3H, d, J = 6.0 Hz), 1.16 (3H, d, J = 6.4 Hz), 1.17 (3H, d, J = 6.1 Hz), 1.29 (3H, d, J = 6.5 Hz), 1.55 (2H, m), 1.78 (5H, m), 2.02 (1H, m), 2.40 (2H, t, J = 7.0 Hz), 3.42 (1H, m), 3.63 (3H, m), 3.79 (2H, m), 4.44 (1H, m), 4.54 (1H, s), 4.76 (1H, s), 4.86 (1H, s.br), 5.19 (2H, s), 6.08 (1H, d, J = 15.3 Hz), 6.98 (1H, dd, J = 15.7 Hz, J = 5.3 Hz), 7.36 (5H, m); ESI-(+)-HR-MS: obs. m/z 831.4512 [M + Na]+, calc. m/z 831.4504 for C42H72ONaO11Si2.
Benzyl (2E,4R)-4-[[3,6-dideoxy-4-O-[(5R)-5-[(2,4-di-O-tert-butyldimethylsilyl-3,6-dideoxy-α-L-arabino-hexopyranosyl)oxy]-1-oxohexyl]-α-L-arabino-hexopyranosyl]oxy]-2-pentenoate.
1H NMR (400 MHz, CD3OD) δ 0.07 (12H, s), 0.90 (9H, s), 0.93 (9H, s), 1.07 (3H, d, J = 6.4 Hz), 1.11 (3H, d, J = 5.9 Hz), 1.17 (3H, d, J = 6.0 Hz), 1.29 (3H, d, J = 6.4 Hz), 1.53 (2H, m), 1.67 (1H, m), 1.80 (4H, m), 2.06 (1H, dt, J = 12.9 Hz, J = 3.3 Hz), 2.36 (2H, m), 3.64 (2H, m), 3.78 (4H, m), 4.44 (1H, m), 4.54 (1H, s), 4.72 (1H, s), 4.85 (1H, m), 5.19 (2H, s), 6.06 (2H, d, J = 15.2 Hz), 6.99 (1H, dd, J = 15.4 Hz, J = 5.7 Hz), 7.36 (5H, m); ESI-(+)-HR-MS: obs. m/z 831.4515 [M + Na]+, calc. m/z 831.4504 for C42H72ONaO11Si2.
Benzyl (2E,5R)-5-[[3,6-dideoxy-2-O-[(5R)-5-[(2,4-di-O-tert-butyldimethylsilyl-3,6-dideoxy-α-L-arabino-hexopyranosyl)oxy]-1-oxohexyl]-α-L-arabino-hexopyranosyl]oxy]-2-hexenoate & Benzyl (2E,5R)-5-[[3,6-dideoxy-4-O-[(5R)-5-[(2,4-di-O-tert-butyldi-methylsilyl-3,6-dideoxy-α-L-arabino-hexopyranosyl)oxy]-1-oxohexyl]-α-L-arabino-hexo-pyranosyl]oxy]-2-hexenoate.
A solution of (5R)-5-[(2,4-di-O-tert-butyldimethylsilyl-3,6-dideoxy-α-L-arabino-hexopyranosyl)oxy]-hexanoic acid (12, 4.5 mg, 9.2 μmol) in dry dichloromethane (0.5 mL) at 0 °C was treated with 4-dimethylaminopyridine (DMAP, 2.3 mg, 18.4 μmol) and 1-ethyl-3-(3-dimethylaminopropyl) carbodiimide hydrochloride (EDC HCl, 3.5 mg, 18.4 μmol). After stirring for 5 minutes a solution of benzyl (2E,5R)-5-[(3,6-dideoxy-α-L-arabino-hexopyranosyl)oxy]-2-hexenoate (11b, 6.5 mg, 18.4 μmol) in dry dichloromethane (0.5 mL) was added. After 2 h at 0 °C the solution was concentrated under reduced pressure and the residue fractionated by column chromatography on silica gel using a stepwise gradient from 10% to 30% (v/v) ethyl acetate in hexane as eluent to afford the 2-linked benzyl (2E,5R)-5-[[3,6-dideoxy-2-O-[(5R)-5-[(2,4-di-O-tert-butyldimethyl-silyl-3,6-dideoxy-α-L-arabino-hexopyranosyl)oxy]-1-oxohexyl]-α-L-arabino-hexopyranosyl]oxy]-2-hexenoate (2.4 mg, 2.89 μmol, 31% yield) and the 4-linked benzyl (2E,5R)-5-[[3,6-dideoxy-4-O-[(5R)-5-[(2,4-di-O-tert-butyldimethylsilyl-3,6-dideoxy-α-L-arabino-hexopyranosyl)oxy]-1-oxohexyl]-α-L-arabino-hexopyranosyl]oxy]-2-hexenoate (1.1 mg, 1.32 μmol, 14% yield) as colourless oils.
Benzyl (2E,5R)-5-[[3,6-dideoxy-2-O-[(5R)-5-[(2,4-di-O-tert-butyldimethylsilyl-3,6-dideoxy-α-L-arabino-hexopyranosyl)oxy]-1-oxohexyl]-α-L-arabino-hexopyranosyl]oxy]-2-hexenoate.
1H NMR (400 MHz, CD3OD) δ 0.08 (12H, s), 0.90 (9H, s), 0.92 (9H, s), 1.12 (3H, d, J = 6.0 Hz), 1.17 (6H, m), 1.46–1.89 (9H, m), 1.99 (1H, dt, J = 13.5 Hz, J = 3.5 Hz), 2.40 (1H, t, J = 6.6 Hz), 2.46 (1H, t, J = 6.6 Hz), 3.42 (1H, m), 3.65 (3H, m), 3.78 (2H, m), 3.80 (1H, s), 3.94 (1H, q, J = 6.1 Hz), 4.54 (1H, s), 4.71 (1H, s), 4.78 (1H, s.br), 5.17 (2H, s), 5.98 (1H, d, J = 15.7 Hz), 7.05 (1H, dt, J = 15.5 Hz, J = 7.8 Hz), 7.34 (5H, m); ESI-(+)-HR-MS: obs. m/z 845.4670 [M + Na]+, calc. m/z 845.4662 for C43H74ONaO11Si2.
Benzyl (2E,5R)-5-[[3,6-dideoxy-4-O-[(5R)-5-[(2,4-di-O-tert-butyldimethylsilyl-3,6-dideoxy-α-L-arabino-hexopyranosyl)oxy]-1-oxohexyl]-α-L-arabino-hexopyranosyl]oxy]-2-hexenoate.
1H NMR (400 MHz, CD3OD) δ 0.08 (12H, s), 0.90 (9H, s), 0.92 (9H, s), 1.07 (3H, d, J = 6.2 Hz), 1.10 (3H, d, J = 6.1 Hz), 1.17 (3H, d, J = 5.3 Hz), 1.19 (3H, d, J = 5.1 Hz), 1.46 (2H, m), 1.54 (2H, m), 1.63 (1H, m), 1.79 (2H, m), 2.02 (1H, dt, J = 11.9 Hz, J = 4.1 Hz), 2.27 (2H, m), 2.45 (2H, t, J = 7.1 Hz), 3.63 (2H, m), 3.72 (1H, s.br), 3.79 (3H, m), 3.95 (1H, m), 4.53 (1H, s), 4.68 (1H, s), 4.84 (1H, m), 5.19 (2H, s), 5.98 (1H, d, J = 15.8 Hz), 7.07 (1H, dt, J = 14.4 Hz, J = 7.7 Hz), 7.31 (5H, m); ESI-(+)-HR-MS: obs. m/z 845.4675 [M + Na]+, calc. m/z 845.4662 for C43H74ONaO11Si2.
(4R)-4-[[3,6-Dideoxy-2-O-[(5R)-5-[(2,4-di-O-tert-butyldimethylsilyl-3,6-dideoxy-α-L-arabino-hexopyranosyl)oxy]-1-oxohexyl]-α-L-arabino-hexopyranosyl]oxy]-pentanoic acid.
A solution of benzyl (2E,4R)-4-[[3,6-dideoxy-2-O-[(5R)-5-[(2,4-di-O-tert-butyldimethylsilyl-3,6-dideoxy-α-L-arabino-hexopyranosyl)oxy]-1-oxohexyl]-α-L-arabino-hexopyranosyl]oxy]-2-pentenoate (2.6 mg, 3.21 μmol) in methanol (0.5 mL) was treated with 10 mol% palladium on carbon (10 mg) and hydrogenated under H2 atmosphere (1 atm). After stirring for 4 h the catalyst was removed by filtration over Celite and the product was obtained without further purification by concentration under reduced pressure to afford (4R)-4-[[3,6-dideoxy-2-O-[(5R)-5-[(2,4-di-O-tert-butyldimethylsilyl-3,6-dideoxy-α-L-arabino-hexopyranosyl)oxy]-1-oxohexyl]-α-L-arabino-hexopyranosyl]oxy]-pentanoic acid (2.1 mg, 2.91 μmol, 90% yield) as a colourless oil. 1H NMR (400 MHz, CD3OD) δ 0.08 (12H, s), 0.90 (9H, s), 0.92 (9H, s), 1.12 (3H, d, J = 6.1 Hz), 1.14 (3H, d, J = 6.0 Hz), 1.16 (3H, d, J = 6.0 Hz), 1.18 (3H, d, J = 6.1 Hz), 1.49 (2H, m), 1.62 (1H, m), 1.81 (6H, m), 2.04 (1H, td, J = 12.2 Hz, J = 4.0 Hz), 2.36 (4H, m), 3.63 (2H, m), 3.72 (1H, s.br), 3.82 (3H, m), 4.54 (1H, s), 4.69 (1H, s), 4.88 (1H, m); 13C NMR (100 MHz, CD3OD) δ −4.8 (4C), 17.9, 18.4, 18.5, 19.1, 22.2, 26.0 (6C), 32.5, 32.9, 34.0, 34.6, 37.4, 37.7, 68.1, 69.3, 70.0, 71.0, 71.2, 71.4, 71.9, 72.1, 97.1, 97.7; ESI-(−)-HR-MS: obs. m/z 719.4237 [M − H]−, calc. m/z 719.4227 for C35H67O11Si2.
(4R)-4-[[3,6-Dideoxy-4-O-[(5R)-5-[(2,4-di-O-tert-butyldimethylsilyl-3,6-dideoxy-α-L-arabino-hexopyranosyl)oxy]-1-oxohexyl]-α-L-arabino-hexopyranosyl]oxy]-pentanoic acid.
Benzyl (2E,4R)-4-[[3,6-dideoxy-4-O-[(5R)-5-[(2,4-di-O-tert-butyldimethylsilyl-3,6-dideoxy-α-L-arabino-hexopyranosyl)oxy]-1-oxohexyl]-α-L-arabino-hexopyranosyl]oxy]-2-pentenoate (2.1 mg, 2.60 μmol) was hydrogenated as described for the 2′-(asc-C6)-asc-C5 derivative to afford (4R)-4-[[3,6-dideoxy-4-O-[(5R)-5-[(2,4-di-O-tert-butyldimethylsilyl-3,6-dideoxy-α-L-arabino-hexopyranosyl)oxy]-1-oxohexyl]-α-L-arabino-hexopyranosyl]oxy]-pentanoic acid (1.8 mg, 2.50 μmol, 96% yield) as a colourless oil. 1H NMR (400 MHz, CD3OD) δ 0.08 (12H, s), 0.90 (9H, s), 0.93 (9H, s), 1.12 (3H, d, J = 6.4 Hz), 1.14 (3H, d, J = 6.8 Hz), 1.18 (3H, d, J = 5.8 Hz), 1.23 (3H, d, J = 6.1 Hz), 1.55 (2H, m), 1.68 (1H, m), 1.79 (5H, m), 1.90 (1H, m), 2.00 (1H, m), 2.25 (1H, m), 2.34 (1H, m), 2.40 (2H, t, J = 7.0 Hz), 3.39 (1H, m), 3.66 (3H, m), 3.81 (3H, m), 4.54 (1H, s), 4.72 (1H, s), 4.78 (1H, s br); 13C NMR (100 MHz, CD3OD) δ −4.8 (4), 17.8, 18.3, 18.8, 19.0, 22.0, 26.0 (6), 33.0, 34.6, 34.6, 34.7, 37.1, 37.7, 68.5, 70.0, 70.4, 71.2, 71.3, 71.9, 72.2, 72.5, 94.1, 97.8; ESI-(−)-HR-MS: obs. m/z 719.4241 [M − H]−, calc. m/z 719.4227 for C35H67O11Si2.
(5R)-5-[[3,6-Dideoxy-2-O-[(5R)-5-[(2,4-di-O-tert-butyldimethylsilyl-3,6-dideoxy-α-L-arabino-hexopyranosyl)oxy]-1-oxohexyl]-α-L-arabino-hexopyranosyl]oxy]-hexanoic-acid.
Benzyl (2E,5R)-5-[[3,6-dideoxy-2-O-[(5R)-5-[(2,4-di-O-tert-butyldimethylsilyl-3,6-dideoxy-α-L-arabino-hexopyranosyl)oxy]-1-oxohexyl]-α-L-arabino-hexopyranosyl]oxy]-2-hexenoate (2.4 mg, 2.89 μmol) was hydrogenated as described for the 2′-(asc-C6)-asc-C5 derivative to afford (5R)-5-[[3,6-dideoxy-2-O-[(5R)-5-[(2,4-di-O-tert-butyldimethylsilyl-3,6-dideoxy-α-L-arabino-hexopyranosyl)oxy]-1-oxohexyl]-α-L-arabino-hexopyranosyl]oxy]-hexanoic acid (1.5 mg, 2.04 μmol, 70% yield) as a colourless oil. 1H NMR (400 MHz, CD3OD) δ 0.08 (12H, s), 0.90 (9H, s), 0.92 (9H, s), 1.12 (3H, d, J = 5.8 Hz), 1.13 (3H, d, J = 5.8 Hz), 1.17 (3H, d, J = 6.0 Hz), 1.22 (3H, d, J = 6.1 Hz), 1.59 (6H, m), 1.83 (5H, m), 2.00 (1H, m), 2.21 (2H, m), 2.40 (2H, m), 3.39 (1H, m), 3.66 (3H, m), 3.80 (3H, m), 4.54 (1H, s), 4.71 (1H, s), 4.78 (1H, s.br); 13C NMR (100 MHz, CD3OD) δ −4.9 (4C), 17.9, 18.3 (2C), 18.9, 22.1, 23.6, 26.0 (6C), 32.7, 34.8, 37.7, 37.7, 38.1, 38.5, 67.8, 69.3, 69.9, 70.9, 71.1, 71.3, 72.1, 72.4, 97.3, 97.7; ESI-(−)-HR-MS: obs. m/z 733.4399 [M − H]−, calc. m/z 733.4384 for C36H69O11Si2.
(5R)-5-[[3,6-Dideoxy-4-O-[(5R)-5-[(2,4-di-O-tert-butyldimethylsilyl-3,6-dideoxy-α-L-arabino-hexopyranosyl)oxy]-1-oxohexyl]-α-L-arabino-hexopyranosyl]oxy]-hexanoic acid.
Benzyl (2E,5R)-5-[[3,6-dideoxy-4-O-[(5R)-5-[(2,4-di-O-tert-butyldimethylsilyl-3,6-dideoxy-α-L-arabino-hexopyranosyl)oxy]-1-oxohexyl]-α-L-arabino-hexopyranosyl]oxy]-2-hexenoate (1.1 mg, 1.32 μmol) was hydrogenated as described for the 2′-(asc-C6)-asc-C5 derivative to afford (5R)-5-[[3,6-dideoxy-4-O-[(5R)-5-[(2,4-di-O-tert-butyldimethylsilyl-3,6-dideoxy-α-L-arabino-hexopyranosyl)oxy]-1-oxohexyl]-α-L-arabino-hexopyranosyl]oxy]-hexanoic acid (0.9 mg, 1.22 μmol, 92% yield) as a colourless oil. 1H NMR (400 MHz, CD3OD) δ 0.08 (12H, s), 0.90 (9H, s), 0.92 (9H, s), 1.12 (9H, m), 1.14 (9H, d, J = 6.1 Hz), 1.17 (3H, d, J = 6.1 Hz), 1.49 (2H, m), 1.62 (4H, m), 1.76 (3H, m), 1.85 (2H, m), 2.03 (1H, m), 2.20 (2H, t, J = 7.3 Hz), 2.35 (2H, m), 3.62 (2H, m), 3.71 (1H, s br), 3.80 (3H, m), 3.87 (1H, m), 4.54 (1H, s), 4.68 (1H, s), 4.86 (1H, m); 13C NMR (100 MHz, CD3OD) δ −4.9 (4C), 17.9, 18.3, 18.6, 18.9, 22.1, 23.6, 26.0 (6C), 32.7, 34.8, 37.7, 37.7, 38.1, 38.5, 67.8, 69.3, 69.9, 70.9, 71.1, 71.3, 72.1, 72.4, 97.3, 97.7; ESI-(−)-HR-MS: obs. m/z 733.4392 [M − H]−, calc. m/z 733.4384 for C36H69O11Si2.
(4R)-4-[[3,6-Dideoxy-2-O-[(5R)-5-[(3,6-dideoxy-α-L-arabino-hexopyranosyl)oxy]-1-oxohexyl]-α-L-arabino-hexopyranosyl]oxy]-pentanoic acid (2′-(asc-C6)-asc-C5, 7).
In a 2 ml polypropylene cryovial a solution of (4R)-4-[[3,6-dideoxy-2-O-[(5R)-5-[(2,4-di-O-tert-butyldimethylsilyl-3,6-dideoxy-α-L-arabino-hexo-pyranosyl)oxy]-1-oxohexyl]-α-L-arabino-hexopyranosyl]oxy]-pentanoic acid (2.1 mg, 2.91 μmol) in dry tetrahydrofuran (500 μL) was treated with dry pyridine (40 μL, 500 μmol) and Olah's reagent (70% (w/w) hydrogen fluoride in pyridine, 13 μl, 500 μmol). After stirring for 10 days the reaction was quenched by transfer into a 4 ml glass vial and addition of saturated aqueous sodium hydrogencarbonate solution (0.1 mL), dried over sodium sulphate, filtered, and concentrated under reduced pressure. The residue was fractionated by solid phase extraction (SPE) on a 100 mg reverse phase C18ec cartridge (Chromabond, Macherey Nagel) using a stepwise gradient of methanol in water (0–100% in 10% steps, v/v) as eluent to afford (4R)-4-[[3,6-dideoxy-2-O-[(5R)-5-[(3,6-dideoxy-α-L-arabino-hexopyranosyl)oxy]-1-oxohexyl]-α-L-arabino-hexopyranosyl]oxy]-pentanoic acid (7, 0.5 mg, 1.02 μmol, 35% yield) as a colourless oil identical to the natural product from C. nigoni. 1H NMR (400 MHz, CD3OD) δ 1.14 (3H, d, J = 6.1 Hz), 1.14 (3H, d, 6.1 Hz), 1.23 (3H, d, J = 6.1 Hz), 1.23 (3H, d, J = 6.1 Hz), 1.56 (2H, m), 1.70 (1H, m), 1.77 (1H, ddd, J = 13.2 Hz, J = 11.1 Hz, J = 3.0 Hz), 1.80 (3H, m), 1.90 (1H, ddd, J = 13.4 Hz, J = 11.8 Hz, J = 3.4 Hz), 1.96 (1H, dt, J = 13.2 Hz, J = 3.7 Hz), 2.01 (1H, dt, J = 13.0 Hz, J = 3.8 Hz), 2.34 (1H, m), 2.37 (1H, m), 2.39 (2H, dt, J = 3.2 Hz, J = 7.3 Hz), 3.40 (ddd, J = 11.4 Hz, J = 9.6 Hz, J = 4.4 Hz), 3.52 (1H, ddd, J = 11.2 Hz, J = 9.5 Hz, J = 4.4 Hz), 3.62 (1H, dq, J = 9.3 Hz, J = 6.2 Hz), 3.71 (1H, dq, J = 9.6 Hz, J = 6.2 Hz), 3.73 (1H, s.br), 3.81 (1H, m), 3.83 (1H, m), 4.65 (1H, s), 4.71 (1H, s), 4.79 (1H, s.br); 13C NMR (100 MHz, CD3OD) δ 17.9, 17.9, 18.7, 18.7, 21.8, 33.4, 34.0, 34.6, 34.9, 35.1, 37.2, 68.2, 68.2, 70.1, 70.1, 70.9, 72.2, 72.2, 72.3, 94.1, 97.6 (Table S3†); ESI-(−)-HR-MS: obs. m/z 491.2502 [M − H]−, calc. m/z 491.2498 for C23H39O11.
(5R)-5-[[3,6-Dideoxy-2-O-[(5R)-5-[(3,6-dideoxy-α-L-arabino-hexopyranosyl)oxy]-1-oxohexyl]-α-L-arabino-hexopyranosyl]oxy]-hexanoic acid (2′-(asc-C6)-asc-C6, 8).
(5R)-5-[[3,6-Dideoxy-2-O-[(5R)-5-[(2,4-di-O-tert-butyldimethylsilyl-3,6-dideoxy-α-L-arabino-hexopyranosyl)oxy]-1-oxohexyl]-α-L-arabino-hexopyranosyl]oxy]-hexanoic acid (1.5 mg, 2.03 μmol) was treated as described for 7 to afford (5R)-5-[[3,6-dideoxy-2-O-[(5R)-5-[(3,6-dideoxy-α-L-arabino-hexopyranosyl)oxy]-1-oxohexyl]-α-L-arabino-hexopyranosyl]oxy]-hexanoic acid (8, 0.5 mg, 0.99 μmol, 49% yield) as a colourless oil identical to the natural product from C. nigoni. 1H NMR (400 MHz, CD3OD) δ 1.14 (3H, d, J = 6.1 Hz), 1.14 (3H, d, 6.2 Hz), 1.22 (3H, d, J = 6.3 Hz), 1.22 (3H, d, J = 6.3 Hz), 1.57 (4H, m), 1.65(1H, m), 1.70 (1H, m), 1.76 (1H, m), 1.77 (1H, ddd, J = 13.3 Hz, J = 11.2 Hz, J = 3.1 Hz), 1.79 (1H, m), 1.88 (1H, ddd, J = 13.4 Hz, J = 11.5 Hz, J = 3.0 Hz), 1.95 (1H, dt, J = 13.4 Hz, J = 3.8 Hz), 2.00 (1H, dt, J = 13.2 Hz, J = 3.6 Hz), 2.24 (2H, t, J = 7.3 Hz), 2.39 (2H, dt, J = 3.1 Hz, J = 7.3 Hz), 3.41 (ddd, J = 11.3 Hz, J = 9.7 Hz, J = 4.7 Hz), 3.51 (1H, ddd, J = 11.1 Hz, J = 9.6 Hz, J = 4.6 Hz), 3.62 (1H, dq, J = 9.5 Hz, J = 6.3 Hz), 3.70 (1H, dq, J = 9.5 Hz, J = 6.3 Hz), 3.72 (1H, s.br), 3.79 (1H, m), 3.80 (1H, m), 4.65 (1H, s), 4.71 (1H, s), 4.78 (1H, s.br); 13C NMR (100 MHz, CD3OD) δ 17.8, 17.8, 18.7, 18.7, 22.6, 22.6, 33.1, 34.7, 35.7, 36.8, 37.4, 37.4, 68.3, 68.5, 70.1, 70.1, 70.9, 72.2, 72.2, 72.3, 94.3, 97.4 (Table S3†); ESI-(−)-HR-MS: obs. m/z 505.2657 [M − H]−, calc. m/z 505.2654 for C24H41O11.
(4R)-4-[[3,6-Dideoxy-4-O-[(5R)-5-[(3,6-dideoxy-α-L-arabino-hexopyranosyl)oxy]-1-oxohexyl]-α-L-arabino-hexopyranosyl]oxy]-pentanoic acid (4′-(asc-C6)-asc-C5, 13a)
(4R)-4-[[3,6-Dideoxy-4-O-[(5R)-5-[(2,4-di-O-tert-butyldimethylsilyl-3,6-dideoxy-α-L-arabino-hexo-pyranosyl)oxy]-1-oxohexyl]-α-L-arabino-hexopyranosyl]oxy]-pentanoic acid (1.8 mg, 2.50 μmol) was treated as described for 7 to afford (4R)-4-[[3,6-dideoxy-4-O-[(5R)-5-[(3,6-dideoxy-α-L-arabino-hexopyranosyl)oxy]-1-oxohexyl]-α-L-arabino-hexopyranosyl]oxy]-pentanoic acid (13a, 0.6 mg, 1.22 μmol, 49% yield) as a colourless oil. 1H NMR (400 MHz, CD3OD) δ 1.13 (3H, d, J = 6.2 Hz), 1.14 (3H, d, J = 6.3 Hz), 1.16 (3H, d, J = 6.2 Hz), 1.22 (3H, d, J = 6.2 Hz), 1.56 (2H, m), 1.67 (1H, m), 1.76 (1H, ddd, J = 13.1 Hz, J = 11.1 Hz, J = 3.0 Hz), 1.79 (1H, m), 1.80 (2H, m), 1.85 (1H, ddd, J = 13.3 Hz, J = 11.3 Hz, J = 3.0 Hz), 1.95 (1H, dt, J = 13.0 Hz, J = 3.8 Hz), 2.04 (1H, dt, J = 12.9 Hz, J = 4.2 Hz), 2.35 (2H, m), 2.40 (2H, t, J = 7.4 Hz), 3.51 (1H, ddd, J = 11.0 Hz, J = 9.5 Hz, J = 4.6 Hz), 3.61 (1H, dq, J = 9.4 Hz, J = 6.2 Hz), 3.72 (1H, s.br), 3.73 (1H, s.br), 3.80 (1H, m), 3.85 (1H, m), 3.85 (1H, dq, J = 9.7 Hz, J = 6.2 Hz), 4.64 (1H, s), 4.69 (1H, s), 4.87 (1H, ddd, J = 11.3 Hz, J = 9.7 Hz, J = 4.5 Hz); 13C NMR (100 MHz, CD3OD) δ 17.8, 18.5, 18.5, 18.5, 22.3, 31.4, 33.2, 33.2, 34.8, 35.6, 37.3, 68.2, 68.2, 69.6, 69.6, 71.1, 71.1, 71.7, 71.9, 97.2, 97.4 (Table S3†); ESI-(−)-HR-MS: obs. m/z 491.2503 [M − H]−, calc. m/z 491.2498 for C23H39O11.
(5R)-5-[[3,6-Dideoxy-4-O-[(5R)-5-[(3,6-dideoxy-α-L-arabino-hexopyranosyl)oxy]-1-oxohexyl]-α-L-arabino-hexopyranosyl]oxy]-hexanoic acid (4′-(asc-C6)-asc-C6, 13b).
(5R)-5-[[3,6-Dideoxy-4-O-[(5R)-5-[(2,4-di-O-tert-butyldimethylsilyl-3,6-dideoxy-α-L-arabino-hexo-pyranosyl)oxy]-1-oxohexyl]-α-L-arabino-hexopyranosyl]oxy]-hexanoic acid (0.9 mg, 1.28 μmol) was treated as described for 7 to afford (5R)-5-[[3,6-dideoxy-4-O-[(5R)-5-[(3,6-dideoxy-α-L-arabino-hexopyranosyl)oxy]-1-oxohexyl]-α-L-arabino-hexopyranosyl]oxy]-hexanoic acid (13b, 0.2 mg, 0.39 μmol, 30% yield) as a colourless oil. 1H NMR (400 MHz, CD3OD) δ 1.13 (3H, d, J = 6.2 Hz), 1.14 (3H, d, J = 6.2 Hz), 1.14 (3H, d, J = 6.2 Hz), 1.22 (3H, d, J = 6.2 Hz), 1.57 (4H, m), 1.67 (2H, m), 1.76 (1H, ddd, J = 13.2 Hz, J = 11.2 Hz, J = 3.0 Hz), 1.77 (1H, m) 1.78 (1H, m), 1.85 (1H, ddd, J = 12.9 Hz, J = 1.4 Hz, J = 2.9 Hz), 1.95 (1H, dt, J = 13.2 Hz, J = 3.9 Hz), 2.03 (1H, dt, J = 12.8 Hz, J = 4.0 Hz), 2.25 (2H, t, J = 7.2 Hz), 2.35 (2H, dt, J = 5.2 Hz, J = 7.2 Hz), 3.51 (1H, ddd, J = 11.2 Hz, J = 9.4 Hz, J = 4.5 Hz), 3.61 (1H, dq, J = 9.5 Hz, J = 6.1 Hz), 3.72 (2H, s.br), 3.80 (2H, m), 3.86 (1H, dq, J = 9.7 Hz, J = 6.2 Hz), 4.64 (1H, s), 4.69 (1H, s), 4.86 (1H, ddd, J = 11.4 Hz, J = 9.7 Hz, J = 4.6 Hz); 13C NMR (100 MHz, CD3OD) δ 17.9, 18.7, 18.7, 18.7, 22.6, 22.6, 32.9, 34.8, 35.8, 36.8, 37.6, 37.6, 67.9, 68.0, 69.5, 69.5, 71.0, 71.3, 72.1, 72.1, 97.3, 97.3 (Table S3†); ESI-(−)-HR-MS: obs. m/z 505.2656 [M − H]−, calc. m/z 505.2654 for C24H41O11.
Conflicts of interest
There are no conflicts to declare.
Acknowledgements
Financial support by the Max Planck Society (MPG), the Jena School of Microbial Communication (JSMC), the Swiss National Science Foundation (SNSF-169700), and the University of Neuchâtel (UniNE) is gratefully acknowledged. We thank Dr Michael Reichelt (Department for Biochemistry, MPICE, Jena) for acquisition of the MS/MS precursor ion scan. All nematode strains were provided by the Caenorhabditis Genetics Center, which is funded by NIH Office of Research Infrastructure Programs (P40 OD010440). Open Access funding provided by the Max Planck Society.
References
- S. H. von Reuss and F. C. Schroeder, Nat. Prod. Rep., 2015, 32, 994–1006 RSC.
- F. C. Schroeder, Chem. Biol., 2015, 22, 7–16 CrossRef CAS PubMed.
- R. A. Butcher, Nat. Chem. Biol., 2017, 13, 577–586 CrossRef CAS PubMed.
- R. A. Butcher, Nat. Prod. Rep., 2017, 34, 472–477 RSC.
- M. Viney and S. Harvey, PLoS Genet., 2017, 13, e1007046 CrossRef PubMed.
- S. H. von Reuss, Chimia, 2018, 72, 297–303 CrossRef CAS PubMed.
- P. T. McGrath and I. Ruvinsky, Curr. Opin. Syst. Biol., 2019, 13, 23–30 CrossRef PubMed.
- J. Y. Park, H.-J. Joo, S. Park and Y.-K. Paik, Int. J. Mol. Sci., 2019, 20, 3898 CrossRef CAS PubMed.
- A. Choe, S. H. von Reuss, D. Kogan, R. B. Gasser, E. G. Platzer, F. C. Schroeder and P. W. Sternberg, Curr. Biol., 2012, 22, 772–780 CrossRef CAS PubMed.
- S. H. von Reuss, N. Bose, J. Srinivasan, J. J. Yim, J. C. Judkins, P. W. Sternberg and F. C. Schroeder, J. Am. Chem. Soc., 2012, 134, 1817–1824 CrossRef CAS PubMed.
- X. Zhang, J. H. Noguez, Y. Zhou and R. A. Butcher, Methods Mol. Biol., 2013, 1068, 71–92 CrossRef CAS PubMed.
- S. H. von Reuss, F. Dolke and C. Dong, Anal. Chem., 2017, 89, 10570–10577 CrossRef CAS PubMed.
- C. Pungaliya, J. Srinivasan, B. W. Fox, R. U. Malik, A. H. Ludewig, P. W. Sternberg and F. C. Schroeder, Proc. Natl. Acad. Sci. U. S. A., 2009, 106, 7708–7713 CrossRef CAS PubMed.
- Y. Izrayelit, S. L. Robinette, N. Bose, S. H. von Reuss and F. C. Schroeder, ACS Chem. Biol., 2013, 8, 314–319 CrossRef CAS PubMed.
- A. B. Artyukhin, Y. K. Zhang, A. E. Akagi, O. Panda, P. W. Sternberg and F. C. Schroeder, J. Am. Chem. Soc., 2018, 140, 2841–2852 CrossRef CAS PubMed.
- K. A. Hollister, E. S. Conner, X. Zhang, M. Spell, G. M. Bernard, P. Patel, A. C. de Carvalho, R. A. Butcher and J. R. Ragains, Bioorg. Med. Chem., 2013, 21, 5754–5769 CrossRef CAS PubMed.
- C. Dong, D. K. Reilly, C. Bergame, F. Dolke, J. Srinivasan and S. H. von Reuss, J. Org. Chem., 2018, 83, 7109–7120 CrossRef CAS PubMed.
- A. Choe, T. Chuman, S. H. von Reuss, A. T. Dossey, J. Yim, R. Ajredini, A. A. Kolawa, F. Kaplan, H. T. Alborn, P. E. Teal, F. C. Schroeder, P. W. Sternberg and A. S. Edison, Proc. Natl. Acad. Sci. U. S. A., 2012, 109, 20949–20954 CrossRef CAS PubMed.
- C. P. Bergame, C. Dong, S. Sutour and S. H. von Reuss, Org. Lett., 2019, 21, 9889–9892 CrossRef CAS PubMed.
- R. A. Butcher, J. R. Ragains and J. Clardy, Org. Lett., 2009, 11, 3100–3103 CrossRef CAS PubMed.
- J. Srinivasan, S. H. von Reuss, N. Bose, A. Zaslaver, P. Mahanti, M. C. Ho, O. G. O'Doherty, A. S. Edison, P. W. Sternberg and F. C. Schroeder, PLoS Biol., 2012, 10, e1001237 CrossRef CAS PubMed.
- Y. Zhou, X. Zhang and R. A. Butcher, ACS Chem. Biol., 2019, 14, 50–57 CrossRef CAS PubMed.
- C. Dong, F. Dolke and S. H. von Reuss, Org. Biomol. Chem., 2016, 14, 7217–7225 RSC.
- F. Dolke, C. Dong, S. Bandi, C. Paetz, G. Glauser and S. H. von Reuss, Org. Lett., 2019, 21, 5832–5837 CrossRef CAS PubMed.
- P.-Y. Jeong, M. Jung, Y.-H. Yim and Y.-K. Paik, Nature, 2005, 433, 541–545 CrossRef CAS PubMed.
- R. Martin, T. Schaefer, G. Theumer, E. V. Entchev, T. V. Kurzchalia and H.-J. Knoelker, Synthesis, 2009, 3488–3492 CAS.
- R. R. Schmidt and J. Michel, Angew. Chem., Int. Ed. Engl., 1980, 19, 731–732 CrossRef.
- M. Scholl, S. Ding, C. W. Lee and R. H. Grubbs, Org. Lett., 1999, 1, 953–956 CrossRef CAS PubMed.
- B. Neises and W. Steglich, Angew. Chem., Int. Ed. Engl., 1978, 17, 522–524 CrossRef.
- Small Molecule identifier (SMID) see http://www.smid-db.org.
- N. Bose, A. Ogawa, S. H. von Reuss, J. J. Yim, E. J. Ragsdale, R. J. Sommer and F. C. Schroeder, Angew. Chem., Int. Ed., 2012, 51, 12438–12443 CrossRef CAS PubMed.
- J. M. Falcke, N. Bose, A. B. Artyukhin, C. Rödelsperger, G. V. Markov, J. J. Yim, D. Grimm, M. H. Claassen, O. Panda, J. A. Baccile, Y. K. Zhang, H. H. Le, D. Jolic, F. C. Schroeder and R. J. Sommer, Cell Chem. Biol., 2018, 25, 787–796.e12 CrossRef CAS PubMed.
-
T. Stiernagle, WormBook, 2006, vol. 11, pp. 1–11 Search PubMed.
Footnotes |
† Electronic supplementary information (ESI) available: Figures as indicated in the main text, NMR spectra of isolated and synthetic compounds. See DOI: 10.1039/d0ob00799d |
‡ These authors contributed equally. |
§ Present Address: Department of Integrative Evolutionary Biology, Max Planck Institute for Developmental Biology, Max-Planck-Ring 9, D-72076 Tübingen, Germany. |
|
This journal is © The Royal Society of Chemistry 2020 |
Click here to see how this site uses Cookies. View our privacy policy here.