DOI:
10.1039/D0FO01728K
(Paper)
Food Funct., 2020,
11, 8648-8658
Bolus rheology and ease of swallowing of particulated semi-solid foods as evaluated by an elderly panel†
Received
2nd July 2020
, Accepted 14th August 2020
First published on 1st September 2020
Abstract
Preparation of a bolus is a complex process with both food comminution and degree of lubrication with saliva playing an important role in a safe swallow. Swallowing disorders i.e. dysphagia, are especially present among the elderly population and often lead to choking and further health complications. The aim of this research was to investigate the relationship between the perception of ease of swallowing in the elderly and the rheological parameters of particulated foods, using broccoli purees as a model system. Particulated foods can be described as a concentrated dispersion of plant particles in a fluid phase. The effect of the fluid phase (Newtonian vs. shear thinning) and dispersed phase (plant particles with different size distribution and morphology) on the rheological properties of simulated boli was studied by characterising shear viscosity, viscoelasticity, yield stress, extensional viscosity and cohesiveness. Ease of swallowing and mouthfeel were evaluated by a semi trained healthy elderly panel (n = 19, aged 61 to 81). Ease of swallowing was correlated with the presence of yield stress and extensional viscosity in the bolus, characteristic of boli with xanthan gum as the fluid phase. Although the properties of the fluid phase played a dominant role in the ease of swallowing, compared to the dispersed phase, both components played a role in the rheological properties of the bolus and the perception of ease of swallowing by the elderly panel. These results provide insights into the design of personalised foods for populations with specific needs such as those suffering from swallowing disorders.
Introduction
Food structure and texture change from the moment food is chewed and mixed with saliva to form a cohesive mass or bolus. Due to the dynamic nature of the oral process, bolus properties determine swallowability to a larger extent than food properties. Populations with swallowing disorders i.e. dysphagia, struggle to consume regular foods, which often leads to choking and aspirational pneumonia due to food entering the airway instead of the oesophagus.1 Texture modification of foods is the most common clinical strategy to aid those suffering from dysphagia, where solid food is processed to reduce the particle size in order to eliminate or minimise the chewing phase during oral processing. Often these foods are reconstituted with the aid of thickeners to increase the visual appeal, which also increases the viscosity of the food. Increasing bolus viscosity reduces the flow of the food during swallowing, allowing sufficient time for individuals with dysphagia to close the airway before the arrival of the bolus, which they are unable to do with low viscosity boli.2 However, high viscosity boli may require additional effort to swallow and could leave residues in the pharynx, which can lead to further health complications. In addition to high shear viscosity, other rheological parameters such as extensional viscosity and viscoelasticity also play an important role in determining ease of swallowing.3,4 However, there is no single convention with respect to which rheological properties determine food swallowability, especially for complex systems such as plant-based particulated foods.
Plant-based particulated foods such as soups, purees, and smoothies are suspensions of plant particles (cell clusters, single cells and cell wall fragments) in a fluid phase containing soluble polymers, organic acid and salts5 The food structure of plant-based particulated foods can be designed by using different processing conditions. For instance, by simply exchanging the order of thermal and mechanical treatments, broccoli and carrot purees had different particle shapes, size distributions and rheological properties.4,5 Among the most common texturisers added to foods for dysphagia is xanthan gum, a bacterial polysaccharide produced by fermentation which has been shown to maintain a coherent bolus during flow, leading to the perception of easy to swallow.6 Due to its structural rigidity and rod-like conformation of its linear, cellulosic backbone, xanthan behaves rheologically as a non-Newtonian shear-thinning fluid;7 this means that its viscosity decreases with increasing shear rate. Furthermore, xanthan gum presents an apparent yield stress i.e. a minimum stress is required to flow. The effect of xanthan on the rheological properties of plant cell wall systems has been previously investigated in carrot suspensions, showing that it depends on the particle concentration.8 At low concentrations, xanthan increased the viscoelastic properties of the suspension, whilst at high concentrations, such as those in reconstituted foods for dysphagia, xanthan did not show a significant effect, as the viscoelastic behaviour was dominated by the plant particle network, with xanthan molecules entrapped in the interstitial regions.
Understanding bolus rheology is a key parameter in designing foods for dysphagia. Shear viscosity, yield stress, and viscoelasticity (G′ and G′′) are useful parameters to describe bolus properties; however, they are not sufficient to describe bolus behaviour during oral processing. Bolus behaviour under extensional flow, such as the one in the pharyngeal cavity during swallowing, is of critical importance to determine bolus flow and ease of swallowing.9
In this study we used broccoli purees as a model system to investigate how the different components of plant-based particulated foods, i.e. dispersed and fluid phases, impacted bolus rheology and ease of swallowing. Suspensions were produced with two different dispersed phases i.e. different particle size distributions and morphologies and two different fluid phases i.e. water as Newtonian fluid and a xanthan solution as shear thinning fluid. Food boli were produced by mixing the purees with artificial saliva and analysed for shear and extensional viscosities, yield stress, viscoelasticity and cohesiveness, and correlated with the swallowing perception by elderly panellists. Our results contribute to the knowledge on how food composition affects bolus rheology and ease of swallowing and would aid in the design of personalised foods for people suffering from dysphagia.
Materials and methods
Materials
Broccoli (Brassica oleracea) was purchased from a local supermarket in Sweden and used for sample preparation after a maximum of 3 days storage at 4 °C. The chemicals and enzymes used to produce the artificial saliva were: ammonium carbonate (Sigma-Aldrich, USA), potassium chloride (Merck, Germany), sodium bicarbonate (Across organics, Spain), potassium dihydrogen phosphate (Merck, Germany), magnesium chloride hexahydrate (Emsure, Germany), calcium chloride dihydrate (Merck, Germany), alfa amylase (Sigma-Aldrich, USA) and mucin from porcine stomach type II (Sigma-Aldrich, USA). Xanthan gum was purchased from Sigma-Aldrich.
Sample preparation
Each broccoli head was cut into 5–8 cm pieces and the large stalks of the broccoli were discarded. Broccoli pieces were cooked using two different methods: heating followed by blending (HB) or blending followed by heating (BH). For the HB method, the broccoli was covered with water and cooked at 90 °C (core temperature measured with a thermocouple) for 20 minutes. The broccoli was mixed with water at a mass ratio of 0.7
:
1 water
:
broccoli and blended for 3 minutes at maximum speed in a kitchen blender (TURBO blender, Moulinex; France). For the BH method, the broccoli was mixed with water at the same water
:
broccoli ratio as for the HB method, blended for 3 minutes and then heated using the same parameters as the HB method.
The samples were centrifuged for 30 minutes at 4000g (Centrifuge Universal 320|320 R, Hettich, Germany) and the pellet was collected from several batches, in order to produce sufficient broccoli particles for all the experiments.
The pellet obtained from each of the cooking methods (HB and BH) was mixed with two different fluid phases, resulting in two sets of samples. The first set was prepared by dispersing different concentrations of the pellet (1, 2, 4, 6 and 8 wt%) in water, and the second set by dispersing in 0.5 wt% xanthan solution at the same concentration as in water. The suspensions were obtained by gently adding the pellet to the fluid phase and mixing with a magnetic stirrer. The pellet will be referred to as the dispersed phase throughout the manuscript.
Simulated bolus
Artificial saliva was produced based on the formulation described by Minekus et al.10 with mucin added (2 g L−1).11 A bolus was prepared by mixing broccoli suspensions and artificial saliva at a ratio of 1
:
1 (ref. 10) resulting in final particle concentrations of 0.5, 1, 2, 3 and 4 wt%.
Dry matter
The measurement of dry matter was carried out with the dehydration of pre-weighed samples in a 105 °C oven for 24 h (UNP 100-800, Memmert, Schawabach, Germany) for 24 hours. Two replicates were measured.
Light microscopy
Light microscopy was performed using a microscope (Microphot-FXA, Nikon) equipped with a video camera (DFK33UX264, the imaging Source Europe GmbH, Bremen Germany). The samples were diluted 10 times with deionised water. Two droplets were placed on a glass slide and covered. The samples were observed with a 10× dry objective lens and at least eight images were obtained per sample. NIS-ElementsD software was used to collect the images.
Particle size distribution
The particle size distribution (PSD) was measured after centrifugation, by laser light scattering (Mastersizer; Malvern Instruments Ltd, Malvern, UK). A refractive index of 1.53 for particles and 1.33 for water was used. The particle absorption index was set to 0.01. The particle size distribution was calculated from the intensity profile of the scattered light using the instrument software (Mastersizer 3000). The volume based (d4,3) and area based (d3,2) diameters were obtained for every sample.
Rheological measurements
Rheological measurements of shear viscosity, viscoelasticity and yield stress were performed on a strain-controlled rheometer (ARG2, TA Instrument, USA) using 40 mm diameter parallel plates. Paraffin oil was added at the edge of the sample and a solvent trap was used to prevent the sample from drying during the measurements. Measurements were performed at a gap of 1 mm and a temperature of 35 °C to mimic biological conditions in the oral cavity. Samples were held in the rheometer for 2 minutes, to ensure that the samples had equilibrated to 35 °C, prior to the measurement. The shear viscosity was measured using a steady state flow sweep by increasing the shear rate from 1 s−1 to 100 s−1. Viscoelastic properties were measured by using frequency sweeps from 10 to 0.1 Hz and a 0.1% strain selected from the linear viscoelastic region. From these measurements, the elastic modulus G′ and viscous modulus G′′ were obtained. Apparent yield stress was measured by the dynamic stress/strain sweep method, in which the elastic G′ and viscous moduli G′′ were measured as a function of the oscillation stress at a frequency of 1 Hz and a strain range between 0.01 and 300%. The apparent yield stress was obtained using TRIOS v.5.0 software (TA instruments, USA) to analyse plots of the storage modulus vs. oscillation stress. The used function creates two tangents to the curve and finds their intersection, the onset point, and the corresponding oscillation stress is selected as the apparent yield stress. Three replicates were measured.
Extensional viscosity
Extensional viscosity was measured at room temperature using a hyperbolic contraction flow geometry12 mounted on an Instron testing instrument (5542 Instron Corporation, Canton, MA, USA), where the samples were forced through a hyperbolic nozzle and the pressure drop over the nozzle was measured. Measurements were performed using a die with an inlet radius of 10 mm and outlet radius of 0.83 mm, imposing a total Hencky strain of 8–10 to the samples. The extensional strain rates were in the range of 10 to 120 s−1, and the extensional viscosity was obtained as described previously.12,13 Extensional viscosity of a simulated bolus containing only 0.5 wt% xanthan gum mixed with artificial saliva to a final concentration of 0.25 wt% was used as a reference. Three replicates were measured.
Cohesiveness
Cohesiveness of the different broccoli samples was determined with a Powder Flow Tester (PFT Powder Flow Tester, Brookfield Engineering Laboratories – Ametek Inc., USA) using the experimental methods described by Tobin et al.14 and Slettengren et al.15 with some modifications. A vane lid was used in the PFT measurements of the flow functions of the samples. The flow function tests were undertaken using the 263 cm3 volume shear cell. The standard flow function test program was used to measure the flow properties over the range of 4 major principal consolidation stresses in a geometric progression that generated values up to 3.5 kPa. The PFT was connected to a computer and Powder Flow Pro V1.2 software was used to collect the data. The flow functions of the broccoli samples represent the average values of at least 2 independent measurements.
The ratio of the consolidation stress σ1 and unconfined yield strength σc defines the flowability ffc (eqn (1))15
|  | (1) |
High flowability (ffc > 10) means that the sample is free-flowing, and low flowability (ffc < 1) means that the sample is non-flowing. When the flowability is determined at several consolidation stresses, it can be presented in a flow function plot.
Sensory evaluation
Descriptive sensory analysis was used to characterise the mouthfeel and ease of swallowing of broccoli suspensions. Four samples (6 wt% broccoli suspension, with HB or BH as the dispersed phase and water or xanthan as the fluid phase) were evaluated by 19 healthy panellists (14 females, 5 males) aged between 61 and 81 years (with an average age of 71). The panellists were selected from RISE's (Research Institutes of Sweden) consumer database, and most of the panellists had previously participated in sensory trials of different types of foods at RISE.
One product familiarisation session was held for 1 hour prior to sensory evaluation of the samples. The list of sensory attributes was prepared based on previous studies.16–19 During the familiarisation session, the protocol and the different sensory attributes were explained and discussed with the panellists to ensure that they were familiar with the samples and the vocabulary used to define the sensory attributes. Reference samples were also evaluated to understand the scales for rating the attributes.
Formal sessions were conducted in the sensory laboratory of RISE Research Institutes of Sweden, in isolated booths equipped with computers, temperature control (22 °C) and daylight equivalent. Samples were served in opaque plastic cups, with each panellist receiving a 20 mL sample of each sample. Samples were randomised and presented with a three-digit code. Panellists were instructed to use a full 5 mL spoon to evaluate the various attributes of the samples.
The sensory attributes were rated on a 5 point categorical scale with 1 = low and 5 = high. The sensory vocabulary, definitions and sensory reference standards were described as follows:
•Difficulty in swallowing: The attribute of ease of swallowing was rated as difficulty in swallowing, where 1 would indicate that there was no difficulty in swallowing i.e. easy to swallow and 5 would indicate that the sample was not easy to swallow.
•Effort required to swallow: Effort required to prepare the bolus for swallowing from low (no effort) to high (a lot of effort).
•Mouth-coating: Sensation of a layer forming on the surfaces of the mouth, from low (water) to high (cream).
•Particle size perception: The proportion of large particles felt on the oral surfaces of the mouth compared to small particles, from low (not many large particles) to high (a lot of large particles).
•Smoothness: The velvety feeling of the sample in the mouth, from low (water) to high (potato puree).
•Residues in mouth: The ease at which the sample clears from the mouth after swallowing. From easy to clear to very difficult to clear.
All the sensory attributes were evaluated using three spoonfuls of samples and the following protocol. The first spoonful was to rate the ease of swallowing. The panellists were instructed to consume and swallow the sample naturally and to start the timer as they put the puree in their mouth and stop the timer once the puree was swallowed. With the second spoonful the panellists were instructed to compress and rub the sample with the tongue against the palate and rate the attributes of smoothness and particle size sensation. The third spoonful was used to rate mouth coating, and effort required to prepare the bolus for swallowing. After swallowing the third spoonful, they were asked to rate residues in mouth. The sensory data were collected using EyeQuestion v.3.8.6, Logic 8 BV software.
Statistical analysis
Panel performance was assessed using XLSTAT panel performance software to assess discrimination power. Sensory scores of each attribute were analysed by two-way analysis of variance ANOVA, with the sample and panellist (and their interactions) as the variation sources. A statistically significant difference was defined as p < 0.05. If a significant difference in means was indicated by the ANOVA, comparisons were performed using Tukey's honest significant difference (HSD) test. Sensory data were also analysed by Principal Component Analysis (PCA). All these analyses were performed with XLSTAT statistical software (Addinsoft, New York, NY, USA).
The relationship between the sensory attributes and the different rheological parameters was explored throughout the use of Partial Least Squares Regression (PLS) and Hierarchical Cluster Analysis (HCA) using SIMCA 16.0.1.7928 software (Sartorium Stedim Data Analytics AB – Malmö, Sweden).
Results
Dispersed phase properties
Fig. 1 shows that processing conditions impacted both the particle size distribution and particle morphology of the broccoli suspensions, resulting in samples with high polydispersity. The particle size determined from dynamic light scattering measurements showed that particles obtained from the heat and blend process (HB) had a smaller average particle size and broader particle size distribution, compared to the blend and heat (BH) process which was characterised by a larger average particle size and narrower particle size distribution. The average d4,3 (volume weighted mean) was 221.3 μm and 409.7 μm for HB and BH, respectively. The average d3,2 (surface weighted mean) was 90.5 μm and 199.5 μm for HB and BH, respectively.
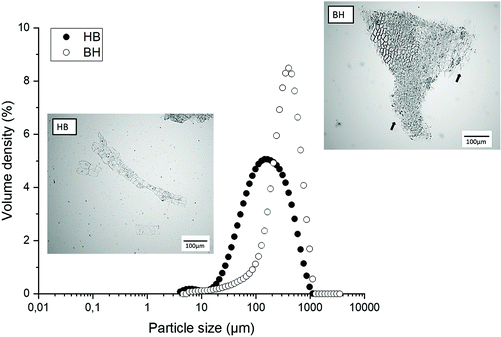 |
| Fig. 1 Particle size distribution of the dispersed phase obtained by exchanging the order of thermal and mechanical treatments, heating followed by blending (HB) and blending followed by heating (BH). Arrows indicate regions of broken cell walls in the cell clusters of BH particles. | |
Particles in the HB samples were characterised by clusters of cells separated by the middle lamella leading to smooth surfaces; in contrast particles from the BH process were larger clusters of cells with regions of cells broken across the cell wall leading to rough surfaces. These results are in agreement with previous work,5 which also showed that smaller particles were obtained from the HB process; during the heating step the pectin from the middle lamella is depolymerized resulting in reduced cell adhesion, causing the tissues to be disrupted through the middle lamella during blending, whereas in the BH process, disruption could occur across the middle lamella or through the cell walls, resulting in clusters with broken cell wall fragments on the surface.
The total solid content of the HB and BH samples was 5.4 ± 1.1 wt% and 5.2 ± 0.7 wt% respectively. After centrifugation, as expected, the total solid content of the pellet from both samples increased to 8.4 ± 0.5 wt% and 8.3 ± 0.1 wt% for HB and BH, respectively. The average pH of the samples was 6.2 ± 0.1.
Rheological properties of simulated bolus
Shear viscosity.
Fig. 2 shows the shear viscosity of the simulated bolus with different concentrations of the dispersed phase. These results can be separated into two concentration regimes: diluted (<1% dispersed phase) and intermediate (≥1% dispersed phase) concentration. The presence of these concentration regimes in the simulated bolus samples has been previously described in suspensions of apple cell materials,20,21 where the authors also identified a third region in which a viscosity plateau was measured as the concentration increased. These three regions are commonly observed in suspensions of deformable particles. As can be seen from Fig. 2, the shear viscosity was higher for simulated boli with xanthan gum as the fluid phase, compared to those with water. At low concentrations (<1% dispersed phase), both HB and BH boli had similar viscosities, indicating that in the dilute regime the shear viscosity was dominated by the fluid phase. As the dispersed phase concentration increased to >1%, the effect of the dispersed phase started to have a significant effect. The shear viscosity started to increase exponentially with increasing concentration of dispersed phase, indicating the beginning of the intermediate concentrate region. HB boli showed higher viscosity than the BH boli at the same concentration, for both water and xanthan samples. The log–log plots of shear viscosity vs. concentration (wt%) in the intermediate concentration were fitted to a power law. The exponent n values for boli in water were 6.4 (R2 = 0.99) and 2.4 (R2 = 0.99) for HB and BH respectively, whereas in xanthan gum the n values were lower, 3.1 (R2 = 0.94) and 1.9 (R2 = 0.99) for HB and BH, respectively, indicating that the viscosity increased faster in HB water boli with increasing particle concentration. Exponents of 2.5–8 have been previously reported for suspensions of apple particles.20,21
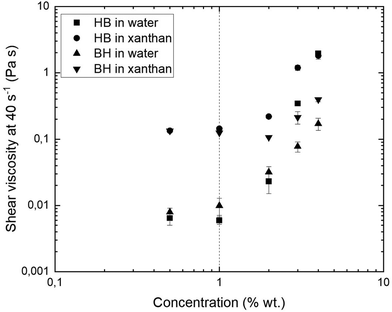 |
| Fig. 2 Shear viscosity as a function of concentration (wt%) in simulated bolus prepared with two types of dispersed phase, HB and BH, and two fluid phases, water and xanthan gum solution (0.5 wt%). Viscosity was selected at a shear rate of 40 s−1. Plots represent the average and the standard deviation of three measurements. The dashed line marks the limit between dilute and intermediate concentration regions. | |
The viscosity of a suspension depends on the viscosity of the fluid phase and the particle phase volume. Therefore, it is expected that the bolus containing xanthan as the fluid phase, and the bolus in which particles pack in a less efficient manner, would have higher viscosities. In this case, this behaviour corresponds to HB samples. In the dilute region, no particle network can be created, and the viscosity is dominated by the fluid phase. In the intermediate region, the dependency on the concentration is more pronounced in the HB particles than BH particles, probably due to the differences in morphology of the particles and how they pack together, but also due to the chemical composition of the particles and their interactions with artificial saliva. The viscosity was higher in xanthan than the water samples, but this difference seemed to be reduced as the total particle concentration increases (especially for HB samples) to 4 wt%. At this concentration the viscosity was dominated by the particles and not the fluid phase as both HB samples in water and xanthan showed similar shear viscosities. Hemar et al.8 reported similar effects in rehydrated cell wall particle mixtures suspended in xanthan solution. They found that at low particle concentration, the suspension behaved as a dispersion of cell particles in a fluid phase of xanthan and that the resulting rheological properties were dominated by xanthan. At high particle concentrations, the xanthan molecules were confined to the interstitial space and thus contributed only marginally to the rheological properties. In a food bolus, saliva can act as a lubricant or a binder for the food particles. The results from this study suggest that the addition of artificial saliva to the samples with water could have a particle binding effect whilst in samples with xanthan, that effect was less apparent.
Broccoli suspensions with a particle concentration of 6 wt% and added artificial saliva (corresponding to a bolus with 3 wt% particle concentration after dilution with artificial saliva) presented a macroscopic aspect of vegetable purees. Therefore, 6 wt% broccoli suspensions were selected for sensory evaluation and further rheological characterisation was carried out with a 3 wt% simulated bolus to represent a potential bolus in the mouth.
Fig. 3 shows shear viscosity vs. shear rate curves for boli at 3 wt% concentration of HB and BH particles suspended in water and xanthan solution. At this concentration the boli showed shear thinning behaviour, especially for samples containing xanthan solution as the fluid phase.22 Shear thinning behaviour has been proposed to be more appropriate for safe swallowing, because the flow of the bolus is reduced during swallowing, which allows time for the airway to be closed.
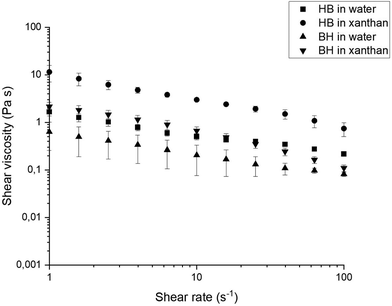 |
| Fig. 3 Shear viscosity as a function of shear rate for bolus containing 3 wt% total solids showing shear thinning behaviour. Values represent average of three measurements and standard deviation. | |
Viscoelasticity.
All boli at 3 wt% particle concentration showed a predominant solid-like behaviour G′ > G′′ (Table 1). The storage and loss moduli were lower in samples with water as the fluid phase than xanthan. Samples with water as the fluid phase had similar G′ and G′′ independent of the type of dispersed phase (Table 1). However, in boli with xanthan as the fluid phase, the dispersed phase had a significant effect with the BH dispersed phase sample showing higher viscoelastic moduli than HB samples (Table 1). These results indicate that the presence of xanthan in the boli led to a stronger and more elastic network, in particular in combination with a BH dispersed phase; suggesting that the binding effect of xanthan remains even after mixing with the artificial saliva. Parameters such as the particle shape, aspect ratio and surface roughness influence the particle packing and the interactions between particles, under rest and flow conditions, and determine rheological properties.23 The rheology of a dispersion of plant particles has both an elastic component and a viscous component.5,24 Viscous effects are due to the viscosity of the fluid phase (both its flow and lubrication between particles), as well as friction between particles which touch and slide over one another. The BH samples in xanthan had a higher G′′ value than HB samples, whilst in water both types of samples, BH and HB, showed similar viscous modulus. This suggests that in BH samples with xanthan additional friction between particles or particles and xanthan molecules could play a role. For the dispersions to display an elastic modulus, under rest, a percolating network of particles must exist and the particles must not be free to slide past one another under small strains, but instead must deform in order to accommodate the imposed strain.25 The interactions between particles can be of various forms, for example steric interactions (particles cannot overlap), electrostatic forces (attractive or repulsive), adhesion from attached (hydrated) polymer chains or van der Waals forces. Consider that the BH particles having rough surfaces with attached cell wall fragments (Fig. 1) would lead to an increase in this type of interaction increasing G′, compared to HB samples in which particles could overlap easier and adhesion forces will be less likely due to their smooth surfaces. Previous studies25 indicated that dispersions of plant particles, at low concentrations, are dominated by particle interactions due to asperities or to weak attractive interactions, which lower the critical particle packing fraction (other than steric repulsion of smooth, elastic bodies). As the concentration is increased above the elastic modulus will increase, and the particles are likely to be increasingly deformed, even when there is no imposed strain. It is also possible that the dominant interaction supporting the network may change. Interactions, via physical entanglements and perhaps other attractive forces such as electrostatic interactions, might play a role in the increase G′ of BH samples compared to HB samples. However, it should also be considered that the particle size and particle size distribution are different in both samples and therefore the combination of all these parameters will result in different rheological properties.
Table 1 Viscoelastic moduli obtained at 1 Hz and 0.1% strain and apparent yield stress of simulated bolus containing 3 wt% broccoli particles. Values represent the average of three measurements. Different letters in the same column indicate significant difference (Tukey’s test, p < 0.05)
|
Elastic modulus G′/Pa |
Viscous modulus G′′/Pa |
Apparent yield stress/Pa |
Heat and blend in water |
15.44 ± 3.40a |
1.78 ± 0.40a |
0.05 ± 0.01a |
Heat and blend in xanthan |
53.60 ± 10.83b |
13.90 ± 3.40b |
0.40 ± 0.11b |
Blend and heat in water |
12.08 ± 2.01a |
1.86 ± 0.37a |
0.03 ± 0.02a |
Blend and heat in xanthan |
354.52 ± 74.01c |
76.96 ± 11.34c |
0.74 ± 0.25b |
Extensional viscosity.
Boli with water as the fluid phase showed no detectable extensional viscosity, independently of the type of dispersed phase, whilst both HB and BH samples with xanthan as the fluid phase showed extensional viscosity. Hence Fig. 4 only shows the extensional viscosity of simulated boli containing xanthan solution as the fluid phase with a particle concentration of 3 wt%. The observed extensional viscosity in the boli containing xanthan is likely due to the alignment of xanthan molecules in extensional flow. Both HB and BH boli showed extensional-thinning behaviour over the extensional strain rates used. The extensional viscosity values for HB and BH boli were similar; however, at low extensional rates HB showed slightly higher extensional viscosity compared to BH. The stretchability of a bolus could play an important role in triggering swallowing and has been previously shown to be correlated with ease of swallowing of different food systems.2
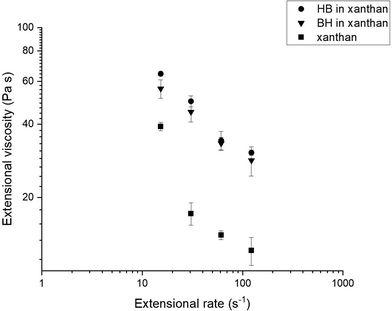 |
| Fig. 4 Extensional viscosity as a function of the extension rate for a 3 wt% concentration boli made from different processing methods and xanthan as the fluid phase. Extensional viscosity of a simulated bolus containing 0.5 wt% xanthan gum mixed with artificial saliva to a final concentration of 0.25 wt% xanthan is used as a reference. Values represent average and standard deviation of three measurements. | |
Apparent yield stress.
Apparent yield stress values for each of the boli are summarised in Table 1. At 3 wt% all boli showed a very low apparent yield stress with a high standard deviation, most likely due to the heterogeneity of the boli. Boli with xanthan gum as the fluid phase showed an apparent yield stress one order of magnitude higher than boli with water. Apparent yield stress values of HB and BH boli with xanthan as the fluid phase were 0.40 ± 0.11 Pa and 0.74 ± 0.25 Pa, respectively. These values are lower than those previously reported for xanthan solutions.6 The yield stress of 0.3 wt% xanthan has been reported to be 1.5 Pa.6 However, in this study, the yield stress of 0.25 wt% xanthan with saliva was 0.19 ± 0.05 Pa, and for the boli with xanthan ranged from 0.4–0.7 Pa. The lower values obtained in this study could be due to a number of reasons: difference in the methodology used to obtain the apparent yield stress data, different types of xanthan and the presence of ions in the saliva which could have interacted with xanthan molecules. The yield stress is the stress at which flow begins and is related to the degree of the internal binding force and the structural order. Particle properties such as size, size distribution, shape, surface roughness and charge all impact the packing of the particles, resulting in the presence of an apparent yield stress.26 Therefore, the yield stress can be regarded as a measure of the cohesiveness of a bolus and has been related to ease of swallowing.27 Despite the low yield stress values, our results suggest that broccoli purees prepared with xanthan could be perceived as easier to swallow, as they have higher yield stress/cohesiveness in the bolus.
Cohesiveness.
In this research cohesiveness refers to the internal strength of compacted material and is linked to flowability by the degree of deformation and destruction of the material when mechanical load is applied.6,14 Subsequently a highly cohesive material would be more difficult to flow. Cohesiveness was measured objectively using a ring shear tester, a method commonly used for powders which has been previously shown to be able to measure flowability of particulated foods at different moisture contents.14 However, this method has limitations in high moisture food systems. Due to this limitation cohesiveness of samples with solid concentrations below 8 wt% could not be measured, as there was phase separation, i.e. separation of the fluid phase and broccoli particles, during the consolidation of the sample. As can be seen from Fig. 5, all 8 wt% broccoli suspensions were very cohesive or cohesive. BH samples showed higher cohesiveness than HB samples at all the applied consolidation stresses. In HB samples the fluid phase did not significantly influence cohesiveness; however, in BH samples, addition of xanthan seemed to increase the cohesiveness in comparison with the water samples. This behaviour results from different interactions between the broccoli particles and the fluid phase. This is because the fluid phase can act as a binder or a lubricant depending on the amount added. These results suggest that the properties of BH particles (larger in size and with broken cell walls on the surface) could be the reason for higher cohesiveness in BH samples compared to HB (smaller particles with smooth surfaces) samples. This observation supports the yield stress and moduli data (Table 1), where BH boli had a higher yield stress than HB boli, with xanthan as the fluid phase in both samples. However, in food samples, the dispersed phase seemed to be the determinant factor for cohesiveness and not the fluid phase. Correlation between yield stress and cohesiveness has already been suggested by Ross et al.28 for thickened fluids. This is because a cohesive bolus does not fracture during swallow27 and can be consumed easily. Mechanical cohesiveness is a synonym of the internal binding force that contributes to the formation of a coherent bolus. Hence, the relationship between objective measure of cohesiveness and yield stress to sensory perception of ease of swallowing needs to be investigated further.
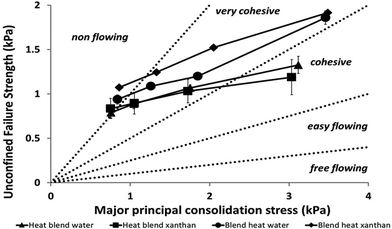 |
| Fig. 5 Flowability regions for broccoli suspensions with 8 wt% solids and different fluid phases. Results represent average and standard deviation. | |
Sensory evaluation
Table 2 summarises the results from the sensory evaluation of the broccoli purees. The fluid phase (water or xanthan) had a greater influence on the perception of ease of swallowing than the dispersed phase (HB vs. BH). Samples with xanthan as the fluid phase were perceived as less difficult to swallow i.e. easier to swallow and required less effort to prepare to swallow than those with water. However, the dispersed phase did impact the swallowing perception, as HB samples were easier to swallow and required less effort to prepare the bolus for swallowing than BH suspensions regardless of whether a thickener was added or not. This could be related to the fact that HB samples were perceived to have a smaller particle size, which is in agreement with the particle size measurements (Fig. 1). The type of dispersed phase had an important impact on the perceived particle size. HB samples were perceived as having a small particle size compared to BH samples. Previous studies have shown that concentrated plant-based suspensions with the softest and smallest particles lead to smooth textures;29 however, in our study the panellists were not able to perceive significant differences in smoothness. This could be because the particle size ranged from 30 to 400 μm in the previous study, whereas in this study the size range was narrower (200 to 400 μm). Furthermore, in the previous study the average age of the panel was 48 years and in our study the panellists were older with an average age of 71 years. In general all the samples were rated in the middle-upper part of the smoothness scale, suggesting that dispersion of plant particles with sizes in the range of 200 to 400 μm is not perceived as grainy, which is in agreement with the conclusions from the study by Appelqvist et al.29
Table 2 Mean scores of sensory attributes using a 5 points categorization scale, where 1 = low and 5 = high. Different letters in the same column indicate significant differences (Tukey’s test, p < 0.05)
|
Difficulty in swallowing |
Effort required to prepare to swallow |
Residues in-mouth |
Mouth-coating |
Particle size perception |
Smoothness |
Heat and blend in water |
2.95ab |
2.11ab |
2.53b |
3.21ab |
1.79b |
3.21a |
Heat and blend in xanthan |
1.90c |
1.74b |
2.26b |
2.42b |
1.47b |
3.32a |
Blend and heat in water |
3.68a |
3.05a |
3.79a |
3.68a |
3.21a |
2.47a |
Blend and heat in xanthan |
2.37bc |
2.05b |
2.47b |
2.68b |
2.11b |
2.74a |
Samples with xanthan as fluid phase left fewer residues in the mouth and were less mouth coating compared to samples with water; this was especially perceived in the case of BH samples. In general, samples prepared with xanthan as the fluid phase were perceived very similarly to each other, independently of the type of dispersed phase. It is worth noting that the panellist could discriminate among the samples with different fluid phase but not among the dispersed phase within the xanthan samples, except for the ease of swallowing.
The PLS model was built introducing the sensory attributes as X variables and the rheological parameters as Y variables. As illustrated in Fig. 6A, the distribution of the samples along F1 (94.1%) is driven by the type of fluid used whereas the type of processing drives F2 (5.27%). HCA analysis unveiled two different groups in which BH in water was found to be different from the other three samples (HB water, BH xanthan and HB xanthan). These results are also strongly supported by the statistical differences in individual sensory attributes shown in Table 2. Thus, although the final characteristics of the samples are driven by the fluid phase, their perception was the result of the combination of the disperse and the fluid phase.
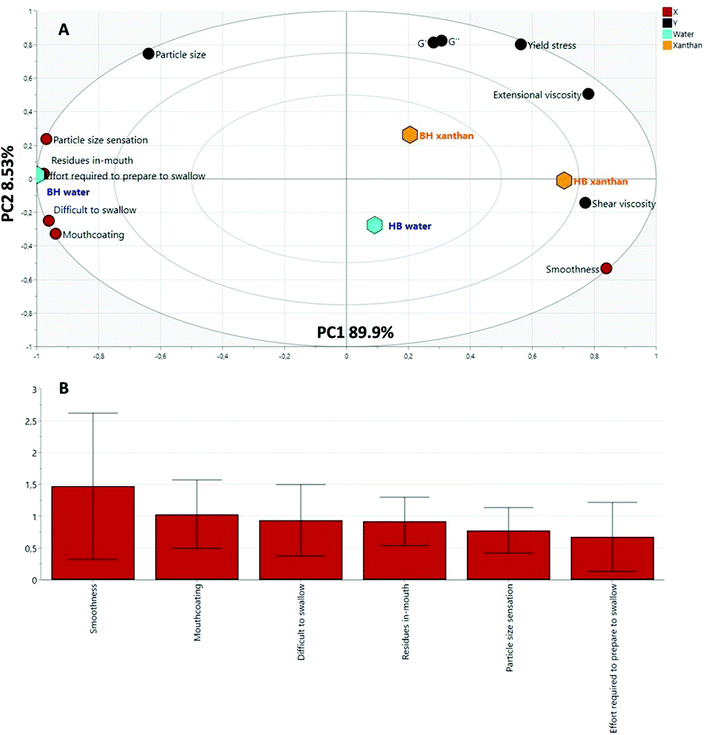 |
| Fig. 6 (A) PLS bi-plot representing the distribution of the broccoli suspensions (HB water, HB xanthan, BH Water, BH xanthan) according to the relationship between sensory attributes and rheological properties. (B) VIP plot for the corresponding PLS model. | |
To assess which variables better describe the entire PLS model, a Variable Importance in Projection (VIP) plot (Fig. 6B) was generated. The VIP plot highlights the most important X variables (sensory attributes) describing the difference between samples in relation with Y variables (rheological parameters). Residues in mouth and particle size perception were found to be the sensory parameters to better represent the model. The perception of residues in mouth showed correlation with the particle size (0.61). Therefore, the larger the particle size, the greater the perception of residues in mouth. Mouth-coating showed a negative correlation with the extensional viscosity. The lower the extensional viscosity of the sample, the higher the mouth-coating sensation as it occurs for samples with water as a fluid phase. The more cohesive nature of the samples with xanthan would reduce the coating of oral surfaces.
Difficulty in swallowing had a high negative correlation with yield stress (−0.71) and especially with extensional viscosity (−0.92). This indicates that the higher the yield stress and extensional viscosity, the easier it is to swallow a bolus, as it was observed for xanthan samples. Furthermore, the higher the extensional viscosity and shear viscosity of the sample, the lower the effort required to swallow (−0.73 and −0.72 respectively). More detailed information regarding the relationship between the different variables is described on the correlation matrix (ESI Table S1†).
Conclusions
In this research we have investigated the contribution of the dispersed and fluid phase to the ease of swallowing of particulated foods during oral processing. We used broccoli suspensions as model systems and a food bolus was mimicked by mixing suspensions with artificial saliva. Our results indicate that the fluid phase has the highest impact on the perception of ease of swallowing, compared to the type of dispersed phase. Although the mouthfeel and ease of swallowing perceived during oral processing were driven by the fluid phase, they were the result of the combination of both the disperse and the fluid phase. This is likely due not only to the properties of the fluid phase itself, but also to how it interacts with the particles and affects the way they pack together in the bolus, leading to specific rheological properties. These findings support the hypothesis that the presence of yield stress and extensibility in the bolus, achieved by addition of xanthan as fluid phase, leads to particulated foods perceived as easy to swallow. In addition, suspensions with a broader particle size distribution and smaller particle size were easier to swallow, with good agreement between particle size measurements and particle size perception by the panel. The results highlight the importance of understanding the role of fluids and dispersed phase of complex food systems to design personalised foods with optimal sensory properties for specific populations such as those suffering from swallowing disorders.
Funding
This work was supported by the Swedish Research Council Formas (2018-01346).
Conflicts of interest
There are no conflicts to declare.
Acknowledgements
Lisa Maria Oberrauter is kindly acknowledged for assistance with sensory evaluations. Jan-Willems Benjamin and Lubica Macakova are acknowledged for particle size measurements. Mats Stading is gratefully acknowledged for useful discussions.
References
- C. M. Steele, W. A. Alsanei, S. Ayanikalath, C. E. Barbon, J. Chen, J. A. Cichero, K. Coutts, R. O. Dantas, J. Duivestein, L. Giosa, B. Hanson, P. Lam, C. Lecko, C. Leigh, A. Nagy, A. M. Namasivayam, W. V. Nascimento, I. Odendaal, C. H. Smith and H. Wang, The influence of food texture and liquid consistency modification on swallowing physiology and function: a systematic review, Dysphagia, 2015, 30, 2–26 CrossRef.
- J. Chen and L. Lolivret, The determining role of bolus rheology in triggering a swallowing, Food Hydrocolloids, 2011, 25, 325–332 CrossRef CAS.
- M. Nystrom, W. M. Qazi, M. Bulow, O. Ekberg and M. Stading, Effects of rheological factors on perceived ease of swallowing, Appl. Rheol., 2015, 25, 40–48 Search PubMed.
- K. R. N. Moelants, R. Cardinaels, S. Van Buggenhout, A. M. Van Loey, P. Moldenaers and M. E. Hendrickx, A Review on the Relationships between Processing, Food Structure, and Rheological Properties of Plant-Tissue-Based Food Suspensions, Compr. Rev. Food Sci. Food Saf., 2014, 13, 241–260 CrossRef CAS.
- P. Lopez-Sanchez, J. Nijsse, H. C. Blonk, L. Bialek, S. Schumm and M. Langton, Effect of mechanical and thermal treatments on the microstructure and rheological properties of carrot, broccoli and tomato dispersions, J. Sci. Food Agric., 2011, 91, 207–217 CrossRef CAS.
- M. Nakauma, S. Ishihara, T. Funami and K. Nishinari, Swallowing profiles of food polysaccharide solutions with different flow behaviors, Food Hydrocolloids, 2011, 25, 1165–1173 CrossRef CAS.
- I. T. Norton, D. M. Goodall, S. A. Frangou, E. R. Morris and D. A. Rees, Mechanism and dynamics of conformational ordering in xanthan polysaccharide, J. Mol. Biol., 1984, 175, 371–394 CrossRef CAS.
- Y. Hemar, S. Lebreton, M. Xu and L. Day, Small-deformation rheology investigation of rehydrated cell wall particles–xanthan mixtures, Food Hydrocolloids, 2011, 25, 668–676 CrossRef CAS.
- E. K. Hadde and J. Chen, Shear and extensional rheological characterization of thickened fluid for dysphagia management, J. Food Eng., 2019, 245, 18–23 CrossRef.
- M. Minekus, M. Alminger, P. Alvito, S. Ballance, T. Bohn, C. Bourlieu, F. Carriere, R. Boutrou, M. Corredig, D. Dupont, C. Dufour, L. Egger, M. Golding, S. Karakaya, B. Kirkhus, S. Le Feunteun, U. Lesmes, A. Macierzanka, A. Mackie, S. Marze, D. J. McClements, O. Menard, I. Recio, C. N. Santos, R. P. Singh, G. E. Vegarud, M. S. Wickham, W. Weitschies and A. Brodkorb, A standardised static in vitro digestion method suitable for food - an international consensus, Food Funct., 2014, 5, 1113–1124 RSC.
- M. R. C. Marques, R. Loebenberg and M. Almukainzi, Simulated Biological Fluids with Possible Application in Dissolution Testing, Dissolution Technol., 2011, 18, 15–28 CrossRef CAS.
- M. Nystrom, H. R. T. Jahromi, M. Stading and M. F. Webster, Hyperbolic contraction measuring systems for extensional flow, Mech. Time-Depend. Mater., 2017, 21, 455–479 CrossRef.
- K. Wikstrom and L. Bohlin, Extensional flow studies of wheat flour dough. I. Experimental method for measurements in contraction flow geometry and application to flours varying in breadmaking performance, J. Cereal Sci., 1999, 29, 217–226 CrossRef.
- A. B. Tobin, P. Heunemann, J. Wemmer, J. R. Stokes, T. Nicholson, E. J. Windhab and P. Fischer, Cohesiveness and flowability of particulated solid and semi-solid food systems, Food Funct., 2017, 8, 3647–3653 RSC.
- K. Slettengren, E. Xanthakis, L. Ahrne and E. J. Windhab, Flow Properties of Spices Measured with Powder Flow Tester and Ring Shear Tester-XS, Int. J. Food Prop., 2016, 19, 1475–1482 CrossRef.
- M. Sharma, E. Kristo, M. Corredig and L. Duizer, Effect of hydrocolloid type on texture of pureed carrots: Rheological and sensory measures, Food Hydrocolloids, 2017, 63, 478–487 CrossRef CAS.
- J. J. X. Ong, C. M. Steele and L. M. Duizer, Challenges to assumptions regarding oral shear rate during oral processing and swallowing based on sensory testing with thickened liquids, Food Hydrocolloids, 2018, 84, 173–180 CrossRef CAS.
- Z. Vickers, H. Damodhar, C. Grummer, H. Mendenhall, K. Banaszynski, R. Hartel, J. Hind, A. Joyce, A. Kaufman and J. Robbins, Relationships Among Rheological, Sensory Texture, and Swallowing Pressure Measurements of Hydrocolloid-Thickened Fluids, Dysphagia, 2015, 30, 702–713 CrossRef CAS.
-
A. Tobin, Insights into Rational Design of Plant Based Foods to Improve Management of Dysphagia, PhD Thesis, The University of Queensland, 2014, DOI:10.14264/uql.2015.160.
- C. Leverrier, G. Almeida, L. Espinosa-Munoz and G. Cuvelier, Influence of Particle Size and Concentration on Rheological Behaviour of Reconstituted Apple Purees, Food Biophys., 2016, 11, 235–247 CrossRef.
- C. Leverrier, G. Almeida, P. Menut and G. Cuvelier, Design of Model Apple Cells Suspensions: Rheological Properties and Impact of the Continuous Phase, Food Biophys., 2017, 12, 383–396 CrossRef.
- N. Vilardell, L. Rofes, V. Arreola, R. Speyer and P. Clave, A Comparative Study Between Modified Starch and Xanthan Gum Thickeners in Post-Stroke Oropharyngeal Dysphagia, Dysphagia, 2016, 31, 169–179 CrossRef CAS.
- D. B. Genovese, J. E. Lozano and M. A. Rao, The Rheology of Colloidal and Noncolloidal Food Dispersions, J. Food Sci., 2007, 72, R11–R20 CrossRef CAS.
- L. Day, M. Xu, S. K. Øiseth, L. Lundin and Y. Hemar, Dynamic rheological properties of plant cell-wall particle dispersions, Colloids Surf., B, 2010, 81, 461–467 CrossRef CAS.
- P. Lopez-Sanchez, V. Chapara, S. Schumm and R. Farr, Shear Elastic Deformation and Particle Packing in Plant Cell Dispersions, Food Biophys., 2012, 7, 1–14 CrossRef.
-
C. Macosco, Rheology:principles,measurements and applications, VCH publishers, New York, 1993 Search PubMed.
- K. Nishinari, M. Turcanu, M. Nakauma and Y. Fang, Role of fluid cohesiveness in safe swallowing, Sci. Food, 2019, 3, 5 Search PubMed.
- A. I. V. Ross, P. Tyler, M. G. Borgognone and B. M. Eriksen, Relationships between shear rheology and sensory attributes of hydrocolloid-thickened fluids designed to compensate for impairments in oral manipulation and swallowing, J. Food Eng., 2019, 263, 123–131 CrossRef CAS.
- I. A. M. Appelqvist, M. Cochet-Broch, A. A. M. Poelman and L. Day, Morphologies, volume fraction and viscosity of cell wall particle dispersions particle related to sensory perception, Food Hydrocolloids, 2015, 44, 198–207 CrossRef CAS.
Footnote |
† Electronic supplementary information (ESI) available. See DOI: 10.1039/d0fo01728k |
|
This journal is © The Royal Society of Chemistry 2020 |